What is Eukaryotic Cell?
- Eukaryotic cells, derived from the domain Eukaryota, are distinguished by the presence of a well-defined, membrane-bound nucleus. This characteristic sets them apart from prokaryotic cells, which lack a true nucleus and are primarily found within the domains Archaea and Bacteria.
- The term “eukaryote” is etymologically rooted in the Greek words “eu”, signifying “true”, and “karuon”, denoting “nut”, alluding to the prominent nucleus within these cells.
- Eukaryotic organisms encompass a diverse range of life forms, including animals, plants, fungi, and many unicellular entities. While eukaryotes represent a numerical minority in the vast spectrum of organisms, their larger size ensures that their collective biomass surpasses that of prokaryotes.
- The genesis of eukaryotes is a topic of significant scientific interest. Current evidence suggests that eukaryotes originated within the Asgard archaea, approximately 2.2 billion years ago during the Proterozoic eon.
- This emergence is believed to have been facilitated by a symbiogenetic event between an anaerobic Asgard archaean and an aerobic proteobacterium, leading to the formation of mitochondria. A subsequent symbiogenetic interaction with a cyanobacterium gave rise to plants, characterized by chloroplasts. The discovery of the Gabonionta fossils in Gabon in 2023, which are multicellular planktonic eukaryotes dating back to 2.1 billion years ago, further underscores the ancient lineage of eukaryotes.
- Structurally, eukaryotic cells are complex and compartmentalized, housing a variety of membrane-bound organelles such as the endoplasmic reticulum, Golgi apparatus, and mitochondria. This intricate organization facilitates specialized functions and metabolic processes.
- Eukaryotic organisms can be unicellular, often referred to as protists, or multicellular, with the latter category including entities like humans, wherein a myriad of cell types collaboratively function to form tissues, organs, and systems. For instance, muscle cells in humans, specialized in form and function, constitute the muscular tissues and systems, enabling movement.
- Reproductively, eukaryotes exhibit versatility, capable of both asexual reproduction via mitosis and sexual reproduction through meiosis, culminating in gamete fusion or fertilization.
- In summary, eukaryotic cells are the foundational units of a diverse array of life forms, characterized by a membrane-bound nucleus and a complex internal structure. Their evolutionary history, intricate cellular organization, and reproductive capabilities make them a pivotal subject of study in the realm of biology.
Definition of Eukaryotic Cell
A eukaryotic cell is a type of cell characterized by a membrane-bound nucleus and the presence of various organelles within its cytoplasm, distinguishing it from prokaryotic cells which lack a true nucleus. Eukaryotic cells are found in organisms such as plants, animals, fungi, and many unicellular entities.
Characteristics of a Eukaryotic Cell
Eukaryotic cells, distinct from their prokaryotic counterparts, exhibit a range of specialized features that underpin their complex functions and roles in multicellular organisms. The following are the salient characteristics of eukaryotic cells:
- Nuclear Membrane: One of the defining features of a eukaryotic cell is the presence of a nucleus that is enveloped by a nuclear membrane. This compartmentalization ensures the protection and regulation of the cell’s genetic material.
- Mitochondria: Eukaryotic cells are equipped with mitochondria, the organelles responsible for energy production through the process of cellular respiration.
- Locomotory Organelles: For movement, eukaryotic cells may possess flagella or cilia. These structures facilitate cellular movement and play roles in processes like signal reception and fluid movement.
- Cell Wall: While not universal to all eukaryotic cells, many, especially plant cells, have a cell wall. This rigid structure provides support, protection, and shape to the cell.
- Cell Division: Eukaryotic cells undergo a specialized form of cell division known as mitosis, ensuring the proper segregation of genetic material into daughter cells.
- Cytoskeletal Structure: Eukaryotic cells contain a cytoskeleton, a network of protein filaments and tubules. This structure provides mechanical support, aids in intracellular transport, and plays a pivotal role in cell division.
- Linear DNA: The nucleus of eukaryotic cells houses linear DNA, which carries the entirety of the cell’s genetic information. This DNA is organized into chromosomes, ensuring efficient replication and transcription processes.
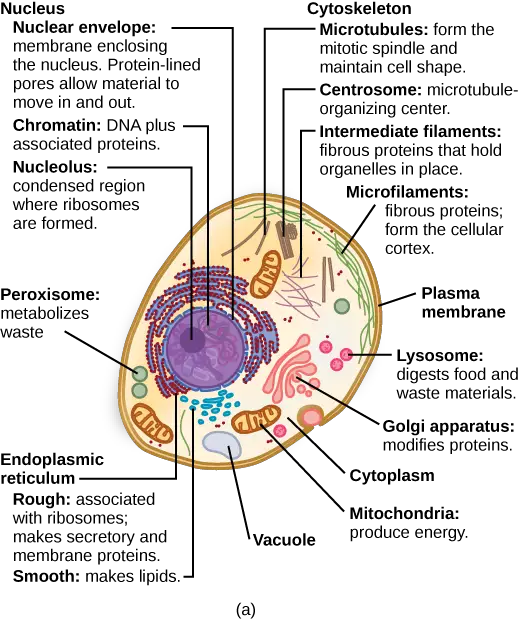
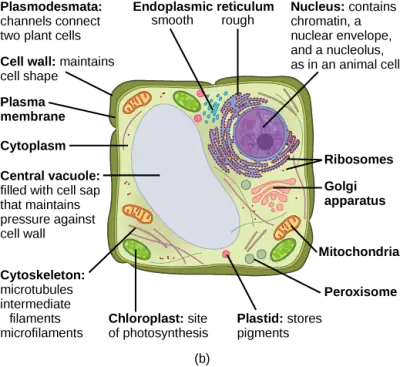
Internal Organelles of Eukaryotic Cell
Eukaryotic cells, in contrast to their prokaryotic counterparts, are characterized by a suite of specialized structures that facilitate a myriad of cellular functions. These structures, both membrane-bound organelles and non-membrane-bound entities, contribute to the cell’s complexity and functionality. Here’s a closer look at these integral components:
- Nucleus:
- The nucleus serves as the cell’s command center, housing the genetic material (DNA). It is enveloped by a double-membrane structure known as the nuclear envelope, which regulates the exchange of substances between the nucleus and the cytoplasm.
- Ribosomes:
- These non-membrane-bound structures are the sites of protein synthesis. Comprising RNA and proteins, ribosomes can be found either freely floating in the cytoplasm or attached to the endoplasmic reticulum.
- Endoplasmic Reticulum (ER):
- The ER is a vast membranous network responsible for various cellular functions. The rough ER, studded with ribosomes, is involved in protein synthesis, while the smooth ER plays roles in lipid synthesis and detoxification processes.
- Golgi Apparatus:
- Often likened to the cell’s post office, the Golgi apparatus modifies, sorts, and packages proteins and lipids for transport to their destined locations within or outside the cell.
- Lysosomes:
- These membrane-bound vesicles contain digestive enzymes that break down waste materials, cellular debris, and foreign invaders, ensuring cellular homeostasis.
- Vacuoles:
- Vacuoles are storage organelles, often prominent in plant cells. They store nutrients, waste products, and, in plants, contribute to turgor pressure.
- Endosomes:
- These membrane-bound vesicles are involved in the sorting and recycling of cellular materials, playing a pivotal role in the endocytic pathway.
- Cytoskeleton:
- A complex network of protein filaments, the cytoskeleton provides structural support, facilitates cellular movement, and plays a role in intracellular transport.
- Plastids:
- Mitochondria:
- Often termed the “powerhouses” of the cell, mitochondria are responsible for producing adenosine triphosphate (ATP), the cell’s primary energy currency, through the process of cellular respiration.
In essence, the intricate architecture of eukaryotic cells, underscored by these structures, enables them to execute a vast array of functions, ensuring the survival and thriving of the organism they constitute.
Structure of Eukaryotic Cell Cycle
1. The Plasma Membrane
The plasma membrane is a fundamental component of both prokaryotic and eukaryotic cells, acting as a selective barrier that delineates the cell’s internal environment from the external milieu. Structurally, the plasma membrane is composed of a phospholipid bilayer interspersed with embedded proteins.
- Phospholipid Bilayer:
- Each phospholipid molecule consists of a glycerol backbone, two fatty acid chains, and a phosphate group. The hydrophilic (water-attracting) phosphate heads face outward, interacting with the aqueous environment, while the hydrophobic (water-repelling) fatty acid tails orient inward, creating a semi-permeable barrier.
- Functionality:
- The plasma membrane plays a pivotal role in regulating the transport of substances. While some molecules, such as ions, organic compounds, and water, traverse the membrane through passive diffusion, others require active transport mechanisms. The membrane’s selective permeability ensures the maintenance of the cell’s homeostasis by controlling the influx and efflux of molecules.
- Microvilli:
- In certain specialized cells, the plasma membrane extends into minute, finger-like projections termed microvilli. These structures amplify the surface area of the membrane, optimizing absorption. A quintessential example of this adaptation is observed in the cells lining the small intestine, which are responsible for nutrient uptake from digested food.
- Clinical Relevance:
- Celiac disease exemplifies the significance of the plasma membrane’s structure to its function. In individuals with this condition, an immune response is triggered against gluten, a protein present in wheat, barley, and rye. This immune reaction damages the microvilli, impairing nutrient absorption. Consequently, affected individuals experience symptoms like malnutrition, abdominal cramping, and diarrhea. The primary therapeutic intervention for celiac disease is adherence to a gluten-free diet.
In summation, the plasma membrane is not merely a passive barrier but a dynamic structure that actively modulates the cell’s interactions with its environment, ensuring its survival and functionality.
2. The Cytoplasm
The cytoplasm is a fundamental component of both plant and animal cells, serving as the milieu in which a plethora of cellular activities transpire. Positioned between the plasma membrane and the nuclear envelope, the cytoplasm is a dynamic environment that plays a pivotal role in cellular metabolism and function.
- Composition:
- The cytoplasm is predominantly aqueous, with water constituting approximately 70 to 80 percent of its volume. Despite its high water content, the cytoplasm exhibits a semi-solid consistency, a characteristic attributed to the proteins dispersed within this matrix.
- Beyond proteins, the cytoplasm is a reservoir of various organic molecules. This includes glucose, polysaccharides, amino acids, nucleic acids, fatty acids, and glycerol derivatives. Additionally, it houses a myriad of ions, such as sodium, potassium, and calcium, which play crucial roles in cellular processes.
- Functionality:
- The cytoplasm is not merely a passive filler of the cellular space. It is the site of numerous metabolic reactions, including the synthesis of proteins. The gel-like cytosol, a component of the cytoplasm, provides a medium in which organelles are suspended and metabolic reactions occur.
- Embedded within the cytoplasm is the cytoskeleton, a structural framework that maintains cellular shape, facilitates intracellular transport, and anchors organelles in place.
- Organelles and Structures:
- The cytoplasm houses various organelles, each specialized for specific cellular functions. These organelles, enveloped in the cytosol, orchestrate a range of activities, from energy production to waste disposal.
- Significance:
- The cytoplasm is indispensable for cellular life. It provides a conducive environment for enzymatic reactions, facilitates the transport of molecules, and ensures that organelles function in harmony. Its composition and characteristics are tailored to support and regulate the myriad processes that ensure the cell’s survival and functionality.
In essence, the cytoplasm is a dynamic and complex environment, integral to the cell’s structure and function. It is a testament to the intricate orchestration of molecules and structures that enable the myriad processes essential for cellular life.
3. The Cytoskeleton
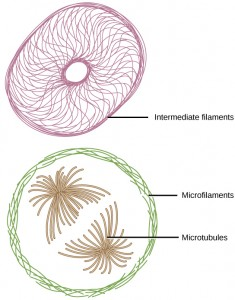
The cytoskeleton is a sophisticated network of protein fibers that permeates the cytoplasm, providing structural integrity and facilitating various cellular functions. Beyond merely maintaining cellular shape, the cytoskeleton plays a pivotal role in a multitude of cellular processes.
- Composition and Types:
- The cytoskeleton comprises three primary categories of fibers:
- Microfilaments (Actin Filaments): These are the most slender fibers within the cytoskeleton. They play crucial roles in cellular movement, especially during cell division. Additionally, they uphold the structure of microvilli, specialized membrane protrusions in cells dedicated to absorption. Notably, microfilaments are integral to muscle cells, driving muscle contraction.
- Intermediate Filaments: These fibers possess an intermediate thickness and primarily serve structural purposes. They stabilize the cell’s shape and anchor organelles. One notable type of intermediate filament is keratin, the protein that fortifies hair and nails.
- Microtubules: These are the most robust fibers of the cytoskeleton. Characterized as hollow tubes, microtubules can rapidly assemble and disassemble. They direct organelle movement and are instrumental during cell division, guiding chromosome segregation. Furthermore, microtubules form the structural foundation of flagella and cilia. In these structures, microtubules are arranged in a specific pattern: nine doublets encircle two central microtubules.
- The cytoskeleton comprises three primary categories of fibers:
- Centrosome and Centrioles:
- The centrosome, situated proximal to the nucleus in animal cells, acts as a hub for microtubule organization. Within the centrosome are two centrioles, cylindrical structures oriented perpendicularly to each other, each composed of nine microtubule triplets.
- While the centrosome duplicates prior to cell division, the precise role of centrioles during this process remains a subject of study. Intriguingly, even in the absence of centrioles, some cells can undergo division. Moreover, plant cells, which inherently lack centrioles, can proficiently divide.
- Flagella and Cilia:
- Flagella: These elongated, whip-like appendages extend from the plasma membrane, propelling cells. Cells may possess a singular flagellum or a few, as seen in sperm cells and certain protists like Euglena.
- Cilia: Contrasting flagella, cilia are shorter and more numerous, covering extensive regions of the plasma membrane. They can propel cells or facilitate the movement of substances along the cell’s exterior. For instance, cilia in the respiratory tract transport mucus-trapped particulates, while those in the fallopian tubes guide the ovum.
In summation, the cytoskeleton is an intricate and dynamic framework that underpins cellular structure and function. Its multifaceted roles, from providing mechanical support to facilitating movement, underscore its indispensability in cellular biology.
4. The Nucleus
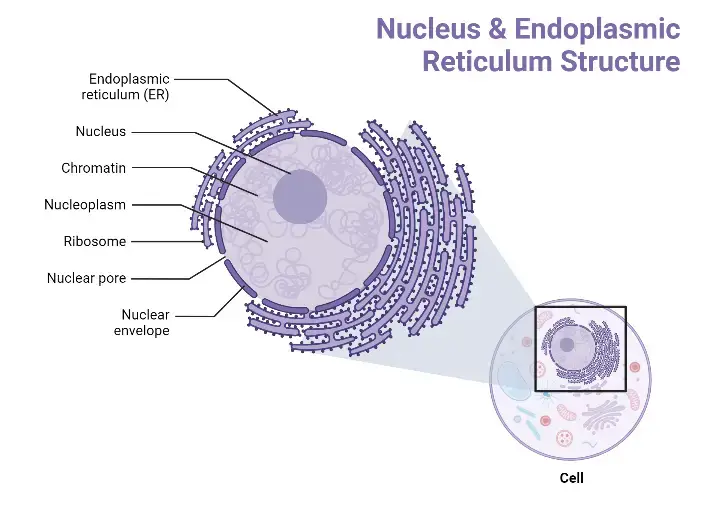
The nucleus, typically the most conspicuous organelle within a cell, serves as the genetic control center. It safeguards the cell’s DNA and orchestrates the synthesis of proteins and ribosomes.
- Nuclear Envelope:
- Encasing the nucleus is the nuclear envelope, a dual-membrane structure. Both its inner and outer layers are composed of phospholipid bilayers.
- This envelope is interspersed with nuclear pores, which regulate the transit of ions, molecules, and RNA between the nucleoplasm (the semi-solid substance within the nucleus) and the cytoplasm.
- Chromatin and Chromosomes:
- DNA within the nucleus is organized into structures known as chromosomes. These linear entities, unique to eukaryotes, vary in number across species. For instance, humans possess 46 chromosomes, while fruit flies have eight.
- When a cell is not dividing, these chromosomes are not distinctly visible. Instead, they appear as a tangle of threads, a state termed chromatin. Chromatin is essentially a combination of DNA and proteins. It represents both the condensed and decondensed forms of chromosomes, ensuring that the genetic material remains organized and accessible for cellular processes.
- The Nucleolus:
- Situated within the nucleus is a distinct, darker region known as the nucleolus. This structure plays a pivotal role in ribosome synthesis.
- Certain chromosomal regions encode ribosomal RNA (rRNA). Within the nucleolus, this rRNA converges with specific proteins to form ribosomal subunits. Once assembled, these subunits traverse the nuclear pores to reach the cytoplasm, where they participate in protein synthesis.
In essence, the nucleus is not just a storage vault for DNA; it is a dynamic entity where critical cellular processes are initiated and regulated. Its intricate structures and their functions underscore the nucleus’s paramount importance in maintaining cellular health and functionality.
5. The Endoplasmic Reticulum
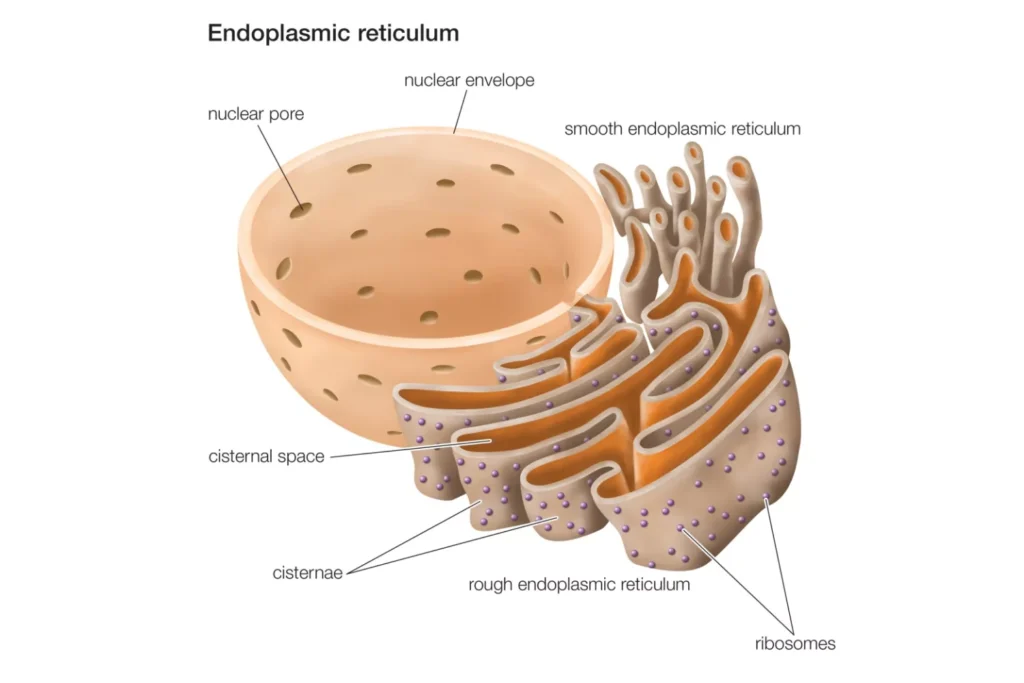
The endoplasmic reticulum (ER) stands as a pivotal organelle within eukaryotic cells, characterized by its intricate network of membranous tubules. This labyrinthine structure is instrumental in both protein modification and lipid synthesis, though these processes occur in distinct regions of the ER.
- Structural Overview:
- The ER’s internal cavity, termed the lumen or cisternal space, is enveloped by a phospholipid bilayer studded with proteins. Notably, this membrane seamlessly extends from the nuclear envelope, underscoring the interconnectedness of cellular components.
- Rough Endoplasmic Reticulum (RER):
- The RER derives its nomenclature from its “rough” appearance under electron microscopy, attributed to the ribosomes dotting its cytoplasmic surface.
- These ribosomes are the sites of protein synthesis. Once synthesized, proteins are ushered into the RER lumen, where they undergo various modifications, including folding and glycosylation.
- Additionally, the RER plays a pivotal role in phospholipid synthesis, essential for crafting cellular membranes.
- Proteins and phospholipids not retained within the RER are encapsulated in vesicles, which bud off from the RER, facilitating their transport to other cellular destinations.
- Given its central role in protein secretion, the RER is especially abundant in cells with a high secretory function, such as hepatocytes in the liver.
- Smooth Endoplasmic Reticulum (SER):
- The SER, devoid of ribosomes on its surface, presents a “smooth” appearance. It is an extension of the RER but serves distinct functions.
- The SER is a hub for carbohydrate synthesis and lipid metabolism, including the production of phospholipids and steroid hormones.
- Additionally, the SER is integral to the detoxification of certain toxins, drugs, and poisons. It plays a role in alcohol metabolism, rendering it crucial in cells exposed to such substances.
- Another vital function of the SER is the storage of calcium ions, which are pivotal for various cellular signaling pathways.
In essence, the endoplasmic reticulum, with its rough and smooth domains, serves as a multifunctional platform in the cell, orchestrating synthesis, modification, and transport of vital biomolecules. Its intricate structure and diverse functions underscore its indispensable role in maintaining cellular homeostasis and functionality.
6. The Golgi Apparatus
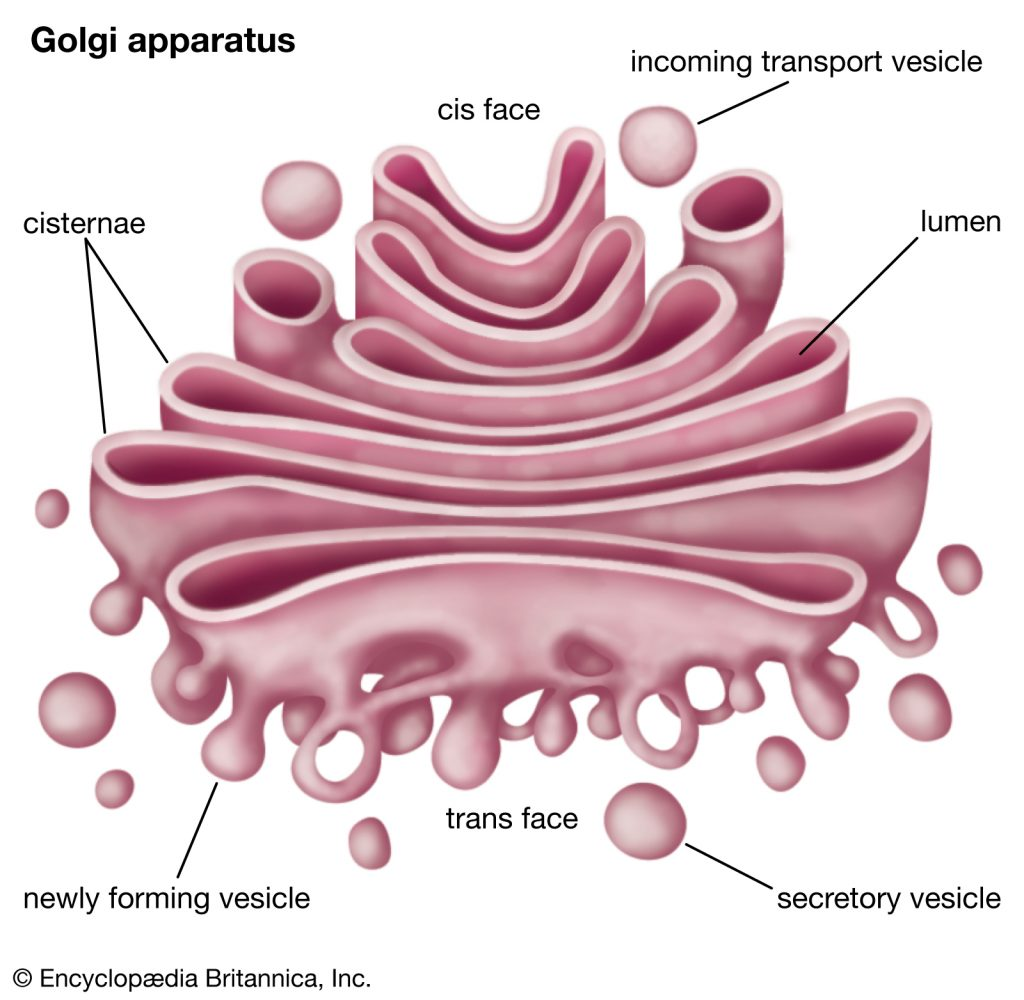
The Golgi apparatus, often referred to as the Golgi body, is a crucial cellular organelle responsible for the intricate processes of sorting, modifying, packaging, and directing cellular products to their respective destinations. Comprising a series of flattened membranous sacs, the Golgi apparatus operates as a central hub for vesicular traffic, ensuring that cellular products are correctly processed and dispatched.
- Structural Overview:
- The Golgi apparatus is characterized by its distinct polarity, with a cis face (receiving face) proximal to the endoplasmic reticulum and a trans face (releasing face) oriented towards the cell membrane. This polarity facilitates the directional flow of vesicular traffic.
- Vesicular Transport and Modification:
- Transport vesicles budding from the endoplasmic reticulum are directed to the Golgi’s cis face. Upon fusion, they release their cargo into the Golgi lumen.
- As lipids and proteins traverse the Golgi stacks, they undergo a series of modifications. One of the most common modifications is glycosylation, where short chains of sugar molecules are appended.
- Concurrently, these modified products are labeled with specific molecular tags, ensuring they reach their designated cellular locations.
- Packaging and Distribution:
- Post-modification, the proteins and lipids are encapsulated into vesicles budding from the Golgi’s trans face.
- Depending on the molecular tags, some vesicles, termed transport vesicles, deliver their contents to specific intracellular locations. In contrast, secretory vesicles merge with the plasma membrane, expelling their contents extracellularly, a process vital for cellular communication and response.
- Cellular Specialization and the Golgi:
- The abundance and activity of the Golgi apparatus vary among cell types, reflecting their functional specialization. For instance, cells with high secretory functions, such as salivary gland cells or immune cells, possess a more pronounced Golgi apparatus.
- Role in Plant Cells:
- Beyond the aforementioned functions, the Golgi apparatus in plant cells is instrumental in synthesizing polysaccharides. Some of these polysaccharides are integrated into the plant cell wall, while others serve various intracellular functions.
In essence, the Golgi apparatus is a dynamic organelle, central to the cell’s secretory pathway. Its ability to modify, package, and direct cellular products is paramount for cellular homeostasis, communication, and response to environmental cues.
7. Lysosomes
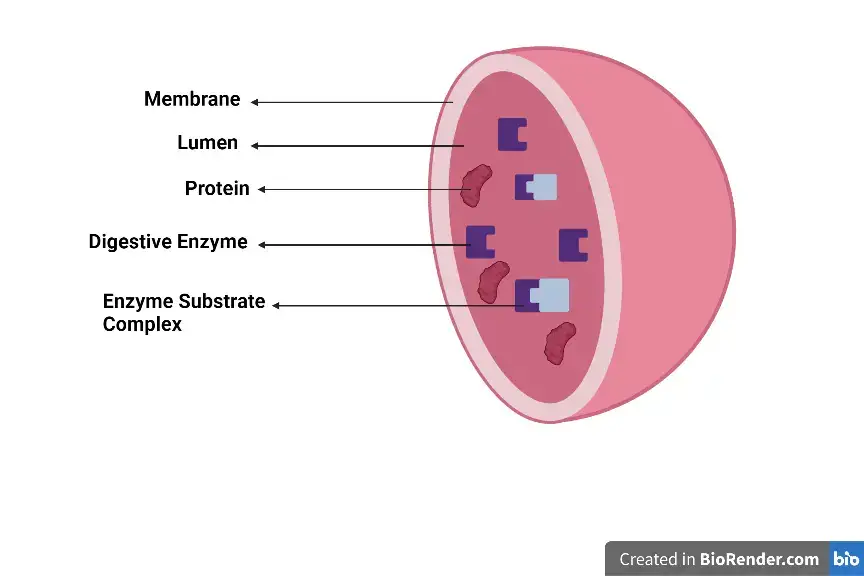
Lysosomes, often termed the “digestive system” of the cell, play a pivotal role in the degradation and recycling of macromolecules and worn-out organelles. These membrane-bound organelles are predominantly found in animal cells and are equipped with a suite of hydrolytic enzymes capable of breaking down a wide range of biological molecules.
- Functional Overview:
- Lysosomes are specialized in the breakdown of proteins, lipids, nucleic acids, polysaccharides, and even obsolete organelles. This degradation process is essential for the cell’s metabolic homeostasis and the recycling of cellular components.
- The enzymes housed within lysosomes function optimally at an acidic pH, a characteristic that distinguishes the lysosomal environment from the more neutral pH of the cytoplasm. This compartmentalization ensures that the potent digestive enzymes do not degrade essential cellular components indiscriminately.
- Role in Immunity:
- Beyond intracellular digestion, lysosomes play a crucial role in the body’s defense mechanisms. In certain white blood cells, such as macrophages, lysosomes are involved in the destruction of invading pathogens. Through a process termed phagocytosis, these cells engulf pathogens, encapsulating them in vesicles. These vesicles subsequently fuse with lysosomes, where the entrapped pathogens are degraded by the lysosomal enzymes.
- Comparison with Plant Cells:
- While lysosomes are a hallmark of animal cells, most plant cells lack these organelles. Instead, plant cells utilize vacuoles for their digestive processes. However, the principle remains the same: compartmentalizing the cell to ensure that potent digestive enzymes function in a controlled environment, preventing potential damage to vital cellular structures.
- Significance of Compartmentalization:
- The presence of lysosomes underscores the importance of compartmentalization in eukaryotic cells. By isolating specific reactions and processes within distinct organelles, cells can maintain internal conditions optimal for each function, thereby enhancing efficiency and safeguarding cellular integrity.
In summary, lysosomes are indispensable organelles in animal cells, orchestrating the controlled breakdown of various molecules and ensuring cellular cleanliness and renewal. Their role in immunity further emphasizes their significance in maintaining cellular and organismal health.
8. Vesicles and Vacuoles
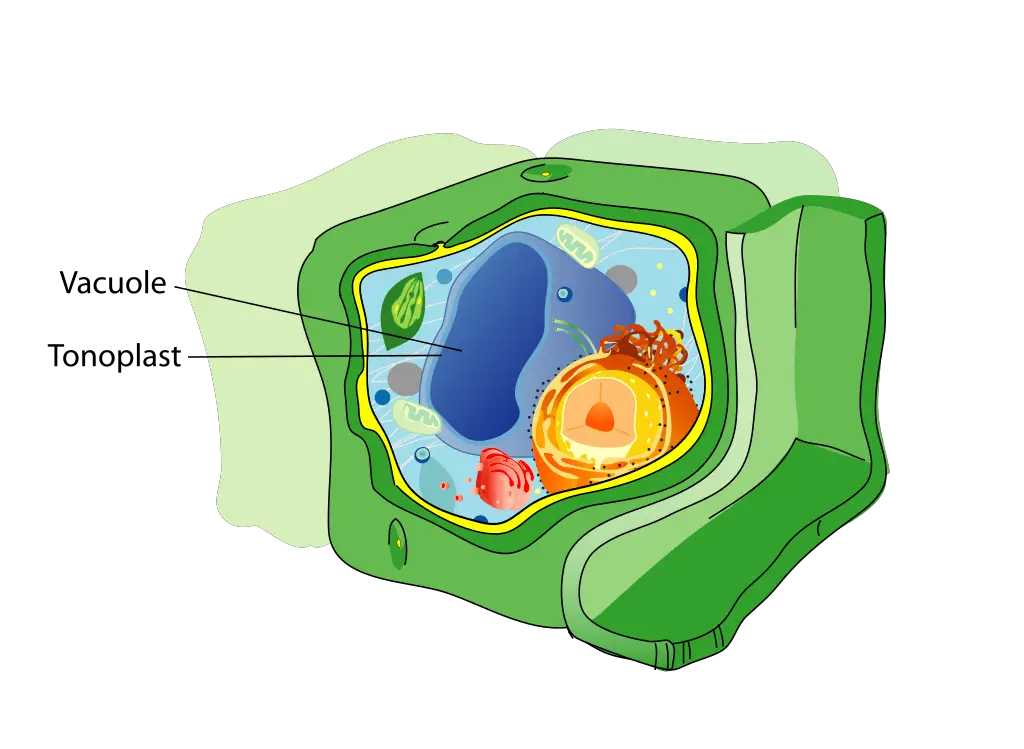
Vesicles and vacuoles are integral components of cellular architecture, each encapsulated by a lipid bilayer membrane. These organelles play pivotal roles in the storage and transport of various cellular materials. While they share certain functional similarities, there are distinct characteristics that differentiate them.
- Size and Structure:
- Vesicles: These are relatively small, membrane-bound sacs that transport materials within the cell. Their membranes have the capability to merge with other cellular membranes, facilitating the transfer of their contents.
- Vacuoles: Larger in comparison to vesicles, vacuoles are prominent storage organelles, especially in plant cells. Unlike vesicles, the membrane of a vacuole, known as the tonoplast, does not readily fuse with other cellular membranes.
- Functional Dynamics:
- Vesicles: Acting as cellular couriers, vesicles transport substances between various cellular compartments or between the cell and its external environment. Their ability to fuse with other membranes allows them to release or intake substances efficiently.
- Vacuoles: Beyond mere storage, vacuoles in plant cells contain enzymes that can degrade macromolecules. This enzymatic activity is crucial for various cellular processes, including the recycling of cellular components.
In essence, while vesicles and vacuoles both serve as cellular storage units, their roles, sizes, and interactions with other cellular components vary. These differences underscore the complexity and specialization of cellular compartments in ensuring the cell’s optimal functioning.
9. Ribosomes
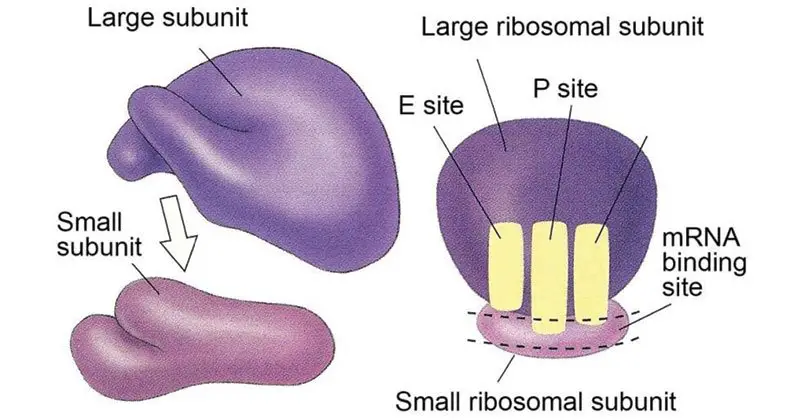
Ribosomes are intricate cellular apparatuses pivotal for the synthesis of proteins, which are essential for the proper functioning of cells. These structures can be visualized as minute entities, either as isolated units or in clusters, dispersed throughout the cytoplasm when observed under an electron microscope. Intriguingly, ribosomes can be anchored to the cytoplasmic facet of the plasma membrane or the endoplasmic reticulum, enhancing their functional versatility.
- Structural Composition:
- Electron microscopic studies reveal that ribosomes are bifurcated into two distinct subunits: a larger and a smaller component. These subunits collaboratively facilitate the intricate process of protein synthesis.
- Functional Mechanism:
- The nucleus, which houses the cell’s genetic material, transcribes DNA into messenger RNA (mRNA). This mRNA then migrates to the ribosomes, serving as a template. The ribosomes, in turn, decode the mRNA’s nucleotide sequence, orchestrating the alignment of amino acids to construct specific proteins. This translation process underscores the ribosome’s role as a cellular interpreter, converting genetic code into functional proteins.
- Ubiquity and Variability:
- Given the universal requirement for proteins in cellular processes, ribosomes are ubiquitous across all cell types. Their abundance is particularly pronounced in cells with heightened protein synthesis demands, exemplified by pancreatic cells that produce digestive enzymes.
- While ribosomes are a common feature in both eukaryotic and prokaryotic cells, they exhibit size variations. Eukaryotic ribosomes, termed 80S, are notably larger than their 70S counterparts in prokaryotic cells. Yet, irrespective of their size, the core function of ribosomes remains consistent across cellular domains: orchestrating protein synthesis.
In summation, ribosomes are paramount to cellular health and functionality, serving as the primary sites for protein synthesis. Their presence across diverse cell types and species accentuates their evolutionary significance and underscores the universality of the protein synthesis process.
10. Mitochondria
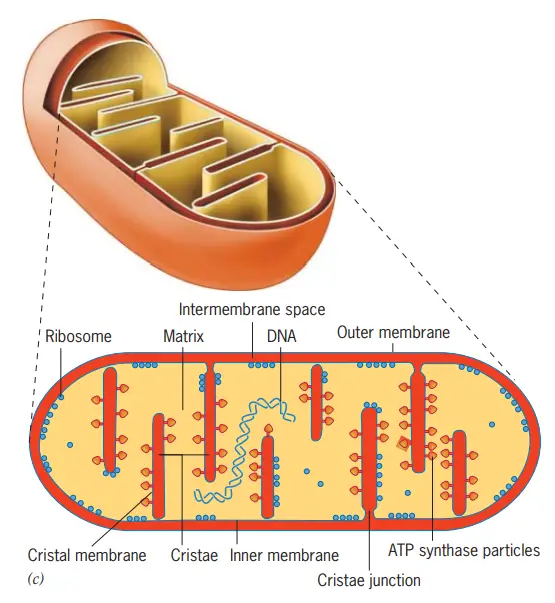
Mitochondria, often characterized as the “energy powerhouses” of the cell, play a pivotal role in energy production, primarily through the synthesis of adenosine triphosphate (ATP). ATP, a molecule of paramount importance, serves as the primary energy currency for cellular processes.
- Structural Attributes:
- Morphologically, mitochondria exhibit an oval configuration and are delineated by two distinct membranes, each constituted by a phospholipid bilayer interspersed with proteins. The inner membrane is intricately folded into structures termed as cristae, which augment the membrane’s surface area. Enclosed by these cristae is the mitochondrial matrix, a compartment distinct from the intermembrane space.
- Functional Dynamics:
- The primary function of mitochondria revolves around cellular respiration, a process that metabolizes glucose to generate ATP, utilizing oxygen in the process and releasing carbon dioxide as a byproduct. This carbon dioxide is eventually expelled from the body during exhalation.
- The cristae and the matrix are not mere structural entities; they are functionally specialized compartments within the mitochondria, each playing a unique role in the process of cellular respiration.
- Distribution and Significance:
- Given the energy demands of muscle cells, it is unsurprising that they are replete with mitochondria, underscoring the principle that cellular structure is intricately linked to its function. The high concentration of mitochondria ensures that muscle cells have a steady supply of ATP, especially crucial during physical exertion.
- Unique Genetic Blueprint:
- Adding to their intrigue, mitochondria possess their own genetic material in the form of circular DNA. This DNA, along with associated ribosomes and other molecular machinery, facilitates the synthesis of certain proteins essential for mitochondrial function. Intriguingly, the mitochondrial genome is often maternally inherited in many organisms.
In essence, mitochondria are not just cellular components; they are dynamic organelles that orchestrate the production of energy, ensuring the cell’s survival and functionality. Their unique genetic makeup and intricate structure further accentuate their significance in cellular biology.
11. Peroxisomes
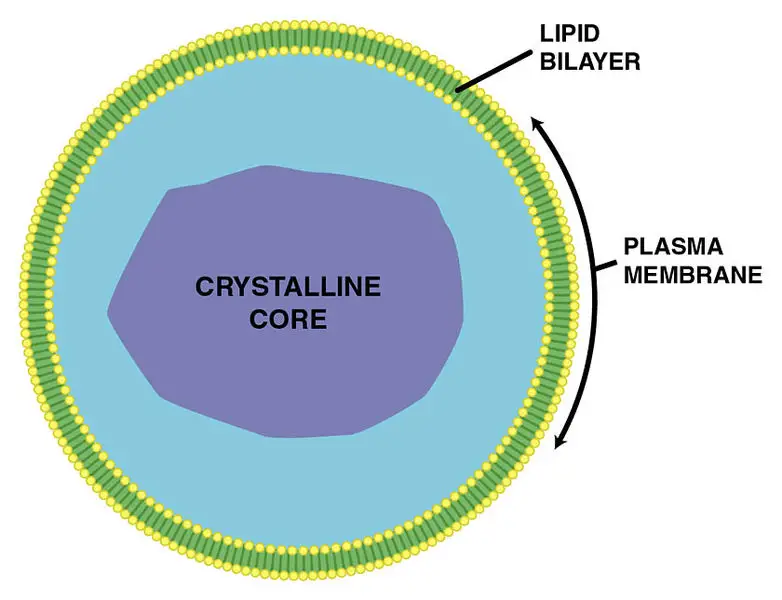
Peroxisomes are specialized cellular organelles, characterized by their round morphology and encasement within a singular membrane. These organelles play a pivotal role in various metabolic processes, particularly in the oxidation of fatty acids and amino acids.
- Oxidative Functions:
- Peroxisomes are renowned for conducting a series of oxidation reactions. These reactions primarily focus on the breakdown of fatty acids and amino acids, which are essential for various cellular functions.
- Detoxification:
- Beyond metabolism, peroxisomes serve as the cell’s detoxification units. They neutralize various potentially harmful substances that might infiltrate the body, including toxins like alcohol. In the liver, peroxisomes are especially crucial, as they actively detoxify ingested alcohol, safeguarding the body from its adverse effects.
- Handling Hydrogen Peroxide:
- A notable byproduct of the oxidation reactions occurring within peroxisomes is hydrogen peroxide (H2O2). While H2O2 can be deleterious to cellular structures, its confinement within peroxisomes ensures it doesn’t wreak havoc. Within the protective confines of the peroxisome, enzymes efficiently decompose hydrogen peroxide into benign substances: water and oxygen, thereby neutralizing its potential threat.
- Plant-Specific Functions:
- In the realm of plant cells, peroxisomes exhibit additional specialized functions. Glyoxysomes, a subset of peroxisomes in plants, are instrumental in converting stored fats into sugars, a vital process for plant energy metabolism. Furthermore, plant cells harbor diverse peroxisomes that participate in various roles, from metabolic activities to defense against pathogens and responses to environmental stressors.
In summation, peroxisomes are indispensable organelles that not only facilitate essential metabolic reactions but also act as cellular guardians, detoxifying harmful substances and managing potentially harmful byproducts. Their multifaceted roles underscore their significance in maintaining cellular health and homeostasis.
12. Plastids
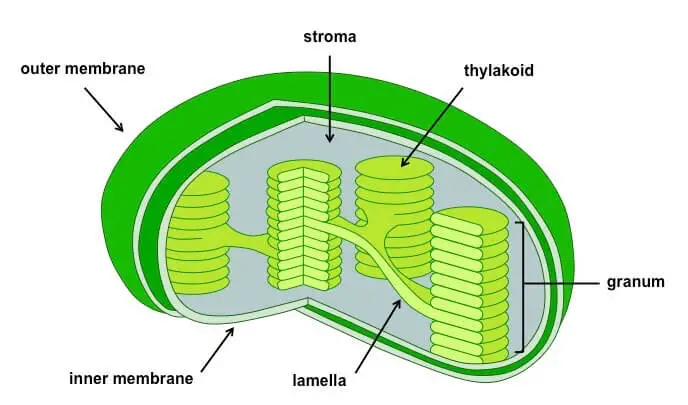
Plastids are large, membrane-enclosed organelles that house pigments vital for various cellular processes in plants. Based on the specific pigments they contain, plastids can be categorized into three primary types:
- Chloroplasts: These are double-membraned organelles that can vary in shape, ranging from spherical to discoid, oval, or even ribbon-like. Predominantly found in the mesophyll cells of leaves, chloroplasts harbor chlorophyll and other carotenoid pigments. These pigments play a pivotal role in capturing light energy essential for the process of photosynthesis. Within the inner membrane of the chloroplast lies a matrix known as the stroma. Within the stroma, one can find flattened, chlorophyll-rich structures termed thylakoids. These thylakoids are organized in stacks, reminiscent of coin piles, with each stack referred to as a granum (plural: grana). Thylakoids from different grana are interconnected by membranous tubules known as stromal lamellae. Analogous to mitochondria, the stroma in chloroplasts also encompasses double-stranded circular DNA, 70S ribosomes, and enzymes vital for carbohydrate and protein synthesis.
- Chromoplasts: Chromoplasts are characterized by the presence of lipid-soluble carotenoid pigments such as xanthophylls and carotene. These pigments bestow plants with distinctive colors ranging from yellow and orange to red.
- Leucoplasts: These are colorless plastids primarily involved in nutrient storage. Depending on the type of nutrient they store, leucoplasts can be further classified into:
- Amyloplasts: Specialized in storing carbohydrates, notably starch, as seen in tubers like potatoes.
- Aleuroplasts: Primarily store proteins.
- Elaioplasts: Engaged in the storage of oils and fats.
In essence, plastids play a multifaceted role in plants, from energy capture and conversion in chloroplasts to storage in leucoplasts and color provision in chromoplasts.
13. Cilia and Flagella
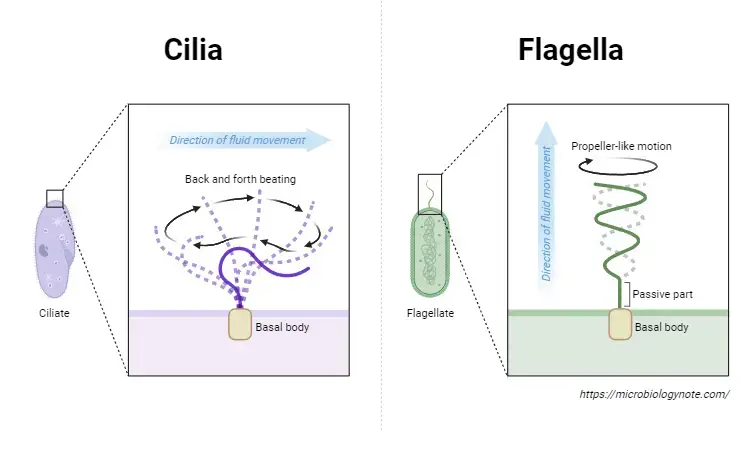
Cilia and flagella are specialized cellular appendages that play a pivotal role in locomotion and fluid movement. Both structures exhibit a distinct architecture, yet they share some common features.
- Cilia: These are slender, hair-like projections extending outward from the cell surface. Functioning akin to oars, cilia can either propel the cell through its environment or move extracellular fluid over the cell’s surface. Their rhythmic, coordinated movements are essential for various biological processes, such as the movement of mucus in the respiratory tract.
- Flagella: These are elongated whip-like structures, larger than cilia, primarily responsible for driving cellular movement. The structural composition of eukaryotic flagella is notably different from that of their prokaryotic counterparts.
Both cilia and flagella possess a core structure termed the axoneme. This axoneme is characterized by a specific arrangement of microtubules: nine pairs of peripherally arranged microtubules encircle a central pair of microtubules. This configuration is often referred to as the “9+2” arrangement. The central microtubules are interconnected by a bridge and are surrounded by a central sheath. Additionally, radial spokes extend from one of the peripheral microtubule pairs to the central sheath, resulting in a total of nine radial spokes. The emergence of cilia and flagella from the cell is facilitated by centriole-like structures known as basal bodies.
In summary, cilia and flagella are vital cellular structures in eukaryotes, aiding in movement and fluid transport, and are distinguished by their intricate microtubule arrangements and specific functions.
Cell Component | Function | Present in Prokaryotes? | Present in Animal Cells? | Present in Plant Cells? |
---|---|---|---|---|
Plasma membrane | Separates cell from external environment; controls passage of molecules | Yes | Yes | Yes |
Cytoplasm | Provides structure; site of metabolic reactions; houses organelles | Yes | Yes | Yes |
Nucleoid | Location of DNA | Yes | No | No |
Nucleus | Houses DNA; directs synthesis of ribosomes and proteins | No | Yes | Yes |
Ribosomes | Protein synthesis | Yes | Yes | Yes |
Mitochondria | ATP production/cellular respiration | No | Yes | Yes |
Peroxisomes | Oxidizes and breaks down fatty acids and amino acids; detoxifies poisons | No | Yes | Yes |
Vesicles and vacuoles | Storage and transport; digestive function in plant cells | No | Yes | Yes |
Centrosome | Role in cell division in animals; organizing center of microtubules | No | Yes | No |
Lysosomes | Digestion of macromolecules; recycling of organelles | No | Yes | No |
Cell wall | Protection, support, and shape maintenance | Yes (mainly peptidoglycan) | No | Yes (mainly cellulose) |
Chloroplasts | Photosynthesis | No | No | Yes |
Endoplasmic reticulum | Modifies proteins and synthesizes lipids | No | Yes | Yes |
Golgi apparatus | Modifies, sorts, tags, packages, and distributes lipids and proteins | No | Yes | Yes |
Cytoskeleton | Maintains shape, secures organelles, allows movement within the cell, enables unicellular movement | Yes | Yes | Yes |
Flagella | Cellular locomotion | Some | Some | No (except some plant sperm) |
Cilia | Cellular locomotion, movement of particles along membrane, and filtration | No | Some | No |
Animal Cells versus Plant Cells
Eukaryotic cells, the hallmark of complex multicellular organisms, can be broadly categorized into animal and plant cells. While they share numerous similarities, distinct differences set them apart, underscoring the diverse evolutionary paths and functional requirements of plants and animals.
- Structural Distinctions:
- Cell Wall: Exclusively found in plant cells, the cell wall is a rigid external layer that offers protection, structural support, and shape. Composed primarily of cellulose, a polysaccharide chain of glucose units, it distinguishes plant cells from animal cells which lack this external boundary. This cellulose-rich wall is also a primary source of dietary fiber in human nutrition.
- Central Vacuole: Plant cells are characterized by a prominent central vacuole, which dominates the cellular space. This organelle plays a pivotal role in osmoregulation, adjusting the cell’s water concentration in response to environmental changes. The central vacuole’s contents provide turgor pressure, crucial for maintaining cell rigidity. A deficit in this pressure, due to water loss, manifests as plant wilting.
- Energy Production and Conversion:
- Chloroplasts: Unique to plant cells and some algae, chloroplasts are the sites of photosynthesis, where light energy is converted into chemical energy in the form of glucose. Enclosed by a double membrane, the internal space of chloroplasts contains thylakoids and the stroma. Chlorophyll, a green pigment in thylakoids, captures sunlight, facilitating the photosynthetic process.
- Mitochondria: Common to both animal and plant cells, mitochondria are the energy powerhouses, producing ATP through cellular respiration.
- Endosymbiotic Theory:
- The presence of DNA and ribosomes in mitochondria and chloroplasts has led scientists to propose the endosymbiotic theory. This theory suggests that mitochondria and chloroplasts originated from ancestral prokaryotes that were engulfed by a host cell. Over evolutionary time, a symbiotic relationship developed, with the engulfed organisms evolving into mitochondria and chloroplasts.
- Distinct Organelles:
- Animal cells are equipped with centrioles, centrosomes, and lysosomes, structures absent in plant cells. Conversely, plant cells possess plasmodesmata and plastids, which are not found in animal cells.
- Functional Implications:
- The presence of chloroplasts allows plant cells to be autotrophic, synthesizing their own food through photosynthesis. Animal cells, being heterotrophic, rely on external sources for organic compounds.
In conclusion, while animal and plant cells share a foundational eukaryotic blueprint, their structural and functional variations reflect the diverse life strategies and environmental interactions of the organisms they constitute. These differences, from energy production to structural support, highlight the intricate and specialized nature of cellular evolution and function.
Eukaryotic Cell Cycle
The eukaryotic cell cycle is a meticulously orchestrated sequence of events through which a cell grows, replicates its DNA, and ultimately divides to produce two daughter cells. This cycle is punctuated by specific checkpoints, ensuring the fidelity of each phase and the readiness of the cell to transition to the subsequent stage.
- Quiescence (G0 Phase):
- Quiescence, often termed G0, is a state where the cell is metabolically active but not actively proliferating.
- Some cells, such as those in the liver, stomach, kidney, and nervous system, can enter this phase and remain non-divisive for extended durations.
- This phase can be a response to DNA damage or other cellular stresses. However, many cells bypass G0 entirely, continuously cycling throughout an organism’s life.
- Interphase:
- Constituting approximately 90% of the cell cycle, interphase is a period of cellular growth and DNA replication. It is subdivided into three phases:
- Gap 1 (G1 Phase): A phase of cellular growth where the cell enlarges, synthesizes proteins, and duplicates organelles like mitochondria.
- Synthesis (S Phase): During this phase, DNA replication occurs. Chromosomes duplicate, resulting in two sister chromatids for each chromosome. Consequently, the cell’s DNA content doubles.
- Gap 2 (G2 Phase): A secondary growth phase where the cell further enlarges, and the remaining organelles are duplicated in anticipation of cell division.
- Constituting approximately 90% of the cell cycle, interphase is a period of cellular growth and DNA replication. It is subdivided into three phases:
- Mitosis (M Phase):
- Mitosis is the phase where the cell segregates its duplicated DNA, ensuring each daughter cell receives an identical set of chromosomes.
- Mitosis is further categorized into distinct stages:
- Prophase: Chromosomes condense and become visible. The mitotic spindle begins to form.
- Metaphase: Chromosomes align at the cell’s equatorial plane, facilitated by the spindle fibers.
- Anaphase: Sister chromatids separate and move to opposite poles of the cell.
- Telophase: Chromatids reach the poles, and the nuclear envelope starts to re-form.
- Following mitosis, the cell undergoes cytokinesis, a process that divides the cytoplasm and results in the formation of two distinct daughter cells.
In essence, the eukaryotic cell cycle is a dynamic and regulated process, ensuring the accurate replication and distribution of genetic material. This cycle is fundamental to growth, development, and tissue repair in multicellular organisms.
Extracellular Matrix of Animal Cells
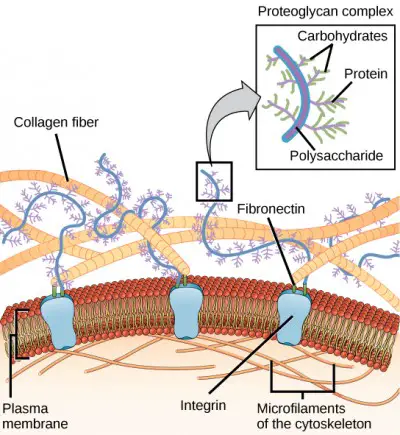
The extracellular matrix (ECM) is a complex and dynamic network that surrounds most animal cells, providing both structural support and a medium for cellular communication. Composed primarily of glycoproteins and the protein collagen, the ECM plays a pivotal role in tissue formation, maintenance, and function.
- Composition:
- Glycoproteins: These are proteins that have carbohydrates attached to them. They play a crucial role in cell-to-cell recognition and interactions.
- Collagen: This is the most abundant protein in the ECM, providing tensile strength and resisting stretching forces. It forms fibrous networks that offer structural support to tissues.
- Functions:
- Tissue Cohesion: The ECM acts as a cellular adhesive, binding cells together to form tissues. This cohesive nature ensures that cells remain in their designated locations and maintain tissue integrity.
- Cellular Communication: Beyond its structural role, the ECM is a hub for cellular signaling. It facilitates communication between cells, allowing them to respond collectively to changes in their environment.
In conclusion, the extracellular matrix is not just a passive scaffold for animal cells. It is an active participant in various cellular processes, from providing structural support to mediating intricate cellular communications. Understanding the intricacies of the ECM is crucial for insights into tissue development, repair, and various pathological conditions.
Intercellular Junctions
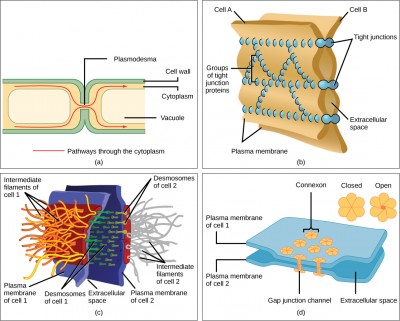
Intercellular junctions are specialized structures that facilitate communication and adhesion between adjacent cells. These junctions vary in their composition and function, and while some are common to both plant and animal cells, others are exclusive to one or the other.
- Plasmodesmata:
- Location: Exclusively found in plant cells.
- Structure: Channels that traverse the cell walls of adjacent plant cells.
- Function: They enable the transport of signaling molecules, ions, and nutrients between cells, ensuring coordinated cellular activities and nutrient sharing.
- Tight Junctions:
- Location: Predominantly in animal cells.
- Structure: Composed of proteins that bind adjacent cells tightly together, creating a virtually impermeable barrier.
- Function: They prevent the leakage of molecules between cells, ensuring compartmentalization. Particularly crucial in epithelial tissues lining organs and cavities, such as the urinary bladder, where they prevent urine from seeping into surrounding tissues.
- Desmosomes:
- Location: Exclusively in animal cells.
- Structure: Resemble “spot welds” or rivets, anchoring cells together.
- Function: Provide strength and rigidity, especially in tissues that undergo significant stress or stretching, such as the skin, heart, and muscles.
- Gap Junctions:
- Location: Found in animal cells.
- Structure: Channels that allow direct communication between the cytoplasm of adjacent cells.
- Function: Similar to plasmodesmata in plants, gap junctions permit the transfer of ions, nutrients, and other molecules, facilitating cellular communication. However, their structural composition differs from plasmodesmata.
In summary, intercellular junctions play a pivotal role in maintaining tissue integrity, facilitating cellular communication, and ensuring coordinated cellular functions. The type and distribution of these junctions are tailored to the specific needs and functions of tissues, reflecting the intricate design and adaptability of cellular structures.
Examples of a Eukaryotic Cell
Eukaryotic cells, characterized by the presence of a membrane-bound nucleus and specialized organelles, can be found in a diverse range of organisms. Here are some representative examples:
- Plant Cells:
- Cell Wall: Plant cells are fortified with a robust cell wall primarily composed of cellulose, providing structural integrity.
- Central Vacuole: Dominating the cell’s interior, the central vacuole is pivotal for maintaining turgor pressure, a result of water exerting an outward force on the cell wall.
- Chloroplasts: These organelles house chlorophyll, a molecule instrumental in photosynthesis, enabling plants to harness light energy to synthesize sugars.
- Fungal Cells:
- Chitin-based Cell Wall: Unlike plant cells, the cell walls of fungi are primarily composed of chitin, a material also found in insect exoskeletons.
- Septa: Some fungi possess septa, perforations that facilitate the exchange of organelles and cytoplasm between cells, leading to a more interconnected cellular network.
- Mycelial Network: In certain environments, fungi form extensive underground or decaying organic matter networks, comprising millions of interconnected cells.
- Animal Cells:
- Plasma Membrane: In the absence of a cell wall, animal cells are delineated by a plasma membrane, granting them the flexibility to adopt various shapes.
- Phagocytosis and Pinocytosis: The malleability of animal cells facilitates processes like phagocytosis (ingestion of particles) and pinocytosis (ingestion of liquids).
- Vacuoles: Unlike the singular, large vacuole in plant cells, animal cells possess multiple smaller vacuoles for various functions.
- Protozoa:
- Single-celled Organisms: Protozoa are unicellular eukaryotes capable of movement and predation on other microorganisms.
- Locomotory Structures: Depending on the species, protozoa may employ cilia (fine, hair-like structures) or flagella (tail-like appendages) for movement.
- Pellicle: Some protozoa are equipped with a pellicle, a supportive layer that reinforces the cell membrane.
In summary, while all eukaryotic cells share certain foundational characteristics, the specific adaptations and features of these cells in different organisms underscore the vast diversity and specialization within the eukaryotic domain.
Eukaryotic Cell Flashcard
Quiz
Which of the following is a characteristic feature of eukaryotic cells?
a) Absence of a nucleus
b) Presence of ribosomes
c) Presence of a defined nucleus
d) Lack of membrane-bound organelles
Which organelle is responsible for producing ATP in eukaryotic cells?
a) Golgi apparatus
b) Lysosome
c) Mitochondria
d) Endoplasmic reticulum
Which structure is present in plant cells but absent in animal cells?
a) Lysosomes
b) Cell wall
c) Mitochondria
d) Centrosomes
The endoplasmic reticulum that has ribosomes attached to its surface is called:
a) Smooth ER
b) Rough ER
c) Granular ER
d) Non-granular ER
Which organelle is responsible for photosynthesis in plant cells?
a) Chloroplast
b) Mitochondria
c) Peroxisome
d) Lysosome
Which of the following is NOT a component of the eukaryotic cell's cytoskeleton?
a) Microfilaments
b) Microtubules
c) Ribosomes
d) Intermediate filaments
Which organelle is often referred to as the "protein factory" of the cell?
a) Nucleus
b) Ribosome
c) Golgi apparatus
d) Lysosome
Which of the following organelles is responsible for lipid synthesis and detoxification processes?
a) Rough ER
b) Smooth ER
c) Golgi apparatus
d) Peroxisome
Which structure in eukaryotic cells is responsible for storing genetic information?
a) Mitochondria
b) Nucleus
c) Ribosome
d) Endoplasmic reticulum
Which of the following is a function of the Golgi apparatus?
a) ATP production
b) Protein synthesis
c) Modification and packaging of proteins
d) Photosynthesis
FAQ
What is a eukaryotic cell?
A eukaryotic cell is a type of cell that has a defined nucleus and other membrane-bound organelles. It is found in organisms like plants, animals, fungi, and protists.
How do eukaryotic cells differ from prokaryotic cells?
The primary difference is that eukaryotic cells have a defined nucleus and membrane-bound organelles, while prokaryotic cells lack these structures.
Do eukaryotic cells have DNA?
Yes, eukaryotic cells contain DNA housed within the nucleus. This DNA carries the genetic information necessary for the cell's functions.
What are the main organelles in a eukaryotic cell?
Some of the main organelles include the nucleus, mitochondria, endoplasmic reticulum, Golgi apparatus, lysosomes, and, in plant cells, chloroplasts.
Why are mitochondria referred to as the "powerhouses" of the cell?
Mitochondria are responsible for producing ATP, the primary energy currency of the cell, through a process called cellular respiration.
Do all eukaryotic cells have a cell wall?
No, only plant cells and some fungi and protists have a cell wall. Animal cells lack a cell wall.
What is the function of the endoplasmic reticulum?
The endoplasmic reticulum (ER) is involved in protein synthesis (rough ER) and lipid synthesis (smooth ER).
How do plant cells produce energy from sunlight?
Plant cells contain chloroplasts, which capture sunlight and convert it into chemical energy through a process called photosynthesis.
What is the role of the cytoskeleton in eukaryotic cells?
The cytoskeleton provides structural support to the cell, aids in cell movement, and helps in the transport of materials within the cell.
Why do animal cells have lysosomes while plant cells typically do not?
Lysosomes contain digestive enzymes that break down waste materials and cellular debris in animal cells. While plant cells may have similar structures called vacuoles for storage and waste disposal, they typically lack lysosomes.