What Is a Peptide Bond?
- A peptide bond, scientifically referred to as an eupeptide bond, is a pivotal chemical bond formed by conjugating the carboxyl group of one amino acid to the amino group of another. This bond is characterized as an amide-type of covalent chemical bond. It serves as a bridge linking two sequential alpha-amino acids, specifically from C1 (carbon number one) of one alpha-amino acid to N2 (nitrogen number two) of another. This specific linkage is a hallmark feature observed along a peptide or protein chain.
- The genesis of a peptide bond is accompanied by the release of water (H2O) molecules, rendering it a dehydration process. This is because the bond is predominantly a covalent bond (CO-NH bond) and the elimination of a water molecule is inherent to its formation. The process predominantly transpires between amino groups.
- The term “peptide” has its roots in the Greek word meaning “digested”. A peptide is essentially a short polymer constituted of amino acid monomers that are interconnected by an amide bond. Peptide bonds are foundational to the structure and function of living organisms. They facilitate the formation of elongated chains of amino acids, which are recognized as proteins. These proteins play multifaceted roles, ranging from providing structural support to catalyzing pivotal biological reactions and discerning molecules in the environment. Hence, a peptide bond is intrinsically linked to a plethora of biological reactions and is indispensable for life. The mechanism underlying the formation of peptide bonds is universally conserved across diverse life forms.
- In the realm of organic chemistry, a peptide bond is delineated as an amide type of covalent chemical bond that interlinks two consecutive alpha-amino acids from C1 of one alpha-amino acid to N2 of another, along a peptide or protein chain. It’s noteworthy that this bond can also be termed a eupeptide bond to differentiate it from an isopeptide bond, another variant of the amide bond between two amino acids.
- Recent scientific literature has shed light on the multifaceted roles of peptides and proteins in biological processes. For instance, a study by Akira Shigenaga highlighted the significance of peptide/amide bond cleavage in the realm of peptide/protein sciences. Another research article by Simon Baldauf et al. emphasized the importance of the α-ketoacid-hydroxylamine (KAHA) ligation in the coupling of unprotected peptide segments through the chemoselective formation of an amide bond. Furthermore, the influence of fluorinated prolines on the conformational preferences of the amide bond in peptides has been elucidated, offering insights into the potential of using 19F nuclear magnetic resonance (NMR) as a tool to probe conformational changes in polypeptides.
- In conclusion, the peptide bond is a cornerstone in the biochemistry of living organisms, playing a pivotal role in the structure and function of proteins. Its formation, characteristics, and implications in biological processes are subjects of extensive scientific research, underscoring its significance in the realm of molecular biology.
Peptide Bond Definition
A peptide bond is a covalent chemical bond formed between two amino acids when the carboxyl group of one amino acid reacts with the amino group of another, releasing a molecule of water. This bond is characteristic of and essential for linking amino acids in protein structures.
Peptide Bond Formation or Synthesis – How are Peptide bonds formed?
- The formation of a peptide bond is a quintessential biochemical process that underpins the synthesis of proteins, the workhorses of the cell. This bond is established through a specific chemical reaction known as dehydration synthesis or condensation reaction, predominantly occurring between amino acids.
- At the molecular level, as illustrated in various biochemical schematics, two amino acids come into proximity, initiating the formation of a peptide bond via dehydration synthesis. In this intricate process, one amino acid donates a carboxyl group, concurrently shedding a hydroxyl group, which comprises hydrogen and oxygen atoms. Simultaneously, the counterpart amino acid relinquishes a hydrogen atom from its NH2 group. As a result of this molecular exchange, the hydroxyl group is replaced by nitrogen, culminating in the formation of a peptide bond. Such bonds are occasionally termed substituted amide linkages due to their unique structural configuration. The covalent linkage established between the two amino acids results in the formation of a dipeptide.
- The hallmark of this reaction is the CO-NH bond, recognized as the peptide bond. The resultant molecule, characterized by the four-atom functional group -C(=O)NH-, is termed an amide, with the specific group being referred to as the amide or peptide group.
- Diving deeper into the mechanistic details, when two amino acids converge to form a dipeptide via a peptide bond, it epitomizes a condensation reaction. In this molecular dance, the non-side chain carboxylic acid moiety (C1) of one amino acid aligns with the non-side chain amino moiety (N2) of its partner. The ensuing reaction witnesses the loss of a hydrogen and oxygen from the carboxyl group (COOH) of one amino acid and a hydrogen atom from the amino group (NH2) of the other. This molecular rearrangement leads to the liberation of a water molecule (H2O) and the birth of a dipeptide, interconnected by a peptide bond (−CO−NH−).
- This synthesis of the amide bond transpires when the carboxyl group of one amino acid reacts with the amino group of another, releasing a water molecule in a dehydration synthesis reaction.
- It’s imperative to note that the genesis of the peptide bond is an energy-intensive process. In biological systems, this energy is harnessed from ATP. Peptides and proteins, the polymers of life, are chains of amino acids interconnected by peptide bonds, with occasional isopeptide bonds adding to the structural diversity. While ribosomes orchestrate the synthesis of ribosomal peptides, specialized enzymes are at the helm of nonribosomal peptide synthesis. A case in point is the tripeptide glutathione, synthesized in a two-step enzymatic process involving glutamate–cysteine ligase and glutathione synthetase.
- In summation, the formation of peptide bonds is a central biochemical event, underpinning the structure and function of proteins, and is governed by a series of intricate molecular interactions and reactions.
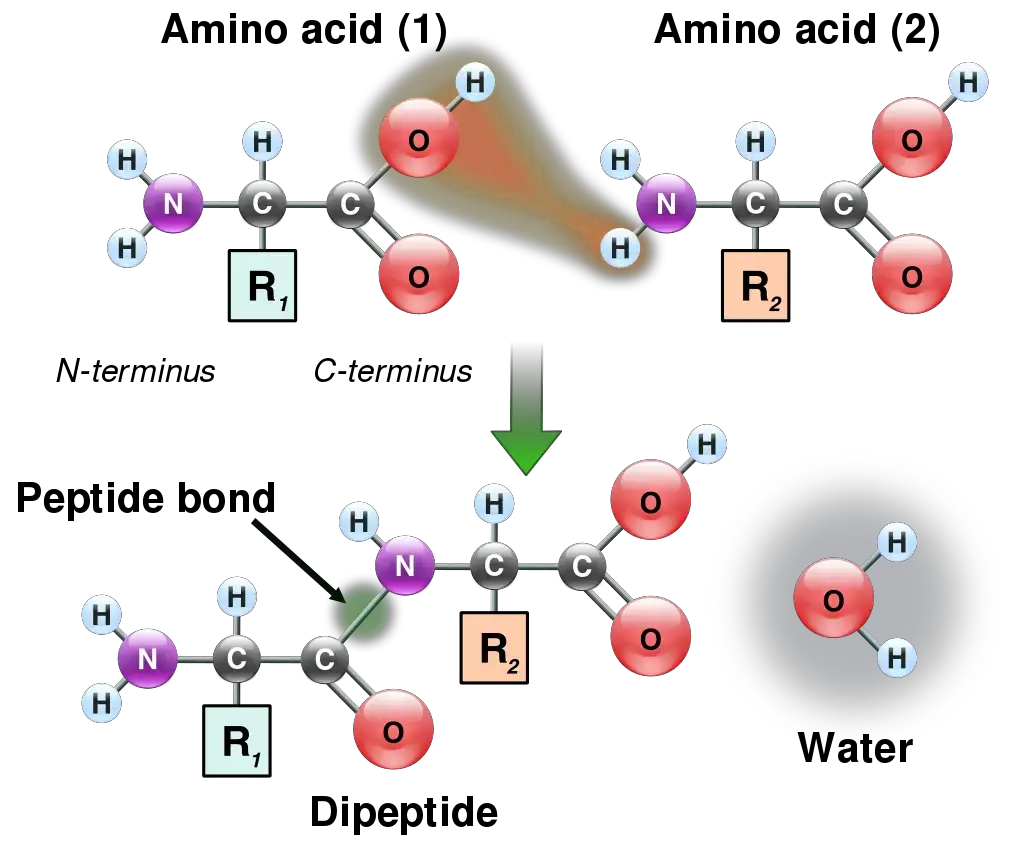
Peptide Bond Formation Steps
The formation of this bond is orchestrated through a series of sequential steps, each contributing to the intricate molecular dance that results in the linkage of amino acids. Herein, we delineate the stepwise progression of peptide bond formation:
- Step 1: Molecular Convergence of Amino Acids: In the initial phase, two distinct amino acid molecules are juxtaposed. The carboxyl group of one amino acid strategically aligns itself towards the amino group of its counterpart. This molecular alignment sets the stage for the subsequent chemical interactions.
- Step 2: Release of Molecular Entities and Water Formation: As the reaction progresses, the carboxyl group undergoes a molecular rearrangement, leading to the release of a hydroxyl ion (OH–). Concurrently, the amino group of the adjacent amino acid sheds a proton (H+). These two ions, the hydroxyl ion and the proton, synergistically combine, culminating in the formation of a water molecule. This water molecule is then extricated from the reaction milieu, a process emblematic of dehydration synthesis.
- Step 3: Establishment of the Peptide Bond: With the precursors primed, the two amino acids undergo a chemical reaction, resulting in the formation of a peptide bond. This bond serves as the molecular bridge, covalently linking the two amino acids.
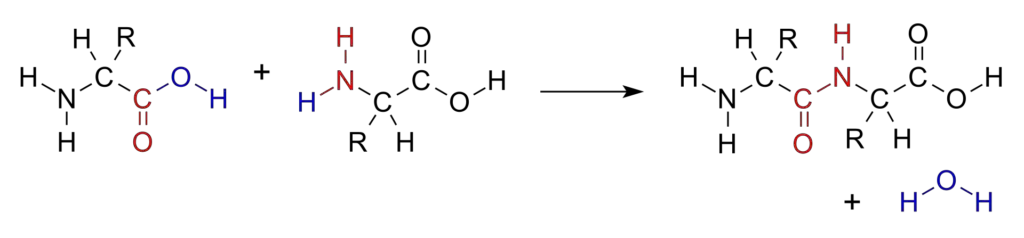
The product of this reaction is termed a dipeptide, aptly named as it is constituted of two amino acids. Analogously, when the process incorporates three amino acids, the resultant molecule is designated as a tripeptide.
In essence, the formation of a peptide bond is a meticulously orchestrated sequence of steps, each pivotal in ensuring the precise linkage of amino acids, thereby facilitating the synthesis of peptides and proteins, the macromolecules essential for life.
Characteristics of Peptide Bond
Peptide bonds, the foundational linkages in proteins, exhibit a range of distinctive characteristics that underscore their significance in biological systems. These bonds are pivotal in connecting amino acids, the building blocks of proteins. Herein, we elucidate the salient characteristics of peptide bonds, drawing insights from the provided contents:
- Formation through Dehydration Synthesis: Peptide bonds are established when two amino acids are linked through a process known as dehydration synthesis. This reaction involves the removal of a water molecule, facilitating the bond formation between the amino and carboxyl groups of the participating amino acids.
- Partial Double Bond Character: One of the hallmark features of peptide bonds is their partial double bond character, attributed to resonance. This characteristic imparts rigidity to the bond, making it resistant to rotation. As a consequence, peptide bonds are kinetically stable, necessitating high activation energy for their cleavage.
- Stability and Resistance: Peptide bonds are robust and are not easily disrupted by factors such as heating or high salt concentrations. Their stability is such that only prolonged exposure to strong acids or bases at elevated temperatures can break them. Additionally, certain specialized enzymes, notably digestive enzymes, can cleave these bonds.
- Planarity and Configuration: Peptide bonds are inherently planar, meaning the C=O and N-H bonds coexist on the same plane. This planarity restricts rotation around the peptide bond. Furthermore, the peptide group can adopt either a cis or trans configuration, with the trans configuration being favored due to reduced steric hindrance from adjacent amino acid side chains. This stereochemical aspect plays a pivotal role in stabilizing protein structures.
- Polarity and Hydrogen Bonding: The peptide bond is characterized by polar entities, specifically the partially positive hydrogen atoms of amino groups and the partially negative oxygen atoms of carboxyl groups. This inherent polarity facilitates the formation of hydrogen bonds between different regions of the peptide, further stabilizing the structure.
- Enzymatic Cleavage: While peptide bonds are inherently stable, they are not impervious to enzymatic action. Specific enzymes, particularly those involved in digestion, can target and cleave these bonds, underscoring the dynamic nature of biological systems.
In summation, peptide bonds are intricate molecular linkages with a suite of characteristics that render them indispensable in the realm of biochemistry. Their stability, planarity, and ability to form hydrogen bonds are central to their role in shaping the three-dimensional structures of proteins.
Different Types of Peptide Bond
Peptide bonds, the covalent linkages that connect amino acids, give rise to a spectrum of molecular structures, each distinguished by the number of amino acid units they encompass. These structures, ranging from simple dipeptides to complex macropeptides, underscore the versatility and diversity of peptide bonds in the realm of biochemistry. Herein, we delineate the various forms of peptide bonds based on their constituent amino acid units:
- Dipeptides: Dipeptides are the simplest form of peptides, constituted by a mere two amino acid units. The linkage between these two amino acids is facilitated by a singular peptide bond.
- Tripeptides: As the nomenclature suggests, tripeptides are composed of three amino acid units. These three units are interconnected by two peptide bonds, forming a linear chain.
- Tetrapeptides: Tetrapeptides are slightly more complex, encompassing four amino acid units. These units are sequentially linked by three peptide bonds.
- Oligopeptides: Oligopeptides represent a broader category of peptides, characterized by a composition of no more than ten amino acid units. Given their relatively short length, oligopeptides often play specialized roles in biological systems.
- Polypeptides: Polypeptides are elongated chains of amino acids, containing more than ten units but not exceeding a hundred residues. Their extended structure allows them to adopt diverse conformations, making them integral to various biochemical processes.
- Macropeptides: At the apex of the peptide hierarchy are macropeptides. These are colossal structures, constituted by more than a hundred amino acids. Given their substantial size, macropeptides often exhibit intricate three-dimensional configurations, underpinning their role in complex biological functions.
In essence, the realm of peptide bonds is marked by a rich tapestry of structures, each distinguished by its amino acid count. From the simplicity of dipeptides to the complexity of macropeptides, these forms highlight the adaptability and diversity of peptide bonds in orchestrating the myriad functions of proteins in living systems.
Resonance Structure of Peptide Bond
The peptide bond, a fundamental linkage in the realm of proteins and peptides, exhibits a unique structural characteristic that contributes to its stability: the phenomenon of resonance. This resonance is not merely a theoretical construct but has profound implications for the bond’s physical and chemical properties.
At the heart of the peptide bond’s configuration is the amide group, which is inherently planar and rigid. The stability conferred upon the peptide bond arises from the resonance of the amide. This resonance is a consequence of the interplay of electrons between the carbonyl group’s double bond (C=O) and the C–N bond. Such electron sharing or delocalization between bonds is emblematic of resonance structures.
This resonance effect imparts a partial double bond character to the C-N bond, while simultaneously bestowing a partial single bond character to the C=O bond. The implications of this are profound for the bond lengths within the peptide structure. Typically, a single bond between two atoms is longer than a double bond between the same pair. However, due to the resonance effect, the C–N bond in a peptide bond is shorter than what one would anticipate for a conventional C–N single bond. Conversely, the C=O bond, because of its partial single bond character, is longer than a standard C=O double bond.
In essence, the resonance structure of the peptide bond is a testament to the intricate dance of electrons that governs the bond’s stability and configuration. This nuanced electron sharing ensures that peptide bonds, the backbone of proteins, maintain their structural integrity and play their pivotal role in the biochemistry of life.
Degradation of Peptide Bond
- At the core of peptide bond degradation is the reaction of hydrolysis, where water is added to break the bond. This hydrolytic process is energetically favorable, releasing a Gibbs energy ranging from 8–16 kJ/mol. However, the spontaneous hydrolysis of peptide bonds in water is an incredibly slow phenomenon. At a temperature of 25°C, the half-life of a peptide bond, without any catalytic assistance, spans between 350 and 600 years. This means that, in the absence of catalysts, it would take several centuries for half of the peptide bonds in a sample to undergo hydrolysis.
- In the dynamic environment of living organisms, such a slow rate of degradation is impractical. Hence, nature employs specialized enzymes, known as proteases or peptidases, to expedite the process. These enzymes act as catalysts, significantly accelerating the rate of peptide bond hydrolysis. Their presence ensures that proteins are efficiently degraded and recycled as needed by the organism.
- Interestingly, there are instances where peptide bond hydrolysis is instigated by conformational strain during the folding of peptides or proteins into their native structures. This non-enzymatic mechanism does not benefit from the transition state stabilization typically provided by enzymes. Instead, it relies on the destabilization of the ground state, leading to bond degradation.
- In conclusion, while peptide bonds are robust and stable structures, they are not impervious to degradation. Through the action of water, enzymes, and even conformational strain, these bonds can be hydrolyzed, ensuring the dynamic equilibrium of proteins in biological systems.
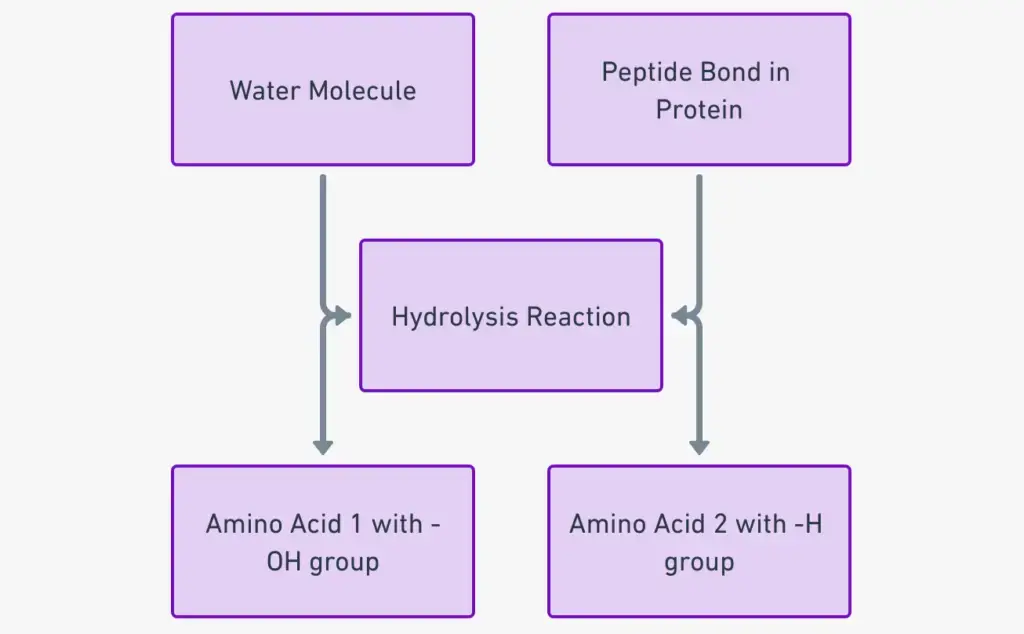
Hydrolysis of a peptide bond
The hydrolysis of a peptide bond refers to the cleavage of the bond between two amino acids in a peptide or protein chain, facilitated by the addition of a water molecule. This process is crucial in biological systems for the digestion and breakdown of proteins. Here, we’ll describe the steps involved in the hydrolysis of a peptide bond, using a simple dipeptide as an example.
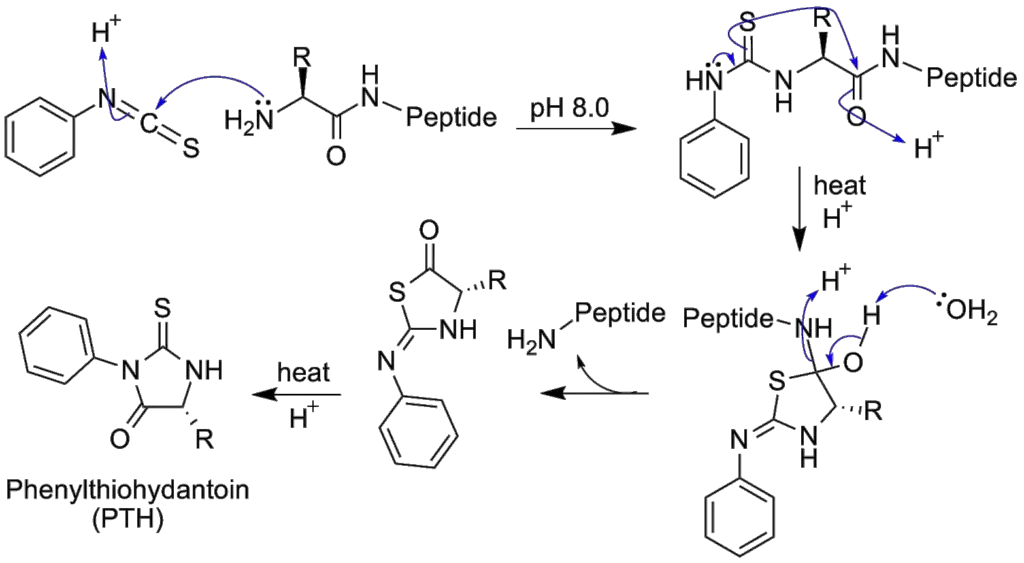
Example: Consider a dipeptide formed by two amino acids, Alanine (Ala) and Glycine (Gly), linked by a peptide bond.
Step 1: Introduction of Water
- The process begins with the introduction of a water molecule (H₂O) to the dipeptide. The water molecule will play a crucial role in breaking the peptide bond.
Step 2: Nucleophilic Attack
- The oxygen atom of the water molecule, being electronegative, acts as a nucleophile. It attacks the carbonyl carbon of the peptide bond, leading to the formation of a tetrahedral intermediate.
Step 3: Proton Transfer
- The hydrogen atom from the water molecule is transferred to the nitrogen atom of the peptide bond. This step is facilitated by an acid, often a side chain from another amino acid or an enzyme in biological systems.
Step 4: Cleavage of the Peptide Bond
- The tetrahedral intermediate collapses, leading to the breaking of the peptide bond. The nitrogen atom forms a bond with the hydrogen atom it received in the previous step, resulting in the formation of an amino group.
Step 5: Formation of Two Separate Amino Acids
- As a result of the hydrolysis, the dipeptide is split into two separate amino acids. In our example, Alanine and Glycine are released as individual amino acids. The carboxyl group of Alanine and the amino group of Glycine are now free and no longer linked by a peptide bond.
Step 6: Release of Energy
- The hydrolysis of the peptide bond is an exergonic reaction, meaning it releases energy. This energy is often utilized by cells for various metabolic processes.
Structure of Peptide Bond
- The peptide bond, a fundamental linkage in proteins, possesses a distinct structural configuration that is both planar and trans. This bond exhibits a partial double bond character, which is a consequence of the resonance or delocalization of electrons between the amide nitrogen and the carboxyl oxygen. This resonance effect imparts stability to the peptide bond and restricts its rotation.
- In terms of spatial arrangement, the atoms constituting the peptide bond, specifically carbon (C), hydrogen (H), nitrogen (N), and oxygen (O), all lie within the same plane. Notably, the hydrogen atom associated with the amide group and the oxygen atom of the carboxyl group are positioned trans to one another, further emphasizing the planarity of the bond.
- The discovery of the rigid and planar nature of peptide bonds is credited to scientists Linus Pauling and Robert Corey. Their findings have been instrumental in understanding the structural intricacies of proteins and the role of peptide bonds in maintaining protein architecture.
What is Cis/trans isomers of the peptide group?
The peptide group, a fundamental component of proteins, exhibits unique structural characteristics due to the significant delocalization of the lone pair of electrons on its nitrogen atom. This delocalization imparts a partial double-bond character to the peptide group, rendering it planar. Consequently, the peptide group can exist in two distinct geometric isomers: cis and trans.
The planarity of the peptide group arises from the partial double bond, allowing it to adopt either the cis or trans configuration. In an unfolded protein state, peptide groups can freely isomerize between these configurations. However, once the protein assumes its folded state, typically only one isomer predominates at each position, with exceptions being rare. The trans isomer is predominantly favored in most peptide bonds, with a striking ratio of approximately 1000:1 in favor of trans over cis. An intriguing exception is observed in X-Pro peptide groups, where the ratio is closer to 30:1. This deviation can be attributed to the symmetrical nature of proline’s Cα and Cδ atoms, rendering the energies of cis and trans isomers nearly equivalent.
The dihedral angle, represented by ω, associated with the peptide group is defined by the sequence of atoms Cα–C’–N–Cα. For the cis isomer, ω is 0°, indicating a synperiplanar conformation. In contrast, the trans isomer exhibits an antiperiplanar conformation with ω being 180°. Isomerization between these forms involves rotation around the C’–N bond. This process, although feasible, is slow, with a typical time frame of around 20 seconds at ambient conditions. The transition state, characterized by ω values of ±90°, necessitates the disruption of the partial double bond, leading to a substantial activation energy of approximately 80 kJ/mol. However, certain factors, such as a hydrophobic environment or hydrogen bond donation to the nitrogen atom in an X-Pro peptide group, can reduce this activation energy. These mechanisms have been observed in peptidyl prolyl isomerases (PPIases), enzymes that facilitate the cis-trans isomerization of X-Pro peptide bonds.
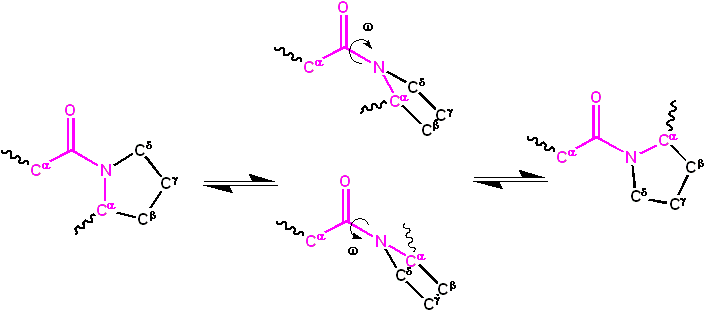
The kinetics of protein folding and cis-trans isomerization are markedly different. While protein folding typically occurs within 10-100 milliseconds, isomerization can take 10-100 seconds. The presence of non-native isomers can significantly impede the protein folding process. Some non-native isomers can either slow down or entirely halt folding until the native configuration is achieved. However, the impact of non-native isomers on folding is not uniform across all peptide groups. Some might have negligible effects, while others can be more disruptive.
In conclusion, the cis/trans isomerism of the peptide group plays a pivotal role in protein structure and function. Understanding this dynamic is crucial for insights into protein folding, stability, and interactions, which are fundamental to numerous biological processes and potential therapeutic interventions.
Peptide Bond Features
The peptide bond, a pivotal linkage in proteins, exhibits a range of distinctive features that underscore its significance in protein structure and function:
- Writing of the Peptide Bond Structure: Typically, peptide bonds are depicted with free amino acids positioned on the left and the free carboxyl group on the right. The amino acid on the left is termed the N-terminal residue, while the one on the right is referred to as the C-terminal residue. The sequence of amino acids is conventionally read from the N-terminal to the C-terminal, mirroring the direction of protein biosynthesis.
- Representation of the Peptide Bond: A unique representation, often likened to a rattlesnake’s movement, is employed to depict the peptide bond. In this analogy, the N-terminal residues correspond to the rattlesnake’s rattle, while the C-terminal residues are likened to its fangs.
- Shorthand for Peptide Bond: For brevity, the amino acids constituting a peptide or protein are often denoted using a three-letter or a single-letter abbreviation.
- Naming Convention of the Peptide Bond: The nomenclature of peptides necessitates an understanding of amino acid suffixes. For instance, suffixes such as -ine (for glycine), -an (for tryptophan), and -ate (for glutamate) are modified to -yl, with the exception of the C-terminal amino acid.
- Stereochemistry of the Peptide Bond: It is imperative to note that proteins are composed of amino acid units with an L-configuration. This configuration dictates the steric arrangement around the alpha carbon.
- Spectroscopic Characteristics: Peptide bonds exhibit an absorption wavelength ranging between 190–230 nm. Such a spectral signature indicates the bond’s vulnerability to ultraviolet (UV) radiation.
- Reactivity of the Peptide Bond: Owing to its resonance stabilization, the peptide bond is remarkably stable and exhibits minimal reactivity under physiological conditions, even less so than esters. Nonetheless, in certain scenarios, peptide bonds can participate in chemical reactions. These reactions predominantly arise from an attack by an electronegative atom on the carbonyl carbon, leading to the disruption of the carbonyl double bond and culminating in the formation of a tetrahedral intermediate.
In summary, the peptide bond’s multifaceted features underscore its pivotal role in shaping the structure and function of proteins, making it a cornerstone of molecular biology.
What is polypeptide?
A polypeptide is a linear chain of amino acids linked together by peptide bonds. The term “polypeptide” is derived from the Greek words “poly,” meaning many, and “peptos,” meaning digested, reflecting the fact that polypeptides are formed by the polymerization of amino acids.
Key Points:
- Formation: Polypeptides are formed during the process of protein synthesis, where individual amino acids are connected in a specific sequence as dictated by the genetic code. This sequence is determined by the order of codons in a segment of mRNA, which is translated by ribosomes.
- Length: Polypeptides can vary in length, ranging from just a few amino acids long to several thousand. Typically, shorter chains are referred to as peptides, while longer chains are termed proteins, especially if they have a well-defined structure and function.
- Structure: The sequence of amino acids in a polypeptide determines its primary structure. This sequence influences the higher levels of protein structure, including the secondary (e.g., alpha helices and beta sheets), tertiary (three-dimensional folding), and quaternary (assembly of multiple polypeptide chains) structures.
- Function: Once a polypeptide folds into its functional three-dimensional shape, it can serve a wide range of roles in the cell, from catalyzing biochemical reactions as enzymes, to providing structural support, to facilitating communication between cells.
- Diversity: The 20 standard amino acids can be combined in countless sequences, allowing for an immense diversity of polypeptides with unique properties and functions.
In summary, a polypeptide is a chain of amino acids linked by peptide bonds. When folded into specific structures, polypeptides can function as proteins, performing a vast array of tasks essential for life.
Importance of Peptide Bond
The peptide bond is a fundamental chemical linkage that plays a pivotal role in the structure and function of proteins. Its significance in biological systems can be understood through the following aspects:
- Primary Structure of Proteins: Peptide bonds covalently link amino acids in a specific sequence, forming the primary structure of proteins. This sequence determines the protein’s identity and dictates its higher-order structures and functions.
- Stability and Rigidity: The peptide bond’s planar and rigid nature, coupled with its partial double bond character, provides stability to the protein’s structure. This rigidity is essential for maintaining the protein’s shape and ensuring its proper functioning.
- Protein Folding: The orientation and spatial arrangement of peptide bonds influence the way a protein folds, leading to the formation of secondary structures like alpha-helices and beta-sheets. These structures are crucial for the protein’s overall three-dimensional conformation.
- Enzymatic Reactions: The formation and breaking of peptide bonds are catalyzed by specific enzymes. Ribosomes facilitate peptide bond formation during protein synthesis, while proteolytic enzymes, like proteases, catalyze the hydrolysis of peptide bonds during protein degradation.
- Information Storage: The sequence of amino acids, linked by peptide bonds, encodes genetic information. This sequence is a direct translation of the genetic code from mRNA, ensuring the accurate expression of genes into functional proteins.
- Biological Activity: Many biologically active molecules, like hormones and neurotransmitters, are peptides. The specific arrangement of amino acids, connected by peptide bonds, imparts these molecules with their unique functionalities.
- Evolutionary Significance: The universality of the peptide bond in all living organisms suggests its evolutionary importance. It serves as a testament to the shared ancestry of life on Earth.
- Target for Therapeutics: Since peptide bonds are crucial for protein structure and function, they are often targeted in therapeutic interventions. For instance, certain antibiotics work by inhibiting bacterial enzymes responsible for peptide bond formation, thereby disrupting protein synthesis.
In conclusion, the peptide bond is not merely a chemical linkage but is central to the myriad processes that sustain life. Its role in determining protein structure and function underscores its paramount importance in biology and medicine.
Peptide Bond Examples
- Insulin: Insulin is a hormone essential for regulating blood sugar levels. It’s a protein made up of two peptide chains, the A and B chains, linked together by disulfide bonds. The individual amino acids within these chains are connected by peptide bonds.
- Oxytocin: This is a peptide hormone and neuropeptide that plays a role in social bonding, reproduction, and childbirth. It consists of nine amino acids linked together by peptide bonds.
- Hemoglobin: Found in red blood cells, hemoglobin is a protein responsible for transporting oxygen throughout the body. It’s made up of four polypeptide chains (two alpha and two beta chains), and within these chains, amino acids are connected by peptide bonds.
- Enzymes: Almost all enzymes are proteins, and they catalyze various biochemical reactions in the body. For instance, the enzyme pepsin breaks down dietary proteins in the stomach. The structure of pepsin, like other enzymes, is maintained by peptide bonds linking its constituent amino acids.
- Antibodies: These are proteins produced by the immune system to neutralize pathogens like bacteria and viruses. Each antibody is made up of two heavy and two light polypeptide chains, with peptide bonds holding the amino acids of these chains together.
- Collagen: This is the most abundant protein in the human body, providing structural support to connective tissues, skin, and bones. It’s a triple helix structure made up of three polypeptide chains, with peptide bonds connecting the amino acids within each chain.
- Dipeptides and Tripeptides: These are the simplest examples. A dipeptide is formed when two amino acids are linked by a single peptide bond, while a tripeptide is formed from three amino acids linked by two peptide bonds. For instance, carnosine is a dipeptide made up of the amino acids beta-alanine and histidine.
These examples underscore the ubiquity and importance of peptide bonds in the structure and function of proteins across various biological systems.
FAQ
What is a peptide bond?
A peptide bond is a covalent bond that forms between the carboxyl group of one amino acid and the amino group of another amino acid.
Are peptide bonds covalent?
Yes, peptide bonds are covalent bonds.
Description with Example:
A covalent bond is formed when two atoms share electrons. In the context of peptide bonds, this covalent linkage occurs between the carboxyl group (-COOH) of one amino acid and the amino group (-NH2) of another amino acid.
Example: Consider two amino acids, Glycine and Alanine:
Glycine has the structure: H2N-CH2-COOH Alanine has the structure: H2N-CH(CH3)-COOH
When these two amino acids form a peptide bond, the hydroxyl group (-OH) from the carboxyl group of Glycine and a hydrogen atom from the amino group of Alanine are removed, producing a water molecule (H2O). The remaining parts of the two amino acids then bond covalently, forming the peptide bond:
H2N-CH2-C(=O)NH-CH(CH3)-COOH
In this structure, the C(=O)NH linkage represents the peptide bond. The bond between the carbon (C) of the carboxyl group and the nitrogen (N) of the amino group is the covalent peptide bond.
How are peptide bonds formed?
Peptide bonds are formed through a dehydration synthesis reaction, where a water molecule is released.
A peptide bond links __________.
A peptide bond links the carboxyl group of one amino acid to the amino group of another amino acid.
Where do peptide bonds form?
Peptide bonds form between amino acids during protein synthesis in ribosomes.
Which is released during the formation of a peptide bond?
A water molecule (H2O) is released during the formation of a peptide bond.
What type of bond is a peptide bond?
A peptide bond is a covalent bond.
Where are peptide bonds found?
Peptide bonds are found in proteins, linking amino acids together.
A long chain of amino acids linked by peptide bonds.
This describes a polypeptide or protein.
Do free amino acids have peptide bonds?
No, free amino acids do not have peptide bonds. Peptide bonds form when amino acids are linked together.
What purpose does the peptide bond serve in protein synthesis?
The peptide bond holds amino acids together, forming the primary structure of proteins.
When a peptide bond is created between two amino acids.
This occurs during protein synthesis when the carboxyl group of one amino acid reacts with the amino group of another, releasing a water molecule.
What do peptide bonds hold together?
Peptide bonds hold amino acids together in a protein.
How many peptide bonds are in a tripeptide?
There are two peptide bonds in a tripeptide.
Where are the peptide bonds located in a polypeptide?
Peptide bonds are located between each pair of adjacent amino acids in a polypeptide.
Which describes the function of a peptide bond?
The peptide bond links amino acids together to form the primary structure of proteins.
Are peptide bonds polar?
The peptide bond itself has a partial double bond character, making it relatively nonpolar. However, the surrounding regions can have polar characteristics depending on the amino acids involved.
How to identify peptide bonds?
Peptide bonds can be identified in a protein structure as the bonds connecting the carboxyl group of one amino acid to the amino group of another.
Where does cleavage of the peptide bond by chymotrypsin occur?
Chymotrypsin cleaves peptide bonds at the carboxyl side of aromatic or large hydrophobic amino acids like tryptophan, tyrosine, phenylalanine, and sometimes methionine.
Which of the following pancreatic enzymes acts on peptide bonds?
Without a specific list of enzymes, I cannot determine which act on peptide bonds. However, enzymes like trypsin and chymotrypsin are pancreatic enzymes that act on peptide bonds.
Why are peptide bonds planar?
Peptide bonds are planar because of the partial double bond character, which restricts rotation around the bond.
A peptide bond forms between which of these groups?
A peptide bond forms between the carboxyl group of one amino acid and the amino group of another amino acid.
Can a peptide bond rotate freely?
No, due to its partial double bond character, a peptide bond cannot rotate freely.
Do free amino acids peptide bonds?
No, free amino acids do not have peptide bonds.
Do proteins have peptide bonds?
Yes, proteins are made up of amino acids linked by peptide bonds.
How are peptide bonds broken?
Peptide bonds are broken through hydrolysis, where a water molecule is added.
How do amino acids form peptide bonds?
Amino acids form peptide bonds through a dehydration synthesis reaction, where a water molecule is released.
Is a peptide bond a hydrogen bond?
No, a peptide bond is a covalent bond.
What are peptide bonds made of?
Peptide bonds are made of the atoms that connect the carboxyl group of one amino acid to the amino group of another amino acid.
What catalyzes peptide bond formation?
Ribosomes, along with transfer RNA (tRNA) molecules, catalyze peptide bond formation during protein synthesis.
What type of bond joins amino acids to make peptides?
Peptide bonds join amino acids to make peptides.
Which bond represents the peptide bond?
The bond between the carboxyl group of one amino acid and the amino group of another represents the peptide bond.
Which of the following polysaccharides contains peptide bonds?
Without a specific list of polysaccharides, I cannot determine which contains peptide bonds. However, typically, polysaccharides do not contain peptide bonds as they are carbohydrates.
Are peptide and amide bonds the same?
Yes, a peptide bond is a type of amide bond that forms between amino acids.
Are peptide bonds charged?
No, peptide bonds themselves are not charged.
Are peptide bonds covalent or ionic?
Peptide bonds are covalent bonds.
Are peptide bonds flexible?
The peptide bond itself is not flexible due to its partial double bond character, but the bonds around it can rotate, allowing flexibility in the protein structure.
Are peptide bonds hydrophobic?
The peptide bond itself is neutral, but the surrounding regions can be hydrophobic or hydrophilic depending on the amino acids involved.
Are peptide bonds intramolecular or intermolecular attraction?
Peptide bonds are intramolecular covalent bonds that connect amino acids within a molecule.
Are peptide bonds only found in proteins?
Primarily, yes. Peptide bonds are most commonly found in proteins, but they can also be found in some peptides and polypeptides.
Are there peptide bonds in tertiary structure?
The tertiary structure of a protein refers to its overall three-dimensional shape. While the tertiary structure is stabilized by various interactions, including hydrogen bonds, ionic bonds, and hydrophobic interactions, the peptide bonds themselves are part of the primary structure, linking amino acids together.
Can proline form peptide bonds?
Yes, proline can form peptide bonds. However, its unique ring structure can introduce kinks or bends in the protein chain.
Do all proteins have peptide bonds?
Yes, all proteins are made up of amino acids linked by peptide bonds.
Do carbohydrates contain peptide bonds?
No, carbohydrates do not contain peptide bonds. Carbohydrates are made up of sugar molecules linked by glycosidic bonds.
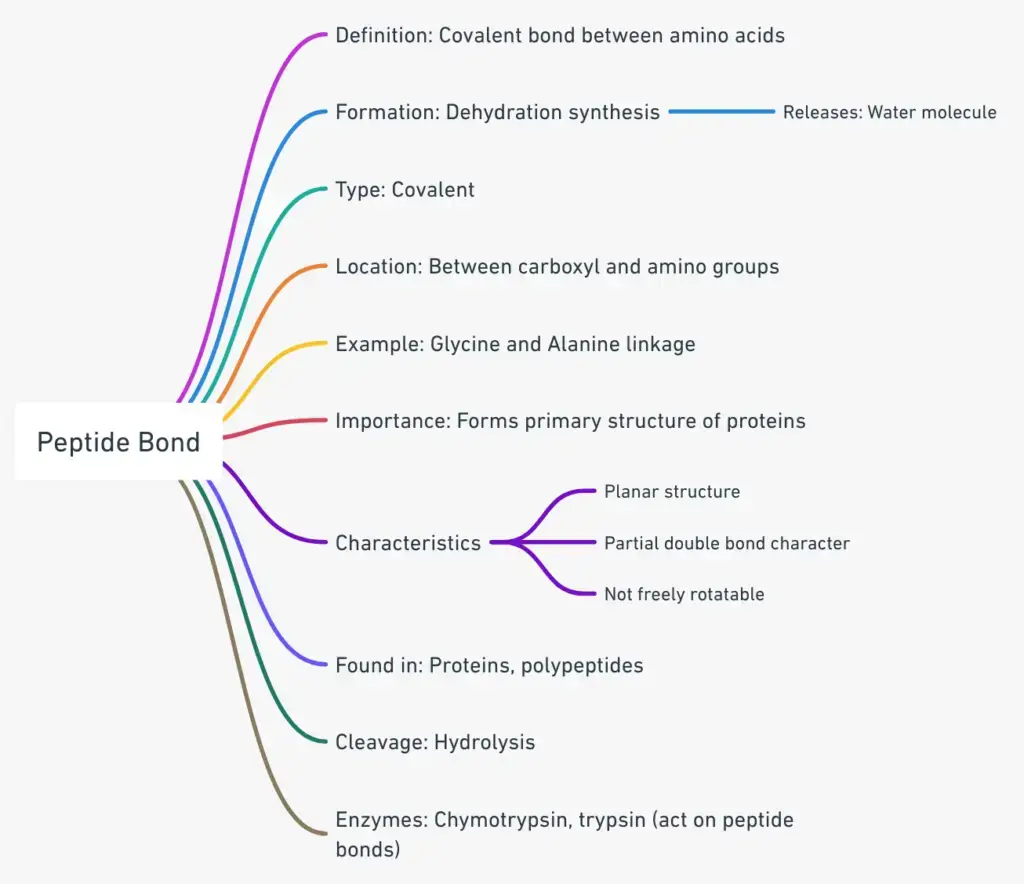
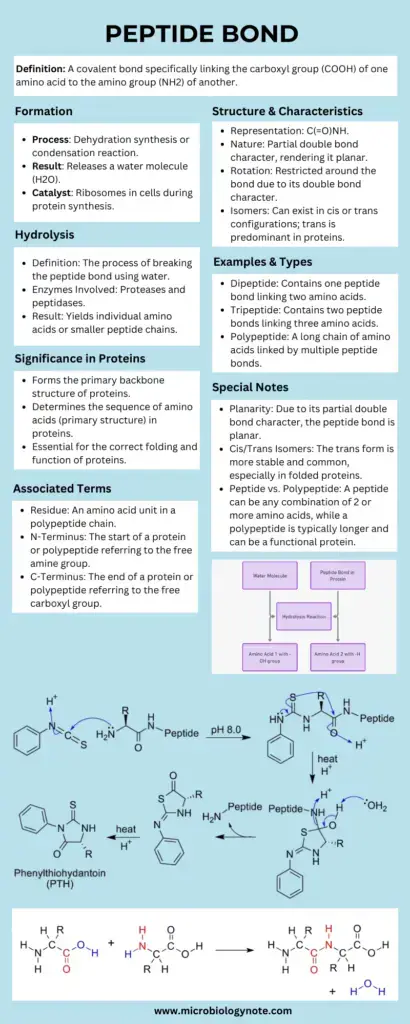
References
- https://biologydictionary.net/peptide-bond/
- https://www.chemistrylearner.com/chemical-bonds/peptide-bond
- https://www.biologyonline.com/dictionary/peptide-bond
- https://www.sciencedirect.com/topics/pharmacology-toxicology-and-pharmaceutical-science/peptide-bond
- https://www.sciencedirect.com/topics/nursing-and-health-professions/peptide-bond
- https://pubs.acs.org/doi/10.1021/jacs.1c02600
- https://www.khanacademy.org/test-prep/mcat/biomolecules/amino-acids-and-proteins1/v/peptide-bond-formation-and-cleavage
- https://www.britannica.com/science/peptide-bond
- https://www.cryst.bbk.ac.uk/PPS95/course/3_geometry/peptide2.html
- https://www2.chem.wisc.edu/deptfiles/genchem/netorial/modules/biomolecules/modules/protein1/prot15.htm
- https://www.nature.com/scitable/topicpage/protein-structure-14122136/
- https://unacademy.com/content/upsc/study-material/chemistry/a-brief-note-on-peptide-bond-formation-or-synthesis/