Antigen Processing and Presentation
- Foreign protein antigen recognition by a T cell needs the presentation of peptides produced from the antigen within the cleft of an MHC molecule on the cell membrane.
- The production of these peptide-MHC complexes requires the degradation of a protein antigen into peptides, a process known as antigen processing.
- The degraded peptides then bind to MHC molecules within the interior of the cell, and the peptide-MHC complexes are transported to the cell membrane, where they are displayed (antigen presentation).
- Class I and class II MHC molecules bind to peptides processed in distinct intracellular compartments.
- Class I MHC molecules bind cytoplasmically processed peptides generated from endogenous antigens (e.g., normal cellular proteins, tumour proteins, or viral and bacterial proteins produced within infected cells).
- Class II MHC molecules bind peptides produced from exogenous antigens that have been ingested by phagocytosis or endocytosis and processed along the endocytic route.
Types of Antigen Processing and Presenting Pathway
- The immune system eliminates intracellular and extracellular antigens via two distinct routes.
- Endogenous antigens are processed in the cytosolic pathway and presented on the membrane with class I MHC molecules; exogenous antigens are processed in the endocytic pathway and presented on the membrane with class II MHC molecules.
- L. A. Morrison and T. J. Braciale’s experiments gave preliminary evidence that the antigenic peptides presented by class I and class II MHC molecules are produced from distinct processing mechanisms.
- The experimental technique of these researchers was based on the qualities of two clones of TC cells, one of which identified influenza hemagglutinin (HA) connected with a class I MHC molecule and another of which recognised the identical antigen associated with a class II MHC molecule. (In this instance, as well as in others, the link between T-cell function and MHC limitation is not absolute.)
- In one series of investigations, target cells expressing both class I and class II MHC molecules were treated with live influenza virus or UV-inactivated influenza virus. (The inactivated virus kept its antigenic features but lost its ability to replicate within the target cells.) The target cells were subsequently treated with class I–restricted or atypical class II–restricted TC cells, and their subsequent lysis was assessed.
- Table demonstrates that class II–restricted TC cells responded to target cells treated with infectious or noninfectious influenza viruses.
- Class I–restricted TC cells only responded to target cells infected with infectious viral particles. Similarly, target cells treated with influenza virions in the presence of emetine, which inhibits viral protein synthesis, stimulated class II–restricted TC cells but not class I–restricted TC cells.
- In contrast, target cells treated with infectious viruses in the presence of chloroquine, a medication that inhibits the endocytic processing pathway, stimulated class I–restricted TC cells but not class II–restricted TC cells.
- These findings demonstrate the distinction between exogenous and endogenous antigen processing, particularly the preferential interaction of foreign antigens with class II MHC molecules and endogenous antigens with class I MHC molecules.
- Association of viral antigen with class I MHC molecules required influenza virus replication and viral protein synthesis in target cells, whereas association with class II MHC molecules did not.
- Class I MHC molecules engage with peptides obtained from the cytosolic degradation of endogenously formed proteins, whereas class II MHC molecules interact with peptides derived from the endocytic degradation of exogenously synthesised antigens.
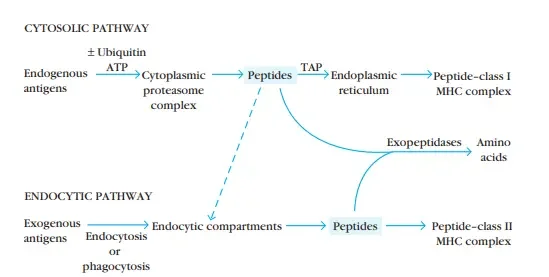
Endogenous Antigens: Cytosolic Pathway
- Protein levels in eukaryotic cells are tightly regulated. Every protein undergoes constant turnover and is destroyed at a pace that is typically stated as its halflife.
- Some proteins (e.g. transcription factors, cyclins, and essential metabolic enzymes) have extremely short half-lives, and denatured, misfolded, or otherwise aberrant proteins are also rapidly destroyed.
- The method through which endogenous antigens are destroyed for presentation with class I MHC molecules utilises the same pathways as intracellular protein turnover.
1. Peptides for Presentation Are Generated by Protease Complexes Called Proteasomes
- Existing in all cells, the cytosolic proteolytic system degrades intracellular proteins into small peptides.
- Proteolysis-targeted proteins frequently have a tiny protein called ubiquitin linked to them.
- A multifunctional protease complex termed a proteasome is capable of degrading ubiquitin-protein conjugates.
- Each proteasome is a big (26S), cylindric particle composed of four rings of protein subunits and a central channel with a diameter between 10 and 50 Å.
- In an ATP-dependent manner, a proteasome can cleave peptide bonds between two or three distinct amino acid combinations.
- It is believed that ubiquitin-protein complexes are degraded within the core cavity of the proteasome.
- The immune system exploits this broad route of protein degradation to generate short peptides for presentation with class I MHC molecules, according to experimental findings.
- Two subunits encoded in the MHC gene cluster, LMP2 and LMP7, and a third non-MHC protein, LMP10, are present in the proteasomes engaged in antigen processing (also called MECL-1).
- Increased levels of the T-cell cytokine IFN-γ trigger all three. The peptidase activities of proteasomes containing LMP2, LMP7, and LMP10 create MHC class I-binding peptides preferentially.
- Such proteasomes exhibit enhanced hydrolysis of peptide bonds following basic and/or hydrophobic residues, for instance.
- Almost exclusively, peptides that bind to class I MHC molecules terminate in hydrophobic or basic residues.
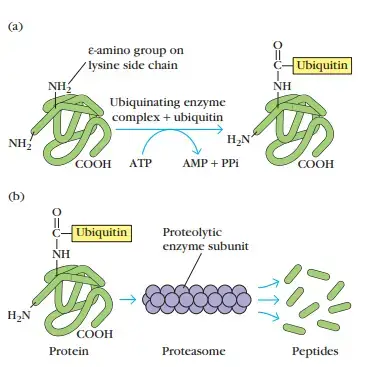
2. Peptides Are Transported from the Cytosol to the Rough Endoplasmic Reticulum
- Studies of cell lines with deficiencies in peptide presentation by class I MHC molecules provided insight into the function of peptide transport, the transfer of peptides to the MHC molecule, in the cytosolic processing pathway.
- RMA-S is a mutant cell line that expresses around 5% of the usual amount of class I MHC molecules in its membrane. Despite the fact that RMA-S cells generate typical amounts of class I α chains and β2-microglobulin, neither molecule is seen on the membrane.
- A. Townsend and his colleagues discovered that “feeding” these cells peptides restored their amount of membrane-associated class I MHC molecules to normal, which provided a hint to the mutation in the RMA-S cell line.
- These researchers hypothesised that peptides may be necessary for stabilising the connection between the class I α chain and β2-microglobulin.
- By feeding the cells predigested peptides, it was possible to reestablish production of class I MHC molecules on the cell membrane, indicating that the RMA-S cell line may have a deficiency in peptide transport.
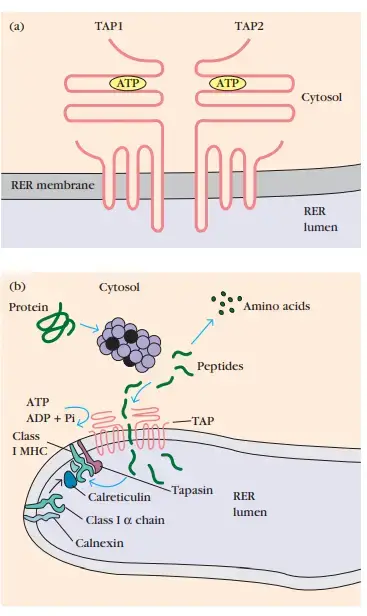
- Subsequent tests demonstrated that the deficiency in the RMA-S cell line is located in the protein that transports peptides from the cytoplasm to the RER, where class I molecules are generated.
- When RMA-S cells were transfected with a functional gene producing the transporter protein, class I molecules were expressed on the cell membrane.
- TAP (transporter associated with antigen processing) is a membrane-spanning heterodimer composed of TAP1 and TAP2 proteins.
- In addition to their numerous transmembrane segments, the TAP1 and TAP2 proteins possess a domain extending into the lumen of the RER and an ATP-binding domain extending into the cytosol.
- These proteins mediate ATP-dependent transport of amino acids, carbohydrates, ions, and peptides and are found in the membranes of many organisms, including bacteria.
- Peptides produced in the cytosol by the proteasome are translocated by TAP into the RER via an ATP-dependent mechanism. TAP has the maximum affinity for 8–10 amino acid peptides, the ideal length for class I MHC binding.
- Moreover, TAP appears to select peptides containing hydrophobic or basic carboxy-terminal amino acids, which are the preferred anchor residues for class I MHC molecules. Thus, TAP is specialised for transporting peptides that bind with MHC molecules of class I.
- The TAP1 and TAP2 genes are located next to the LMP2 and LMP7 genes within the class II MHC region.
- Both the transporter genes and these LMP genes are polymorphic, meaning that different alleles occur in the population.
- Allelic differences in LMP-mediated proteolytic cleavage of protein antigens or in the transit of distinct peptides from the cytosol into the RER may contribute to the reported diversity in individuals’ responses to various endogenous antigens.
- TAP deficiency can result in a condition characterised by immunodeficiency and autoimmune.
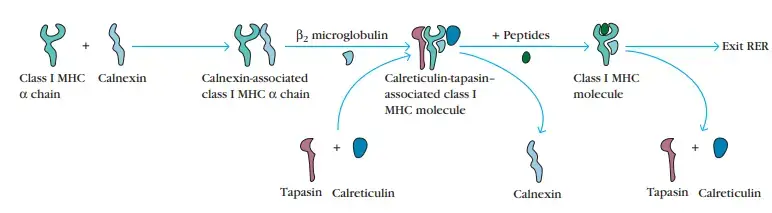
3. Peptides Assemble with Class I MHC Aided by Chaperone Molecules
- The chain and β2-microglobulin components of the class I MHC molecule are formed on polysomes along the rough endoplasmic reticulum, similar to other proteins.
- In order for these components to assemble into a stable class I MHC molecular complex that can escape the RER, a peptide must be present in the binding groove of the class I molecule.
- The assembly process comprises multiple phases including the engagement of molecular chaperones, which aid in polypeptide folding.
- The first molecular chaperone involved in class I MHC assembly is calnexin, an endoplasmic reticulum-resident membrane protein. Calnexin binds to the free class I α chain and facilitates its folding.
- When β2-microglobulin binds to the α chain, calnexin is released and the class I molecule binds to the chaperone calreticulin and the protease tapasin. Tapasin (TAP-associated protein) permits the TAP transporter to acquire an antigenic peptide by bringing it into close proximity with the class I molecule.
- Before the peptides are exposed to the luminal milieu of the RER, the physical connection between the α chain–β2-microglobulin heterodimer and the TAP protein increases peptide capture by the class I molecule.
- Peptides that are not bound by class I molecules breakdown fast. As a result of peptide interaction, the class I molecule becomes more stable and is able to dissociate from calreticulin and tapasin, leave the RER, and go to the cell surface via the Golgi.
- With calnexin and calreticulin complexes, an additional chaperone protein, ERp57, has been identified.
- This resident endoplasmic reticulum protein is believed to contribute to the production of disulfide bonds during the maturation of class I chains, although its specific function in the assembly and loading of class I peptides has yet to be determined. Because its function is not well-defined.
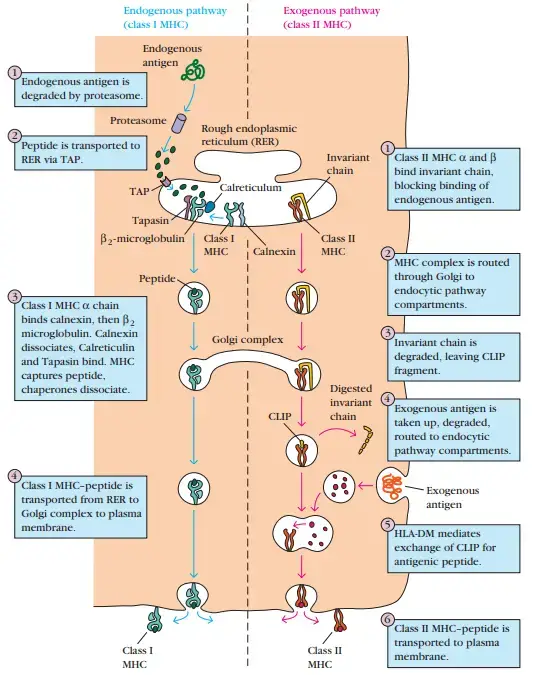
Exogenous Antigens: Endocytic Pathway
- The mechanism of entry into the cell, exogenous or endogenous, and the location of processing determine whether an antigenic peptide connects with class I or class II molecules.
- Internalization of antigen by antigen-presenting cells can occur via phagocytosis, endocytosis, or both. Macrophages internalise antigen via both phagocytosis and endocytosis, whereas the majority of APCs are neither phagocytic or are phagocytically inefficient and consequently ingest exogenous antigen only via endocytosis (either receptor-mediated endocytosis or pinocytosis).
- B cells, for instance, efficiently ingest antigen via receptor-mediated endocytosis employing antigen-specific membrane antibody as the receptor.
1. Peptides Are Generated from Internalized Molecules in Endocytic Vesicles
- Once an antigen has been internalised, it is digested into peptides within endocytic processing pathway compartments.
- Internalized antigen requires 1–3 h to traverse the endocytic route and generate peptide–class II MHC complexes at the cell surface.
- The endocytic pathway appears to involve three increasingly acidic compartments: early endosomes (pH 6.0–6.5), late endosomes (pH 5.0–6.0), and lysosomes (pH 4.5–5.0). As antigen is transported from early to late to lysosomes, it encounters hydrolytic enzymes and a decreasing pH in each compartment.
- Lysosomes, for instance, include more than 40 acid-dependent hydrolases, including proteases, nucleases, glycosidases, lipases, phospholipases, and phosphatases.
- Antigen is digested within the compartments of the endocytic route into oligopeptides with 13–18 residues, which bind to class II MHC molecules.
- Since hydrolytic enzymes are most active in acidic environments (low pH), antigen processing can be blocked by chemical agents that elevate the pH of the compartments (e.g., chloroquine) as well as by protease inhibitors (e.g., leupeptin).
- The method by which antigen is transported from one endocytic compartment to the next has not been shown satisfactorily. It has been proposed that peripheral early endosomes migrate inward to become late endosomes and then lysosomes.
- Alternately, antigens may be transported from one compartment to the next via tiny transport vesicles.
- Eventually, the endocytic compartments, or sections thereof, return to the cell’s periphery and fuse with the plasma membrane. The surface receptors are regenerated in this manner.
2. The Invariant Chain Guides Transport of Class II MHC Molecules to Endocytic Vesicles
- Since antigen-presenting cells express both class I and class II MHC molecules, there must exist a mechanism to prevent class II MHC molecules from attaching to the same antigenic peptides as class I molecules.
- When class II MHC molecules are generated in the RER, three pairs of class II chains bind to a preassembled trimer of the protein invariant chain (Ii, CD74).
- This trimeric protein interacts with the peptide-binding cleft of class II molecules, inhibiting the binding of any endogenously produced peptides to the cleft while the class II molecule is within the RER.
- The invariant chain appears to be involved in the folding of class IIα and β chains, their escape from the RER, and the subsequent routing of class II molecules from the trans-Golgi network to the endocytic processing route.
- Transfection experiments using cells devoid of the genes for class II MHC molecules and the invariant chain have revealed the role of the invariant chain in the routing of class II molecules.
- Immunofluorescent labelling of these cells transfected with exclusively class II MHC genes indicated the localization of class II molecules within the Golgi complex.
- In contrast, class II molecules were localised in the cytoplasmic vesicular structures of the endocytic pathway in cells transfected with both class II MHC and invariantchain genes.
- In its cytoplasmic tail, the invariant chain carries sorting signals that direct the transport of the class II MHC complex from the trans-Golgi network to endocytic compartments.
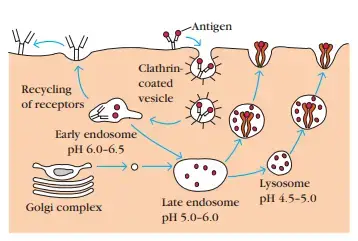
3. Peptides Assemble with Class II MHC Molecules by Displacing CLIP
- Recent research indicates that the majority of class II MHC–invariant chain complexes are carried from the RER, where they are generated, to the Golgi complex and trans-Golgi network, and then through the endocytic route, travelling from early endosomes to late endosomes and subsequently to lysosomes.
- As the proteolytic activity in each successive compartment rises, the invariant chain degrades gradually. CLIP (class II–associated invariant chain peptide) is a small remnant of the invariant chain that remains tethered to the class II molecule after the invariant chain has been broken within the endosomal compartment.
- CLIP physically fills the peptide-binding groove of the class II MHC molecule, likely preventing antigenic peptide from binding prematurely. HLA-DM is highly conserved throughout mammalian species.
- HLA-DM, like other class II MHC molecules, is a heterodimer composed of α and β chains. Unlike other class II molecules, however, HLA-DM is not polymorphic and is not expressed at the cell membrane; instead, it is primarily located in the endosomal compartment.
- The DMα and DMβ genes are in close proximity to the TAP and LMP genes in the human MHC complex, and DM is expressed in cells that express classical class II molecules.
- In the presence of HLA-DO, the interaction between HLA-DM and the class II CLIP complex, which facilitates the exchange of CLIP for another peptide, is inhibited because HLA-DO binds to HLA-DM and reduces the efficacy of the exchange reaction.
- Similar to HLA-DM, HLADO is a nonclassical and nonpolymorphic class II protein identified in the MHC of various animals.
- HLA-DO is solely expressed by B cells and the thymus, unlike HLA-DM, and its expression is not triggered by IFN-γ, unlike other class II molecules.
- In addition, the genes encoding the α and the β chains of HLA-DO are not next to one another in the MHC, unlike all other class II α and β pairs.
- A CLIP-associated HLA-DR3 molecule was recovered from a cell line that lacked HLA-DM and was consequently incapable of antigen processing. CLIP attaches to class II in the same stable manner as the antigenic peptide, as demonstrated by the superimposition of the structure of HLA-DR3–CLIP onto another DR protein coupled to an antigenic peptide.
- The identification of this stable compound in a cell lacking HLA-DM lends credence to the claim that HLA-DM is essential for CLIP replacement.
- Although it controls the activity of HLA-DM, the specific function of HLA-DO is still unknown. It may function in the selection of peptides linked to class II MHC molecules in B cells.
- In these cells, DO exists in combination with DM, and this relationship persists in endosomal compartments. Higher acidity conditions impair the connection between DM and DO and increase the likelihood of antigenic peptide binding despite the presence of DO.
- This pH-dependent interaction may cause B lymphocytes to preferentially select class II-bound peptides from lysosomal compartments over other APCs.
- Similar to class I MHC molecules, class II MHC molecules require peptide interaction to preserve their structure and stability.
- Once a peptide binds, the peptide–class II complex is carried to the plasma membrane, where the neutral pH enables the complex to assume a compact, stable structure.
- Under physiological settings, it is difficult to replace a class II–attached peptide on the membrane with another peptide when the peptide is bound in this compact state.
References
- Pishesha, Novalia & Harmand, Thibault & Ploegh, Hidde. (2022). A guide to antigen processing and presentation. Nature Reviews Immunology. 1-14. 10.1038/s41577-022-00707-2.
- Santambrogio, L., Berendam, S. J., & Engelhard, V. H. (2019). The Antigen Processing and Presentation Machinery in Lymphatic Endothelial Cells. Frontiers in Immunology, 10. doi:10.3389/fimmu.2019.01033
- Vyas JM, Van der Veen AG, Ploegh HL. The known unknowns of antigen processing and presentation. Nat Rev Immunol. 2008 Aug;8(8):607-18. doi: 10.1038/nri2368. PMID: 18641646; PMCID: PMC2735460.
- Roche PA, Cresswell P. Antigen Processing and Presentation Mechanisms in Myeloid Cells. Microbiol Spectr. 2016 Jun;4(3):10.1128/microbiolspec.MCHD-0008-2015. doi: 10.1128/microbiolspec.MCHD-0008-2015. PMID: 27337472; PMCID: PMC6300129.
- Vyas, J., Van der Veen, A. & Ploegh, H. The known unknowns of antigen processing and presentation. Nat Rev Immunol 8, 607–618 (2008). https://doi.org/10.1038/nri2368
- https://www.ndvsu.org/images/StudyMaterials/Micro/Antiigen-Processing-and—Presentation.pdf
- https://mgcub.ac.in/pdf/material/20200429090459d03efc9cf1.pdf
- https://currentprotocols.onlinelibrary.wiley.com/doi/toc/10.1002/(ISSN)1934-368X.AntigenProcessingandPresentation
- https://www.lkouniv.ac.in/site/writereaddata/siteContent/202004131505182831sudhir_biochem_Antigen_Processing.pdf
- https://www.davuniversity.org/images/files/study-material/BCH327-7.pdf
- https://njms.rutgers.edu/sgs/olc/mci/prot/2009/AgProcPresent2009.pdf
- https://www.slideshare.net/rajpalchoudharyjat/antigen-processing-and-presentation
- https://www.microbiologybook.org/mobile/m.immuno-11.htm
- https://www.genome.jp/entry/hsa04612
- https://www.thermofisher.com/in/en/home/life-science/antibodies/antibodies-learning-center/antibodies-resource-library/cell-signaling-pathways/antigen-processing-presentation-mhc.html
- https://teachmephysiology.com/immune-system/adaptive-immune-system/antigen-processing-presentation/
- https://step1.medbullets.com/immunology/105065/antigen-processing-and-presentation
- https://www.onlinebiologynotes.com/antigen-processing-and-presentation-cytosolic-and-endocytic-pathway/