Antigen-Antibody (Ag-Ab) Interaction refers to the biochemical reaction that occurs between antibodies and specific antigens when they come into close proximity, typically within a distance of several nanometers. This interaction plays a critical role in the immune response against foreign substances and pathogens in the body.
The binding of antibodies to specific antigens is facilitated by the complementary regions on their respective molecules. The regions on the antibody that recognize and bind to antigens are called paratopes, while the corresponding regions on antigens are known as epitopes. When the paratopes of antibodies come into contact with the epitopes of antigens, a series of immunological responses are initiated to counteract the antigens and eliminate them from the body.
The interaction between antigens and antibodies can be represented by the following equation:
Antigen + Antibody ⇄ Ag-Ab complex → Immune Response
This equation illustrates that the antigen and antibody can reversibly bind to form an Ag-Ab complex, which ultimately triggers an immune response against the antigen.

The law of mass action governs the kinetics of this interaction. It states that the rate of the association constant (Ka), which represents the binding of the antigen and antibody, is proportional to the concentrations of the reactants. On the other hand, the rate of the dissociation constant (Kd), which represents the separation of the antigen and antibody, is proportional to the concentration of the Ag-Ab complex.
At equilibrium, the ratio of the concentration of the Ag-Ab complex to the concentrations of the antigen and antibody is constant, and this ratio is known as the equivalence constant (Keq). The equilibrium state signifies that the forward and reverse reactions occur at an equal rate, leading to a stable complex or dissociation, depending on the conditions.
The antigen-antibody interaction is a fundamental process underlying various immunological phenomena, including immune defense against pathogens, antibody-mediated neutralization, and diagnostic techniques such as immunoassays. Understanding the mechanisms and dynamics of antigen-antibody interactions is crucial for advancing our knowledge of immunology and developing therapeutic strategies in medicine.
What is Antigen (Ag)?
- An antigen (Ag), derived from the terms “anti” (against) and “gen” (thing that produces or causes), refers to any foreign substance that enters the body and has the potential to induce an immune response. Antigens can elicit a range of immune responses, leading to the activation of the immune system and the production of specific antibodies.
- Immunogens are a specific type of antigen that directly elicit an immune response upon entering the body. These substances are capable of stimulating the immune system independently and can trigger the production of antibodies or activate immune cells. Immunogens can include various types of molecules such as proteins, peptides, lipids, or polysaccharides.
- In contrast, haptens are substances that are not capable of eliciting an immune response on their own. Instead, they require the assistance of other molecules, known as carrier proteins, to become immunogenic. Once a hapten binds to a carrier protein, the resulting complex can stimulate the immune system and induce an immune response.
- Collectively, both immunogens and haptens are referred to as antigens. They play a crucial role in the immune system’s recognition of foreign substances and the subsequent activation of the appropriate immune responses.
- When an antigen interacts with the immune system, specific regions on the antigen, known as epitopes, bind to complementary regions on antibodies. These binding sites on antibodies are responsible for recognizing and binding to specific epitopes on antigens. The interaction between epitopes and antibodies is essential for the immune system to mount an effective response against the antigen.
- In scientific notation, the abbreviation “Ag” is commonly used to represent antigens. This abbreviation helps to identify and differentiate antigens from other biological molecules during discussions and scientific research.
- Understanding the nature and characteristics of antigens is crucial in various fields, including immunology, vaccine development, diagnostics, and biomedical research. By studying antigens and their interactions with the immune system, scientists can gain insights into immune responses, develop targeted therapies, and enhance our understanding of diseases and immune-related disorders.
What is Antibody (Ab)?
- Antibodies have a Y-shaped structure. This structure consists of two identical heavy chains and two identical light chains held together by disulfide bonds. The Y shape allows antibodies to bind to antigens and form antigen-antibody complexes.
- Antibodies are glycoproteins, meaning they are proteins with attached carbohydrate molecules. These carbohydrates can influence the function and stability of the antibody.
- Plasma B-cells are responsible for producing antibodies. B-cells are a type of white blood cell that undergoes differentiation and maturation to become plasma cells, which are specialized in antibody production.
- The region of an antibody that recognizes and binds to antigens is called the paratope. The paratope is complementary to the epitope, which is the specific region on the antigen that the antibody recognizes and binds to. The binding of the paratope to the epitope is highly specific, enabling antibodies to selectively target and interact with particular antigens.
- There are different types of antibodies, known as immunoglobulin classes or isotypes. The five main classes of immunoglobulins are IgG, IgA, IgM, IgE, and IgD. These classes have distinct functions and are involved in different aspects of the immune response. To remember these classes, the mnemonic “GAMED” can be used.
- IgG antibodies are the most abundant in the bloodstream and are involved in long-term immune protection.
- IgA antibodies are primarily found in bodily secretions such as saliva, tears, and mucous membranes, providing localized protection.
- IgM antibodies are the first antibodies produced during an initial immune response and are involved in early defense against pathogens.
- IgE antibodies are associated with allergic reactions and immune responses against parasites.
- IgD antibodies have a role in the activation and regulation of the immune system, but their functions are not yet fully understood.
Understanding the properties and functions of antibodies is essential for studying the immune system, developing vaccines, and diagnosing and treating various diseases. The specific interactions between antigens and antibodies are at the core of many immunological processes and are central to our understanding of immunity.
What is an Antigen-Antibody Interaction?
- Antigen-antibody interaction, also referred to as antigen-antibody reactions, describes the specific interactions that occur between antigens and antibodies. These interactions play a crucial role in the immune response and are characterized by their specificity and affinity.
- Antibodies are produced by the immune system in response to the presence of antigens. Antigens are recognized by antibodies based on their molecular shapes, specifically the epitopes. Epitopes are specific regions on antigens that antibodies bind to.
- The interaction between antigens and antibodies is highly specific, meaning that an antigen will typically only react with antibodies that are produced against itself or closely related antigens. This specificity ensures that the immune response is targeted and directed towards the invading antigen.
- The quality of the fit between the epitope and the combining site of the antibody is crucial in determining the strength of the antigen-antibody interaction. Factors such as the geometry and chemical characteristics of the epitope and the antibody combining site contribute to the strength of the interaction. A better fit between the epitope and the combining site leads to more favorable interactions and results in higher affinity, meaning the antibody has a stronger binding to the antigen.
- Affinity refers to the strength of the binding between an antibody and its corresponding antigen. It is one of the most important factors in determining the efficacy of the antibody in vivo, meaning its effectiveness in the body. Antibodies with high affinity for their target antigens are more effective at neutralizing or eliminating the antigens, leading to a more robust immune response.
- The antigen-antibody interaction involves a bimolecular irreversible association between the antigen and antibody. This association is facilitated by various non-covalent interactions between the epitope (also called antigenic determinant) and the variable region (VH/VL) domain of the antibody. These non-covalent interactions include hydrogen bonding, electrostatic interactions, van der Waals forces, and hydrophobic interactions.
- Understanding the mechanisms and dynamics of antigen-antibody interactions is essential in immunology, as it forms the basis for various immunological processes such as immune defense, immunotherapy, and diagnostic techniques like immunoassays. The specific and high-affinity interactions between antigens and antibodies are key components of the immune system’s ability to recognize and eliminate harmful substances, contributing to our overall health and well-being.
Strength of Ag-Ab interactions
Similar to how proteins bind to their biological receptors or enzymes bind to their substrates, antigen and antibody bind through noncovalent linkages. In contrast, antigen–antibody reactions do not result in irreversible chemical changes in either participant, i.e. the antigen or the antibody. The interaction between antigen and antibody is reversible and can be blocked or disrupted by a strong ionic force or an extreme pH. The following are some general characteristics of these interactions:
Physicochemical Properties
- Antigen–antibody interactions involve electrostatic connections, hydrogen bonds, van der Waals bonds, and hydrophobic interactions as intermolecular forces.
- All of these intermolecular pressures are dependent upon the close proximity of antigen and antibody molecules. Determining the stability of the antigen–antibody reaction, therefore, is the “excellent match” between an antigenic determinant and an antibody-combining site.
- Multiple interactions between the antigen and antibody guarantee that the antigen is securely bound to the antibodies.
Affinity
- Affinity denotes the intensity of antigen and antibody attraction.
- Low-affinity antibodies bind antigen poorly and tend to dissociate quickly, whereas high-affinity antibodies bind antigen more strongly and remain bound for a longer period of time.
- It is hypothesised that a highly close match between the antigen-binding sites and the corresponding antigenic determinants favours the formation of strong noncovalent interactions between antigen and antibody, resulting in high-affinity binding.
Affinity quantifies the strength of contact between an epitope and the antigen-binding site of an antibody. It is defined by the same fundamental thermodynamic rules that govern any biomolecular interaction that is reversible:
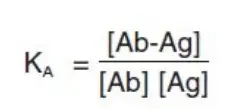
- KA = affinity constant
- [Ab] = molar concentration of unoccupied binding sites on the antibody
- [Ag] = molar concentration of unoccupied binding sites on the antigen
- [Ab-Ag] = molar concentration of the antibody-antigen complex.
In other words, KA specifies the amount of antibody-antigen complex that occurs at equilibrium. The time required for this to occur is proportional to the diffusion rate and is the same for every antibody. However, high-affinity antibodies bind more antigen in less time than low-affinity antibodies. Therefore, KA can range from below 105 mol-1 to above 1012 mol-1 for antibodies, and can be affected by variables such as pH, temperature, and buffer composition.
- The overall strength of non-covalent interactions between a single Ag- binding site on an antibody and a single epitope is the affinity of the antibody for that epitope.
- Low affinity Ab: poorly bind Ag and rapidly dissociate.
- High affinity Ab: Bind Ag tightly and remain bound for an extended period of time.
Avidity
- Avidity is a measure of the overall binding strength of multiple antigenic determinants and multivalent antibodies to an antigen.
- In actual biological systems, avidity is a better predictor of the strength of interactions than affinity.
- Consequently, the avidity of an antigen–antibody response depends on the valencies of both antigens and antibodies and is greater than the sum of the individual affinities.
- Avidity is influenced by three variables;
- The binding affinity: The binding affinity is the strength of the connection between two molecules at a single binding site.
- The valency: The total number of involved binding sites.
- The structural arrangement: the antigen and antibody structures involved.
- All antibodies are multivalent e.g. IgMs are decavalent while IgGs are bivalent. The greater the valency (number of antigen binding sites) of an immunoglobulin, the more antigen it can bind. Antigens can also exhibit multivalency since they can bind to several antibodies. The stability of an antibody and antigen is facilitated by their multimeric interactions.
- Additionally, a favourable structural arrangement between the antibody and antigen can result in a more stable antibody-antigen complex.
- Avidity is the strength of numerous interactions between multivalent Ab and Ag. Avidity is a more accurate indicator of the binding potential of an antibody than affinity. A high avidity might make up for a poor affinity.
Specificity
- Specificity refers to the ability of an antibody-combining site to react with a single antigenic determinant or the ability of a population of antibody molecules to respond with a single antigen.
- Typically, antigen–antibody responses have a high degree of specificity.
- Despite this, cross-reactions between antigens and antibodies sometimes occur and are occasionally responsible for producing diseases in hosts and generating erroneous diagnostic test findings.
- Antibodies can recognise specific distinctions in:
- primary structure of an antigen.
- isomeric forms of an antigen.
- secondary and tertiary structure of an antigen.
Cross-Reactivity
- Although antigen–antibody responses are very specific, in some instances an antibody produced by an unrelated antigen might cross-react.
- This occurs when two distinct antigens share an identical or highly similar epitope.
- In the latter instance, the affinity of the antibody for the cross-reacting epitope is typically lower than its affinity for the original epitope.
- Antisera containing polyclonal antibodies frequently cross-react with immunogens somewhat related to those used for immunisation, as a result of the existence of common epitopes or epitopes with comparable conformations.
Antigen-Antibody Interaction Properties
Antigen-antibody interaction possesses several distinct properties that contribute to its essential role in the immune response. These properties include:
- Highly specific reaction: Antigen-antibody interactions exhibit a high degree of specificity. Antibodies recognize and bind to specific epitopes on antigens, resulting in a targeted immune response against particular foreign substances.
- Observable interaction: The binding of antigens and antibodies can be observed through various techniques, such as immunoassays or microscopy, allowing for the detection and analysis of these interactions.
- Non-covalent interactions: Antigen-antibody interactions are mediated by non-covalent forces, including van der Waals forces, ionic bonds, hydrogen bonds, and hydrophobic interactions. These weak but specific interactions enable reversible binding between antigens and antibodies.
- No denaturation of antibodies and antigens: The binding of antigens and antibodies does not result in denaturation or irreversible structural changes in either molecule. This allows for the dissociation of the antigen-antibody complex under appropriate conditions.
- Reversibility: Antigen-antibody interactions are reversible, meaning that the complex formed between the antigen and antibody can dissociate back into separate entities. This reversibility is important for the flexibility and adaptability of the immune response.
- Affinity: Affinity refers to the strength with which an antigen binds to a single antigen-binding site on an antibody. It is a measure of the specificity and tightness of the interaction between a particular antigen and antibody.
- Avidity: Avidity is a broader concept than affinity and represents the overall strength of the antigen-antibody complex. It takes into account factors such as the affinity of the antibody, the valency (number of binding sites) of both the antibody and antigen, and the structural arrangement of epitopes and paratopes.
- Cross-reactivity: Cross-reactivity refers to the ability of an antibody to bind to similar epitopes on different antigens. This property allows antibodies to recognize and respond to structurally related antigens, even if they were initially generated against a different antigen.
Antigen-Antibody Interaction/Reaction Stages
There are two stages to the antigen–antibody reaction: primary and secondary.
1. Primary Stage
- The initial contact between antigen and antibody is known as the primary stage. It is quick and reversible, but its consequences are invisible.
- The ionic bonds, hydrogen bonds, van der Waals forces, and hydrophobic interactions are the weaker intermolecular forces that bind antigen and antibodies together in this primary stage.
- However, covalent binding, a stronger intermolecular force between antigen and antibody, does not occur at this stage.
2. Secondary Stage
- The secondary stage involves an irreversible interaction between antigen and antibody, accompanied by observable results such as agglutination, precipitation, neutralisation, complement fixation, and immobilisation of motile organisms.
- Covalent binding occurs between the antigen and antibody during this step.
- A single antibody is capable of producing a variety of antigen–antibody interactions, and a single antigen is capable of stimulating the creation of immunoglobulin classes with distinct biological features.
- Generally, titers are used to express the outcomes of agglutination, precipitation, neutralisation, and other tests.
- Titer is defined as the highest serum dilution that produces a positive test result. Higher titer indicates a higher concentration of antibodies in serum. A serum with a titer of 1/128 has more antibodies than one with a titer of 1/8, for example.
Factors Affecting Antigen-Antibody Interaction/Reaction
Several factors influence the antigen-antibody interaction, affecting the strength and specificity of the reaction. Some common factors include:
- Temperature: The temperature at which the antigen-antibody reaction takes place can impact the stability and kinetics of the interaction. Different bonds involved in the reaction, such as hydrogen bonds and hydrophobic bonds, have varying temperature sensitivities. For example, hydrogen bonds are more stable at lower temperatures, while hydrophobic interactions are favored at higher temperatures.
- pH: The pH of the environment can affect the conformation of antibodies and antigens, influencing their binding capability. The optimal pH range for most antigen-antibody reactions is between 6.5 and 8.5. Extreme pH values can disrupt the conformational integrity of antibodies and inhibit the interaction.
- Ionic strength: The presence of ions, particularly sodium (Na+) and chloride (Cl-) ions, can impact antigen-antibody interactions. In blood group serology, the ionic strength of the solution can significantly influence the reaction. Sodium and chloride ions present in normal saline solution can cluster around the antigen-antibody complex, partially neutralizing charges and potentially interfering with antibody binding to the antigen.
- Enzyme treatment: Proteolytic enzymes, such as papain, ficin, and bromelain, can be used to enhance antigen-antibody reactions. These enzymes can cleave specific regions of antibodies, resulting in improved binding capabilities or fragment production with altered binding properties.
- Concentrations of antigen and antibody: Increasing the concentrations of both the antigen and antibody can enhance the likelihood and strength of the antigen-antibody interaction. Higher concentrations provide more opportunities for the binding of antibodies to antigens.
- Number of antigen-binding sites: The number of antigen-binding sites on an antibody can impact the efficiency of the interaction. Antibodies with multiple binding sites, such as IgM (pentamer with ten binding sites), have a higher chance of interacting with antigens compared to antibodies with fewer binding sites, such as IgG (monomer with two binding sites).
- Structural arrangement: The structural compatibility between the epitope on the antigen and the paratope on the antibody is crucial for a strong interaction. If the epitope and paratope have complementary shapes, akin to a lock and key, it enhances the binding efficiency between the antigen and antibody.
Considering these factors is important for optimizing antigen-antibody reactions in various applications, including diagnostic tests, immunological research, and therapeutic interventions. Understanding how these factors influence the interaction can help in designing experiments, controlling reaction conditions, and ensuring accurate and reliable results.
Chemical Bonds Responsible for the Interaction/Reaction
The antigen-antibody interaction is mediated by various types of non-covalent chemical bonds. These bonds play a crucial role in the binding between the antigen and the antibody, allowing for specificity and reversible interaction. The following are the main chemical bonds responsible for the antigen-antibody reaction:
- Electrostatic bonds: Electrostatic bonds arise from the attraction between oppositely charged ionic groups of two protein side chains. For example, an ionized amino group (NH4+) on lysine in the antibody can interact with an ionized carboxyl group (COO-) on an aspartate residue in the antigen.
- Hydrogen bonding: Hydrogen bonds can form between specific hydrophilic groups in close proximity when the antigen and antibody interact. Hydrogen bonding occurs between groups such as hydroxyl (OH) and carbonyl (C=O), amino (NH) and carbonyl (C=O), and amino (NH) and hydroxyl (OH) groups.
- Hydrophobic interactions: Hydrophobic interactions play a significant role in antigen-antibody binding. Hydrophobic groups, such as the side chains of amino acids like valine, leucine, and phenylalanine, tend to associate with each other. These interactions result in the exclusion of water molecules from their vicinity and contribute to the overall strength of the antigen-antibody bond. Hydrophobic interactions can account for up to 50% of the total strength of the bond.
- Van der Waals bonds: Van der Waals forces are weak attractive forces that arise from interactions between the electron clouds surrounding the antigen and antibody molecules. These forces depend on the fluctuations in electron density and can be likened to interactions between alternating dipoles in two molecules. The close apposition of oppositely oriented dipoles in the antigen and antibody allows for these attractive forces to contribute to the antigen-antibody bond.
These non-covalent interactions operate over very short distances, typically around 1 Å (angstrom). As a result, the antigen-antibody interaction relies on a close fit between the antigen and the antibody, where the binding site of the antibody (paratope) aligns precisely with the molecular shape of the antigen (epitope). The strength and specificity of the antigen-antibody bond are determined by the combined effects of these non-covalent chemical bonds, ensuring a selective and reversible interaction between the two molecules.
Types of Antigen-Antibody Interaction/Reaction
Serological tests are commonly used to identify serum antibodies or antigens in order to diagnose a vast array of infectious disorders. These serological tests are also utilised for the identification of autoimmune illnesses as well as the typing of blood and tissues prior to transplantation. Here are some instances of antigen–antibody reactions:
- Precipitation
- Agglutination
- complement-dependent serological tests
- neutralization test
- Opsonization
- Immunofluorescence
- enzyme immunoassay
- Radioimmunoassay
- Western blotting
- chemiluminescence assay
- immunoelectronmicroscopic tests.
1. Precipitation Reaction
- It is a type of antigen–antibody response wherein the antigen is soluble.
- It is a test in which antibody reacts with soluble antigen in the presence of electrolyte at a predetermined pH and temperature to form a precipitate. Antigens and antibodies form a lattice; in certain instances, it is apparent as an insoluble precipitate.
- Precipitins are antibodies that aggregate soluble antigens. When precipitate remains suspended as floccules rather than settling, the process is known as flocculation.
- Formation of an antigen–antibody lattice is dependent on both the valency of the antigen and the antibody.
- The antibody must be bivalent; monovalent Fab fragments will not create a precipitate.
- The antigen must be either bivalent or polyvalent, with at least two copies of the same epitope or various epitopes that respond with different antibodies present in polyclonal antisera.
Types of precipitation reactions
Precipitation reactions often fall into three categories:
- Precipitation in solution: Precipitation in solution is illustrated by the ring and flocculation tests.
- Agar precipitation: The agar gel precipitation test is known as the immunodiffusion test. In this test, reactants are introduced to the gel, and the antigen–antibody combination takes place through diffusion. The rate of diffusion is influenced by the particle size, temperature, gel viscosity, quantity of hydration, and matrix-reactant interactions. Immunodiffusion reactions are characterised according to (a) the number of diffusing reactants and (b) the direction of diffusion:
- Single diffusion in one dimension
- Single diffusion in two dimensions
- Double diffusion in two dimension
- Double diffusion in one dimension
- Precipitation in agar with an electric field: Examples of Precipitation in agar using an electric field include Immunoelectrophoresis, Counter-current immunoelectrophoresis, Rocket electrophoresis, Two-dimensional immunoelectrophoresis, Turbidimetry, and nephelometry.
Application of Precipitation reaction
- Detection of an unknown antibody to diagnose an infection, for instance. The VDRL test detects syphilis.
- Toxins and antitoxins should be standardised.
- Identifying Bacteria, such as E. coli Clusters of streptococci that have been calcified
- Identification of bacterial component, such as the thermoprecipitin test for Bacillus anthracis developed by Ascoli.
2. Agglutination Reaction
- The interaction between an antibody and a particle antigen results in a phenomenon known as agglutination.
- Antibodies that induce such responses are known as agglutinins. IgM antibody agglutination is superior to IgG antibody agglutination.
- Antibody excess also reduces the agglutination response; this inhibition is known as the prozone phenomenon.
- Antibody detection is more sensitive via agglutination than precipitation.
- Optimal agglutination occurs when antigens and antibodies react in quantities that are equal.
- When an antibody or antigen is overproduced, the prozone phenomenon may be observed. Although incomplete or monovalent antibodies bind with the antigen, they do not generate agglutination.
- They may function as blocking antibodies, inhibiting agglutination by the subsequent addition of the full antibody.
Types of agglutination
Agglutination is divided into 3 groups such as;
- Direct agglutination: Direct agglutination can be of the following types: (a) slide agglutination, (b) tube agglutination, (c) heterophile agglutination, and (d) antiglobulin (Coombs’) test.
- Passive agglutination: Passive agglutination utilises antigen-coated carrier particles. This is typically done to transform precipitation reactions into agglutination reactions, as the latter are simpler to run and understand and more sensitive for detecting antibodies than precipitation reactions.
- Reverse passive agglutination: Reverse passive agglutination refers to the detection of antigens by adsorption of the antibody instead of antigens on the carrier particle.
Application of Agglutination reaction
- Cross-matching and blood grouping
- Characterization of Bacteria Serotyping of Vibrio cholera, Salmonella Typhi, and Paratyphi, for example.
- Diagnosis of numerous diseases using serology. Example: Rapid plasma regains (RPR) and Antistreptolysin O (ASO) tests for syphilis and rheumatic fever, respectively.
- Detection of an unidentified antigen in clinical specimens. Detection of the Vi antigen of Salmonella Typhi in the urine, for instance.
3. Complement-Dependent Serological Tests
- Normal serum contains a set of serum proteins known as the complement system. Twenty or more serum proteins interact with one another and the cell membrane to form the system.
- It is a metabolic cascade that helps rid the body of infections.
- It assists antibodies in lysing germs, stimulating phagocytosis, and increasing immunological adhesion.
Types of Complement-Dependent Serological Tests
The following types of complement-dependent serological assays are possible: 1. Complement fixation test 2. Immune adherence test 3. Immobilization test 4. Cytolytic or cell-killing reactions
a. Complement fixation test
- The complement fixation test is based on the premise that when antigen and IgM or IgG antibodies are combined, complement is “fixed” to the antigen–antibody combination.
- If this occurs on the surface of RBCs, the complement cascade will be activated, resulting in hemolysis.
- Two antigen–antibody complement systems comprise the complement fixation test: (a) an indicator system and (b) a test system.
b. Immune adherence test
- Certain infections (e.g., Vibrio cholerae, Treponema pallidum, etc.) respond with certain antibodies in the presence of complement and adhere to erythrocytes or platelets during an immune adherence test.
- Immune adherence is the adhesion of cells to bacteria, which aids the phagocytosis of germs.
c. Immobilization test
- Certain live bacteria, such as T. pallidum, are immobilised when mixed with a patient’s serum in the presence of complement in an immobilisation test dependent on complement.
- This is the basis for the immobilisation test for T. pallidum. A positive result indicates that the serum contains treponemal antibodies.
d. Cytolytic or cytocidal reactions
- When a live bacteria such as V. cholerae is combined with its particular antibody in the presence of complement, it is destroyed and lysed.
- This is the basis of the test used to assess serum anti-cholera antibodies.
Application of Complement-Dependent Serological Tests
Historically, complement fixation reactions were used to diagnose numerous illnesses, including:
- The Wassermann test is used to detect syphilis.
- Antibody screenings against M. smegmatis pneumoniae, Bordetella pertussis, numerous other viruses, and to fungus (such as Cryptococcus spp., Histoplasma, and Coccidioides immitis). This test is no longer administered since it is technically very complicated and frequently difficult.
4. Neutralization Tests
- Neutralization is an antigen–antibody response in which homologous antibodies known as neutralising antibodies negate the biological effects of viruses and poisons.
Types of Neutralization Tests
Generally speaking, these tests fall into two categories: (a) viral neutralisation tests and (b) toxin neutralisation testing.
a. Virus neutralization tests
- Testing for the neutralisation of viruses by their specific antibodies is known as virus neutralisation. Inoculation of viruses into cell cultures, eggs, and animals leads to viral replication and proliferation.
- Injecting virus-specific neutralising antibodies into these systems inhibits viral replication and proliferation.
- This is the foundation for the virus neutralisation test. The viral hemagglutination inhibition test is an example of a virus neutralisation test commonly used to diagnose viral diseases like influenza, measles, and mumps.
- If the patient’s serum includes antibodies against particular viruses that have the property of agglutinating red blood cells, these antibodies will bind to the viruses and prevent agglutination of the red blood cells.
b. Toxin neutralization tests
Toxin neutralisation tests are based on the premise that the biological effect of a toxin can be negated by specialised neutralising antibodies known as antitoxins. The following are examples of neutralisation tests:
- In vivo—(a) Schick test to demonstrate immunity against diphtheria and (b) Clostridium welchii toxin neutralization test in guinea pig or mice.
- In vitro—(a) antistreptolysin O test and (b) Nagler reaction used for rapid detection of C. welchii.
5. Opsonization
- By combining with opsonin, a particulate antigen becomes more vulnerable to phagocytosis through the process of opsonization.
- The opsonin is a heat-labile substance found in normal fresh serum.
- Unlike opsonin, bacteriotropin is a heat-stable serum constituent with similar functions to opsonin.
- The definition of the word “opsonic index” is the ratio of the phagocytic activity of a patient’s blood for a certain bacterium to the phagocytic activity of blood from a healthy individual.
- It is used to examine the progression of resistance during the disease’s progression. It is determined by incubating fresh citrated blood with a bacterial suspension at 37 °C for 15 minutes and calculating the average number of phagocytic bacteria from stained blood films.
6. Immunofluorescence
- Fluorescence is the property of some dyes to absorb light rays at a specific wavelength (ultraviolet light) and emit them at an other wavelength (visible light).
- Antibody molecules can be attached to fluorescent dyes such fluorescein isothiocyanate and lissamine rhodamine.
- Under ultraviolet (UV) rays, they emit blue-green and orange-red fluorescence, respectively, in the fluorescence microscope.
- This is the basis for the immunological examination. Immunofluorescence tests have numerous diagnostic and research applications.
Types of Immunofluorescence
These examinations often fall into two categories: 1. Test of direct immunofluorescence 2. Test by indirect immunofluorescence
a. Direct immunofluorescence test
- Utilizing a tagged antibody that interacts directly with the unknown antigen, a direct immunofluorescence test is used to identify unknown antigen in a cell or tissue.
- If antigen is present, it reacts with labelled antibody, and the antibody-coated antigen can be detected under UV fluorescent light.
- The requirement to prepare a distinct labelled antibody for each pathogen is the most significant drawback of the direct immunofluorescence test.
b. Indirect immunofluorescence test
- The indirect immunofluorescence test is utilised for the serodiagnosis of numerous infectious disorders to identify particular antibodies in serum and other body fluids. Immunofluorescence indirecte is a two-step method.
- In the initial step, a known antigen is attached to a slide.
- The testing patient’s serum is then put to the slide, followed by a thorough washing. If the patient’s serum contains antigen-specific antibodies, they will bind to the antigen on the slide.
- In the second step, the combination of antibody and antigen can be identified by adding a fluorescent dye-labeled antibody to human IgG, which is then analysed using a fluorescence microscope.
- In the first step of the indirect immunofluorescence test, a fixed antigen (e.g., in a cell or tissue) is incubated with an unlabeled antibody, which binds to the antigen.
- After a thorough washing, a fluorescent antibody (e.g., anti-IgG with a fluorescent label) is added to the smear. This second antibody will bind to the first, allowing visualisation of the antigen–antibody combination under a fluorescent microscope.
- The indirect method has the advantage that a single labelled antiglobulin (antibody to IgG) can be used as a “universal reagent” to detect a variety of unique antigen–antibody responses.
- Typically, the test is more sensitive than direct immunofluorescence.
- Immunofluorescence is limited in that it requires (a) a costly fluorescence microscope and chemicals, (b) trained workers, and (c) a subjective aspect that may lead to inaccurate results.
Application of Immunofluorescence
- Direct immunofluorescence test for antemortem diagnosis of rabies: This test is used to detect rabies virus antigen in the skin smear taken from the nape of the neck of humans and the saliva of dogs.
- Also employed for N detection. gonorrhoeae, C. T. diphtheriae pallidum, etc. directly in relevant clinical specimens.
- Detect particular antibodies for the serodiagnosis of infectious disorders such as syphilis, leptospirosis, amoebiasis, and toxoplasmosis.
- Utilize fluorescent antibodies specific for various immunoglobulin isotypes to determine the class of a given antibody.
- Utilizing monoclonal antibodies and cytofluorographs, identify and quantify lymphocyte subpopulations.
- In autoimmune illnesses, detect autoantibodies, such as antinuclear antibodies.
7. Enzyme Immunoassays
- Enzyme immunoassays (EIAs) can be used to detect antigens or antibodies in the patient’s serum and other body fluids.
- Enzyme-labeled antigen or antibody is utilised in EIA methods. EIA tests utilise alkaline phosphatase, horseradish peroxidase, and galactosidase as enzymes.
- EIAs are routinely employed enzyme-linked immunosorbent tests (ELISAs). Peter Perlmann and Eva Engvall of Stockholm University, Sweden, first conceptualised and developed the ELISA technique.
- In these tests, an immunosorbent specific to the antigen or antibody is utilised.
- After the antigen-antibody reaction, an enzyme-specific chromogenic substrate (o-phenyldiamine dihydrochloride for peroxidase, p-nitrophenyl phosphate for alkaline phosphatase, etc.) is added.
- To detect the reaction, the optical density is measured. Typically, the unknown concentrations of antigen or antibody are determined using a standard curve based on known concentrations of antigen or antibody.
Types of Enzyme Immunoassays
There are a variety of ELISAs for detecting and quantifying antigens or antibodies in serum and other body fluids.These include: (a) indirect ELISA, (b) sandwich ELISA, (c) competitive ELISA, and (d) ELISPOT assay.
- Indirect ELISA: The indirect ELISA is used to quantify antibody concentrations in serum and other bodily fluids.
- Sandwich ELISA: Sandwich ELISA is utilised for antigen detection. This test involves coating and immobilising the known antibody on the wells of microtiter plates. The antigen-containing test sample is added to the wells and allowed to react with the antibodies in the wells.
- Competitive ELISA: Competitive ELISA is another method for determining the amount of antibodies in a sample, such as serum. The test employs two specific antibodies, one coupled with an enzyme and the other present in the test serum (if the serum is antibody-positive). There is competition between the two antibodies for the identical antigen.
- ELISPOT Assay: The ELISPOT assay is a variation of the ELISA assay. It enables the quantitative assessment of the number of cells in a population that produce antibodies specific for a certain antigen or an antigen for which a specific antibody exists. These tests have found widespread application in cytokine quantification.
Application of Enzyme Immunoassays
- Sandwich ELISA is utilised to detect rotavirus and Escherichia coli enterotoxin in faeces.
- Competitive ELISA is the most popular method for detecting HIV antibodies in the serum of HIV-positive patients.
- The test is widely utilised to determine blood antibodies for the diagnosis of human immunodeficiency virus (HIV) infection, Japanese encephalitis, dengue, and numerous other viral infections.
8. Radioimmunoassay
- When radioisotopes rather than enzymes are employed to mark antigens or antibodies, the technique for detecting the antigen–antibody combination is known as radioimmunoassay (RIA).
- Solomon Berson and Rosalyn Yalow of the New York Veterans Administration Hospital were the first to describe RIA in 1960 for measuring endogenous plasma insulin.
- Yalow was given the 1977 Nobel Prize in Medicine for the discovery of the RIA for peptide hormones, but Berson was unable to share the award due to his tragic death in 1972.
- The classical RIA methods are founded on the competitive binding principle. In this technique, unlabeled antigen competes with radiolabeled antigen for binding to the particular antibody.
- When mixtures of radiolabeled and unlabeled antigen are incubated with the matching antibody, the amount of unbound radiolabeled antigen is directly proportional to the amount of unlabeled antigen in the mixture.
- In the first step of the test, mixtures of known variable amounts of cold antigen and fixed amounts of labelled antigen are generated, as well as mixtures of samples with unknown concentrations of antigen and identical amounts of labelled antigen. A comparable amount of antibody is added to each mixture.
- Antigen–antibody complexes are precipitated by either crosslinking with a second antibody or by adding chemicals that facilitate antigen–antibody complex precipitation.
- By counting the radioactivity in the precipitates, the amount of radiolabeled antigen precipitated with the antibody can be determined.
- Antigen concentrations in patient samples are inferred using a standard curve created by graphing the fraction of antibody-bound radiolabeled antigen against known quantities of a standardised unlabeled antigen.
- RIA’s primary benefit is its extraordinarily high sensitivity.
- Antigens and antibodies in the serum can be determined down to the nanogram level using this test.
- This test is utilised to determine the concentration of hormones, medications, HBsAg, and other viral antigens.
- The principal disadvantages of the RIA include:
- the price of equipment and chemicals.
- The limited shelf life of radiolabeled substances.
- the issues linked with radioactive waste disposal.
9. Western Blotting
- Western blotting is so named because it is comparable to Southern blotting, which Edwin Southern invented for the detection of DNA.
- Western blotting is used to detect proteins, whereas Southern blotting is used to detect DNA. Western blotting is often performed on homogenates or extracts of tissue.
- It employs SDS-PAGE (sodium dodecyl sulphatepolyacrylamide gel electrophoresis), a form of gel electrophoresis, to initially separate proteins in a mixture based on their shape and size using gel electrophoresis.
- The resulting protein bands are transferred to a nitrocellulose or nylon membrane, where they are “probed” with antibodies specific to the target protein.
- The antigen–antibody complexes that form on the band containing the antibody-recognized protein can be seen in a number of different ways.
- If the target protein was captured by a radioactive antibody, its position on the blot can be identified by exposing the membrane to a sheet of X-ray film, a technique known as autoradiography.
- However, the most common detection methods involve enzyme-linked antibodies to the protein.
- After the enzyme–antibody combination has bound to the target antigen, the addition of a chromogenic substrate that yields a highly coloured and insoluble product promotes the formation of a colourful band at the site of the antigen.
- If a chemiluminescent chemical and suitable boosting agents are used to produce light at the antigen site, the site of the target protein can be determined with far greater sensitivity.
Application of Western Bloting
- It is used to identify a specific protein within a complicated protein mixture. Antigens of well-defined molecular weight are separated by SDS-PAGE and blotted onto nitrocellulose in this procedure. The separated bands of known antigens are next examined with a sample thought to have antibodies specific to one or more of these antigens. Using either a radiolabeled or enzyme-linked secondary antibody that is specific for the species of the antibodies in the test sample, the reaction of an antibody with a band is identified.
- It is also used to estimate the protein’s size and the amount of protein in a combination.
- The Western blot test is most commonly employed as a confirming test for the HIV diagnosis, where it is utilised to evaluate whether or not the patient has antibodies that respond with one or more viral proteins.
- For the diagnosis of neurocysticercosis and tuberculous meningitis, Western blotting is also performed to demonstrate the presence of particular antibodies in the blood.
10. Immunoelectronmicroscopic Tests
These are the types of antigen–antibody interactions that can be directly observed using an electron microscope. These fall into the following categories:
a. Immunoelectronmicroscopy
- This test is used to directly detect rotavirus and hepatitis A virus in faeces.
- In this test, viral particles are combined with specific antisera and observed under an electron microscope as clusters of virion particles.
b. Immunoenzyme test
- This test is used to directly detect antigen in tissue samples by treating tissue sections with peroxidase-labeled antisera to detect corresponding antigen.
- Under an electron microscope, the peroxidase-antigen complex is visualised.
c. Immunoferritin test
- Electron-dense compounds, such as ferritin, are conjugated with antibody, allowing the electron microscope to visualise labelled antibodies reacting with antigen.
11. Chemiluminescence Assay
- During antigen–antibody reactions, the chemiluminescence assay employs chemiluminescent substances that emit energy in the form of light.
- The emitted light is measured, and the concentration of the substance being analysed is computed.
- The assay is a completely automated approach often used to evaluate Mycobacterium TB for drug sensitivity.
Alternatives to Antigen-Antibody Reactions
- Some bacteria have evolved the ability to produce proteins that bind to the Fc region of IgG molecules with high affinity (Ka ~ 108) as a protection against host antibodies.
- Protein A is present in the cell walls of some strains of Staphylococcus aureus, while protein G is present in the cell walls of group C and G Streptococcus.
- By cloning the genes for protein A and protein G and making a hybrid of both, it is possible to create protein A/G, a recombinant protein that combines some of the best characteristics of each.
- These molecules are advantageous because they can bind IgG from numerous species. Consequently, they can be labelled with flourochromes, radioactivity, or biotin to detect IgG molecules in antigen-antibody complexes generated during ELISA, RIA, or fluorescence-based tests such as flow cytometry or fluorescence microscopy.
- These bacterial IgG-binding proteins can also be employed to create IgG-isolating affinity columns. Egg whites include avidin, a protein that binds biotin, a necessary nutrient for fat formation.
- Avidin is thought to have evolved as a protection against nest-robbing rats that consume the stolen eggs. The binding between avidin and biotin is extraordinarily selective and has a significantly greater affinity (Ka ~ 1015) than any known antigen-antibody response.
- Streptavidin, a bacterial protein produced by streptomyces avidinii, possesses comparable affinity and specificity. The high affinity and perfect specificity of these proteins’ interactions with biotin are routinely utilised in numerous immunological processes.
- After labelling the main or secondary antibody with biotin and allowing it to react with the target antigen, the unbound antibody is removed by washing.
- The bound antibody is then detected using streptavidin or avidin coupled with an enzyme, fluorochrome, or radioactive marker.
Limitations of Antigen-Antibody Interaction
While antigen-antibody interactions have significant applications, there are also certain limitations associated with these interactions. Here are some notable limitations:
- Limited Accessibility in Developing Countries: Techniques based on antigen-antibody interactions often require specialized equipment and expertise, which may not be readily available in developing or underdeveloped countries. This lack of resources can hinder the widespread use of these techniques in such regions, impacting their ability to benefit from these diagnostic and research tools.
- Inadequate Sensitivity in Rapid Diagnostic Tests (RDTs): Rapid diagnostic tests (RDTs) based on antigen-antibody interactions may not reliably detect low-density parasitemia, particularly in the case of diseases like malaria. RDTs may have a threshold for detection, and parasitemia levels below this threshold may produce false-negative results. Therefore, these tests may not accurately identify infections with low parasite densities (≤200 parasites/µL).
- Challenges in Distinguishing Early and Late Infections: In some cases of antibody detection, it can be difficult to differentiate between early and late infections. Antibodies can persist in the bloodstream for a prolonged period, even after the infection has been successfully treated or resolved. This means that the presence of antibodies alone does not necessarily indicate an ongoing infection. Additional tests and clinical considerations are often required to distinguish between active and past infections accurately.
- Cross-Reactivity and Specificity: Antigen-antibody interactions can sometimes exhibit cross-reactivity, where antibodies recognize similar epitopes on different antigens. This cross-reactivity can lead to false-positive results or make it challenging to identify the specific target antigen accurately. Ensuring the specificity of the interaction is crucial for accurate diagnosis and interpretation of results.
- Sensitivity and Limit of Detection: The sensitivity of antigen-antibody interactions can vary depending on the specific assay or test being used. Some assays may have limitations in detecting low levels of antigens or antibodies, which could result in false-negative results or reduced sensitivity. The limit of detection refers to the lowest concentration of the target analyte that can be reliably detected by the assay, and it can vary between different tests and techniques.
- Variability and Interference: Antigen-antibody interactions can be influenced by various factors, including the presence of interfering substances in the sample. Some substances present in biological samples may interfere with the interaction, affecting the accuracy and reliability of the results. These interferences can lead to false-positive or false-negative results and may require additional steps to mitigate their impact.
- Limitations of Specific Assays: Different assays based on antigen-antibody interactions may have specific limitations related to their design, sensitivity, specificity, or other factors. It is important to consider these limitations when selecting and interpreting assay results to ensure accurate diagnosis, monitoring, and research outcomes.
Applications of Antigen-Antibody Interaction
Antigen-antibody interaction plays a crucial role in various applications across different fields. Here are some notable applications:
- Blood Typing: One of the most common uses of antigen-antibody interaction is in determining blood groups. By introducing specific antibodies against antigens present on red blood cells, the presence or absence of antigens can be detected, enabling the identification of blood types such as A, B, AB, or O.
- Rapid Diagnostic Tests: Rapid diagnosis test kits utilize antigen-antibody interactions to detect the presence of specific antigens or antibodies in biological samples. These tests are widely used for various purposes, including pregnancy detection and the identification of infectious diseases such as malaria, dengue, and many others. These kits offer quick results, requiring minimal time for the tests to be performed.
- Serological Testing: Antigen-antibody interactions are employed in serological tests to determine the exposure to infectious agents. By detecting the presence of specific antibodies in a person’s blood serum, healthcare professionals can ascertain whether an individual has been exposed to a particular pathogen, aiding in the diagnosis and surveillance of infectious diseases.
- Quantification of Substances: Antigen-antibody interactions are utilized in immunoassays to quantify various substances in biological samples. For example, the concentration of drugs, hormones, viral antigens, and other biomarkers can be measured using techniques like enzyme-linked immunosorbent assay (ELISA). These quantitative assays provide valuable information for medical diagnosis, research, and monitoring treatment effectiveness.
- Protein Detection: Antigen-antibody interactions are employed to detect the presence or absence of specific proteins in serum or other biological samples. Techniques such as immunoblotting or Western blotting utilize antibodies to identify and characterize target proteins based on their molecular weight, allowing researchers to study protein expression patterns and investigate diseases or abnormalities related to protein expression.
- Immunodeficiency Disease Studies: Antigen-antibody interactions help in studying the characteristics of different immunodeficiency diseases. By analyzing the interactions between antigens and antibodies, researchers can gain insights into the functioning of the immune system and identify deficiencies or abnormalities associated with various immune disorders.
- Confirmatory Tests for Infections: Antigen-antibody interactions are critical for confirmatory tests in infectious disease diagnostics. Western blotting, for example, is commonly used as a confirmatory test for HIV infection. It detects specific viral antigens or antibodies present in a patient’s blood sample, providing a more accurate diagnosis of HIV infection compared to initial screening tests.
FAQ
What is antigen-antibody interaction?
Antigen-antibody interaction is a specific binding between an antigen (a foreign substance) and an antibody (a protein produced by the immune system in response to the antigen). This interaction forms the basis of immune responses and various diagnostic techniques.
How does antigen-antibody interaction work?
Antibodies recognize and bind to specific antigens through complementary molecular structures. The binding occurs between the antigen’s epitopes (specific regions) and the antibody’s antigen-binding sites, facilitating immune responses or diagnostic assays.
What are the applications of antigen-antibody interactions?
Antigen-antibody interactions have various applications, including blood typing, diagnostic tests (e.g., pregnancy tests), serological assays, quantification of substances, protein detection, immunodeficiency disease studies, and confirmatory tests for infections like HIV.
How are antigen-antibody interactions used in diagnostic tests?
In diagnostic tests, antigen-antibody interactions are utilized to detect the presence or absence of specific antigens or antibodies in biological samples. These interactions form the basis of assays such as ELISA, lateral flow assays, and Western blotting.
What are the challenges in antigen-antibody-based diagnostic tests?
Some challenges include sensitivity issues at low analyte concentrations, cross-reactivity with related antigens, distinguishing between active and past infections, and the need for proper equipment and expertise to perform the tests accurately.
Can antigen-antibody interactions be used for therapeutic purposes?
Yes, antigen-antibody interactions can be harnessed for therapeutic purposes. For example, monoclonal antibodies are used in immunotherapy to target specific antigens on cancer cells or pathogens, aiding in their destruction.
What is cross-reactivity in antigen-antibody interactions?
Cross-reactivity refers to the ability of an antibody to bind to multiple antigens that share structural similarities. This can lead to false-positive results or challenges in identifying the specific target antigen.
How do researchers study antigen-antibody interactions?
Researchers study antigen-antibody interactions using techniques like immunoprecipitation, immunofluorescence, flow cytometry, and surface plasmon resonance. These techniques allow for the characterization and analysis of binding interactions.
Can antigen-antibody interactions be used to identify unknown substances?
Yes, antigen-antibody interactions can be used to identify unknown substances through techniques like immunoassays. By comparing the binding patterns of known antigens and antibodies, unknown substances can be detected and identified.
How long do antibodies remain in the bloodstream after an infection?
Antibodies can persist in the bloodstream for a variable period, depending on the type of infection and the individual’s immune response. In some cases, antibodies can remain detectable in the blood even after the infection has been cured, making it challenging to distinguish between active and past infections based solely on antibody presence.
References
- Reverberi R, Reverberi L. Factors affecting the antigen-antibody reaction. Blood Transfus. 2007 Nov;5(4):227-40. doi: 10.2450/2007.0047-07. PMID: 19204779; PMCID: PMC2581910.
- MILLER, J. J., & LEVINSON, S. S. (1996). INTERFERENCES IN IMMUNOASSAYS. Immunoassay, 165–190. doi:10.1016/b978-012214730-2/50008-x
- http://www.jiwaji.edu/pdf/ecourse/pharmaceutical/Antigen-and-antibody-reaction.pdf
- https://www.microbiologybook.org/mayer/ab-ag-rx.htm
- https://byjus.com/biology/antigen-antibody-reaction-types/
- http://dhingcollegeonline.co.in/attendence/classnotes/files/1603721363.pdf
- https://www.sscollegejehanabad.org/study-material/1565396598Antigen-antibody%20interaction.pdf
- https://www.notesonzoology.com/antigens/antigen-antibody-interaction-with-reactions-zoology/4651
- https://www.rnlkwc.ac.in/pdf/study-material/zoology/Antigen-Antibody%20interaction.pdf
- https://www.slideshare.net/Haddies/antigen-and-antibody-reaction
- https://www.sigmaaldrich.com/IN/en/technical-documents/technical-article/protein-biology/elisa/antibody-antigen-interaction
- https://microbenotes.com/introduction-to-antigen-antibody-reactions/