What is Meiosis?
- Meiosis is a specialized form of cell division in sexually reproducing eukaryotic organisms that leads to the production of gametes, such as sperm and egg cells. This process ensures the reduction of chromosome number by half, resulting in four non-identical haploid cells from a single diploid parent cell. The significance of meiosis lies in its ability to generate genetic diversity and maintain chromosome number consistency across generations.
- In the context of the cell cycle, meiosis comprises two sequential divisions: meiosis I and meiosis II. Unlike mitosis, where one division yields two genetically identical daughter cells, meiosis involves a series of intricate steps that ensure the reshuffling of genetic material and the halving of chromosome numbers.
- During meiosis I, homologous chromosomes, which are chromosomes with the same genes but possibly different alleles, pair up and exchange genetic material in a process known as recombination or crossing over. This event occurs during the prophase I stage and results in the formation of chiasmata, physical links between the paired chromosomes. The aligned chromosome pairs, now called tetrads, are then separated during anaphase I, ensuring that each daughter cell receives one chromosome from each homologous pair.
- Meiosis II resembles mitosis in its mechanisms but acts on the haploid cells produced from meiosis I. Here, the sister chromatids of each chromosome are separated, resulting in four genetically distinct haploid daughter cells. These cells, each containing a unique combination of genetic material, serve as the precursors for gametes.
- The importance of meiosis extends beyond the mere production of gametes. The genetic recombination introduced during prophase I introduces variability, a crucial factor for evolution and adaptation. Moreover, the reduction of chromosome number ensures that when gametes fuse during fertilization, the resulting zygote will have the correct diploid number of chromosomes, preserving the genetic integrity of the species.
- In cytogenetics, the study of chromosomes, meiosis offers a window into understanding genetic disorders and chromosomal abnormalities. Errors during meiosis, such as non-disjunction where chromosomes fail to separate correctly, can lead to conditions like Down syndrome, where an individual has an extra chromosome 21.
- In conclusion, meiosis is a fundamental biological process that ensures genetic diversity and the stability of chromosome numbers across generations. Its intricate steps, from recombination to the reductional division, underscore its significance in reproduction, evolution, and genetic health.
Definition of Meiosis
Meiosis is a specialized form of cell division in sexually reproducing organisms that results in four non-identical haploid cells (gametes) from a single diploid parent cell, ensuring genetic diversity and maintaining consistent chromosome numbers across generations.
Types of Meiosis
Meiosis occurs in the germ cells of organisms that reproduce sexually. Germ cells are localised in the gonads of plants and animals alike. Different species undergo meiosis at different times; hence, the process can be classed as terminal, intermediate, or initial.
1. Terminal meiosis
- This process, also known as gametic meiosis, occurs in animals and a few lower plants.
- In terminal meiosis, the meiotic division occurs just prior to gamete production or gametogenesis.
2. Intermediary or sporic meiosis
- It’s a trait shared only by plants with flowers. Between fertilisation and the production of gametes, this meiosis occurs.
- It also plays a role in microsporogenesis (in the anthers) and megasporogenesis (in the ovary or pistil), the processes by which microspores and megaspores are created.
3. Initial or zygotic meiosis
- It can be found in certain types of algae, fungus, and diatoms. Because only the egg undergoes meiosis after fertilisation, only the egg is diploid.
- Meiocytes: Meiocytes are the cells that undergo meiosis. In males, gonocytes are meiocytes that develop into spermatocytes, and in females, oocytes. The sporangium’s meiocytes are known as sporocytes (i.e., microsporocytes and megasporocytes).
Phases of Meiosis
Meiosis is a specialized form of cell division that gives rise to non-identical haploid cells, essential for sexual reproduction. This process is categorized into two sequential stages: meiosis I and meiosis II. Each of these stages is further subdivided into distinct phases that meticulously orchestrate the distribution of genetic material.
Meiosis I:
- Prophase I: This is the longest phase of meiosis. During prophase I, chromosomes condense and become visible. Homologous chromosomes (chromosomes with the same genes but possibly different alleles) pair up in a process called synapsis. This pairing leads to the formation of tetrads, which facilitate genetic recombination or crossing-over, where sections of chromatids may exchange places. This ensures genetic diversity in the offspring.
- Metaphase I: Tetrads align at the metaphase plate in the cell’s center. Spindle fibers from opposite poles of the cell attach to the centromere of each homologous chromosome.
- Anaphase I: The spindle fibers contract, pulling apart the homologous chromosomes toward opposite poles. Unlike mitosis, the sister chromatids remain attached.
- Telophase I: The separated chromosomes reach the poles, and the nuclear membrane reforms around them. The cell then undergoes cytokinesis, resulting in two haploid daughter cells.
Meiosis II:
- Prophase II: Chromosomes, each still composed of two chromatids, condense and become visible. Spindle fibers start to form in each haploid daughter cell from meiosis I.
- Metaphase II: Chromosomes align at the metaphase plate in each of the haploid cells.
- Anaphase II: The sister chromatids of each chromosome are finally pulled apart, driven by the contracting spindle fibers, and move toward opposite poles.
- Telophase II: Chromatids reach the poles, and the nuclear membrane forms around each set. Cytokinesis follows, resulting in four non-identical haploid daughter cells.
It’s crucial to note that the preparatory steps preceding meiosis resemble the interphase of mitosis, encompassing the G1 phase (cell growth), the S phase (DNA replication), and the G2 phase (preparation for cell division). However, in the context of meiosis, the S phase ensures that each chromosome consists of two sister chromatids, which are vital for the subsequent meiotic divisions. Some species also exhibit a resting phase, termed interkinesis, between meiosis I and II.
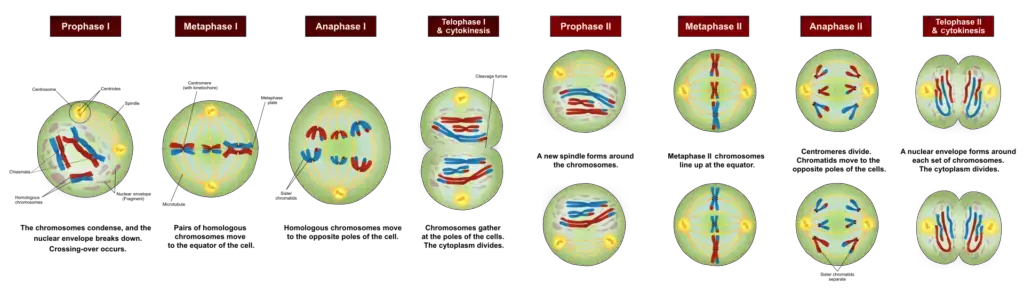
In summary, meiosis is a meticulously orchestrated process that ensures genetic diversity through the formation of unique haploid cells, which are foundational for sexual reproduction.
A. Phases of Meiosis I
- Meiosis I, the initial phase of meiosis, is a pivotal stage in cell division that ensures the reduction of chromosome number by half, transitioning from a diploid to a haploid state. This division is distinctively characterized by the intimate interaction and exchange of genetic material between homologous chromosomes.
- During the onset of Meiosis I, following the interphase where DNA replication occurs in the S phase, chromosomes undergo condensation. This is a preparatory step for the intricate processes that follow. In the early stages of prophase I, two centrosomes migrate to opposite poles of the cell, setting the stage for subsequent nuclear division.
- The hallmark of Meiosis I is the pairing of homologous chromosomes, each comprising two chromatids. These paired chromosomes, termed bivalents, align at the spindle equator during metaphase I. This alignment ensures the accurate segregation of chromosomes. As the cell progresses to anaphase, these homologous chromosomes are pulled apart. Notably, while the homologous chromosomes are separated, the sister chromatids of each chromosome remain conjoined.
- Meiosis I is often referred to as the “reductional division” because it reduces the chromosome number from diploid (2n) to haploid (n). This reduction is crucial for maintaining chromosome stability across generations. By the end of Meiosis I, two haploid cells are formed, each containing pairs of chromatids. These cells are primed for the subsequent phase, Meiosis II, where further segregation of chromatids occurs.
- In essence, Meiosis I is a meticulously orchestrated process that ensures genetic diversity and stability by facilitating the exchange of genetic material between homologous chromosomes and reducing the chromosome number, setting the stage for the production of haploid gametes.
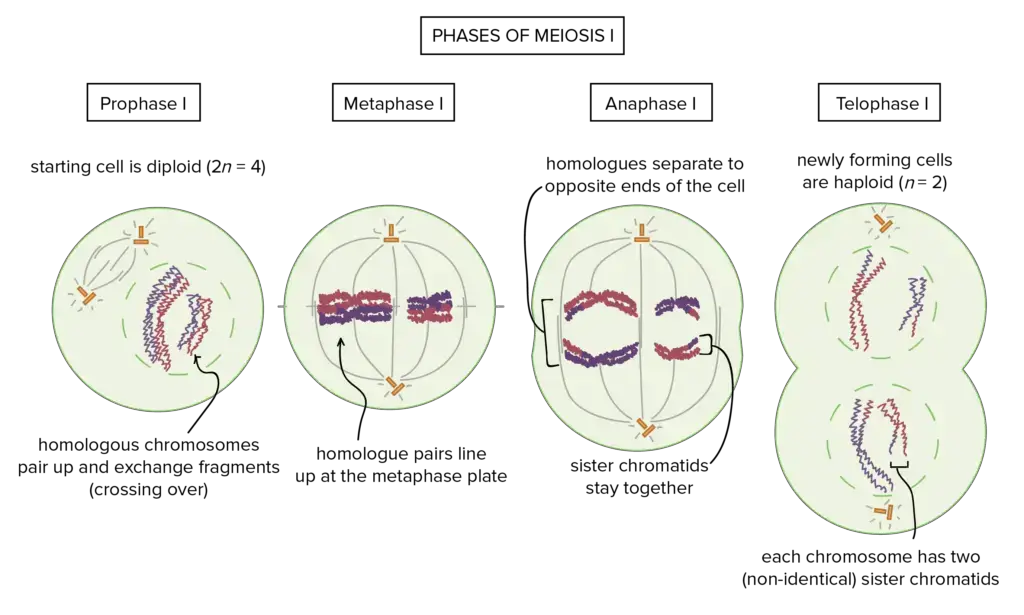
1. Interphase
- Interphase, a foundational phase preceding both mitosis and meiosis, is a period of cellular preparation and growth. During this phase, the cell undergoes several critical processes that set the stage for subsequent divisions.
- Within the confines of the interphase, the nuclear envelope remains intact, safeguarding the genetic material. The chromosomes, at this juncture, are not distinctly visible. Instead, they manifest as elongated, coiled chromatin fibers that are diffused throughout the nucleus. A significant event during interphase is the duplication of DNA, effectively doubling the genetic content of the cell. This amplification is crucial for the subsequent distribution of genetic material during cell division.
- Another notable feature of interphase is the enlargement of the nucleolus, attributed to the accumulation of ribosomal RNA (rRNA) and ribosomal proteins. This increase in size underscores the nucleolus’s role in ribosome synthesis, which is vital for protein production.
- In animal cells, centrioles play a pivotal role in cell division. During interphase, a new pair of centrioles begins to form adjacent to the pre-existing pair, resulting in two pairs of centrioles in the cell. These centrioles are instrumental in establishing the mitotic spindle during cell division.
- The G2 phase of interphase is particularly significant as it introduces a pivotal shift in the cell’s trajectory. It is during this phase that the cell is directed towards meiosis, diverging from the path of mitosis. As the first meiotic division commences, the nucleus of the cell undergoes a transformation. By absorbing water from the cytoplasm, the nuclear volume expands approximately threefold, preparing the cell for the intricate processes of meiosis.
- In essence, interphase is not a mere period of cellular inactivity but a phase of meticulous preparation, ensuring that the cell is primed for the subsequent stages of division, whether mitotic or meiotic.
2. Prophase I
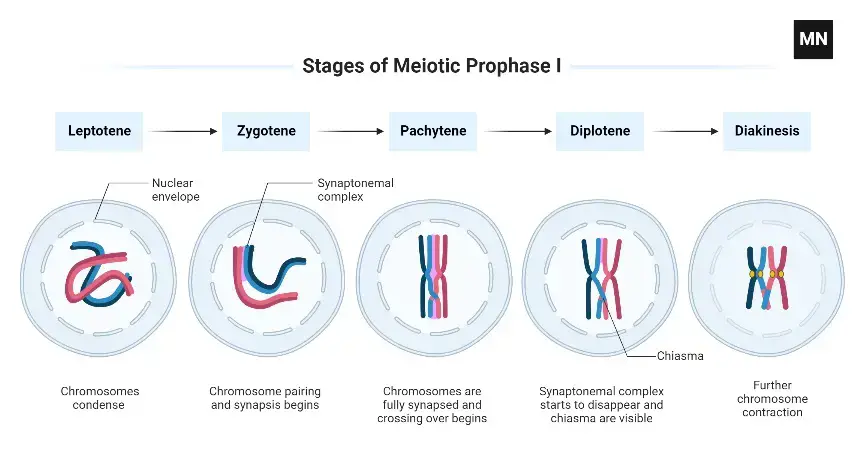
Prophase I is a pivotal and extended phase in meiosis, characterized by a series of distinct substages that facilitate the intricate processes of chromosome pairing and genetic recombination. Each substage plays a unique role in preparing the cell for subsequent phases of meiosis.
- Leptotene: During the leptotene substage, chromosomes undergo further uncoiling, adopting a thread-like morphology. These elongated structures exhibit bead-like formations known as chromomeres. Intriguingly, in animal cells, the orientation of these chromosomes towards the centrioles gives the nucleus a bouquet-like appearance, leading to the moniker “Bouquet Stage.”
- Zygotene or Synaptotene: The zygotene phase is marked by the initiation of synapsis, a process where homologous chromosomes pair up. This pairing is stabilized by a proteinaceous structure termed the synaptonemal complex. This complex not only solidifies the association between homologous chromosomes but also sets the stage for genetic recombination. The initiation of synapsis can occur in various patterns: from the chromosome ends moving towards the centromeres, from the centromeres extending towards the ends, or even at random points along the chromosomes.
- Pachytene: As the cell transitions into the pachytene substage, the paired chromosomes become intricately coiled around each other, rendering them indistinguishable as separate entities. A significant event during this phase is the longitudinal splitting of each homologous chromosome into two chromatids. However, these chromatids remain anchored together at their shared centromere. This configuration, with its two chromosomes and four chromatids, is referred to as a bivalent or tetrad. The pachytene stage is of paramount importance due to the occurrence of “crossing over,” a process where genetic material is exchanged between non-sister chromatids of homologous chromosomes. This genetic reshuffling is facilitated by enzymes that break and rejoin chromatid segments, ensuring genetic diversity in the offspring.
- Diplotene: During diplotene, the synaptonemal complex dissolves, but the chromatids of the paired chromosomes remain physically connected at specific points termed chiasmata. As this stage progresses, these chiasmata migrate towards the chromosome ends, resembling the motion of a zipper.
- Diakinesis: In the concluding substage, diakinesis, the bivalent chromosomes undergo further condensation and are evenly dispersed within the nucleus. Concurrently, the nuclear envelope disintegrates, and the nucleolus vanishes. The chiasmata, having migrated to the chromosome ends, ensure that the chromatids remain interconnected until the subsequent metaphase stage.
In summary, Prophase I is a multifaceted stage in meiosis, marked by chromosome pairing, genetic recombination, and the establishment of physical connections between homologous chromosomes, setting the stage for their eventual segregation.
3. Metaphase I
- Metaphase I is a critical juncture in the meiosis I process, characterized by the alignment of homologous chromosome pairs at the cell’s central region, known as the metaphase plate. This phase is distinct from mitosis, where individual chromosomes line up, and is emblematic of the reductional nature of meiosis I.
- During Metaphase I, each homologous chromosome pair, consisting of two chromosomes from maternal and paternal origins, aligns side by side at the metaphase plate. This alignment is not predetermined, meaning the maternal or paternal chromosome can be oriented towards either pole, leading to the principle of independent assortment. This principle posits that each allele has an equal likelihood of being transmitted to the subsequent generation, a foundational concept in genetics.
- The alignment of these homologous pairs is facilitated by spindle fibers, which attach to the chromosomes’ centromeres. These spindle fibers originate from opposite poles of the cell and connect to the kinetochores of the homologous chromosomes. This unique attachment, termed a bipolar attachment, ensures that each member of the homologous pair is anchored to opposite poles. The cohesin protein complex plays a pivotal role in holding the sister chromatids of each chromosome together until they are separated in the subsequent anaphase.
- A noteworthy aspect of Metaphase I is the tension generated by the opposing forces exerted by the spindle fibers. This tension ensures the correct bi-orientation of chromosomes, a prerequisite for the cell to progress to the next phase. In meiosis, the establishment of this tension typically necessitates at least one crossover event per chromosome pair, in conjunction with the cohesin complex.
- The culmination of Metaphase I sees the bivalents, each consisting of two homologous chromosomes with four chromatids, precisely aligned along the metaphase plate. Each bivalent possesses four kinetochores, which, due to their proximity, appear as a singular unit. These kinetochores face the same pole, allowing them to attach to spindle fibers emanating from the opposite pole. This configuration sets the stage for the subsequent separation of homologous chromosomes during anaphase. The random orientation of bivalents ensures that the resulting daughter cells inherit a diverse combination of maternal and paternal chromosomes, underscoring the genetic variability introduced during meiosis.
4. Anaphase I
- Anaphase I is a pivotal stage in the meiosis I process, distinguished by the separation and movement of homologous chromosomes towards opposite spindle poles. This phase starkly contrasts with mitosis, where sister chromatids are the entities that separate and migrate.
- During Anaphase I, the homologous chromosomes, each comprising two sister chromatids, are pulled apart by the kinetochore microtubules. These kinetochore microtubules, emanating from the spindle poles, attach to the kinetochores of the chromosomes, facilitating their movement. It’s noteworthy that in this phase, the sister chromatids remain conjoined at their centromeres, ensuring that only homologous chromosomes, not the sister chromatids, are segregated. This distinction is fundamental to the reductional nature of meiosis I, resulting in two cells, each with a haploid chromosome number by the end of this phase.
- The mechanism that ensures the retention of sister chromatid cohesion during Anaphase I is the protein shugoshin. Shugoshin acts as a protector, specifically safeguarding the centromeric region of the chromosomes. In mitosis, an enzyme called separase cleaves the cohesin complex holding the sister chromatids together, allowing their separation. However, in meiosis I, shugoshin prevents separase from acting on the centromeric cohesin, ensuring that the sister chromatids remain intact.
- Furthermore, due to the genetic recombination events during prophase I, specifically the chiasma formation, the two sister chromatids of a homologous chromosome are not genetically identical. This introduces genetic diversity, a hallmark of sexual reproduction.
- In summary, Anaphase I is characterized by the segregation of homologous chromosomes to opposite poles, facilitated by kinetochore microtubules, while the sister chromatids remain attached. This phase underscores the reductional division of meiosis I, setting the stage for the subsequent meiotic division.
5. Telophase I
- Telophase I marks the culmination of meiosis I and is characterized by the segregation of chromosomes to the opposite spindle poles. As the phase progresses, each pole receives a haploid set of chromosomes, signifying a reduction in chromosome number from the original diploid parent cell.
- Upon the chromosomes reaching their respective poles, the nuclear envelope starts to reassemble around them, encapsulating each haploid set. This reformation of the nuclear envelope signifies the establishment of two distinct daughter nuclei within the cell. Concurrently, the tightly coiled chromosomes begin to relax and revert to their more extended chromatin state, preparing the cell for the subsequent meiotic division.
- It’s noteworthy that while these chromosomes have been halved in number, each still comprises a pair of sister chromatids. These chromatids remain conjoined at their centromeres, emphasizing the distinction between meiosis I and mitosis, where sister chromatids are separated.
- Following the nuclear events, the cell undergoes cytokinesis. This process involves the division of the cytoplasm, leading to the formation of two distinct daughter cells. In some instances, cytokinesis may not complete immediately, resulting in cytoplasmic bridges. These bridges facilitate the sharing of cytoplasm between daughter cells until the conclusion of meiosis II.
- Subsequent to telophase I, cells might enter a brief resting phase termed interkinesis or interphase II. Importantly, this phase does not involve DNA replication, distinguishing it from the interphase preceding mitosis or meiosis I.
- In summary, telophase I is characterized by the segregation of haploid sets of chromosomes to opposite poles, the reformation of nuclear envelopes around these sets, and the eventual division of the cell into two daughter cells, each with a haploid set of chromosomes. This phase sets the stage for the second meiotic division, ensuring the continuation of the process of sexual reproduction.
6. Cytokinesis I
- Cytokinesis I represents the final step of meiosis I, facilitating the physical separation of the cell into two distinct daughter cells. This process is executed differently in animal and plant cells, reflecting their unique cellular structures and requirements.
- In animal cells, cytokinesis I is achieved through a mechanism known as cleavage furrow formation. Here, the cell membrane undergoes constriction, gradually tightening until the parent cell is pinched into two separate entities. This constriction is driven by a ring of actin and myosin filaments that contract, pulling the membrane tightly inwards.
- Conversely, plant cells, with their rigid cell walls, employ a different strategy for cytokinesis I. Instead of membrane constriction, plant cells develop a cell plate at the midline of the dividing cell. This cell plate is composed of vesicles from the Golgi apparatus that fuse together, laying down components for a new cell wall. As these vesicles continue to merge, the cell plate enlarges until it fuses with the cell membrane, effectively partitioning the cell into two daughter cells, each encased in its own cell wall.
- In essence, cytokinesis I ensures the successful division of a parent cell into two daughter cells, each inheriting a haploid set of chromosomes. The specific mechanism of this division, whether through membrane constriction in animals or cell plate formation in plants, is tailored to the structural and functional needs of the organism.
Results of Meiosis I
Meiosis I culminates in the formation of two distinct daughter cells, each characterized by a haploid set of chromosomes. Unlike the diploid state of the original cell, where two sets of chromosomes existed, these daughter cells possess only one set. However, it’s crucial to note that each chromosome within these haploid cells is composed of two sister chromatids, which remain conjoined at the centromere.
This reduction from a diploid to a haploid state is a defining feature of meiosis I, setting the stage for the subsequent division in meiosis II. While the daughter cells are haploid in terms of chromosome number, the presence of two sister chromatids for each chromosome means that they still retain two copies of the genetic information.
Following meiosis I, the cells typically undergo a brief interlude, often termed as a resting phase, before progressing to meiosis II. This subsequent phase will further segregate the sister chromatids, ensuring the production of four genetically unique haploid gametes by the conclusion of the entire meiotic process.
B. Phases of Meiosis II
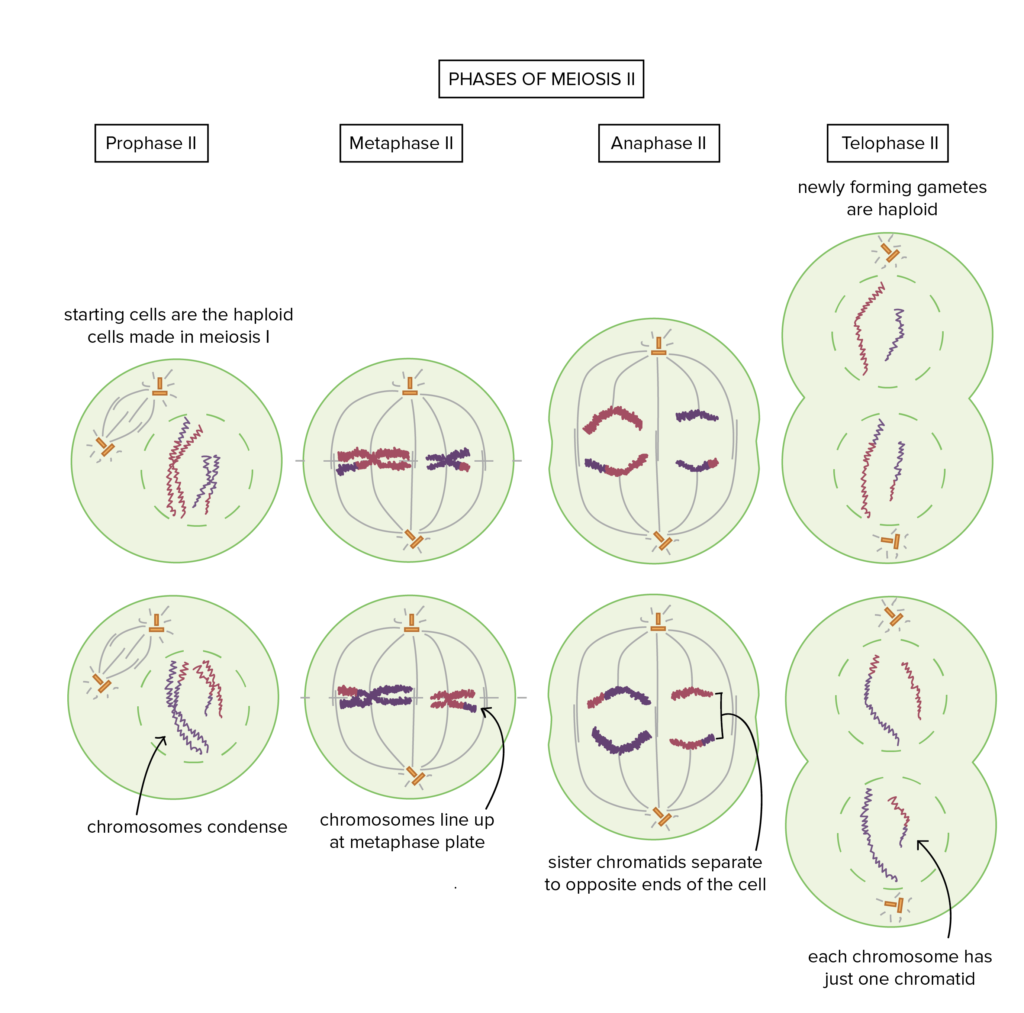
- Meiosis II, often termed the equational division, is the subsequent phase of the meiotic process, closely mirroring the mechanisms observed in mitosis. However, its genetic outcomes are distinct, primarily due to the haploid nature of the cells entering this phase. The primary objective of meiosis II is to segregate the sister chromatids of each chromosome into separate cells, ensuring genetic diversity.
- Initiating after the completion of meiosis I, meiosis II encompasses four primary stages: prophase II, metaphase II, anaphase II, and telophase II. Unlike the preceding meiotic phase, meiosis II does not involve the pairing of homologous chromosomes or recombination events. Instead, it focuses on the separation of sister chromatids.
- During prophase II, the nuclear envelope disintegrates, and the chromosomes, each composed of two chromatids, become more condensed. Spindle fibers begin to form, preparing for the alignment of chromosomes. In metaphase II, these chromosomes align at the metaphase plate, akin to mitosis. Anaphase II witnesses the separation of the sister chromatids, which are then pulled to opposite poles of the cell. Finally, during telophase II, nuclear envelopes reform around the separated chromatids, now considered individual chromosomes, leading to the formation of four genetically distinct haploid cells.
- It’s pivotal to note that DNA replication does not occur before meiosis II. The chromatids entering this phase were generated during the DNA replication that preceded meiosis I. Thus, meiosis II operates without an intervening S phase, ensuring the production of four non-identical haploid cells from the initial diploid cell. This equational division serves to maintain the haploid state, setting the stage for subsequent fertilization events in sexually reproducing organisms.
1. Prophase II
- During Prophase II, the initial phase of the second meiotic division, cells undergo several critical transformations to prepare for subsequent stages of meiosis II. This phase, while bearing similarities to Prophase I, is notably distinct in its processes and outcomes.
- In Prophase II, the nuclear envelope that encases the chromosomes begins to disintegrate, leading to the disappearance of the nucleolus. Concurrently, the chromatids, which are the replicated forms of chromosomes, undergo condensation, becoming thicker and more compact. This condensation facilitates their subsequent alignment and separation.
- A pivotal event during this phase is the replication and migration of centrosomes. These cellular structures play a crucial role in orchestrating the movement of chromosomes during cell division. In Prophase II, each centriole within the centrosome duplicates, resulting in two pairs.
- These pairs then migrate to opposite poles of the cell, setting the stage for the formation of the spindle apparatus. Microtubules, emanating from these centrosomes, extend across the cellular space, seeking to connect to the kinetochores present on individual chromatids. This connection is vital for the accurate alignment and eventual separation of chromatids during the subsequent phases.
- It’s essential to highlight the distinction between Prophase I and Prophase II. While both phases involve chromosomal condensation and spindle formation, Prophase I is unique in its involvement of chromosomal crossing over, a process absent in Prophase II. This crossing over in Prophase I contributes to genetic diversity, a hallmark of meiosis.
- In summary, Prophase II is a preparatory stage in meiosis II, characterized by chromosomal condensation, nuclear envelope breakdown, and centrosome migration. Its primary function is to ready the cell for the precise alignment and separation of chromatids in the ensuing stages of meiosis II.
2. Metaphase II
- Metaphase II, a pivotal stage in the second meiotic division, is marked by the strategic alignment of chromosomes at the cell’s equatorial plane. This phase, while bearing resemblance to the metaphase of mitosis, is distinct due to the halved chromosome number present in the cell.
- In Metaphase II, chromosomes, each consisting of two sister chromatids, align themselves at the cell’s central region, known as the metaphase plate. This alignment is facilitated by spindle fibers, which are microtubular structures emanating from the centrosomes located at the cell’s poles. These spindle fibers attach to the centromere of each chromosome, ensuring their precise positioning at the metaphase plate.
- A critical event during this phase is the division of the centromere, a region that holds the two sister chromatids together. However, it’s essential to note that while the centromere divides, the sister chromatids remain connected. The protein cohesin plays a vital role in maintaining this connection, ensuring that the chromatids do not prematurely separate.
- The alignment of chromosomes in Metaphase II is of paramount importance, as it sets the stage for the subsequent and accurate segregation of chromatids during Anaphase II. The precise positioning ensures that each resulting daughter cell will inherit a complete set of haploid chromosomes.
- In summary, Metaphase II is characterized by the central alignment of chromosomes, facilitated by spindle fibers and stabilized by the protein cohesin. This alignment is foundational for the accurate distribution of genetic material in the subsequent phases of meiosis II.
3. Anaphase II
- Anaphase II is a critical juncture in the meiotic process, succeeding Metaphase II. This phase is characterized by the segregation of sister chromatids, which are then directed towards the opposite poles of the cell. This movement is facilitated by the shortening of the kinetochore microtubules, which are attached to the chromatids.
- Drawing parallels with mitotic anaphase, Anaphase II also witnesses the separation of chromatids. However, it’s essential to differentiate between the two processes. In mitosis, the division ensures that each daughter cell receives an identical set of duplicated chromosomes. In contrast, during Anaphase II of meiosis, the division ensures that each daughter cell receives a unique set of unduplicated chromosomes.
- Upon the commencement of Anaphase II, the cohesin proteins, which previously held the sister chromatids together, are degraded, allowing for their separation. Once separated, these chromatids, now termed sister chromosomes, are actively pulled towards the centrioles located at the cell’s poles.
- This phase, termed the equational division, ensures that while the number of chromosomes in each resulting cell remains consistent with the parent cell, each chromosome is now unduplicated. In essence, Anaphase II is instrumental in maintaining the chromosome count while ensuring the genetic uniqueness of each resulting daughter cell.
4. Telophase II
- Telophase II marks the culmination of the meiotic process. In this phase, the separated chromatids, now recognized as individual chromosomes, finalize their migration to the opposite poles of the cell. As they reach their respective poles, the endoplasmic reticulum orchestrates the formation of a new nuclear envelope around each set of chromosomes, signifying the re-establishment of the nucleus. Concurrently, the nucleolus, essential for ribosomal RNA synthesis, re-emerges within these newly formed nuclei.
- Following these nuclear events, the cell undergoes cytokinesis, a process that divides the cytoplasm, leading to the formation of four distinct haploid cells from the original diploid cell. These haploid cells possess half the chromosome number of the original cell, rendering them genetically unique.
- It’s noteworthy that while Telophase II concludes the meiotic divisions, the resultant cells, especially in the context of spermatogenesis in many animals, including humans, may require further maturation. For instance, sperm cells necessitate the development of flagella to attain motility and functionality.
- In essence, Telophase II is a pivotal phase ensuring the genetic diversity inherent in sexual reproduction, producing four genetically distinct haploid cells, each poised for subsequent developmental processes or fertilization events.
5. Cytokinesis II
- Cytokinesis II is the concluding phase of meiosis II, responsible for the partitioning of the cytoplasm among the nascent daughter cells. This phase ensures that each of the four daughter cells, which are already genetically distinct due to the preceding meiotic events, receives an equitable share of the cellular organelles, enzymes, and other cytoplasmic components.
- The mechanism of cytokinesis II mirrors that of cytokinesis I. In animal cells, a contractile ring composed of actin filaments forms at the center, constricting the cell and leading to the formation of a cleavage furrow. This furrow deepens and eventually bisects the cell, culminating in the separation of the two daughter cells. In contrast, plant cells form a cell plate at the midline, which eventually develops into a cell wall, thereby segregating the two offspring cells.
- The culmination of cytokinesis II ensures that the four resultant daughter cells are not only haploid, with half the chromosome number of the original cell, but also possess the requisite cellular machinery to function and mature into gametes.
Results of Meiosis II
Upon the conclusion of meiosis II, the cell yields four distinct daughter cells, each of which is haploid in nature. This means that these cells possess half the number of chromosomes as the original diploid cell from which they originated. Each of these chromosomes carries a unique combination of genetic material, a consequence of the genetic recombination and independent assortment events that transpired during meiosis I.
These resultant haploid cells are primed to differentiate into gametes: in females, they mature into ova or eggs, while in males, they develop into spermatozoa or sperm. The significance of these gametes lies in their role in sexual reproduction. When two such gametes – one from each parent – fuse during fertilization, they restore the diploid chromosome number in the zygote, ensuring the continuity of genetic information across generations.
The genetic diversity introduced during meiosis, manifesting in these haploid cells, is a cornerstone of evolutionary biology. It not only provides a basis for variation within a population but also equips species with the genetic flexibility to adapt to changing environments, thereby playing a pivotal role in the evolutionary trajectory of organisms.
Errors in Meiosis
Meiosis is a highly regulated and intricate process responsible for the formation of gametes in humans and other sexually reproducing organisms. However, like any biological process, meiosis is susceptible to errors, which can have significant implications for human reproduction and genetic health. This discussion delves into various types of errors that can occur during meiosis and their consequences.
1. Asynapsis – Disrupted Chromosomal Pairing:
One common error in meiosis is known as “asynapsis.” Asynapsis occurs when homologous chromosomes fail to pair correctly during meiotic prophase I. This disruption in chromosomal pairing can lead to significant problems. In humans, it has been observed that more than 30% of oocyte pachytene stages exhibit asynapsis.
Consequences: Asynapsis can result in infertility and the production of gametes (sperm and eggs) with genetically imbalanced features. When asynapsis occurs, it often triggers cell checkpoints. In yeast, for example, such errors can lead to cell death. In humans, it contributes to the depletion of germ cells, which can lead to premature menopause in women. This condition is more significant in females because women produce a limited number of oocytes during their lifetime compared to the continuous production of sperm in males.
2. Chromosomal Pairing Failures at Metaphase I:
At metaphase I of meiosis, errors can occur when chromosome pairs fail to cross over properly. Cross-over is essential for genetic recombination and ensures proper chromosome segregation. When cross-over fails, unpaired chromosomes may segregate randomly during anaphase I.
Consequences: These errors can result in the production of aneuploid gametes, which contain an imbalanced number of chromosome copies. Aneuploid gametes can contribute to genetic abnormalities in offspring. Additionally, spermatocytes with failed crossing-over may undergo apoptosis or necrosis, reducing the number of functional sperm, potentially leading to infertility.
Sex-Specific Differences:
It’s important to note that the consequences of meiotic errors can vary between males and females due to differences in gametogenesis. Males continuously produce a large number of sperm throughout their lives, reducing the impact of errors. In contrast, females have a finite number of oocytes, making them more vulnerable to meiotic errors.
Genetic Abnormalities and Mental Implications:
Meiotic errors are major contributors to congenital genetic abnormalities, such as Down syndrome, and can result in various developmental disorders. These genetic anomalies can have lifelong implications for individuals.
In summary, meiosis is a complex and highly regulated process, but it is not immune to errors. Errors in meiosis can result in infertility, the production of genetically imbalanced gametes, and a higher risk of genetic abnormalities in offspring. Understanding these errors and their consequences is crucial in the fields of reproductive medicine and genetic counseling, as it allows for better management of reproductive health and genetic conditions.
Significance of Meiosis/Biological Importance of Meiosis
Meiosis is of paramount significance in biology due to its several biological and evolutionary roles. Its primary importance lies in the production of gametes (sperm and egg cells) and genetic diversity, which are fundamental to the continuation of life and the adaptation of species to changing environments. Here are the key biological importance and significance of meiosis:
- Gamete Formation: Meiosis is the specialized cell division that produces haploid gametes (sperm and eggs) from diploid germ cells. These haploid gametes are essential for sexual reproduction in most organisms. When fertilization occurs, the fusion of two gametes (sperm and egg) restores the diploid chromosome number in the zygote, which is the first cell of the new organism.
- Genetic Diversity: Meiosis introduces genetic diversity within a population through several mechanisms:
- Crossing Over: During prophase I of meiosis, homologous chromosomes exchange genetic material through a process called crossing over. This genetic recombination creates novel combinations of alleles and contributes to genetic diversity.
- Independent Assortment: During meiosis I, homologous chromosomes segregate independently into daughter cells. The random orientation of chromosome pairs at the metaphase plate results in different combinations of maternal and paternal chromosomes in gametes, further increasing genetic diversity.
- Adaptation and Evolution: Genetic diversity generated by meiosis is the raw material for natural selection and evolution. It allows populations to adapt to changing environments over generations. Beneficial genetic variations are more likely to be passed on to offspring, enhancing the fitness of the species.
- Repair of DNA Damage: Meiosis can repair genetic damage by allowing for homologous recombination between damaged DNA strands and their intact homologous counterparts. This process can help correct mutations and maintain genomic stability.
- Determination of Sex: In many species, including humans, meiosis plays a crucial role in determining an individual’s sex. The type of gamete (sperm or egg) produced during meiosis determines the genetic sex of the offspring.
- Polyploidy: Meiosis can lead to polyploidy, a condition where an organism has more than two complete sets of chromosomes. Polyploidy can result in new species and is particularly common in plants, contributing to their diversity.
In summary, meiosis is biologically significant because it ensures the continuity of life through the production of gametes, contributes to genetic diversity, fuels evolution, repairs genetic damage, determines sex, and even leads to the creation of new species in some cases. It is a fundamental process that underpins the genetic variability and adaptability of life on Earth.
Applications of Meiosis
Meiosis, a specialized form of cell division, plays a pivotal role in various scientific and biotechnological applications. Here are some of the key applications derived from the process of meiosis:
- Tissue Culture: Meiosis is instrumental in tissue culture techniques, especially when the objective is to study gametic cells or to induce genetic variation in cultured tissues.
- Gametic Cell Generation: In the realm of biotechnology, meiosis is harnessed to achieve a gametic state in cells. This is particularly valuable when studying the genetic makeup and behavior of gametes under controlled conditions.
- Evolutionary Studies: The inherent variability introduced by meiosis, through processes like crossing over and independent assortment, is crucial for understanding evolutionary dynamics. By generating genetic diversity, meiosis provides a substrate for natural selection, driving evolutionary change.
- In-vitro Gamete Formation: One of the groundbreaking applications of meiosis is the in-vitro formation of gametes. In cases of infertility stemming from gamete production failure, researchers can differentiate embryonic stem cells into germ-like cells using meiotic division. These in-vitro produced gametes can then be introduced into individuals, offering a potential solution to certain infertility challenges.
- Genetic Variation Generation: Meiosis, in tandem with mitosis, is employed to introduce genetic variations. This is particularly valuable in research settings where the objective is to study the effects of genetic variability on certain traits or conditions.
In summary, meiosis is not just a biological process restricted to reproduction; its applications extend to various fields of biotechnology and research, making it an indispensable tool in modern science.
Examples of Meiosis
Meiosis, the specialized cell division process, is a fundamental biological mechanism that occurs in various organisms, including fungi, plants, algae, and animals, including humans. Its significance and occurrence in different organisms shed light on the diversity and complexity of life cycles. Below, we explore examples of meiosis in various organisms:
1. Meiosis in Plants and Algae:
In plants and algae, meiosis plays a crucial role in their life cycle, which involves an alternation of generations between haploid and diploid phases. This phenomenon is known as “sporic meiosis.” Here’s how it works:
- Sporophyte and Gametophyte: Plants and algae have both haploid and diploid forms of cells in their life cycle. The diploid phase is called the sporophyte, which produces haploid spores through meiosis. These spores then germinate and undergo mitotic divisions to form the haploid phase, known as the gametophyte.
- Gamete Formation: Within the gametophyte, haploid gametes (sperm and egg cells) are produced through mitotic divisions. These gametes fuse during fertilization, giving rise to the diploid sporophyte once again.
- Meiosis Role: Meiosis occurs during the formation of spores, reducing the chromosome number from diploid to haploid. It ensures genetic diversity in offspring by shuffling genetic material between homologous chromosomes.
2. Meiosis in Fungi:
Fungi exhibit both asexual and sexual phases in their life cycle. Meiosis is involved in the sexual phase and contributes to genetic diversity:
- Plasmogamy and Karyogamy: In the sexual phase of fungi, the haploid mycelium undergoes plasmogamy (fusion of protoplasts) and karyogamy (fusion of haploid nuclei). This leads to the formation of a diploid zygote.
- Meiosis and Spore Formation: The diploid zygote develops into a stalked sporangium, where meiosis occurs. Meiosis produces haploid spores, known as meiospores, which are released from the sporangium. Each meiospore can germinate into a new haploid mycelium, contributing to genetic diversity.
3. Meiosis in Humans and Animals:
Meiosis is essential for the formation of gametes (sperm and egg cells) in humans and other animals:
- Spermatogenesis: In males, meiosis starts at puberty and continues throughout their lifetime. It leads to the production of haploid sperm cells with specialized features, such as a functional flagellum.
- Oogenesis: In females, meiosis begins before birth, with primary oocytes arrested at prophase I. At puberty, one primary oocyte each month resumes meiosis and progresses to metaphase II. However, meiosis is completed only upon fertilization. If fertilization doesn’t occur, the secondary oocyte disintegrates, leading to menstruation.
Significance of Meiosis: Meiosis is significant as it ensures genetic diversity, facilitates sexual reproduction, contributes to adaptation and evolution, repairs DNA damage, determines an individual’s sex, and can lead to polyploidy in plants, enhancing species’ diversity.
In summary, meiosis occurs in various organisms, and its specific roles and mechanisms vary depending on the organism’s life cycle and reproductive strategies. It is a fundamental process that contributes to genetic diversity and the continuation of species.
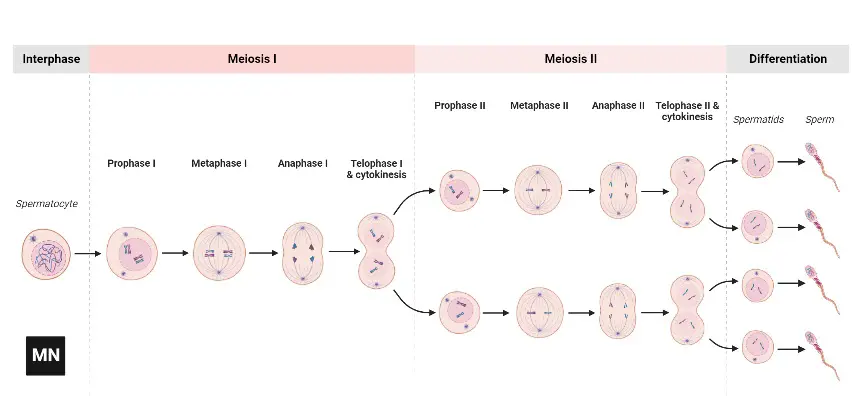
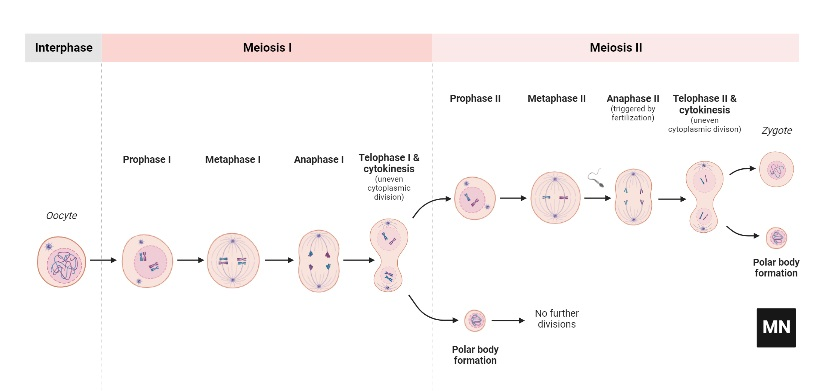
Comparison/Difference Between Mitosis And Meiosis
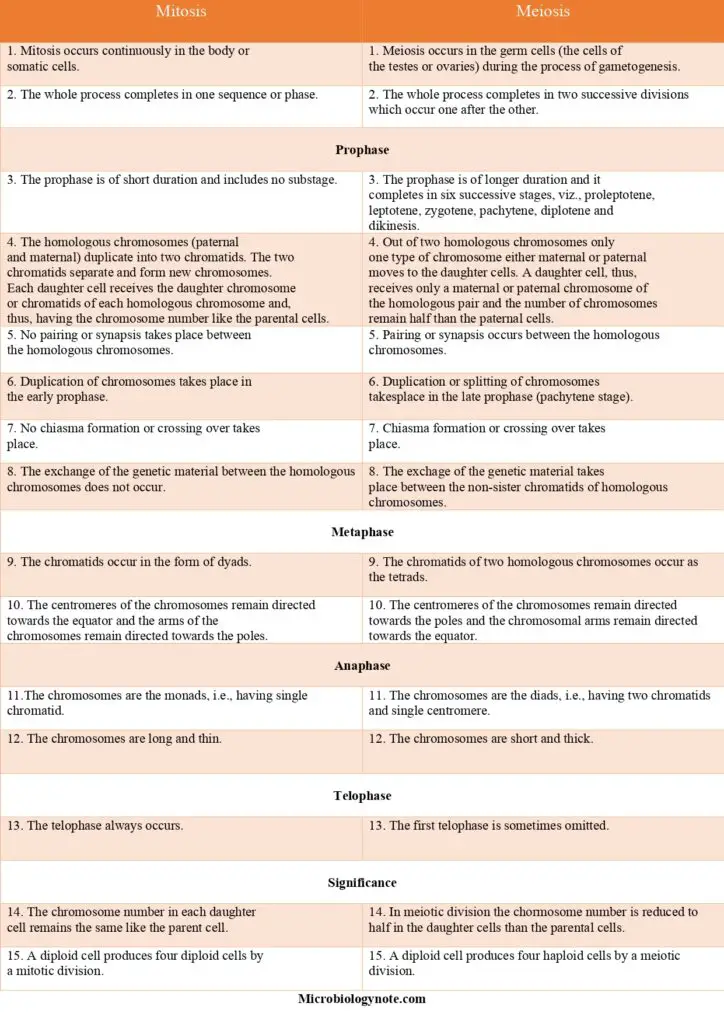
Quiz
Question 1: During which phase of meiosis do homologous chromosomes separate?
A) Prophase I
B) Metaphase I
C) Anaphase I
D) Telophase I
Question 2: How many daughter cells are produced at the end of meiosis I?
A) 1 haploid cell
B) 2 haploid cells
C) 2 diploid cells
D) 4 haploid cells
Question 3: What is the main purpose of meiosis?
A) To produce genetically identical cells
B) To increase the chromosome number
C) To produce gametes with genetic diversity
D) To repair damaged DNA
Question 4: During which phase of meiosis do sister chromatids separate?
A) Prophase I
B) Metaphase I
C) Anaphase I
D) Anaphase II
Question 5: What is the result of meiosis in humans?
A) 4 haploid sperm cells
B) 4 haploid egg cells
C) 2 haploid sperm cells and 2 haploid egg cells
D) 2 diploid sperm cells and 2 diploid egg cells
Question 6: In which phase of meiosis does crossing-over occur?
A) Prophase I
B) Metaphase I
C) Prophase II
D) Metaphase II
Question 7: What is the term for the exchange of genetic material between homologous chromosomes?
A) Separation
B) Replication
C) Crossing-over
D) Aneuploidy
Question 8: How does meiosis II differ from meiosis I?
A) Meiosis II results in diploid cells, while meiosis I results in haploid cells.
B) Meiosis II involves crossing-over, while meiosis I does not.
C) Meiosis II produces four haploid cells, while meiosis I produces two haploid cells.
D) Meiosis II has a longer prophase than meiosis I.
Question 9: In humans, when does meiosis in females begin?
A) At birth
B) During puberty
C) Before fertilization
D) During pregnancy
Question 10: What is the primary outcome of meiosis in terms of chromosome number?
A) Reduction from diploid to haploid
B) Duplication from haploid to diploid
C) No change, remains diploid
D) Varied, depends on the organism
FAQ
Can a haploid cell undergo meiosis?
Haplodiploid cells are incapable of meiosis. By mitotic division, a haploid organism (n) generates gametes (n). A zygote that is diploid is created after the fertilisation of these gametes (n). After meiosis, this zygote or diploid cell divides once more to create a haploid organism.
Can meiosis occur in plants?
Meiosis occurs in both plants and animals. The end result, the production of gametes with half as many chromosomes as the parent cell, is identical, but the process is distinct.
Is meiosis an animal or plant?
Animals create gametes directly through the process of meiosis, whereas plants make spores. The spores generate a gametophyte, which then splits into gametes.
can meiosis occur without crossing over?
If crossing over does not occur, the resulting gametes are parental. The products of crossing over are recombinant gametes. The allelic mix of parental and recombinant gametes is determined by whether the initial cross involved genes in the coupling or repulsion phase.
Without crossing over, the alleles of two genes on each chromosome travel together and remain linked during meiosis. Therefore, we obtain gametes that are 100 percent “parental” and are classified into two categories based on allele separation.
can meiosis occur in all organisms?
Meiosis occurs in both plants and animals. The ultimate outcome, the creation of gametes with half as many chromosomes as the parent cell, is identical, but the mechanism is distinct. Meiosis creates gametes directly in mammals.
Haploid creatures conduct meiosis only during the zygote phase. The diploid zygote undergoes meiosis to generate the haploid thallus.
References
- Griswold, M. D., & Hunt, P. A. (2013). Meiosis. Brenner’s Encyclopedia of Genetics, 338–341. doi:10.1016/b978-0-12-374984-0.00916-5
- Meiosis. (2017). Cell Biology, 779–795. doi:10.1016/b978-0-323-34126-4.00045-1
- Moens, P. B. (2001). Meiosis. Encyclopedia of Genetics, 1163–1165. doi:10.1006/rwgn.2001.0807
- Becker, W. M., Kleinsmith, L. J., Hardin, J., & Bertoni, G. P. (2004). The world of the cell (Vol. 4). Menlo Park, CA: Benjamin/Cummings.
- Hultén, M. A. (2010). Meiosis. Encyclopedia of Life Sciences.
- Cooper, G. M., & Hausman, R. E. (2000). A molecular approach. The Cell. 2nd ed. Sunderland, MA: Sinauer Associates.
- Alberts, B., Johnson, A., Lewis, J., Raff, M., Roberts, K., & Walter, P. (2002). Meiosis. In Molecular Biology of the Cell. 4th edition. Garland Science.