What is Receptor?
- Receptors are specialized protein structures that play a pivotal role in cellular signal transduction. These proteins are designed to specifically bind to certain molecules, termed ligands.
- Ligands encompass a broad range of molecules, from simple inorganic entities to complex proteins, hormones, and neurotransmitters produced by organisms. The interaction between a receptor and its ligand culminates in a conformational alteration in the receptor, subtly modifying its function.
- Upon ligand binding, several outcomes are possible. The receptor might transform into an enzyme, facilitating the synthesis or breakdown of specific molecules.
- Alternatively, this interaction can trigger a cascade of changes in associated proteins, ultimately conveying a message to the cell. Such messages can regulate metabolic processes or act as sensory signals.
- The binding affinity, which refers to the receptor’s ability to retain the ligand, determines the duration of this interaction. Over time, the receptor releases the ligand, reverts to its original conformation, and the signaling ceases.
- Interestingly, other molecules can also bind to the ligand-binding site of receptors. If these molecules emulate the effects of the natural ligand, they are termed agonists. Many therapeutic and illicit drugs act as synthetic agonists, mimicking molecules like endorphins that induce feelings of well-being.
- However, these synthetic agonists often exhibit a higher binding affinity than natural ligands, leading to prolonged receptor occupation and necessitating increased dosages over time due to tolerance.
- Conversely, antagonist molecules can obstruct the ligand-binding site without inducing a conformational change in the receptor, effectively blocking the signal. Some antagonists are employed in treatments for substance dependencies, rendering drug consumption non-rewarding.
- Others, like certain proteins in snake venom, can inhibit vital physiological processes, resulting in severe consequences like internal bleeding.
- From a broader perspective, receptors are integral components in biochemistry and pharmacology, acting as conduits for chemical signals to be integrated into biological systems. They can be categorized based on their location: cell surface receptors (transmembrane receptors) and intracellular receptors.
- The former group encompasses ligand-gated ion channels, G protein-coupled receptors, and enzyme-linked hormone receptors, while the latter includes cytoplasmic and nuclear receptors.
- Ligands for these receptors can be diverse, ranging from neurotransmitters and hormones to pharmaceuticals and toxins. Each receptor type is intricately linked to specific cellular biochemical pathways, ensuring precise and targeted responses to external stimuli.
Definition of Receptor
A receptor is a specialized protein molecule that selectively recognizes and binds to specific ligands, leading to cellular responses or signal transduction within the cell.
Types of Receptors
Receptors are specialized protein structures integral to cellular communication and response mechanisms. They are primarily categorized based on their cellular location into:
- Internal Receptors: These receptors are located within the cell and are responsible for responding to signals that have penetrated the cell membrane. Key types of internal receptors include:
- Growth Factor Receptors: These are pivotal in modulating cell growth, differentiation, and development. Notable examples encompass:
- Neurotrophin receptor
- FGF receptor (Fibroblast Growth Factor Receptor)
- EGF receptor (Epidermal Growth Factor Receptor)
- IGF receptor (Insulin-like Growth Factor receptor)
- PDGF receptor (Platelet-Derived Growth Factor Receptor)
- VEGF receptor (Vascular Endothelial Growth Factor Receptor)
- Cytokine Receptors: Essential for cellular communication, these receptors facilitate cells to interact with their extracellular milieu. They are instrumental in gene expression, mediator release, and alterations in the cell cycle.
- Growth Factor Receptors: These are pivotal in modulating cell growth, differentiation, and development. Notable examples encompass:
- Cell-surface Receptors: Positioned on the cell membrane, these receptors detect external signals and transmit them into the cell. Prominent cell-surface receptors include:
- B Cell Receptors: B cells, or B lymphocytes, originate in the bone marrow and circulate in the blood and lymphatic system. Their primary role is the production of antibodies against foreign entities or pathogens, thereby playing a crucial part in adaptive immunity and initiating allergic reactions.
- T Cell Receptors: T cells, a subset of lymphocytes, mature in the thymus gland. Their primary function is to combat pathogens and ensure their removal from the body.
- Granulocyte Receptors: These immune cells contain granules and release enzymes during infections, allergies, and asthma episodes. The granulocyte category encompasses three white blood cell types: Basophils, Eosinophils, and Neutrophils.
In essence, receptors are the molecular gatekeepers of cells, facilitating precise responses to diverse external and internal signals, ensuring the cell’s proper function and survival.
Structure of Receptors
Receptors are intricate protein structures that play a pivotal role in cellular communication. Their structural diversity can be categorized into several primary types, each with distinct characteristics:
- Ligand-gated Ion Channels (Ionotropic Receptors): These receptors respond to fast neurotransmitters like acetylcholine (nicotinic) and GABA. Upon activation, they modulate ion flux across membranes. Structurally, they are heteromeric, with each subunit comprising an extracellular ligand-binding domain and a transmembrane domain containing four alpha helices. The interface between the subunits houses the ligand-binding cavities.
- G Protein-coupled Receptors (Metabotropic Receptors): Representing the most extensive receptor family, these receptors interact with hormones and slow transmitters such as dopamine and metabotropic glutamate. Their structure encompasses seven transmembrane alpha helices connected by loops that form both extracellular and intracellular domains. Ligand binding sites vary based on the ligand’s size and nature. These receptors are linked to diverse intracellular effector systems through G proteins, which are heterotrimers consisting of α (alpha), β (beta), and γ (gamma) subunits. G protein activation induces structural changes, facilitating GDP-GTP exchange and subsequent subunit dissociation.
- Kinase-linked and Related Receptors: These receptors possess an extracellular domain for ligand binding and an intracellular domain, often with enzymatic properties, connected by a single transmembrane alpha helix. An exemplar of this category is the insulin receptor.
- Nuclear Receptors: Contrary to their name, these receptors are initially located in the cytoplasm and migrate to the nucleus post-ligand binding. Their structure comprises a C-terminal ligand-binding region, a core DNA-binding domain (DBD), and an N-terminal domain housing the AF1 (activation function 1) region. The core region’s dual zinc fingers enable DNA sequence recognition specific to the receptor. The N-terminal domain can interact with other transcription factors, modulating receptor activity. Steroid and thyroid-hormone receptors are classic representatives of this category.
To study these receptors, they can be isolated from cell membranes using intricate extraction techniques involving solvents, detergents, and affinity purification. Biophysical methodologies, including X-ray crystallography, NMR, circular dichroism, and dual polarisation interferometry, offer insights into receptor structures and functions. Additionally, computer simulations provide a dynamic perspective on receptor mechanisms.
Binding and activation
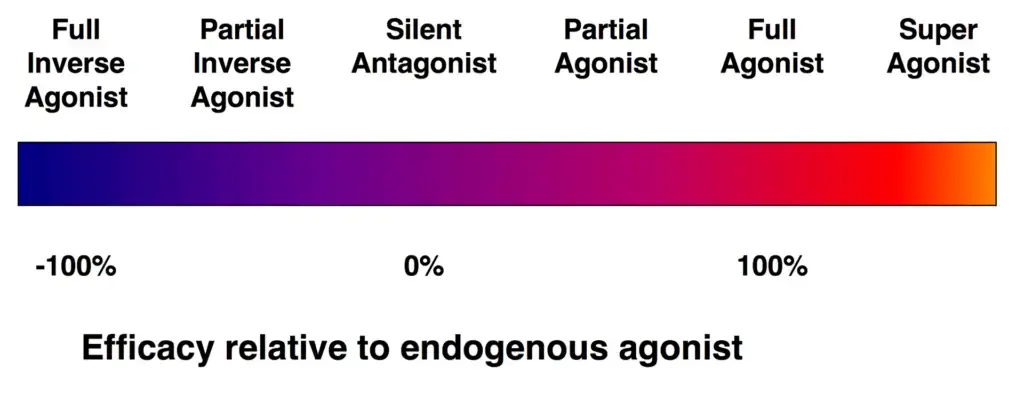
Receptors play a pivotal role in cellular signaling, and their function is intricately tied to the binding of specific molecules, known as ligands. The interaction between ligands and receptors is governed by the principles of equilibrium, and can be represented by the equation:
L+R⇌LR
Here, L represents the ligand, R denotes the receptor, and LR signifies the ligand-receptor complex.
The affinity of a ligand for its receptor is a crucial parameter, inversely proportional to the dissociation constant (Kd). A high affinity indicates a low Kd value, signifying a strong ligand-receptor interaction. However, binding alone does not guarantee a biological response; a significant number of receptors must be activated to elicit a response.
Affinity and Efficacy: Affinity measures the propensity of a ligand to bind to its receptor, while efficacy gauges the ability of the bound ligand to activate the receptor. Ligands can be categorized based on their effects:
- Agonists: These are molecules that can activate receptors, leading to a robust biological response. The endogenous ligand with the highest efficacy for a receptor is termed a full agonist.
- Partial Agonists: These bind and activate receptors but not to their maximum efficacy.
- Antagonists: These molecules bind to receptors without activating them, thereby blocking the binding and action of agonists. Antagonists can be competitive, vying with the agonist for the receptor, or irreversible, forming strong bonds that completely block the receptor.
- Inverse Agonists: These reduce receptor activity by inhibiting its inherent activity.
- Allosteric Modulators: These molecules bind to sites other than the primary ligand-binding site, modulating the receptor’s response to an agonist.
It’s imperative to understand that the terms agonism and antagonism pertain solely to ligand-receptor interactions and not their broader biological effects.
Constitutive Activity: Some receptors exhibit constitutive activity, meaning they can produce a biological response even in the absence of a bound ligand. Inverse agonists can block this activity. For instance, the GABAA receptor, which displays constitutive activity, can be inhibited by beta carboline, an inverse agonist. Mutations leading to heightened constitutive activity can result in diseases, such as precocious puberty or hyperthyroidism, due to altered hormone receptor functions.
Theories of drug-receptor interaction
- Occupation Theory: Stemming from early pharmacological concepts, this theory posits that the pharmacological effect of a drug is directly proportional to the number of receptors it occupies. Once the drug dissociates from the receptor, its effect terminates. Two pivotal terms introduced by Ariëns & Stephenson to describe ligand actions are:
- Affinity: Represents the propensity of a drug to bind with a receptor, forming a drug-receptor complex.
- Efficacy: Denotes the capability of the drug to elicit a response subsequent to the formation of the drug-receptor complex.
- Rate Theory: Offering a different perspective from the Occupation Theory, the Rate Theory suggests that receptor activation is contingent upon the cumulative encounters between a drug and its receptors within a specific timeframe. The pharmacological effect is tied to the rates of drug association and dissociation, rather than mere receptor occupancy. Drugs can be categorized based on these rates:
- Agonist: Exhibits rapid association and dissociation.
- Partial-agonist: Characterized by moderate rates of association and dissociation.
- Antagonist: Binds quickly to receptors but dissociates slowly.
- Induced-fit Theory: This theory postulates that when a drug approaches its receptor, the receptor undergoes a conformational shift, optimizing its binding site to accommodate the drug, leading to the formation of a drug-receptor complex.
- Spare Receptors Concept: Certain receptor systems, exemplified by the acetylcholine system in smooth muscle, have the unique ability to produce a maximal response even when a minuscule fraction of receptors (<1%) are occupied by agonists. Such systems are believed to possess a reservoir of spare receptors. This configuration ensures an efficient mechanism for neurotransmitter synthesis and release.
In essence, these theories provide a comprehensive framework to comprehend the nuanced dynamics governing drug-receptor interactions, laying the foundation for advancements in therapeutic strategies.
Regulation of Receptor
In the intricate cellular environment, the regulation of receptors plays a pivotal role in ensuring appropriate cellular responses to external stimuli. This regulation is multifaceted and encompasses several mechanisms:
- Receptor Density Modulation: Cells possess the inherent ability to adjust the density of specific receptors on their surface. This is achieved through two primary processes:
- Upregulation: An increase in the number of receptors, enhancing the cell’s sensitivity to a particular hormone or neurotransmitter.
- Downregulation: A decrease in receptor numbers, diminishing the cell’s responsiveness to specific molecules. This modulation acts as a local feedback mechanism, allowing cells to fine-tune their responsiveness based on the prevailing extracellular environment.
- Conformational Alterations: Some receptors undergo structural changes that prevent the binding of agonists, even if the agonist is present. This phenomenon is particularly observed in ion channel receptors, where a change in receptor conformation ensures that the agonist binding does not activate the receptor.
- Receptor-Effector Uncoupling: In certain scenarios, the effector molecules associated with receptors become uncoupled. This is predominantly seen in G protein-coupled receptors, where the receptor might still bind to its ligand, but the subsequent intracellular signaling cascade is interrupted due to this uncoupling.
- Receptor Sequestration: Another regulatory mechanism is receptor sequestration or internalization. In this process, receptors are internalized into the cell, effectively removing them from the cell surface and rendering them unavailable for ligand binding. This is exemplified in the context of hormone receptors, where internalization ensures a controlled response to hormonal signals.
In summary, the regulation of receptor dynamics is a complex interplay of various mechanisms, ensuring that cells maintain a balanced and appropriate response to external cues. This regulation is crucial for maintaining cellular homeostasis and ensuring precise cellular signaling.
Examples of ligands
Receptors, integral to cellular signaling, interact with a myriad of ligands, each exhibiting unique characteristics and functions. The vastness of this interaction landscape is evident in the extensive family of G Protein-Coupled Receptors (GPCRs), which alone boasts over 810 distinct members. Ligand-Gated Ion Channels (LGICs) further diversify this landscape, interacting with a multitude of endogenous ligands.
1. Ion Channels and G Protein-Coupled Receptors:
- Glutamate: Interacts with ionotropic receptors such as NMDA, AMPA, and Kainate receptors, facilitating the flow of ions like Na+, K+, and Ca2+. Exogenously, compounds like Ketamine can modulate these interactions. On the metabotropic front, Glutamate receptors (mGluRs) are influenced by G proteins like Gq or Gi/o.
- GABA: GABAA receptors, including the GABAA-rho variant, predominantly allow the flow of Cl− ions. Benzodiazepines are exogenous ligands for these receptors. Additionally, the GABAB receptor, a GPCR, operates via the Gi/o G protein and can be modulated by compounds like Baclofen.
- Acetylcholine: The nicotinic acetylcholine receptor (nAChR) facilitates the passage of Na+, K+, and Ca2+ ions. Nicotine is a known exogenous ligand. Muscarinic acetylcholine receptors (mAChR), on the other hand, function through Gq or Gi proteins and can be influenced by Muscarine.
- Serotonin: The 5-HT3 receptor is an ion channel that allows Na+ and K+ ions to pass. Various GPCRs, such as 5-HT1-2 or 4-7, operate through G proteins like Gs, Gi/o, or Gq.
- ATP: P2X receptors are ion channels that facilitate the movement of Ca2+, Na+, and Mg2+ ions. P2Y receptors, GPCRs in nature, function via Gs, Gi/o, or Gq G proteins.
- Dopamine: Dopamine receptors, which are GPCRs, operate through Gs or Gi/o G proteins.
2. Enzyme-Linked Receptors: Enzyme-linked receptors encompass a broad category, including Receptor Tyrosine Kinases (RTKs). Notable examples include:
- EGFR: Binds with the endogenous ligand EGF. Gefitinib is an exogenous ligand.
- Insulin Receptor: Interacts with the hormone Insulin. Chaetochromin is an exogenous modulator.
- VEGFR: Binds with VEGF. Lenvatinib is an exogenous ligand.
3. Intracellular Receptors: These receptors function within the cell and include:
- cyclic nucleotide-gated ion channels: Interact with cGMP (in vision) and cAMP or cGTP (in olfaction), facilitating the flow of Na+ and K+ ions.
- IP3 receptor: Binds with IP3, allowing Ca2+ ions to pass.
- Ryanodine receptor: Interacts with Ca2+, facilitating its movement.
In essence, the realm of receptor-ligand interactions is vast and intricate, with each ligand-receptor pair playing a pivotal role in cellular signaling and function.
Advantages of Receptor
Receptors are specialized protein molecules that play a central role in cellular communication and response to external and internal stimuli. Their presence and function confer several advantages to organisms:
- Specificity: Receptors are highly specific for their ligands, ensuring that cells respond only to relevant signals. This specificity minimizes erroneous responses and ensures precise cellular actions.
- Amplification: Binding of a ligand to its receptor can trigger a cascade of intracellular events, amplifying the initial signal. This means that even a small number of ligand-receptor interactions can result in a significant cellular response.
- Modulation: Receptors allow for fine-tuning of responses. Depending on the cellular context, the same ligand-receptor interaction can lead to different outcomes, providing flexibility in cellular reactions.
- Rapid Response: Many receptor-mediated actions, especially those involving ion channels or second messengers, occur rapidly, allowing cells to quickly adapt to changes in their environment.
- Integration of Signals: Some receptors can integrate multiple signals, allowing cells to make coordinated decisions based on various inputs. This is especially important in complex physiological processes like immune responses or neural processing.
- Feedback Mechanisms: Receptors enable feedback loops, where the outcome of a receptor-mediated process can influence the activity of the receptor itself. This can lead to upregulation or downregulation of receptor numbers or activity, allowing cells to adapt to chronic or changing conditions.
- Therapeutic Targets: The specificity and central role of receptors in physiological processes make them ideal targets for therapeutic interventions. Many drugs are designed to modulate receptor activity, providing a means to treat various diseases.
- Diversity and Evolutionary Adaptation: The vast diversity of receptors allows organisms to detect and respond to a wide range of stimuli. Over evolutionary time, the emergence of new receptors or modifications to existing ones can provide adaptive advantages.
- Protection: Receptors on immune cells can recognize foreign or harmful entities, triggering defensive responses. This recognition is crucial for the immune system to differentiate between self and non-self, protecting the organism from infections.
- Sensory Perception: Receptors in sensory organs allow organisms to interact with their environment, perceive changes, and make appropriate behavioral decisions. This is vital for survival, reproduction, and overall well-being.
In essence, receptors provide organisms with the ability to perceive, interpret, and respond to their environment in a controlled and coordinated manner. Their advantages lie in their specificity, adaptability, and central role in mediating a wide range of physiological processes.
Disadvantages of Receptor
While receptors play crucial roles in cellular communication and response, they also have inherent limitations and can be associated with certain disadvantages:
- Over-sensitivity: Hyperactivity or overexpression of certain receptors can lead to excessive cellular responses. For instance, overactive receptors can contribute to allergic reactions or autoimmune disorders.
- Target for Pathogens: Many pathogens, including viruses and bacteria, exploit specific receptors to gain entry into cells. For example, the HIV virus binds to the CD4 receptor on certain immune cells to infect them.
- Desensitization: Continuous exposure to a ligand can lead to receptor desensitization, where the receptor becomes less responsive over time. This can be problematic in situations like chronic drug use, where higher doses are needed to achieve the same effect.
- Cross-reactivity: Some receptors might bind to unintended ligands, leading to undesired cellular responses. This can be seen in cases of drug side effects or allergic reactions to substances with similar structures.
- Mutations: Genetic mutations in receptor genes can alter receptor function, leading to diseases or disorders. For instance, mutations in hormone receptors can result in endocrine disorders.
- Complex Regulation: The intricate regulation of receptors, involving various cofactors and secondary messengers, can sometimes lead to unpredictable or non-linear responses.
- Dependency: Cells can become overly reliant on certain receptor-mediated pathways, making them vulnerable if that pathway is disrupted.
- Therapeutic Challenges: While receptors are targets for many drugs, the same receptor can be expressed in multiple tissues. This can lead to unintended effects in non-target tissues, complicating therapeutic interventions.
- Downregulation: Prolonged exposure to high levels of a ligand can lead to a decrease in the number of available receptors, a process called downregulation. This can reduce the cell’s sensitivity to that ligand.
- Potential for Overcompensation: In feedback mechanisms, the blocking or enhancing of one receptor-mediated pathway can lead to the overcompensation of another pathway, potentially causing imbalances in cellular functions.
In summary, while receptors are fundamental to many physiological processes, their inherent characteristics and the complexities of their regulation can also lead to vulnerabilities, challenges, and potential disadvantages in certain contexts.
Importance of Receptor
Receptors play a pivotal role in the physiology and biochemistry of organisms, acting as the primary mediators of cellular responses to external and internal stimuli. Their significance spans a wide range of biological processes:
- Signal Transduction: Receptors are integral to the process of signal transduction, where they receive signals, often in the form of molecules or ions, and convert them into cellular responses. This allows cells to communicate and coordinate actions.
- Mediation of Physiological Responses: Hormones, neurotransmitters, and other signaling molecules exert their effects by binding to specific receptors. For instance, insulin binds to its receptor to regulate glucose uptake, and neurotransmitters like serotonin or dopamine bind to their respective receptors in the brain to modulate mood and behavior.
- Pharmacological Targets: Many drugs exert their therapeutic effects by interacting with receptors. By either activating (agonists) or inhibiting (antagonists) these receptors, drugs can modulate physiological processes. This principle forms the basis for many therapeutic interventions in medicine.
- Regulation of Gene Expression: Some receptors, especially intracellular receptors like steroid hormone receptors, directly influence gene expression. Upon ligand binding, these receptors translocate to the nucleus and interact with DNA, regulating the transcription of specific genes.
- Adaptive Mechanisms: Receptors can be upregulated or downregulated based on cellular needs. For instance, in conditions of chronic exposure to a particular ligand, cells might decrease the number of receptors (downregulation) to reduce sensitivity. Conversely, in the absence of a ligand, cells might increase receptor numbers (upregulation) to enhance sensitivity.
- Immune Responses: Receptors on immune cells recognize pathogens or foreign substances, triggering immune responses. For example, T-cell receptors (TCRs) on T lymphocytes recognize antigens, leading to immune activation against pathogens.
- Sensory Perception: Receptors in sensory organs detect external stimuli, allowing organisms to perceive their environment. Photoreceptors in the eyes detect light, while olfactory receptors in the nose recognize different odors.
- Endocytosis and Transport: Some receptors mediate the transport of large molecules into cells through a process called receptor-mediated endocytosis. For instance, low-density lipoprotein (LDL) receptors facilitate the uptake of cholesterol into cells.
- Cell Adhesion: Certain receptors, like integrins, play roles in cell adhesion, helping cells attach to the extracellular matrix or other cells. This is crucial for tissue integrity and cellular communication.
- Development and Differentiation: Receptors play a role in embryonic development, guiding cell differentiation and tissue formation. For instance, growth factor receptors regulate cell proliferation and differentiation during development.
In summary, receptors are fundamental to the intricate network of cellular communication and response mechanisms in biological systems. Their multifaceted roles underscore their importance in maintaining homeostasis, mediating physiological processes, and serving as therapeutic targets in medicine.
Examples of a Receptor
Receptors play a pivotal role in various physiological processes, serving as the primary interface for cellular responses to external stimuli. Here are two illustrative examples of receptors and their intricate functions:
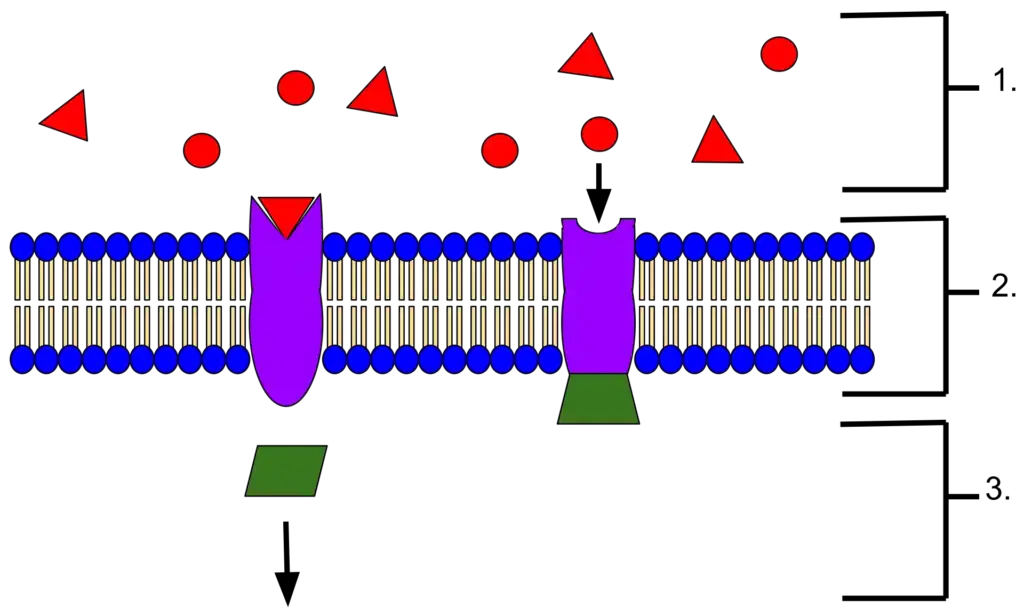
- Insulin Receptor:
- Function: The insulin receptor is crucial for regulating blood glucose levels. Glucose, the primary cellular fuel, requires a transporter molecule, Glut4, to enter cells.
- Mechanism: When blood glucose levels rise, receptors in the pancreas detect this surge and initiate insulin production and release. Almost every cell possesses insulin receptor proteins. Upon interaction with insulin, these receptors undergo a conformational change, triggering a cascade of reactions involving various proteins. These proteins produce a messenger molecule that influences the movement of Glut4 towards the cell membrane. In the presence of insulin, Glut4-containing vesicles swiftly move to the membrane, facilitating glucose uptake into the cell. The cessation of insulin presence halts this process, regulating glucose uptake. This intricate system involves not only the insulin receptor but also several other receptors participating in associated reactions.
- Taste Receptors:
- Function: Taste receptors are specialized nerve structures that enable the sensation of taste, such as sweetness or saltiness.
- Mechanism: Protrusions of taste nerves are embedded within the mouth’s mucous membrane. Consumed molecules like sugar or salt dissolve in saliva and interact with specific cells containing receptors tailored to these ligands. These receptors are gated ion channels, akin to those in nerve cells. Ligand binding prompts these channels to permit ion passage, leading to membrane depolarization. Sufficient ligand presence activates numerous receptors simultaneously, inducing an action potential. This electrical signal travels along the nerve until it reaches synaptic terminals, where it triggers the release of neurotransmitters. These neurotransmitters then bind to receptors on adjacent nerves, perpetuating the signal. This chain of events culminates in the brain’s processing centers, where the sensation, such as “sweetness,” is perceived. Remarkably, this intricate process unfolds in mere fractions of a second.
- Adrenergic Receptors:
- Function: Adrenergic receptors are integral in the cardiovascular system, mediating responses to catecholamines like adrenaline and noradrenaline. They play a pivotal role in regulating heart rate, blood pressure, and overall cardiovascular function.
- Mechanism: There are two primary types of adrenergic receptors: alpha (α) and beta (β). Each type has subtypes, such as α1, α2, β1, and β2. When adrenaline or noradrenaline binds to these receptors, various cellular responses are triggered. For instance, β1 receptor activation in the heart increases heart rate and contractility, while β2 receptor activation in the lungs leads to bronchodilation. Conversely, α1 receptor activation causes vasoconstriction, increasing blood pressure. These receptors are also targets for various medications, such as beta-blockers, which are used to treat hypertension and other cardiovascular conditions.
- Opioid Receptors:
- Function: Opioid receptors are primarily found in the central nervous system and are responsible for mediating pain relief, euphoria, and respiratory depression. They are the primary targets of opioid analgesics like morphine and fentanyl.
- Mechanism: There are three main types of opioid receptors: mu (μ), delta (δ), and kappa (κ). When an opioid binds to these receptors, it inhibits the release of neurotransmitters, thereby reducing the perception of pain. The mu receptor, in particular, is associated with analgesia, euphoria, and respiratory depression. Activation of the kappa receptor can lead to analgesia and sedation, while delta receptor activation has antidepressant effects. However, prolonged activation, especially of the mu receptor, can lead to tolerance, dependence, and respiratory depression, which can be fatal. This highlights the need for careful opioid administration and monitoring.
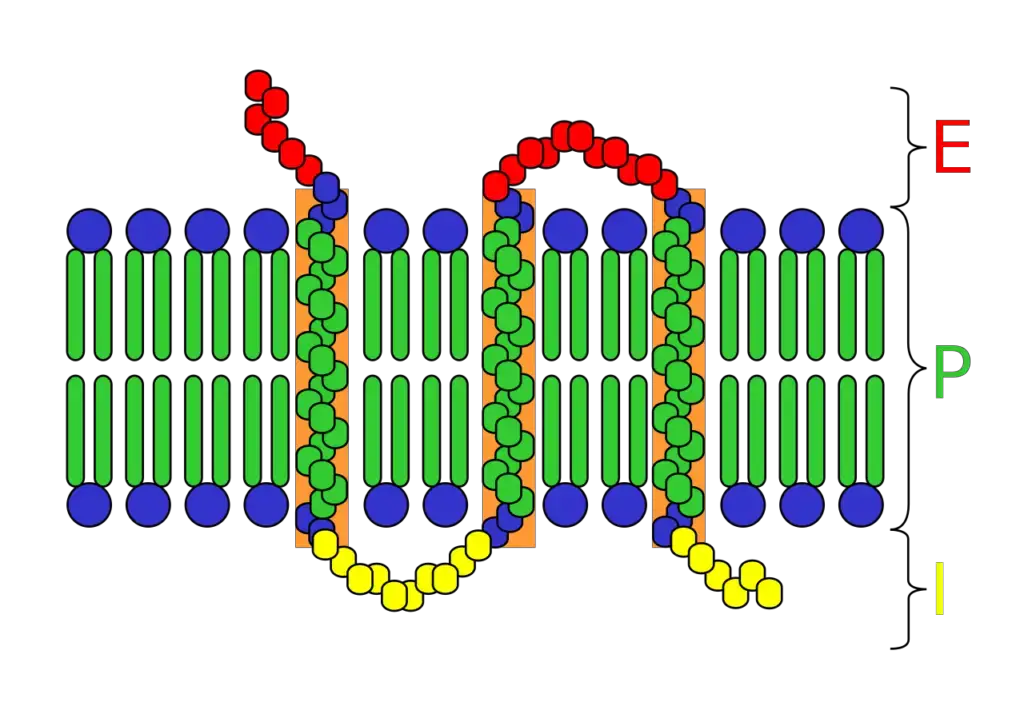
In summary, receptors are integral to various physiological responses, from metabolic regulation to sensory perception. Their complex mechanisms underscore the precision and efficiency of cellular communication pathways.
Practice Quiz
Which of the following best describes a receptor?
a) A type of enzyme
b) A protein that binds to a specific ligand
c) A type of neurotransmitter
d) A type of hormone
Which receptor type is primarily responsible for fast synaptic transmission in the nervous system?
a) G-protein coupled receptors
b) Enzyme-linked receptors
c) Ligand-gated ion channels
d) Intracellular receptors
Which of the following is NOT a function of receptors?
a) Facilitate cell-to-cell communication
b) Initiate cellular responses
c) Synthesize new ligands
d) Transduce external signals into cellular actions
Which receptors alter cell function by activating intracellular signal transduction pathways?
a) Intracellular receptors
b) Ligand-gated ion channels
c) G-protein coupled receptors
d) Enzyme-linked receptors
Which type of receptor is primarily found inside the cell and binds to lipid-soluble ligands?
a) G-protein coupled receptors
b) Enzyme-linked receptors
c) Ligand-gated ion channels
d) Intracellular receptors
Which of the following ligands binds to the GABAA receptor?
a) Glutamate
b) Dopamine
c) GABA
d) Serotonin
Which receptor type has intrinsic enzymatic activity?
a) Ligand-gated ion channels
b) G-protein coupled receptors
c) Enzyme-linked receptors
d) Intracellular receptors
Which process describes the reduction in the number of receptors on the cell surface after prolonged exposure to high levels of a ligand?
a) Desensitization
b) Downregulation
c) Cross-reactivity
d) Overcompensation
Which of the following is a characteristic of spare receptors?
a) They are always active
b) They are only found in the brain
c) They allow maximal cellular response even with low receptor occupancy
d) They are synthetic receptors
Which receptor type is targeted by the drug Ketamine?
a) Dopamine receptors
b) GABAA receptors
c) NMDA receptors
d) Serotonin receptors
FAQ
What is a receptor?
A receptor is a protein molecule that receives and responds to specific signals or stimuli, often in the form of molecules called ligands.
How do receptors work?
Receptors work by binding to specific ligands, which triggers a series of cellular responses. This binding can activate or inhibit cellular functions, depending on the type of receptor and ligand.
What are the different types of receptors?
There are several types of receptors, including G-protein coupled receptors, enzyme-linked receptors, ligand-gated ion channels, and intracellular receptors.
Why are receptors important in pharmacology?
Receptors are the primary targets for many drugs. By binding to and modulating the activity of receptors, drugs can produce therapeutic effects or cause side effects.
What is receptor specificity?
Receptor specificity refers to the ability of a receptor to bind selectively to a particular ligand or a group of related ligands.
How do cells regulate the number of receptors on their surface?
Cells can upregulate (increase) or downregulate (decrease) the number of receptors in response to changes in the concentration of ligands or other factors.
What is the difference between an agonist and an antagonist?
An agonist is a molecule that activates a receptor, while an antagonist is a molecule that blocks or inhibits receptor activation.
What are spare receptors?
Spare receptors are excess receptors that are not needed to produce a maximal response. They allow cells to respond fully even if only a fraction of the receptors are occupied.
Can receptors be found inside cells?
Yes, some receptors, known as intracellular receptors, are located inside the cell, typically in the cytoplasm or nucleus. These receptors often bind to lipid-soluble ligands that can cross the cell membrane.
Why do some drugs lose their effectiveness over time?
One reason is receptor downregulation or desensitization, where prolonged exposure to a drug leads to a decrease in the number or sensitivity of its target receptors.