What is Stem cell?
- Stem cells are body cells that have the extraordinary potential to develop into multiple cell types or divide endlessly to make new stem cells. They are critical in replacing damaged or destroyed cells as a result of illnesses. Stem cells are the earliest cells in the cell lineage of all tissues and can be found in both embryonic and adult organisms.
- Embryonic stem cells are present in the blastocyst’s inner cell mass, which is the early stage of development. These cells have the ability to develop into any form of cell in the body. Adult stem cells, on the other hand, are found in specific locations such as the bone marrow and the gonads. These cells have a lower differentiation capacity but can nevertheless give rise to specialized cells in some organs.
- Stem cells are gaining popularity in the medical industry as a potential therapy option for a variety of diseases and ailments. They can mend damaged cells and have a high potential for regeneration. Stem cells can differentiate into a variety of cell types, including muscle cells, brain cells, and others.
- The human body has about 200 cell types, all of which originate from a fertilized egg formed by the union of an ovum and sperm. Different types of stem cells give rise to specialized cells such as skin, blood, muscle, liver, and nerve cells during early development and throughout life.
- Scientists have been studying stem cells to learn how they differentiate into different types of cells. This understanding is critical for creating novel medical treatments that target these cells or repair cells that have been lost or destroyed. The regenerative capabilities of stem cells suggest that they could be used to treat diseases such as diabetes, heart disease, and degenerative ailments.
- More research, however, is required to fully realize the potential of stem cells for cell-based therapeutics. Scientists are actively looking at how to best use these cells and their healing abilities. Stem cell research also aids in the development of new treatments, the study of normal developmental processes, and the comprehension of the genesis of animals from a single cell.
- While stem cell research is fascinating, it also raises numerous questions. Scientists are constantly attempting to learn more about stem cells and how they differ from other types of cells. The field shows enormous promise, but further research and development are required to attain its full potential in the realm of medicine.
Stem Cells Definition
Stem cells are unique cells in the body that have the potential to develop into different types of specialized cells or divide to produce more stem cells.
Properties of Stem Cells
Stem cells possess several intrinsic properties that make them unique and give them remarkable capabilities:
- Undifferentiated and Unspecialized: Stem cells are undifferentiated and do not possess tissue-specific features that allow them to perform specialized functions. They are in an unspecialized state and have the potential to become any type of cell in the body.
- Self-Renewal: Stem cells have the ability to divide and generate identical copies of themselves. This process is known as self-renewal and ensures the maintenance of the stem cell population. Through self-renewal, stem cells can continually produce more stem cells while also preserving their undifferentiated state.
- Differentiation: Stem cells have the capacity to differentiate into specialized cells with specific functions. Through the process of differentiation, an undifferentiated stem cell gives rise to a specialized cell, such as a brain cell, muscle cell, or red blood cell. Different types of stem cells have the potential to differentiate into specific cell lineages. For example, hematopoietic stem cells give rise to all blood cells, neural stem cells give rise to neurons, astrocytes, and oligodendrocytes, and mesenchymal stem cells differentiate into fibroblasts, osteoblasts, and chondroblasts.
- Homeostasis: The balance between self-renewal and differentiation is essential for tissue homeostasis. Stem cells play a crucial role in maintaining tissue health and function by continuously renewing and replacing damaged or lost cells. Their ability to self-renew and differentiate in response to specific signals helps to regulate the overall balance and integrity of the tissue.
These properties of stem cells contribute to their regenerative potential and make them invaluable in various fields, including medicine and research. Stem cells hold promise for treating diseases, generating new tissues and organs, and understanding the mechanisms of development and tissue regeneration.
Types of Stem Cells Based on their potency or differentiation ability
On the basis of their potency or capacity for differentiation, stem cells can be categorised into five major groups.
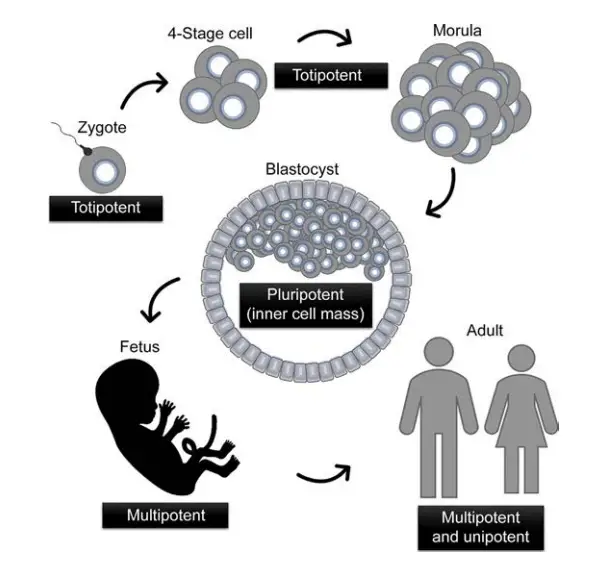
1. Totipotent Stem Cells
- These are stem cells that can differentiate into any cell type in an organism, including extraembryonic tissues.
- They have the ability to develop into a completely viable embryo and subsequently into a fully functional creature.
- The fertilised egg or zygote is regarded as the first stem cell and is said to be totipotent (from the Latin word totus, meaning entire) since it has the ability to develop all the cells and tissues that comprise an entire organism.
- As the zygote splits and differentiates further, the cells lose their regenerative capacity until they reach the eight-cell stage, at which point they are no longer totipotent. Later on, embryonic cells retain pluripotency, which allows them to differentiate into all 210 types of body cells, with the exception of the extraembryonic tissues of the placenta and umbilical cord. All of these cells descended from a single totipotent zygote or fertilised egg cell.
- Examples of totipotent stem cells are zygote cells.
2. Pluripotent Stem Cells
- Pluripotent refers to stem cells that can generate cells generated from each of the three embryonic germ layers (ectoderm, mesoderm, and endoderm).
- These three germ layers serve as the embryonic origin of all body cells. All the various types of specialised cells in the body are developed from one of these germ layers (“pluri,” derived from the Latin plures, meaning several or many).
- Consequently, pluripotent cells are capable of giving rise to any type of cell, a characteristic shown during embryonic development and under specific laboratory settings.
- They have the ability to differentiate into any foetal or adult cell type, but cannot create embryos (e.g., embryonic stem cells).
- Examples of Pluripotent Stem Cells are ES cells, iPs cells, gPS cells, pES cells, and foetal stem cells.
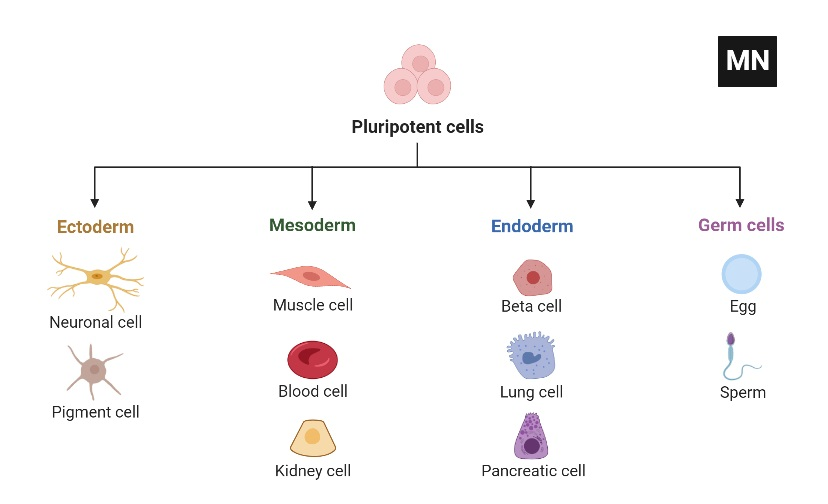
3. Multipotent Stem Cells
- Typically, these are progenitor cells that can differentiate into a restricted number of cell types.
- This group includes somatic cells such as neural, bone marrow-derived, and hematopoietic stem cells (HSCs).
- Multipotent stem cells include adult stem cells like HSCs and neural stem cells.
4. Oligopotent Stem Cells
- These stem cells often stay in the tissue and can develop into specialised tissue cells.
- A recent study, for instance, revealed the ubiquitous presence of oligopotent stem cells on the ocular surface of mammals.
5. Unipotent Stem Cells
- Despite possessing the capability of self-renewal, unipotent stem cells are often capable of differentiating into a single cell type.
- This is a phrase typically used to describe a cell in adult organisms, indicating that these cells can differentiate into only one cell lineage.
- The origin of “uni” is the Latin word unus, which signifies one. In many developed, undamaged organs, adult stem cells are often unipotent and generate only one cell type under normal conditions.
- This technique would permit the tissue to maintain a continuous state of self-renewal. Pluripotent stem cells may be activated to heal damaged tissue if the replacement of many cell types is required.
- Pluripotency is a characteristic of embryonic stem cells (i.e. it can give rise to cells derived from all three germ layers).
- In a recent study, the significance of unipotent progenitor cells to postnatal prostate development was successfully highlighted.
Stem Cell Classes on the Basis of Potency
Type | Characteristic | Example |
Totipotent | Up to 8-cell stage produced after fusion of egg and sperm; each can give rise to embryonic as well as extra embryonic tissues (total organism). Ability to differentiate into cell linages from all three germ layers: mesoderm, endoderm, and ectoderm including placental cell. | cells of the zygote |
Pluripotent | Descendents of totipotent cells. Embryonic stem cells. Induced pluripotent stem cells. Ability to differentiate into cells from all three germ layers with the exception of placenta. | ES cells, iPs cells, gPS cells, pES cells, and fetal stem cells |
Multipotent | Tissue-specific family of stem cells such as hematopoietic stem cells (HSCs) and mesenchymal stem cells (MSCs). Ability to differentiate into a limited number of types from one germ layer. | adult stem cells such as HSCs and neural stem cells |
Unipotent | These cells can produce only one type of cells (e.g. muscle stem cells or spermatogonial stem cells). Ability to produce cells of their own type. | stem cells present on the mammalian ocular surface |
Oligopotent | Ability to differentiate into a few type of cells | progenitor cells present during postnatal prostate development |
Types of Stem Cells Based on Source/location
Based on the preceding classification, our body contains many types of stem cells, which are addressed in greater detail in the following sections.
1. Adult Stem Cells
- Adult stem cells are present in mature tissues and serve a function in the lifelong maintenance and growth of these tissues.
- They are commonly multipotent and are also known as tissue progenitor cells.
- Numerous adult tissues have stem cell or progenitor cell populations, including bone marrow/peripheral blood (hematopoietic stem cells), gastrointestinal tract, brain, skin, muscle, nerve cells, liver, eye, pancreas, and dental pulp.
- Typically, they are tissue-specific and can only differentiate into cell types found in the tissue in which they reside.
- Their microenvironment or niche governs their behaviour. Hematopoietic stem cells, which are CD34+, are among the most extensively investigated and utilised cell types for therapeutic applications.
- Multipotent mesenchymal stem cells (MSCs), which are produced from the stroma of bone marrow, deviate from the tissue-specific nature of adult stem cells.
- Adult multipotent progenitor cells are capable of differentiating into a range of tissues, including neuronal, adipose, muscle, gut, liver, lungs, and spleen cells, but not bone marrow or gonad cells.
- When injected into a blastocyst, they can also undergo developmental differentiation.
- Under optimal growing conditions, one kind of adult stem cell is capable of differentiating into a different type of tissue. This quality is referred to as “plasticity.”
2. Fetal Stem Cells
- The foetus has pluripotent stem cells that contribute to the initial development of all tissues before birth.
- These include umbilical cord blood (UCB), amniotic fluid (AF), Wharton’s jelly, amniotic membrane, and the placenta, which are derived from either foetal tissue or supportive extra-embryonic tissue.
- As these tissues are often eliminated after birth, there are no ethical considerations regarding their stem cell populations.
- In terms of their capacity for self-renewal and differentiation, they are comparable to embryonic and adult multipotent stem cells.
- Next, each type of foetal stem cell is discussed in further depth.
a. Umbilical Cord Blood Stem Cells
- Multipotent umbilical cord blood (UCB) cells are present in umbilical cord blood. They have genetic similarities with the infant.
- Approximately 1% of the UCB cell population is CD34, CD34+ (a marker for hematopoietic stem cells), and CD38 (which is a marker for immune cells such as white blood cells).
- These cells have achieved differentiation and self-renewal capabilities. In addition, cord blood is a significant supply of MSCs, which promote the proliferation of HSCs in vivo.
- Cord blood cells, the second most important source of MSCs after bone marrow, contain a subpopulation of cells that express neural markers and may function as neuronal cell progenitors.
b. Amniotic Fluid Stem Cells
- Amniotic fluid (AF) contains a heterogeneous population of cells from each of the three germ layers, including a number of partially differentiated cell types and progenitors.
- These cells arise from the foetus or the inner surface of the amniotic membrane and are predominantly epithelial in nature.
- AF cells isolated from earlier gestational periods display larger amounts of mesodermal and endodermal markers than their later counterparts, whereas ectodermal marker expression remains unchanged.
- MSCs and other multipotent stem cells expressing stem cell-specific markers such as Oct4, SSEA4, and Nanog are also present.
- AF-MSCs can differentiate into numerous cell types, including adipocytes, chondrocytes, osteocytes, hepatocytes, neuronal cells, and cardiomyocytes.
c. Wharton’s Jelly Stem Cells
- Umbilical cord matrix stem cells (UCMSCs), umbilical cord perivascular cells (UCPVs), and umbilical cord stromal cells make up Wharton’s jelly cells (UCSCs).
- It is known that UCMSCs enhance muscle formation; UCPVs lead to a population of MSCs that differentiate into bone nodules; and UCSCs differentiate into a variety of lineages with the exception of neuronal type 1 cells.
d. Amniotic Membrane Stem Cells
- The amniotic membrane is formed from the epiblast and is composed of three layers: epithelial cells (inner layer), mesenchymal cells (outer layer), and a layer devoid of biological components (intermediate layer).
- These cells can develop in vitro into cardiomyocytes, hepatocytes, and endothelial cells since they are derived from the epiblast.
e. Embryonic Stem Cells
- Embryonic stem (ES) cells are derived from the inner cell mass of a blastocyst and are pluripotent. In other words, they are capable of unlimited self-renewal in addition to being able to maintain an undifferentiated or pluripotent condition.
- When they are reintroduced into the blastocyst, they also possess the unusual potential to colonise cells of several lineages, including the germline.
- In addition to their pluripotent potential in vivo, these cells can also self-renew and differentiate in vitro.
- Additionally, ES cells express alkaline phosphatase. They are produced from the inner cell mass (ICM) of the blastocyst and are widely used.
- ES cells are generated during embryonic development from the cleavage of the zygote, which typically occurs prior to the formation of the blastocyst.
- Typically, they are called totipotent, as they can differentiate into every type of cell in the body and produce a full creature.
- In recent years, ES cells have been produced from numerous mammalian species. In 1981, the first mammalian ES cells were generated from a mouse blastocyst.
- They were capable of differentiating into cells of all three germ layers, could create teratomas when injected into immunocompromised mice, were karyotypically normal, and could generate chimaeras.
- A successful ES cell line has also been created from the nonhuman primate Rhesus monkey.
- Other than the presence of the enzyme alkaline phosphatase, the cells exhibited all the characteristics that define pluripotency, including self-renewal, the ability to differentiate into all three germ layers, the maintenance of a normal karyotype, and the ability to form tumours when injected into immunocompromised mice.
- In 1998, the first ES cell line was successfully produced from frozen embryos obtained through in vitro fertilisation in humans.
- They met all the criteria for pluripotency and have made significant contributions to stem cell research during the past few years.
- Other species’ blastocysts, such as rats’, have also been used to produce ES cells, which have effectively contributed to germline transmission and the generation of chimaeras.
Facts of Embryonic Stem Cells
- Derived from the inner cell mass of a blastocyst (4–6 days old fertilised human egg).
- Can be obtained from embryos fertilised in vitro or those that have been aborted.
- Capable of differentiating into each of the body’s 210 cell types and three germ layers (ectoderm, mesoderm, and endoderm).
- Self-renewing capacity; pluripotent. Have the capacity to replace injured or destroyed organs or tissue.
3. Parthenogenetic Embryonic Stem Cells
- Using substances such as ethanol, parthenogenetic embryonic stem (pES) cells were originally discovered in mice by activating haploid embryos parthenogenetically.
- Parthenogenesis is a procedure that often occurs in lower species to produce a living organism directly from an egg without sperm activation.
- This technique produces mouse embryos that can only survive to the blastocyst stage.
- In addition to non-human primates and mice, embryonic stem cell lines have been successfully produced from human blastocysts.
- In addition to contributing to the development of chimaeras, they exhibit ES-like characteristics and pluripotency when cultivated under suitable conditions.
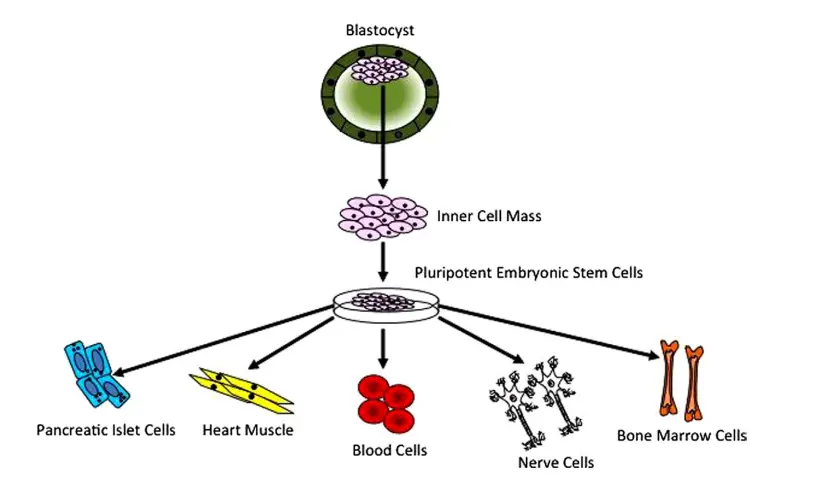
4. Germline-Derived Pluripotent Stem Cells
- When formed as germline stem cells, germline-derived pluripotent stem (gPS) cells are often produced from unipotent spermatogonial stem cells (SSCs) (GSCs).
- When these GSCs are cultivated under conditions similar to those of ES cells, they give rise to pluripotent stem cells known as germline-derived pluripotent stem (gPS) cells.
- Recent investigations have demonstrated the isolation of GSCs from testicular biopsies of adult mice aged 5–7 weeks.
- Eventually, these procedures can be utilised to harvest GSCs from human testicular biopsies, which will have significant therapeutic promise.
5. Induced Pluripotent Stem Cells
- In 2006, Yamanaka et al. were the first to create induced pluripotent stem (iPS) cells from somatic cells by inducing pluripotency; this approach has transformed stem cell research.
- Using retroviral vector constructs, Yamanaka and coworkers successfully reprogrammed embryonic or adult mouse fibroblasts by introducing four factors: Oct4, Sox2 (SRY, or sex-determining region Y) box 2, c-Myc, and Klf4.
- These cells share the same characteristics as ES cells. They are both pluripotent and capable of self-renewal.
- When injected into immunocompromised mice, they lead to the development of teratomas and all three germ layers.
- The addition of OCT4, SOX2, NANOG, and LIN28 will also promote pluripotency in mature human fibroblasts. These human iPS cells have the same capacity for self-renewal and pluripotency as human ES cells.
- They have also been produced from cells of a number of other animal species, such as pigs and cattle, and may have significant therapeutic significance. Various techniques are used to create pluripotent stem cells induced by artificial means.
- Viral transduction can be used to reprogram somatic cells (retroviral (lentiviral) and adenoviral). Retroviruses, such as lentiviruses, pose a danger of insertional mutagenesis due to genomic integrations, whereas adenoviruses generate iPS cells less efficiently and require repeated transductions.
- Recent research has demonstrated that Sendai viruses, which may be eliminated by antibody-mediated negative selection after reprogramming, are effective for reprogramming.
- Several nonviral methods of gene delivery have also been employed for reprogramming, such as nucleofection of nonviral polycistronic constructs and subsequent removal of the reprogramming components using a Lox/Cre system, as well as the use of episomal vectors such as the piggyback transposon.
- Typically, these nonintegrating approaches are less effective for reprogramming. As they all entail the exploitation of genetic material, the majority of the aforementioned techniques are unfit for therapeutic reasons.
- Consequently, fresh reprogramming procedures including the use of mRNAs for the delivery of reprogramming factors for the formation of iPS cells have also been described.
Stem cells | Source | Characteristic |
Embryonic stem cells (ESC) | Blastocysts | • Pluripotent • Endless proliferation • Use ethically questionable in many countries • Due to the lack of complete immunocompatibility, organs and tissues generated from them, will likely be immunorejected • Risk of teratoma formation upon injection in undifferentiated state |
Adult stem cells (ASC) | Adipose tissue/bone marrow/peripheral blood | • Multipotent • No risk of rejection during autotransplantation • Less/no risk of tumor formation • Limited number in tissue |
Induced pluripotent stem cells (iPS) | Virtually any type of cells, most commonly: fibroblasts, keratinocytes, peripheral blood mononuclear cells | •Pluripotent • Obtained through reprogramming of somatic cell • Low rate of reprogramming • No ethical problems • Personal regenerative medicine • Low risk of immune rejection • Risk of teratoma formation upon injection in undifferentiated state |
Are Stem Cells Immortal?
- In contrast to the majority of adult differentiated cells, which perish due to natural programming (homeostasis) or injury, the stem cell compartment that refills them must survive for the duration of an organism’s lifetime.
- Consequently, these long-lived cell populations must be able to withstand two major biological phenomena: (1) the absence of DNA damage that causes gene mutations, and (2) a normal senescence mechanism.
- Tissues of long-lived creatures are more sensitive to DNA damage from environmental agents or metabolic byproducts, such as reactive oxygen species.
- Since mutations can lead to cancer, they must be either repaired by gene repair processes or eliminated by cell death.
- Non-random chromosomal segregation appears to be one of the techniques utilised by stem cells to prevent mutations. This hypothesis suggests the segregation of the original (“immortal”) DNA template to the stem cell derived through asymmetric division, while the newly synthesised strands (more susceptible to replication-induced mutation) are handed on to the daughter cells committed to differentiation.
- On the other hand, senescence is a physiological characteristic of normal cells. Most normal human cells, when cultivated in vitro, are programmed for a specific number of cell divisions (the “Hayflick limit”) due to gradual telomere shortening, which results in replicative cell senescence.
- However, immortal cells, such as cancer cells and stem cells, have increased telomerase activity, allowing them to multiply indefinitely.
- Understanding the biological processes that regulate asymmetric cell dynamics may hold the key to deciphering the “immortal DNA strand theory” for stem cell vitality/viability.
What do you mean by Stem Cell Plasticity?
- In general, plasticity refers to the ability of adult stem cells to traverse lineage boundaries and adopt the expression patterns and functional phenotypes of cells specific to other tissues. A commonly accepted definition of plasticity has yet to be defined (i.e. they differentiate into multiple cell types).
- For example, hematopoietic stem cells can differentiate into skeletal muscle cells, cardiac muscle cells, liver cells, or brain cells. Blood and skeletal muscle cells can differentiate from bone marrow stromal cells.
Mechanisms of Stem Cell Plasticity
- These events could be explained by a variety of plausible explanations. In reaction to environmental stimuli, transdifferentiation of a cell straight into another cell type is one possibility.
- Transdifferentiation has primarily been demonstrated in vitro. Additionally, some in vivo results support this approach.
- Direct trans-differentiation is therapeutically constrained by the number of cells that can be put into an organ without removing the existing cells.
- However, if bone marrow cells could generate stem cells of another tissue, they should be able to repopulate entire organs from a small number of beginning cells.
- This notion of de-differentiation is consistent with animal model findings. The genetic study of donor cells has revealed an additional potential pathway.
- Without trans- or de-differentiation, the union of host and donor cells can produce mature tissue cells.
- The generated heterokaryons are capable of curing a fatal genetic flaw and do not appear cancer-prone.
- The definitive showing of stem cell plasticity should be a direct demonstration of nuclear reprogramming from one stem cell destiny to another.
Sources of Stem Cells
There are several significant sources from which a substantial number of stem cells can be extracted:
Embryos
- During embryonic development, embryonic stem cells (ESCs) are produced from the inner cell mass of the blastocyst.
- Embryonic stem cells are capable of differentiating into any cell type and self-replicating for multiple generations.
- A possible drawback of ESCs is their capacity to multiply indefinitely if not managed.
Fetal Tissue
- These embryonic germ cells (EGCs) are produced from cells in the gonadal area of a foetus that was aborted.
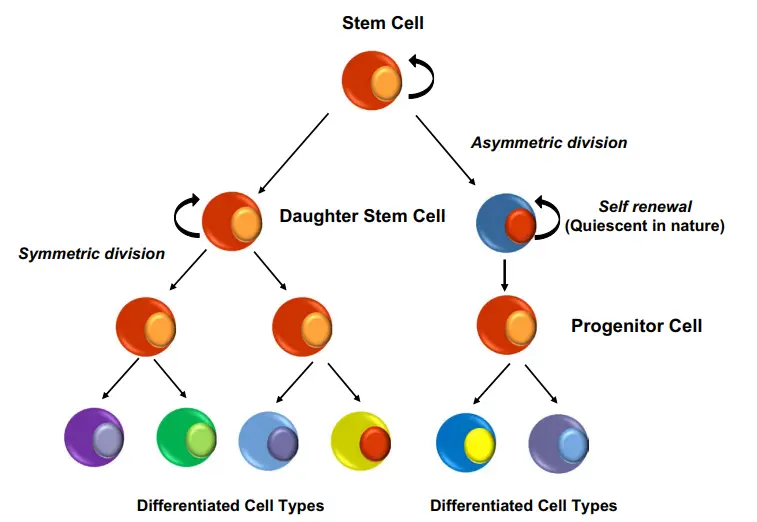
Amniotic Fluid-Derived Stem Cells (AFSCs)
AFSCs can be extracted from amniocentesis aspirates during genetic testing. Numerous investigations have shown that AFSCs are capable of extraordinary proliferation and differentiation into numerous lineages, including chondrocytes, adipocytes, osteoblasts, myocytes, endothelial cells, neuron-like cells, and liver cells.
Placental Tissue
- This is a unique source of a variety of stem cell populations, including mesenchymal, hematopoietic, and trophoblastic stem cells, and they may be more primitive stem cells.
Umbilical Cord Blood Stem Cells (UCBSCs)
- These stem cells are derived from the umbilical cord’s blood. The capacity of these cells for self-replication and multi-lineage differentiation is gaining increasing attention.
- Stem cells from umbilical cord blood can differentiate into numerous cell types, including liver, skeletal muscle, neural tissue, pancreatic, immunological, and mesenchymal stem cells.
Where Do Stem Cells Come From?
From the earliest stages of human development to the end of life, stem cells are present. All stem cells have the potential to be useful, but each type has both promise and limitations. The genesis or lineage of stem cells in embryonic stem (ES) cells is well recognised; the origin of stem cells in adults is less apparent and in some cases debatable. It is possible that embryonic stem (ES) cells arise prior to germ layer commitment, suggesting that this may be a mechanism for the generation of multipotent stem cells, including adult stem cells. Several sources of stem cells have been identified, including embryonic, foetal, and adult stem cells, each of which has unique features.
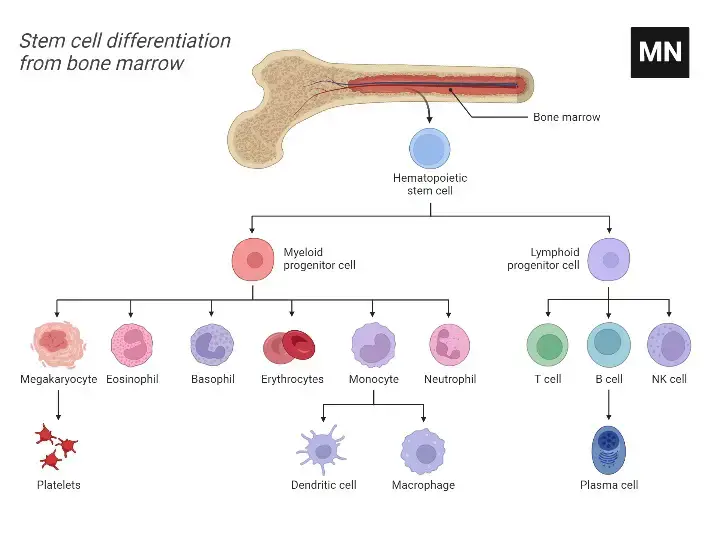
Embryonic Stem Cells (ESCs)
- When a sperm fertilises an egg, a zygote is formed, which is the ultimate stem cell since it can develop into any cell — not just embryonic cells, but also cells of surrounding tissues such as the placenta.
- The zygote up to the 8-cell stage has the highest degree of trans-differentiation and is referred to as a totipotent stem cell, meaning the totipotent cells are the initial stage stem cells as the zygote develops into embryonic and extra embryonic organs.
- The zygote begins to divide thirty hours after fertilisation, and by the fifth or sixth day, the cells have become a blastocyst.
- A blastocyst is a preimplantation embryo that possesses all the necessary components for the development of a fully formed human.
- The blastocyst is predominantly a hollow cell sphere. Within its interior is the inner cell mass, which consists of 30–34 pluripotent cells that are capable of differentiating into all cell types of the body.
- These cells have less differentiation potential and are more specialised than totipotent zygote stem cells.
- Those on the outer surface of the blastocyst differentiate into placental and other tissues that surround the foetus, while those within are known as the inner cell mass or embryonic stem cells.
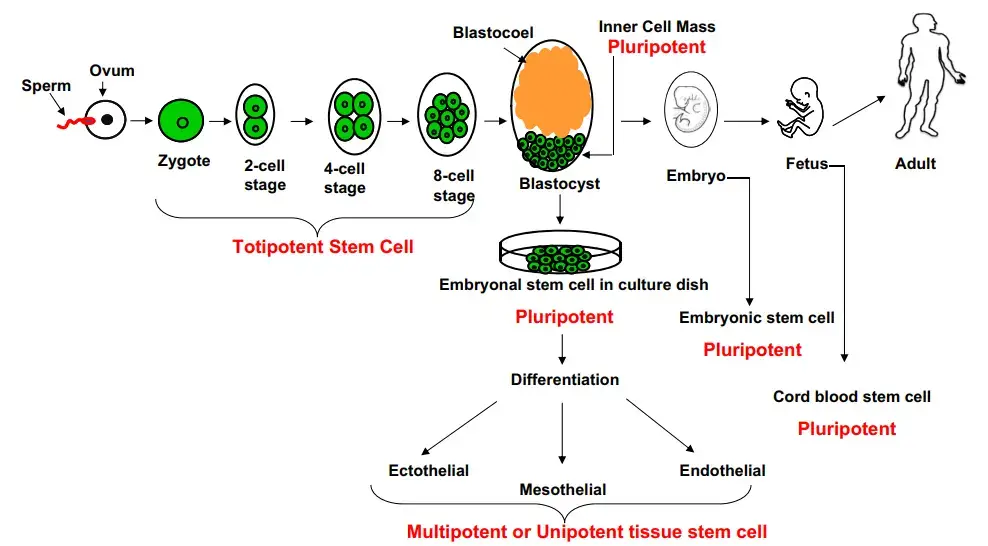
Embryonic Germ Cells (EGCs)
- Five to nine weeks after fertilisation, the embryo, now known as the foetus, develops an area called the gonadal ridge.
- The gonadal ridge contains the germ cells from which eggs and sperm will eventually form.
- These primordial germ cells of the five-to-nine-week-old foetal tissue resulting from elective abortions are used to separate embryonic germ cells. EGCs, like ESCs, are multipotent.
- As the embryo grows, it acquires more embryonic stem cells in the yolk sac; from embryonic and foetal stem cells eight weeks old, the foetus creates tissues and organs.
- At this stage, stem cells are more tissue-specific than they are responsible for producing all cell types in the body.
- In the liver, for instance, foetal stem cells tend to produce liver and blood cell families. Typically, such cells are referred to be multipotent.
- However, foetal cells may have an advantage over embryonic stem cells in that they are less likely to develop teratomas. It has been demonstrated that foetal liver tissue is a rich source of stem cells.
- Until week 12, foetal stem cells (as well as the ES cells that preceded them) have the crucial virtue of not being rejected when transplanted into a human.
- This is because their surface contains little or no immunogenic protein (Class II HLA).
- After the 12th week, when foetal stem cells begin to express these immune-stimulating proteins, the foetus has the ability to reject tissues.
- Therefore, stem cells produced from these sources may only have therapeutic potential when administered to the individual from whom they were extracted (autologous transplantation) or to an immunologically compatible donor (allogenic transplantation).
Adult Stem Cells (ASCs)
- The term adult stem cell refers to the cells seen in adult organs that replenish the somatic cells in the tissue of their origin on a continuous basis.
- For a thorough evaluation of adult stem cells, it is necessary to purify a population of cells using cell surface markers, transplant a single cell from the purified population into a syngeneic host without in vitro culture, and observe self-renewal and tissue or organ regeneration to determine their multipotency.
- Under pathological conditions or after an injury, these cells have a potent capacity to regenerate and replace ageing or diseased cells in the tissue in which they dwell. ASCs in living creatures serve primarily to maintain and repair the tissue in which they are present.
- These cells have a limited capacity for differentiation, hence they are referred to as multipotent stem cells. ASCs are present in numerous body tissues and can be exploited for transplantation.
- Certain types of ASCs appear to be capable of differentiating into a variety of cell types. If this differentiation of ASCs can be controlled in the laboratory, these cells could serve as the basis for treatments for a variety of serious, prevalent disorders.
- These ASCs exist in a specific region of each tissue, where they stay quiescent (non-dividing) for many years until awakened by a disease signal or tissue damage.
- It is considered that embryonic and adult stem cells are the only two forms of stem cells.
- Induced pluripotent stem cells are a “new” type of high-potential stem cell that has been developed recently (iPSCs).
- Using a procedure known as nuclear reprogramming, scientists have effectively converted ordinary adult cells into stem cells. By modifying the somatic activity of the gene in adult cells, it is feasible to reprogram the cells to behave like embryonic stem cells.
- This innovative method would aid in avoiding the controversy surrounding embryonic stem cells and prevent immunological rejection.
- Researchers have been able to transform normal connective tissue cells to become heart cells.
- Injecting these fresh cardiac cells into animals with heart failure enhanced heart function and prolonged survival.
- This clever method has cleared the path for the manipulation of somatic cells into egg and sperm. It offers a potential remedy for infertility as well as choices for motherhood for same-sex couples and individuals with sterility.
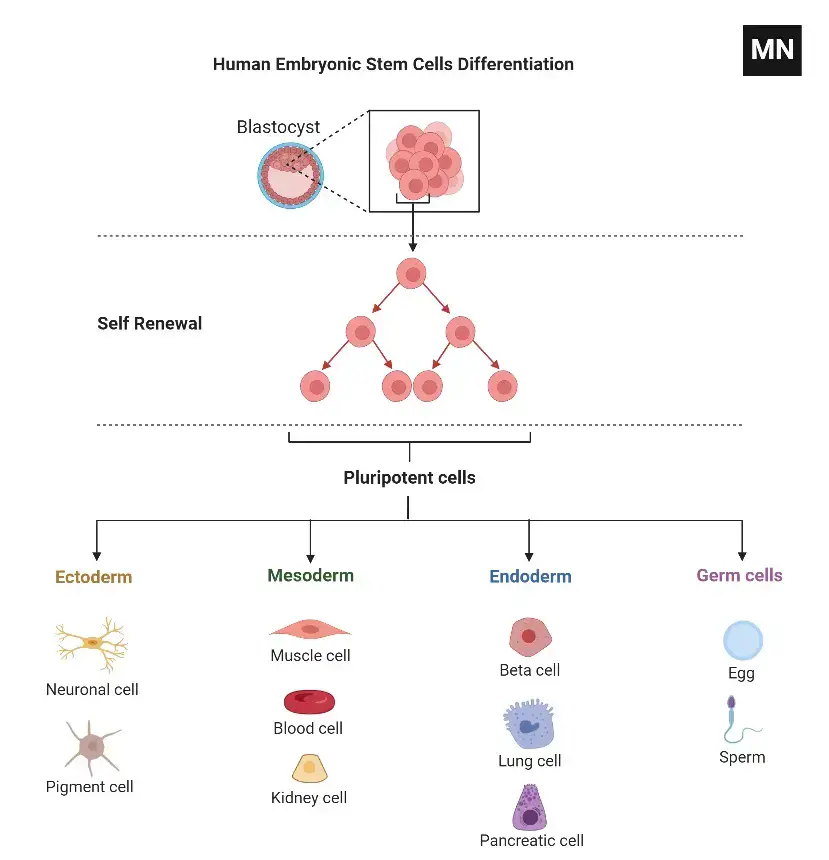
Stem Cell Culture Methods
- The physiological, pharmacological, and biological evaluation of stem cells at the tissue and molecular levels requires cell culture techniques.
- Stem cells are typically grown in an incubator at 37 °C with 5% CO2 and 95% O2.
- Stem cell maintenance in an undifferentiated state is dependent on the composition of the cell culture media. Fetal bovine serum is one of the most often utilised components in stem cell cultivation (FBS).
- This has a number of drawbacks, including batch-to-batch variation, the existence of unknown components such as hormones and growth factors (which may cause issues in cell culture), etc.
- The risk of transmitting proteins of animal origin to the patient is another key issue.
- Thus, scientists have shifted to serum-free culture conditions to make stem cell cultivation more applicable for clinical usage.
- There are numerous culture systems available for stem cell maintenance and storage. To separate MSCs from BM stroma, for instance, one of the proposed methods is the addition of 10ml BM suspension to 20ml of DMEM (Dulbecco’s modified essential medium) containing 10% FBS; this solution can be centrifuged to separate the fat layer, and the cell pellet can then be subjected to density gradient centrifugation for 20min at 13,000 g using a 70% Percoll solution.
- The MSC-enriched low fraction can be grown in DMEM supplemented with 10% FBS at 37 °C, 5% CO2 and 95% O2 in a humidified incubator.
- For subsequent passage, the cells can be enzymatically disseminated to single cells using 0.25 % trypsin and 1 mM ethylenediaminetetraacetic acid (EDTA) and replated in DMEM supplemented with FBS.
- MSCs can be resuspended in 90% FBS and 10% DMSO (dimethylsulfone) for cryopreservation by gradual freezing and long-term storage in liquid nitrogen.
- MSCs can be extracted, maintained, and grown using xeno-free materials such as serum-free MesenCult-XF™, TC-protector™, and recombinant trypsin (TrypLESelect™) for uses in regenerative medicine.
For fetal stem cells
- Depending on the cell type, culturing procedures for foetal stem cells vary. In normal, full-term pregnancies, umbilical cord blood can be extracted from newborn umbilical cords.
- It can then be sedimented for 40–60 minutes with hydroxyethyl starch.
- Mononucleocytes are isolable by density gradient centrifugation at 400 g for 30 minutes, followed by further washing in phosphate buffer saline and resuspension in buffer solution.
- Positive selection employing commercially available immunomagnetic separation techniques can further isolate CD34+ cells.
- These separated cells can be counted and grown in 10% FBS-supplemented Iscove’s Modified Dulbecco’s Medium (IMDM).
- To separate MSCs from umbilical cord blood, the umbilical cord can be cleaned with phosphate buffer saline, disinfected in 75% ethanol, and chopped and digested further.
- The cells may then be cultivated in DMEM/F-12 media supplemented with 10% FBS and 10ng/ml epidermal growth factor (EGF) and passed using trypsin.
- The cells can be resuspended in the standard cryo-preservant solution, which consists of 90% FBS and 10% DMSO, for cryo-preservation.
For ES cells
- For the maintenance, cultivation, and cryopreservation of ES cells, numerous techniques are available. Those utilised for ES cell culture are often applicable to iPS cell culture as well.
- Initially, human ES cells were isolated and cultured in a solution that could be supplemented with foetal calf serum and grown on a mouse embryonic fibroblast feeder layer (MEF).
- However, many components in the serum are unclear, and the production quality of serum may vary between batches. Aside from this, the usage of animal components in culture medium can constitute a barrier to the clinical application of these cells.
- To tackle these issues, scientists have established more precise medium conditions for the cultivation and preservation of stem cells.
- These conditions consist of hESF9 (consisting of a basal medium supplemented with FGF-2 (fibroblast growth factor 2) and heparin sulphate), mTeSR1TM, and STEMPROTM, which are commercially available and use multiple chemicals and growth factors to facilitate signalling in the promotion of human ES cell growth.
- Numerous components, such as FGF, transferrin, albumin, cholesterol, and lipid combinations, are frequently added to the media.
- ES cells can be passed either enzymatically or mechanically (e.g., using dispase, accutase, collagenase, and trypsin, or with Life Technologies’ STEMPRO® EZPassageTM disposable stem cell passage equipment).
- The recovery of cryopreserved human embryonic stem (ES) cells is greatly enhanced by the addition of the ROCK inhibitor (Y-27632) following the freezing and thawing of the cells.
- The cells can be frozen in liquid nitrogen containing 60% KOSR (knockout serum replacement), 20% DMSO, and 20% DMEM.
For parthenogenetic ES cells
- For parthenogenetic ES cells, the isolated ICMs can be cultured for 5–9 days on mitomycin-C–treated mouse embryonic fibroblast cells (MEFs); primary ES cell colonies can then be dissociated mechanically and cultured in human ES cell culture medium containing 80% knockout-DMEM, 20% serum-replacement, 1mM glutamine, 1% nonessential amino acid, 0.1mM 2-mercaptoethanal, and 50UI/
For Human germ cells
- Human germ cells can be grown in gelatin-coated dishes containing DMEM with high glucose, 15% knockout serum replacement, 2mM L-glutamine, 0.1μM β-mercaptoethanol, 100 nonessential amino acids, 1 x penicillin-streptomycin, 10ng/ml bFGF, and 0.12ng/ml TGF-β (transforming growth factor-β).
- These settings result in the production of tiny, pluripotent colonies resembling ES cells after four days of cultivation.
- pES, gPS, and iPS cells can be cryopreserved similarly to human embryonic stem (ES) cells.
Stem Cells Identification, Isolation, And Characterized
The ultimate objective of stem cell research is to produce cell-based therapeutics for currently untreatable illnesses. To do this, methods must be developed to expand, characterise, and isolate sufficient numbers of purified stem cell populations of a certain lineage. When confronting the challenge of identifying, isolating, and defining a stem cell population, the following standards and criteria may be implemented.
Embyronic Stem Cell Identification
- Self-renewal, multi-lineage differentiation in vitro and in vivo, clonogenicity, a normal karyotype, considerable proliferation in vitro under well-defined culture conditions, and the ability to be frozen and thawed are the fundamental properties of an ES cell.
- In animal species, the ability of embryonic stem (ES) cells to contribute to all somatic lineages and produce germline chimerism permits a rigorous evaluation of in vivo differentiation.
- These cells must produce embryoid bodies and teratomas with differentiated cells from the three germ cell layers.
- Therefore, it must be demonstrated that ES cells express well-known molecular markers of pluripotent cells.
Adult Stem Cell Identification
- An adult stem cell is essentially a single (clonal) cell that self-renews and forms differentiated cells.
- Prospectively purifying a population of cells (typically by cell surface markers), transplanting a single cell into an acceptable host without intervening in vitro culture, and observing selfrenewal and tissue, organ, or lineage reconstitution constitutes the most stringent evaluation of these characteristics.
- Continued search for a particular molecular signature to define stem cells. Until then, stemness will be a concept with limited application but enormous potential.
Methods To Identify Stem Cells
- Today, a variety of techniques are being employed to uncover the stem cell’s mysteries. For instance, confocal microscopy can be used to examine their morphology.
- In addition to providing information about the size and shape of a cell, confocal microscopy can be used to analyse the interior structures and components of a cell in three dimensions.
- Monitoring the genetic integrity of the cells and measuring the expression of proteins linked with potency are the two basic types of stem cell analysis.
- Genomic analysis is required to determine whether stem cells maintained in culture for extended periods have experienced chromosomal loss, duplication, or epigenetic changes, whereas proteomic analysis assures that the cells are expressing the components required to sustain potency.
- Thus, characterization of the differentiated state by genomic and proteomic techniques guarantees the identification and proliferation of the correct stem cell population. The study of cellular differentiation and cell-fate specification requires molecular markers.
- To research the transition from one cell type to another, it is necessary to identify cell types with absolute certainty.
- Despite the fact that stem cells are best characterised by their function, a number of molecular markers have been utilised to distinguish between diverse stem cell populations.
- Although the activities of many of these early indicators remain unknown, their distinct expression pattern and timing provide a useful tool for identifying and isolating stem cells.
- In vitro and in vivo evidence supports the significance of particular markers in defining embryonic, hematopoietic, mesenchymal/stromal, and neural stem cell types. Listed below are some often utilised techniques:
A. By Cell Surface Marker Expression
1. Embryonic Stem Cell Markers
a. Oct-4
- Oct-4 (octamer-binding transcription factor 4) is a protein encoded by the POU5F1 gene in humans. It is also known as POU5F1 (POU domain, class 5, transcription factor 1).
- It was first identified as a DNA-binding protein that triggers gene transcription by use of a cis-element bearing an octamer pattern. It is expressed in embryonic stem cells and germ cells that are totipotent.
- This protein is essential for the self-renewal and pluripotency of undifferentiated embryonic stem cells.
- Not only is Oct-4 a master regulator of pluripotency that controls lineage commitment, but it is also the first and most widely recognised marker for identifying totipotent ES cells.
- It has been linked to adult germ cell cancer.
b. Stage-Specific Embryonic Antigens (SSEAs)
- Three monoclonal antibodies (Abs) targeting specific carbohydrate epitopes linked with lacto- and globo-series glycolipids initially identified SSEAs: SSEA-1, -3, and -4.
- SSEA-1 is expressed on the surface of preimplantation-stage (8-cell) murine embryos and teratocarcinoma stem cells, but not their differentiated counterparts.
- It has been postulated that these carbohydrate-associated molecules play biological roles in regulating cell surface contacts during development.
- SSEA-3 and SSEA-4 are expressed by undifferentiated primate ES cells, human EC and ES cells, but not SSEA-1.
2. Hematopoietic Stem Cell Markers
a. CD133
- CD133 is a member of the penta-span transmembrane glycoproteins (5-transmembrane, 5-TM) that localise exclusively to cellular protrusions.
- A freshly cloned CD133 isoform, AC133-2, has been identified as the initial surface antigen detected by the AC133 antibody.
- CD133 may offer an alternative to CD34 for the selection and growth of HSCs ex vivo. Recent studies have shown that CD133 expression is not restricted to primitive blood cells, but also defines unique cell populations in non-hematopoietic tissues, as well as hematopoietic stem cells, glioblastoma, neuronal and glial stem cells, and adult kidney, mammary gland, placental, digestive tract, and testicular cells.
b.CD34 (Sialomucin)
- CD34 (Sialomucin) is a single-pass transmembrane sialomucin protein found in early hematopoietic and vascular-associated tissue.
- It may facilitate the attachment of stem cells to extracellular matrix or stromal cells in bone marrow.
- It is regarded as the most important marker for hematopoietic stem cells (HSCs) because it was discovered on human bone marrow cells.
- It is also present on clonogenic progenitors and some committed-to-lineage cells.
- Osawa originally proved that CD34-negative mouse HSCs are possible. In transplantation trials, foetal sheep CD34 cells demonstrated repopulating activity.
- All clinical and experimental methods, including gene therapy and HSC transplantation, utilise CD34+-enriched cell populations at this time.
c. ABCG2
- ABCG2; commonly known as BCRP1 (CDw338), is a member of the ATP-binding cassette (ABC) transporters and the multi-drug resistance (MDR) protein family.
- ABCG2 appears to play a physiological role in the elimination of genotoxic chemicals from the body and from primitive repopulating cell populations.
- Only a fraction of the approximately 49 ABC proteins encoded by the human genome have been functionally described.
- According to phylogenetic study, they have been divided into seven subfamilies. It was initially discovered in breast cancer cell lines.
- It is a member of the half-transporter family and is exclusive to the plasma membrane.
- ABCG2 appears to be highly expressed in the mammary gland during pregnancy, in addition to a range of other tissues, including the intestine, brain, kidney, and placenta (and hematopoietic stem cells).
- Therefore, it is regarded a more promising marker than CD34 for the isolation and characterisation of primitive HSCs.
- It is extensively expressed in Side Population (SP) primitive stem cells. Based on the efflux of fluorescent dyes such as Rhodamine 123, Hoechst 33342, and Dye cycle violet, this SP phenotype was determined (DCV).
- Exclusive expression of ABCG2 on SP cells shows that ABCG2 may be a functional marker for positive selection of pluripotent stem cells from a variety of adult sources, and its functional involvement in stem cell biology has been postulated.
3. Mesenchymal/Stromal Stem Cell Markers
- mAb STRO-1 recognises the cell surface antigen STRO-1.
- It identifies mesenchymal stromal cells and nucleated erythroid precursors in bone marrow, but not committed hematopoietic progenitors.
- STRO-1 is an effective marker for identifying mesenchymal stem cells when combined with glycophorin A (GPA).
- The STRO-1+/GPA bone marrow cell subset has been found to serve as a universal stromal feeder layer for hematopoietic stem/progenitor cells.
- These subsets of cells are multipotent and differentiate into fibroblasts, adipocytes, osteoblasts, chondrocytes, and smooth muscle-like cells of the vascular smooth muscle.
4. Neural Stem Cell Markers
a. PSA-NCAM (Polysialic Acid-Neural Cell Adhesion Molecule or CD56)
- It is a homophilic binding glycoprotein whose expression regulation, along with that of its isoforms, is essential for numerous brain developmental processes.
- A neuronal-restricted precursor characterised by high PSA-NCAM+ expression is capable of self-renewal and differentiation into diverse neuronal phenotypes, whereas newborn brain precursors are constrained to a glial fate.
- Growing evidence suggests that the poly-sialic acid (PSA) part of NCAM is crucial for a variety of developmental processes, including cell migration, axon growth, nerve branching, and synaptic organisation.
b. Nestin
- Nestin is an intermediate filament protein of class VI. These intermediate filament proteins are largely produced in stem cells of the central nervous system (CNS), namely in nerve cells, where they play a role in axon radial expansion.
- However, its expression is typically ephemeral, indicating that it is involved in a crucial step in the brain differentiation route; it does not remain into adulthood.
- Nestin has been the most widely utilised marker for identifying CNS stem cells in diverse regions of the developing nervous system and in cells produced in vitro.
- Nestin expression has also been identified in non-neural stem cell groups, including follicular stem cells and their immediately differentiated progeny, pancreatic islet progenitors, and hematopoietic progenitors.
c. Low-Affinity Nerve Growth Factor Receptor
- Low-Affinity Nerve Growth Factor Receptor (also known as LNGFR or p75 neurotrophin receptor) is a type I trans-membrane protein that is a member of a broad receptor family that includes tumour necrosis factor receptors (TNFs), Fas, and roughly 25 other members.
- It is now known that p75NTR has a wide range of functions, from cell death regulation to axon elongation regulation.
- It binds with comparable affinity to nerve growth factor (NGF), brain-derived neurotrophic factor (BDNF), neurotrophin-3 (NT-3) and neurotrophin-4 (NT-4).
- Together with Trk receptors, p75NTR identifies neurotrophins and conveys trophic signals into the cell, indicating its essential role in the development of the nervous system.
- On the basis of their surface expression of p75NTR, neural crest stem cells (NCSCs) have been identified from peripheral nerve tissues. These NCSCs can self-renew and create neurons and glia both in vitro and in vivo.
- Additionally, in culture, neuroepithelial-derived p75NTR+ cells can develop into neurons, smooth muscle, and Schwann cells.
- Recently, p75NTR has been utilised as a marker for distinguishing mesenchymal progenitors and hepatic stellate cells.
B. By Dye Efflux Property
- In the lack of definitive cell surface markers, the side population (SP) phenotype can be used as an alternative to surface antigens.
- Hoechst 33342-stained cells and fluorescence-activated cell sorting (FACS) analysis can generate a profile in which cells that actively efflux the dye appear as a distinct population on the side of the dual-color emission spectra (blue versus red) FACS profile on a density plot; hence the name “Side Population” (SP).
- In accordance with tradition, the SP is determined by depletion using Hoechst transporter inhibitors reserpine, verapamil, or Fumitremorgin C.
- This SP phenotype is partially related to the activity of several adenosine triphosphate (ATP)-binding cassette (ABC) transporter family members (such as ABCG2), which are expressed in normal stem cells and the majority of cancer stem cells.
- ABC transporters are a superfamily of multi-span transmembrane proteins that may pump a variety of endogenous and exogenous chemicals, such as metabolic products, lipids and sterols, and medicines, out of cells, thereby shielding them from harmful agents.
- In addition, SP cells are in a quiescent condition and have an adherent endoplasmic reticulum with a small number of ribosomes, which is indicative of low metabolic activity and is characteristic of stem cells.
- This technique was initially employed to isolate mouse hematopoietic stem cells. Hoechst side population (SP) analysis has shown to be an effective technique for identifying and sorting putative stem and early progenitor cells in a wide variety of normal and malignant tissues across species since its initial application.
C. On the Basis of Functional Assays
1. Self-Renewal Assay
- Self-renewal is the process by which stem cells divide to produce additional stem cells, hence sustaining the stem cell pool throughout life.
- In 1996, it was discovered that undifferentiated multipotent neural stem cells could be cultivated and maintained as a neurosphere in suspension, marking a significant advancement in stem cell research.
- This anchorage-independent cultivation of stem cells as spheres was found to be useful for enlarging the populations of presumed stem cells when the particular markers for stem cell identification were unknown.
- This experiment entails the cultivation of stem cells dissociated as a single-cell suspension from tissues on nonadherent substrata in the presence of specified conditions that support the growth of stem cells until they form “spheres”
- What is the precise nature of the cells dividing within these spheres? How is the growth of cells in the sphere stopped after a particular number of cells has been reached? How is the homeostasis of spheres maintained? These are some of the intriguing questions that require investigation.
- Sphere clonality, which entails the development of a secondary clonal sphere from single cells acquired from the first sphere, was one of the principal drawbacks of this assay.
- This approach has the practical advantage of being able to cryopreserve the spheres for future study.
2. Slow-Cycling Population Assay
- Labeling of nucleotides by 5-bromo-2-deoxyuridine (BrdU) or by (3H) thymidine incorporation in proliferating cells for phenotypic determination via immunofluorescence or radiolabeling are standard approaches for detecting stem cell or progenitor cell populations in adult tissues.
- The phrase “slow-cycling test” is an idiom due to the unique stem cell characteristic of slow cycling.
- The premise of this assay is that cells with slow cycling times are distinguished by the retention of the label, which is integrated into DNA during DNA synthesis in cells with fast cycling rates.
- Once administered with a label, cells can be tracked for an extended period of time. As only slow-cycling cells are expected to retain the anti-BrdU antibody staining or radioactive label, these cells are referred to as “label-retaining cells or slow-cycling cells.”
3. Lineage Labeling Assay
- The lineage labelling test is the most effective and dependable method for detecting stem cells; it was originally created for understanding the evolution at the cellular level, such as cellular origin detection, lineage association, distribution pattern, and differentiation.
- In brief, a single cell is labelled in such a way that the label is tightly transferred to all of its daughters, resulting in a labelled clone. The labelling of cells provides information about their differentiation state.
- Numerous tracing techniques rely on cell labelling, including genetic fate mapping, generation of genetic mosaics, chromosomal loss, recombination, and replication-defective retroviral vectors.
- This methodology has the potential to be an effective tool for detecting new stem cells with single-cell resolution.
Stem Cell Banking
- A stem cell bank is a facility that maintains natural, unaltered stem cells that have not been exposed to chemicals or medications for future usage in goods or medical procedures.
- The cells are examined before, during, and after the cell banking procedure, as well as prior to their usage in treatment.
- It is the simplest approach to provide natural pluripotent stem cells to the same person, provided they were stored for personal use, or as a pooled source for allogenic purposes.
- Cord blood is one of the most abundant sources of hematopoietic stem cells (HSCs), is a vital supply, and is genetically unique to the newborn and his or her family.
- Cord blood banking is a simple, painless, and non-invasive procedure, and if the need ever arose, these stem cells might be employed to create potent medicines.
Importance
- Stem cell banking could aid in the treatment of a vast variety of life-threatening disorders for decades to come.
- It provides the chance to have access to a potent source of cells in the event of an emergency medical treatment without the risk of immunological rejection.
- It is difficult to identify a compatible donor at the moment of an emergency transplant, making stem cell banking a crucial choice. To far, cord blood has been utilised in over 12,000 transplants including children and adults.
- Stem cells have been identified as a valuable biological resource that can be safely kept for future use in treating diseases such as Alzheimer’s, diabetes, heart and liver disease, muscular dystrophy, Parkinson’s disease, and spinal cord injury.
- Public cord blood banks hold cord blood donations for prospective transplant patient usage.
- Family cord blood banks hold cord blood for the client’s benefit. It provides the family with peace of mind in the event that a kid or family member requires it for treatment.
- Babycell, a subsidiary of Regenerative Medical Services Pvt. Ltd., is India’s first bank for preserving samples of umbilical cord blood and an internationally approved laboratory.
- Developed by the WiCell Research Institute, the U.S. National Stem Cell Bank (NSCB) is a renowned stem cell bank that offers hESCs to eligible scientists for use in NIH-funded research projects that fulfil standard quality control requirements.
What is Stem Cell Therapy?
- Stem cell therapy, also known as regenerative medicine, is one of the stem cell applications that stimulate the restoration of damaged and dysfunctional tissues and their derivatives.
- Pluripotent stem cells are rarely employed therapeutically in humans since some of these cells may result in the creation of teratomas, which are uncommon solid tumours.
- However, it has been utilised to treat spinal injuries and visual impairment in animals.
- Since the 1960s, multipotent stem cells extracted from bone marrow have been employed to treat leukaemia, myeloma, and lymphoma.
- The ability of mesenchymal stem cells to create entire joints may also be advantageous for treating other illnesses.
- It is also preferable to employ multipotent stem cells instead of pluripotent cells since multipotent stem cells would prevent the immune system from rejecting transplants.
- Thus, stem cell therapy is a good avenue for the enhancement of treatment approaches and facilities for chronic diseases.
- However, much remains to be known about their biology, modification, and safety before they can reach their full therapeutic potential.
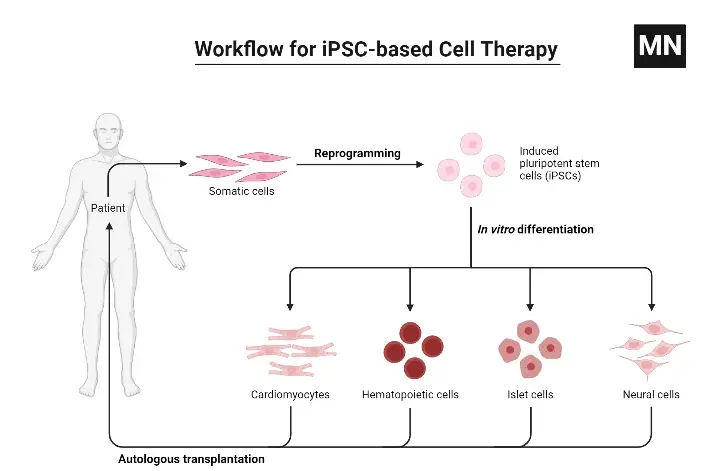
Applications of Stem Cells
The following are the most important uses of stem cells:
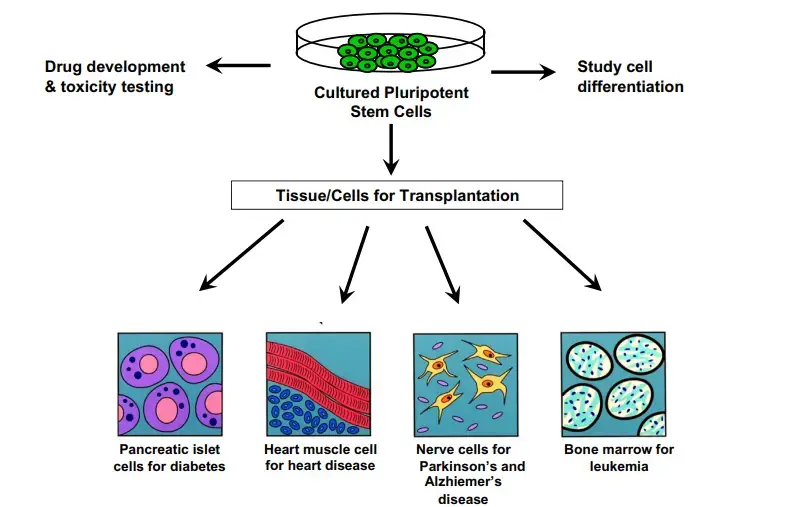
1. Tissue Regeneration
- This is the most significant use of stem cells. The stem cells can be utilised to generate a certain type of tissue or organ.
- This can be beneficial for organ transplants including the kidney and liver. The scientists have already employed stem cells from beneath the epidermis to create skin tissue that can be used for tissue transplantation to treat severe burns and other injuries.
2. Treatment of Cardiovascular Disease
- Using human stem cells, a team of researchers has produced blood arteries in mice.
- Within two weeks of implantation, the artificial blood vessels developed a network and were as effective as real blood vessels.
3. Treatment of Brain Diseases
- Stem cells can also be used to treat Parkinson’s and Alzheimer’s disorders. These can aid in the replacement of injured brain cells.
- Researchers have attempted to develop embryonic stem cells into these sorts of cells to enable the treatment of disorders.
4. Blood Disease Treatment
- Cancers, sickle cell anaemia, and other immunodeficiency disorders are treated with adult hematopoietic stem cells.
- These stem cells can be utilised to generate red and white blood cells throughout the body.
5. Damaged Tissue Replacement
- Human stem cells could potentially be employed to generate cells and tissues for cell-based therapeutics.
- This involves treating patients by transplanting specialised cells generated in the laboratory from stem cells.
- Stem cells could be utilised to treat a variety of illnesses, including as heart failure, spinal cord injuries, diabetes, and Parkinson’s disease, due to their capacity to replace damaged cells in the body.
- Scientists anticipate that transplanting and growing suitable stem cells in injured tissue will rebuild the tissue’s numerous cell types.
- For instance, hematopoietic stem cells might be put into leukaemia patients to make new blood cells, while neural stem cells could rebuild spinal cord injury-damaged nerve tissue.
6. Human Development Studies
- Stem cells could be utilised to investigate the earliest stages of human development in order to learn more about cell differentiation and function.
- This could aid researchers in determining why some cells become malignant and how certain hereditary illnesses arise.
- This understanding could lead to hints about how to avoid or treat certain disorders.
7. New Drug Testing
- Laboratory-grown stem cells may be beneficial for testing medicines and chemicals prior to their usage in human clinical trials.
- The cells could be programmed to develop into the essential cell types for testing the medication.
- These cells may be more likely than animal models to mimic the reaction of human tissue to the medication being evaluated.
- This could make drug testing safer, less expensive, and more ethically acceptable to individuals who reject not only the use of animals in pharmaceutical testing but all animal experiments.
8. Screening Toxins
- Before compounds such as insecticides are utilised in the field, stem cells may be beneficial for screening for possible toxicities.
9. Testing Gene Therapy Methods
- Stem cells may prove valuable during the development of novel gene therapy techniques that could aid people with hereditary illnesses.
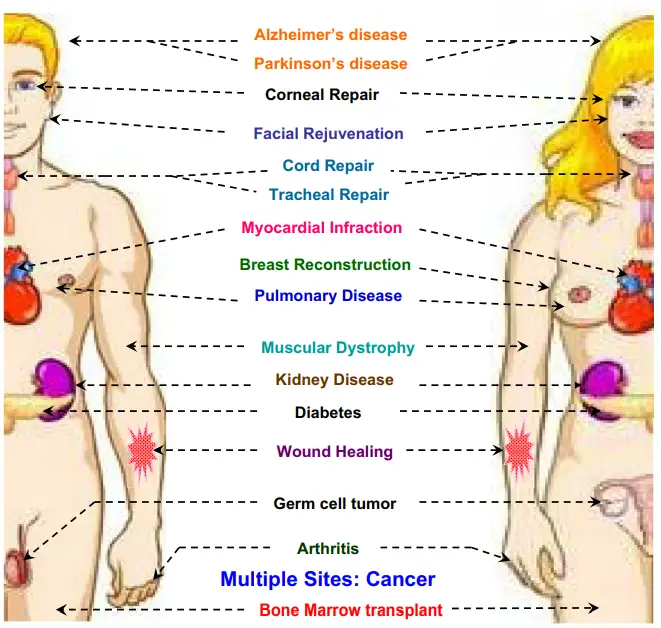
New Stem Cell Therapy Against HIV/AIDS
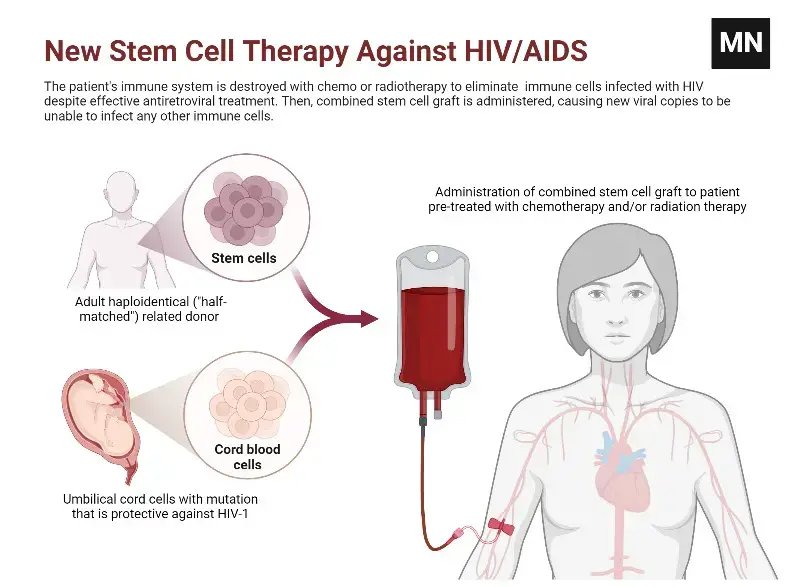
Autologous Haematopoietic Stem Cell Transplant
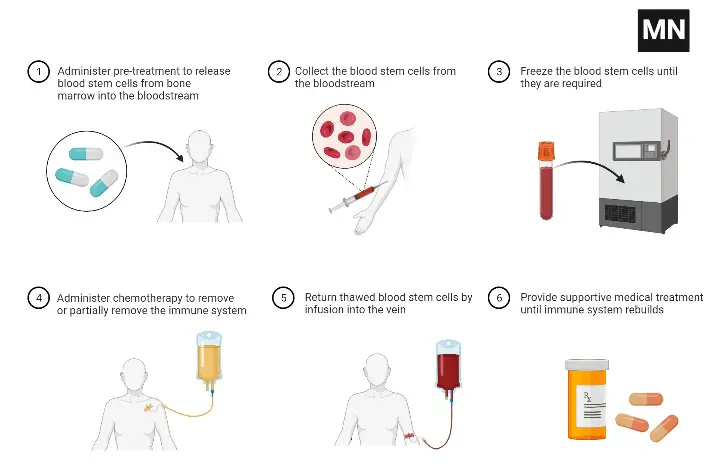
Stem the Therapy Advantages
- Stem cell therapies may alleviate the symptoms of the disease or disorder being treated. Patients may be able to lessen their medicine consumption for their disease or condition if their symptoms diminish.
- Stem cell therapy may also contribute to society’s understanding of stem cells and future treatments.
- The physicians’ creed is to cause no harm, and stem cells make this easier than ever. Surgical procedures are inherently hazardous. To achieve a successful outcome, tissue must be eliminated.
- Using stem cells, one can prevent the risks of surgical operations. In addition, disease is a risk, and if the procedure fails, another surgery may be necessary. Stem cells may potentially be used to remove anesthesia-related dangers.
- Additionally, stem cells have been extracted from the patient’s body and redeployed where desired. Since they originate from the patient’s own body, this treatment is referred to as autologous. Autologous treatments are believed to be the safest because there is almost certainly no chance of donor substance rejection.
Stem the Therapy Advantages
- Stem cell therapies may necessitate immunosuppression due to the need for pre-transplant radiation to eliminate the patient’s prior cells or because the patient’s immune system may attack the stem cells. Utilizing stem cells from the same individual being treated is one method for avoiding the second risk.
- The pluripotency of certain stem cells may also make it challenging to obtain a certain cell type. Additionally, it is challenging to collect the precise cell type required because not all cells in a population differentiate consistently. Undifferentiated cells can generate non-desired tissue types.
- Some transplanted stem cells develop cancers; pluripotency is associated with tumour formation, particularly in embryonic stem cells, foetal normal stem cells, and induced pluripotent stem cells. Fetal appropriate stem cells produce malignancies despite multipotency.
- The technique of utilising or investigating embryonic stem cells also raises ethical considerations. The blastocyst is destroyed when its cells are extracted. The question is whether the blastocyst should be regarded as a human life. This discussion is predominantly philosophical, rather than scientific.
Difference Between Embryonic and Adult Stem Cells
Characteristics | Embryonic Stem Cells (ESCs) | Adult Stem Cells (ASCs) | |
Potency | ES cells are pluripotent cells capable of differentiating into any type of cells of the body (e.g. embryos). | Adult stem cells are unipotent/multipotent and can differentiate into the type of cells/tissue from which they are derived (e.g. bone marrow, stromal cells, brain, baby teeth, etc.). | |
Culture | Large numbers of these cells can be easily cultured in vitro. | Rarely found, and difficult to grow in vitro. | |
Transplant | Transplant rejection occurs with ESCs | No transplant rejection occurs as it uses cells from the same patient’s body | |
Possibility of infection | Possibility of infection and teratoma formation. | No such possibilityImmune responseMay induce immune response. | Immune privileged. |
Ethical issues | Ethically controversial. | No ethical issues. |
FAQ
What are stem cells?
Stem cells are unique cells in the body that have the ability to differentiate into different types of specialized cells or divide to produce more stem cells. They play a crucial role in the development, growth, and repair of tissues and organs.
Where do stem cells come from?
Stem cells can be derived from various sources, including embryonic tissue, adult tissues (such as bone marrow and adipose tissue), and induced pluripotent stem cells (iPSCs) generated from reprogrammed adult cells.
What is the difference between embryonic stem cells and adult stem cells?
Embryonic stem cells are derived from the inner cell mass of a blastocyst and have the potential to differentiate into any cell type in the body. Adult stem cells, also known as somatic or tissue-specific stem cells, are found in specific tissues and can give rise to a limited range of specialized cell types.
What are the potential applications of stem cells?
Stem cells have significant potential for regenerative medicine, disease modeling, and drug discovery. They can be used to develop new therapies for various conditions, including degenerative diseases, injuries, and genetic disorders.
Are there any ethical concerns associated with stem cell research?
The use of embryonic stem cells raises ethical concerns for some individuals due to the destruction of embryos during the process of obtaining these cells. However, alternative sources of stem cells, such as adult stem cells and induced pluripotent stem cells, have alleviated some of these ethical concerns.
What are induced pluripotent stem cells (iPSCs)?
iPSCs are adult cells that have been reprogrammed to resemble embryonic stem cells. They are created by introducing specific genes or factors into the adult cells, which enables them to regain pluripotency and the ability to differentiate into various cell types.
Are stem cell therapies currently available?
Some stem cell therapies have been approved for specific medical conditions, such as bone marrow transplants for certain blood disorders. However, many potential stem cell therapies are still in the experimental stage and undergoing rigorous research and clinical trials.
What are the risks associated with stem cell therapies?
While stem cell therapies hold great promise, there are risks involved. These include the potential for the formation of tumors, inappropriate differentiation leading to adverse effects, and the rejection of transplanted cells by the immune system. Rigorous testing and safety measures are necessary to ensure the effectiveness and safety of stem cell-based treatments.
Can stem cells be used to treat neurological disorders?
Stem cells show promise in the field of neurology for conditions such as Parkinson’s disease, Alzheimer’s disease, and spinal cord injuries. Research is ongoing to understand how stem cells can be used to replace damaged neurons and restore neurological function.
How can I donate or bank my own stem cells?
There are various stem cell banking facilities available where you can store your own stem cells for potential future use. Additionally, you can also consider donating your stem cells to public stem cell banks to help others in need. It is important to research and consult with healthcare professionals to understand the options and processes involved in stem cell banking or donation.
References
- Fontes PA, Thomson AW. Stem cell technology. Interview by Abi Berger. BMJ. 1999 Nov 13;319(7220):1308. doi: 10.1136/bmj.319.7220.1308. PMID: 10559059; PMCID: PMC1129084.
- Das, B. C., & Tyagi, A. (2014). Stem Cells. Animal Biotechnology, 425–450. doi:10.1016/b978-0-12-416002-6.00023-7
- Cole, C. (2006). STEM CELLS. Encyclopedia of Respiratory Medicine, 120–124. doi:10.1016/b0-12-370879-6/00373-2
- Łos, M. J., Skubis, A., & Ghavami, S. (2019). Stem Cells. Stem Cells and Biomaterials for Regenerative Medicine, 5–16. doi:10.1016/b978-0-12-812258-7.00002-2
- Lin, S. C., & Talbot, P. (2014). Stem Cells. Encyclopedia of Toxicology, 390–394. doi:10.1016/b978-0-12-386454-3.00088-9
- Hammond-Browning, N. (2012). Stem Cells. Encyclopedia of Applied Ethics, 227–234. doi:10.1016/b978-0-12-373932-2.00426-9
- Loya, K. (2014). Stem Cells. Handbook of Pharmacogenomics and Stratified Medicine, 207–231. doi:10.1016/b978-0-12-386882-4.00011-6
- Neman, J., Persson, A., Ho, A., Levy, M. L., Snyder, E. Y., & Jandial, R. (2010). Stem Cells. Encyclopedia of Movement Disorders, 159–166. doi:10.1016/b978-0-12-374105-9.00544-x
- https://www.closerlookatstemcells.org/learn-about-stem-cells/types-of-stem-cells/
- https://www.urmc.rochester.edu/encyclopedia/content.aspx?contenttypeid=160&contentid=38
- https://www.thermofisher.com/in/en/home/life-science/stem-cell-research.html
- https://www.mayoclinic.org/tests-procedures/bone-marrow-transplant/in-depth/stem-cells/art-20048117#:~:text=What%20are%20stem%20cells%3F,more%20cells%20called%20daughter%20cells.
- https://www.britannica.com/science/stem-cell
- https://stemcells.nih.gov/info/basics/stc-basics
- https://microbenotes.com/stem-cells/
- https://www.cell.com/cell-stem-cell/home