The concept of using fluorescence to study biological materials dates back to the late 19th century, but the first practical fluorescence microscope was not developed until the 1930s. In 1938, Robert Goldstein and Richard Manly, researchers at the University of California, Berkeley, published a paper describing the use of fluorescence to study cells and tissues in living animals. This marked the beginning of the modern era of fluorescence microscopy.
Since then, fluorescence microscopy has undergone significant technological advances. In the 1960s, the development of lasers allowed for the use of laser-excited fluorescence, which greatly increased the sensitivity and resolution of fluorescence microscopes. In the 1980s, the development of confocal laser scanning microscopy allowed for the creation of high-resolution, three-dimensional images of fluorescence samples. And in the 1990s and 2000s, the development of superresolution fluorescence microscopy techniques, such as stimulated emission depletion (STED) and photoactivated localization microscopy (PALM), allowed for the visualization of subcellular structures with resolutions much higher than was previously possible.
What is Fluorescence Microscopy?
- A fluorescence microscope is a specialized optical instrument designed to study the properties of organic or inorganic substances using the principles of fluorescence and phosphorescence. These phenomena are distinct from reflection and absorption, which are commonly employed in traditional microscopy.
- Fluorescence is the process where a substance absorbs light or electromagnetic radiation and subsequently emits light. On the other hand, phosphorescence is a type of photoluminescence related to fluorescence. However, the primary distinction between the two is the duration of light emission. In phosphorescence, the emitted light persists for a more extended period after the initial excitation source is removed.
- The inception of the fluorescence microscope can be traced back to the early twentieth century, credited to pioneers like August Köhler, Carl Reichert, and Heinrich Lehmann. Their innovative work laid the foundation for the development of this powerful microscopy technique.
- Therefore, the term “fluorescence microscope” encompasses any microscope that employs fluorescence to produce an image. This can range from simple configurations, such as an epifluorescence microscope, to more intricate designs like the confocal microscope. The latter, in particular, utilizes optical sectioning to enhance the resolution of the fluorescence image.
- In the realm of fluorescence microscopy, optical filters play a pivotal role. These filters illuminate the specimen with a specific wavelength and are designed to selectively detect only a particular wavelength of light emitted from the specimen. Besides, any segment of the specimen that absorbs light at the illumination wavelength and subsequently emits it at the detection wavelength becomes visible to the observer. This unique capability offers a contrast technique not available in other microscopy forms.
- Furthermore, some materials naturally exhibit fluorescence, termed ‘auto-fluorescent’ materials. Detecting these materials predominantly involves choosing the right filters that match their natural excitation and emission wavelengths. However, for a majority of specimens, specific fluorescent dyes, known as fluorophores, are employed. These fluorophores can be incredibly selective, enabling, in certain instances, the detection of individual molecules.
- Due to the enhanced contrast provided by fluorophores, fluorescence microscopy has emerged as an invaluable tool in the life sciences. Specimens in this field can be intricate in composition and structure, necessitating the additional contrast offered by fluorescence tagging. Over the years, advancements like Immunofluorescence and expressible fluorescent proteins have further augmented the capabilities of fluorescence microscopy.
- Fluorophores, typically organic molecules, often contain conjugated aromatic groups. A prevalent example is fluorescein isothiocyanate (FITC). Some fluorophores can even alter their fluorescence properties in the presence of specific compounds, facilitating indirect detection of those compounds. A notable instance of this is calcium imaging.
- In conclusion, fluorescence microscopy is a powerful and versatile tool, offering insights into the intricate world of biological specimens. Its ability to provide high contrast and detailed images makes it indispensable in modern labs. As research progresses, the potential applications and techniques associated with fluorescence microscopy are bound to expand, further emphasizing its significance in scientific exploration. [2,10,1]
Types of Fluorescence Microscopes
Fluorescence microscopy is a powerful imaging technique used to visualize specimens that emit light at specific wavelengths. There are several types of fluorescence microscopes, each with its own set of features and applications.
- Wide-field Epifluorescence Microscopy: Wide-field epifluorescence microscopy is a common and relatively straightforward form of fluorescence microscopy. In this technique, the entire specimen is uniformly illuminated with light at the excitation wavelength. The fluorescent parts of the specimen absorb this light and re-emit it at a different emission wavelength. The key optical components of a wide-field epifluorescence microscope include filters and dichroic mirrors that ensure only the excitation wavelength reaches the specimen and only the emission wavelength reaches the detector. This type of microscope is often an enhancement to a standard optical microscope, allowing for the visualization of fluorescently labeled specimens.
- Confocal Fluorescence Microscopy: Confocal fluorescence microscopy offers increased resolution and optical sectioning capabilities. This technique uses a pinhole to ensure that only light from a single focal plane is detected, significantly reducing the blur from out-of-focus light. The specimen is scanned point by point and layer by layer, which allows for the reconstruction of a three-dimensional image of the specimen. Confocal microscopes often use laser light sources to provide intense, focused illumination. This method is particularly beneficial for thick specimens where out-of-focus light can obscure details.
- Total Internal Reflection Fluorescence Microscopy (TIRF): TIRF microscopy is a specialized technique that provides high-resolution imaging of events occurring near the cell membrane. It utilizes an evanescent wave, which is generated when light reflects at the interface between two media (e.g., glass and water) at a specific angle, to excite fluorophores. The evanescent wave penetrates only about 100-200 nm into the specimen, which means only the molecules very close to the glass surface are excited and emit fluorescence. This selective illumination is ideal for studying cellular processes like vesicle trafficking or receptor-ligand interactions at the cell surface.[2]
Principle of Fluorescence Microscope – How does fluorescence microscopy work?
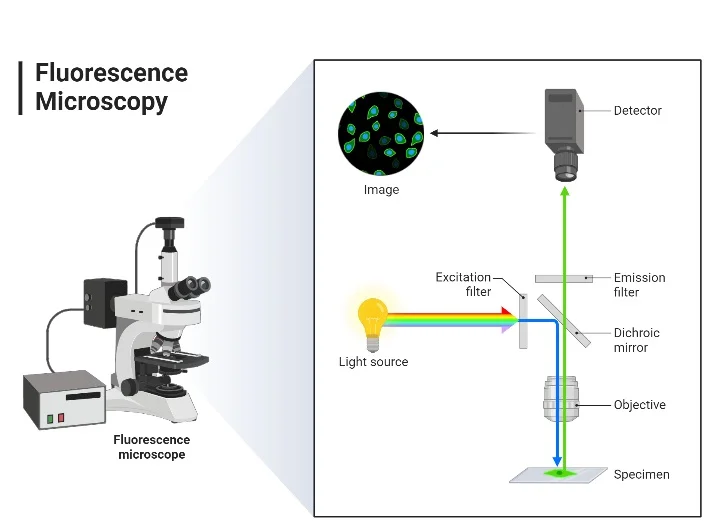
Fluorescence microscopy is a powerful imaging technique that allows researchers to visualize specific components within a specimen based on their ability to emit light after being excited by a specific wavelength. The underlying principles of this technique are rooted in the properties of fluorescent molecules and the way they interact with light.
- Fluorescent Dyes (Fluorophores) Fluorescent dyes, also known as fluorophores or fluorochromes, are specialized molecules that can absorb light at a specific wavelength and then emit light at a longer wavelength. The time delay between absorption and emission is typically very short, often on the order of nanoseconds.
- Excitation and Emission The fundamental process in fluorescence microscopy involves the excitation of fluorophores using a specific wavelength of light. Once excited, these molecules emit light at a different, typically longer, wavelength. This emitted light is what is visualized in fluorescence microscopy.
- Optical Components A fluorescence microscope is equipped with specific optical components designed to separate the excitation light from the emitted fluorescence. These components include:
- Excitation Filter: This filter ensures that only the desired excitation wavelength reaches the specimen.
- Dichroic Mirror: This mirror reflects the excitation light onto the specimen and allows the emitted fluorescence to pass through.
- Emission Filter: Positioned before the detector, this filter ensures that only the emitted fluorescence is detected, filtering out any residual excitation light.
- Forms of Fluorescence Microscopy The term “fluorescence microscope” encompasses various configurations. The most common form is the epifluorescence microscope, where both excitation and detection of fluorescence occur through the same optical path, typically the objective lens. This design is often an enhancement to standard optical microscopes. More advanced designs, such as confocal microscopes, offer improved resolution and optical sectioning capabilities.
- Image Formation The image produced in fluorescence microscopy is based on the emitted light from the fluorophores, not the initial excitation light. This distinction is crucial as it allows for high-contrast imaging of specific components within a specimen, even if those components are transparent or similar in appearance under standard light microscopy.
- Applications and Enhancements Fluorescence microscopy has been further enhanced with techniques like confocal microscopy, which provides optical sectioning capabilities. By scanning the specimen point by point and layer by layer, confocal microscopy allows for the reconstruction of three-dimensional images of the specimen.[2,10,1]
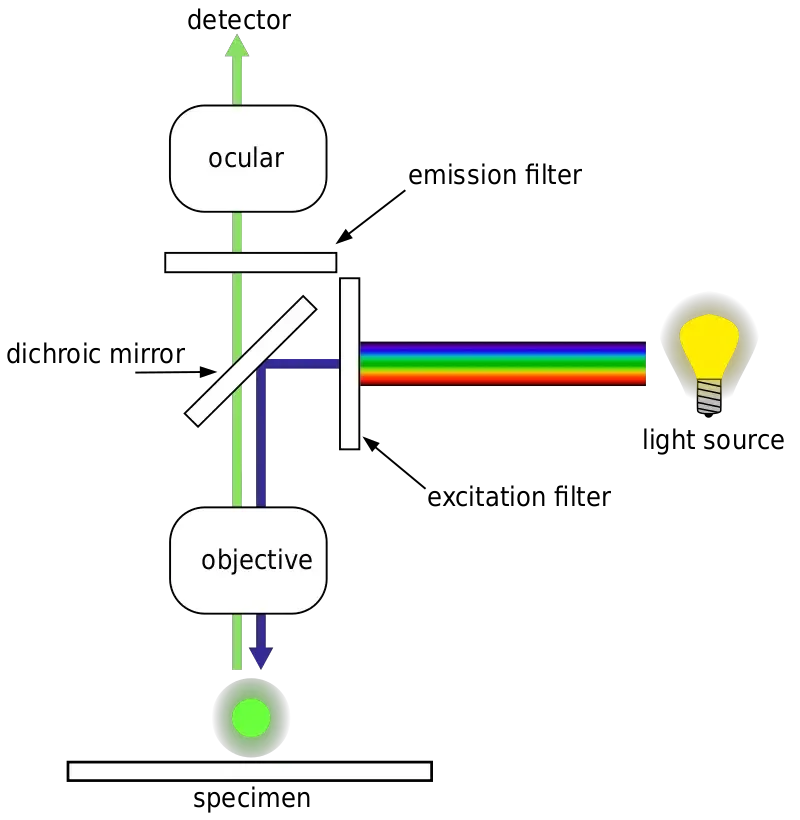
Parts of Fluorescence Microscope
Fluorescence microscopy is a specialized technique that allows for the visualization of specimens based on their ability to emit light after being excited. This method requires specific components to effectively separate the excitation light from the emitted fluorescence. Here are the essential parts of a fluorescence microscope:
- Fluorescent Dyes (Fluorophore)
- Fluorophores are fluorescent chemical compounds capable of re-emitting light upon excitation.
- They often contain several combined aromatic groups or plane/cyclic molecules with multiple π bonds.
- Various fluorescent stains have been developed for different biological molecules, such as nucleic acid stains like DAPI and Hoechst, and phalloidin for staining actin fibers in mammalian cells.
- Light Source
- A high-intensity light source is crucial due to the low signals in fluorescence microscopy.
- Common sources include mercury or xenon arc sources, high-powered LEDs, and lasers.
- The choice of light source depends on the required intensity, type, and luminance.
- Excitation Filter
- This filter narrows the wavelength of the light that illuminates the sample, ensuring that only the desired excitation wavelength reaches the specimen.
- Dichroic Mirror
- Positioned after the excitation filter, the dichroic mirror reflects the excitation light towards the specimen.
- It acts as a gate, reflecting the excitation wavelength while allowing the emitted wavelength to pass through.
- Objective
- The objective collects the light into a narrow beam on the sample and also focuses the light emitted back from the specimen.
- Sample Stage
- This component holds the specimen and typically has x, y, and z-axis motion, either manually or computer-controlled.
- Emission Filter
- After passing through the specimen, the emitted light contains both the excitation wavelength and the fluorescence from the specimen.
- The emission filter removes all undesired wavelengths, allowing only the emitted fluorescence to pass through.
- Eyepieces and Detector
- Modern fluorescence microscopes usually have a trinocular eyepiece setup.
- The light passing through the emission filter is directed either to eyepieces for observation or to a camera for digital imaging.
- Digital imaging combined with image processing can be used for quantitative analysis of the fluorescence images.[2]
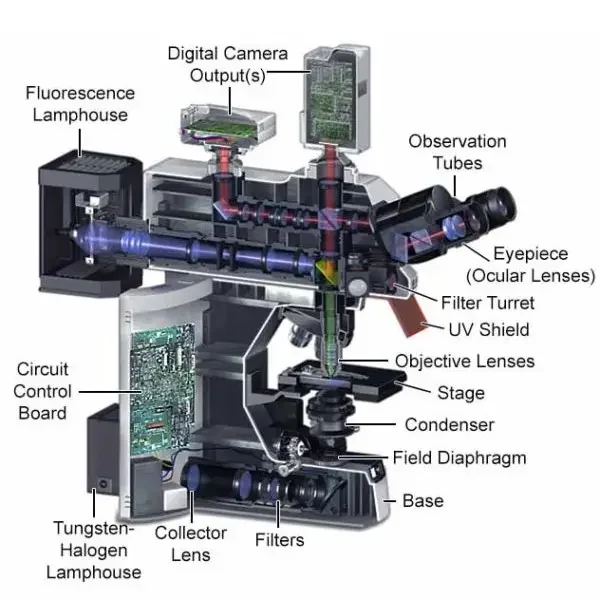
Sample Preparation for Fluorescence Microscope
- Introduction to Fluorescent Samples
- For a sample to be suitable for fluorescence microscopy, it must exhibit fluorescence. This fluorescence can be achieved through various methods, including labeling with fluorescent stains or, for biological samples, expressing a fluorescent protein. Some samples may also exhibit intrinsic fluorescence, known as autofluorescence.
- Biological Fluorescent Stains
- A wide range of fluorescent stains has been developed to target specific biological molecules.
- Some stains are intrinsically fluorescent small molecules that bind to specific biological molecules. For instance, nucleic acid stains like DAPI, Hoechst, DRAQ5, and DRAQ7 bind to the minor groove of DNA, labeling cell nuclei.
- Other stains, such as drugs, toxins, or peptides, bind to specific cellular structures and are derivatized with a fluorescent reporter. A notable example is phalloidin, used to stain actin fibers in mammalian cells. Another emerging peptide, the Collagen Hybridizing Peptide, can be conjugated with fluorophores to stain denatured collagen fibers.
- For plant cells, stains or dyes that bind to cellulose or pectin are used to stain the cell walls.
- Immunofluorescence
- Immunofluorescence is a powerful technique that leverages the specificity of antibody-antigen binding to label specific proteins or other molecules within a cell.
- The process involves treating a sample with a primary antibody that specifically targets the molecule of interest. This primary antibody can be directly conjugated with a fluorophore. Alternatively, a secondary antibody, labeled with a fluorophore, can be used to bind to the primary antibody, amplifying the fluorescent signal.
- An example of this technique involves using a primary antibody raised in a mouse to recognize tubulin, combined with a fluorophore-conjugated secondary anti-mouse antibody to label microtubules in a cell.
- Fluorescent Proteins
- Advances in genetics and molecular biology techniques have enabled scientists to genetically modify proteins to carry a fluorescent protein reporter.
- This modification allows direct fluorescence of the protein of interest, enabling its location to be tracked, even in live cells. This approach eliminates the need for external staining, providing a more direct and often more accurate representation of protein localization and dynamics.[10]
Light Path In Fluorescence Microscopy
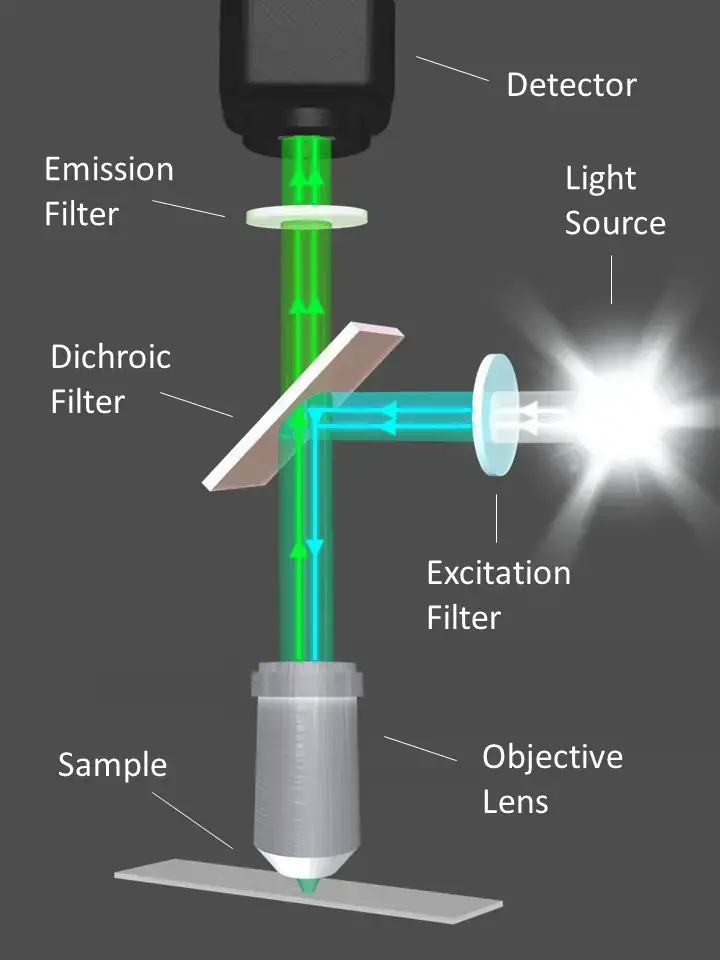
- Source of Light
- The fluorescence microscope utilizes a high-intensity Mercury lamp as its light source. This lamp is capable of emitting light across the visible spectrum, commonly referred to as white light.
- Excitation of the Sample
- The exciter filter is a critical component that selectively transmits blue light while blocking other wavelengths. This blue light is then directed toward the specimen, ensuring that only the desired excitation wavelength reaches the sample.
- Reflection and Direction
- A dichroic mirror is strategically placed in the light path. This mirror is designed to reflect certain colors—blue light in this case—downward toward the specimen. Conversely, it is engineered to transmit light of other colors, particularly those that will be emitted by the fluorescent dye.
- Interaction with the Specimen
- The specimen, previously stained with a fluorescent dye, interacts with the blue light. Specific portions of the specimen that retain the dye absorb the blue light and subsequently emit light at a different wavelength, typically green.
- Emission and Filtration
- The emitted green light from the stained portions of the specimen travels upward, penetrating the dichroic mirror due to its transmission properties at the emission wavelength.
- Barrier Filter
- As the emitted light continues its journey, it encounters the barrier filter. This filter is designed to allow the passage of green light while blocking any residual blue light that may not have been completely deflected by the dichroic mirror.
- Visualization
- The result of this selective filtration is that the observer perceives only the stained portions of the specimen, which appear as glowing green against a stark black background. The unstained portions, which do not emit the green light, remain invisible.
This sequential and selective process of light manipulation—beginning with the high-intensity Mercury lamp and culminating in the observer’s perception—allows fluorescence microscopy to visualize specific components within a specimen with high contrast and specificity.[3,5,6]
Fluorescence microscope light source
The light source of a fluorescence microscope is an important component that is responsible for providing the excitation light that is used to excite the fluorophores in the sample.
There are several types of light sources that are commonly used in fluorescence microscopy, including:
- Mercury arc lamps: Mercury arc lamps are one of the most commonly used light sources in fluorescence microscopy. They produce a broad spectrum of light that can be used to excite a wide range of fluorophores. However, they may produce unwanted emissions, which can interfere with fluorescence imaging.
- Metal halide lamps: Metal halide lamps are a newer type of light source that produce a more focused spectrum of light than mercury arc lamps. They are often used to excite specific fluorophores and can provide higher intensity and more stable light output than mercury arc lamps.
- LED light sources: LED (light-emitting diode) light sources are becoming increasingly popular in fluorescence microscopy due to their long lifetimes and low power consumption. They produce a narrow spectrum of light that can be used to excite specific fluorophores and can be easily controlled and modulated for advanced imaging applications.
- Laser light sources: Laser light sources produce a highly focused and coherent beam of light that can be used to excite specific fluorophores. They are often used in advanced imaging techniques, such as superresolution microscopy, that require high-intensity, highly focused light.
Overall, the choice of light source for a fluorescence microscope depends on the specific requirements of the research application and the type of fluorophores being imaged.[6]
Fluorescence microscope camera
A camera is an important component of a fluorescence microscope that is used to capture and record images of the sample. Fluorescence microscopes typically use specialized cameras that are sensitive to the wavelengths of light emitted by the fluorophores in the sample.
There are several types of cameras that are commonly used in fluorescence microscopy, including:
- CCD cameras: CCD (charge-coupled device) cameras are widely used in fluorescence microscopy due to their high sensitivity and low noise. They are based on a linear array of pixels that convert light into an electrical signal, which can be processed and stored as an image.
- CMOS cameras: CMOS (complementary metal-oxide-semiconductor) cameras are an alternative to CCD cameras that are based on a different type of pixel technology. They offer similar performance to CCD cameras and are often used in fluorescence microscopy due to their lower power consumption and smaller size.
- Scientific-grade cameras: Scientific-grade cameras are specialized cameras that are designed for use in scientific research applications. They offer higher sensitivity and resolution than consumer-grade cameras and are often used in advanced imaging techniques, such as superresolution microscopy.
Overall, the choice of camera for a fluorescence microscope depends on the specific requirements of the research application and the type of images being captured. It is important to choose a camera that is compatible with the microscope and has the necessary sensitivity and resolution for the research application.[6]
Analyzing a Specimen With a Fluorescence Microscope
- Sample Preparation
- Proper sample preparation is paramount for fluorescence microscopy. The primary challenge lies in ensuring that the structures of interest within the sample are fluorescent. If the structures are not naturally fluorescent, they must be labeled using specific techniques, such as fluorescent dyes or fluorescent proteins.
- Fluorescent Labeling
- There are multiple methods to achieve fluorescent labeling:
- Biological Fluorescent Stains: These are designed to bind specific biological molecules. Examples include nucleic acid stains like DAPI, Hoechst, and phalloidin for actin fibers.
- Immunofluorescence: This technique leverages the specificity of antibodies to target antigens. A primary antibody binds to the molecule of interest, and a secondary antibody, conjugated with a fluorophore, binds to the primary antibody.
- Fluorescent Proteins: Genetic engineering allows proteins of interest to be tagged with fluorescent proteins, enabling direct visualization.
- There are multiple methods to achieve fluorescent labeling:
- Microscope Setup
- Once the sample is prepared, the microscope must be set up with optics that match the chosen fluorophore. Manufacturers often provide guides to assist in matching filters to fluorophores.
- Slide Preparation
- The microscope slide and coverslip must be cleaned to ensure they do not exhibit autofluorescence. A droplet of the sample is placed on the slide, covered with a coverslip, and then observed under the microscope.
- Analysis
- Start with low magnification, making adjustments to focus, illumination, and positioning. As the magnification increases, continue to fine-tune the focus and positioning to achieve the best image clarity.
- Digital images of areas of interest can be captured for further analysis. It’s crucial to name these files descriptively or make notes for easy retrieval later.
- Consideration of Photobleaching
- Fluorophores can undergo photobleaching, where they lose their fluorescence properties upon prolonged exposure to excitation light. This is due to chemical reactions within the fluorophore or with the surrounding medium. Some fluorophores are more susceptible to photobleaching than others. To mitigate this, reduce exposure time or intensity, or switch to a more stable dye.
- Photobleaching can also be utilized as an analytical tool. In techniques like FRAP (fluorescence recovery after photobleaching), a region of the specimen is intentionally photobleached, and the recovery of fluorescence indicates the diffusion rate of fluorescent molecules in that area.[2]
Care of a Fluorescence Microscope
- Protection from Contaminants
- Dust and other contaminants can adversely affect the performance of a fluorescence microscope. Therefore, always cover the microscope with a dust cover when it’s not in use to protect it from external contaminants.
- Handling Optical Components
- The optical components of a fluorescence microscope are delicate and sensitive. Avoid touching these components with bare hands. Instead, always use non-powdered gloves when handling them to prevent any contamination or damage.
- The optics within the filter cube are particularly sensitive to scratches and contamination. Handle these with utmost care to ensure their longevity and optimal performance.
- Orientation of Optical Components
- When replacing or installing optical components, it’s essential to be aware of their correct orientation in relation to the light path. Some components, like the dichroic mirror, may have specific markings indicating the correct side that should face the light path. Always refer to the manufacturer’s guidelines or markings to ensure proper orientation.
- Cleaning Procedures
- If any optical components become dirty or smudged, it’s crucial to clean them using methods recommended by the microscope’s manufacturer. Avoid using abrasive materials or inappropriate solvents that might damage the optics.
- Avoid Physical Impact
- Bumping or jostling the microscope can knock the optical elements out of alignment, affecting the quality of the images produced. Ensure that the microscope is placed on a stable surface and avoid any physical impact to maintain its alignment and functionality.
- Professional Maintenance
- Some alignment and maintenance tasks are intricate and require specialized knowledge. It’s recommended to have these tasks performed only by trained technicians to ensure the microscope’s optimal performance and longevity.[2]
Application of Fluorescence Microscope
- Identification of Structures in Biological Samples
- Fluorescence microscopy is extensively used to identify and visualize structures within both fixed and live biological samples.
- By using specific fluorescent dyes or fluorophores, researchers can target and highlight specific components within a cell or tissue, allowing for detailed structural analysis.
- Multicolor Staining and Labeling
- One of the significant advantages of fluorescence microscopy is the ability to use multicolor staining.
- This means that multiple structures or molecules can be labeled with different fluorophores, each emitting light at a distinct wavelength. Therefore, researchers can simultaneously visualize and differentiate multiple targets within a single sample.
- Viability Studies on Cell Populations
- Fluorescence microscopy can be used to determine the viability of cell populations.
- By using specific fluorescent markers that differentiate between live and dead cells, researchers can assess the health and viability of a cell population, which is crucial in studies related to cell growth, drug treatments, and toxicology.
- Imaging Genetic Material
- The technique is particularly useful for visualizing genetic material within cells, such as DNA and RNA.
- By using fluorophores that bind specifically to these genetic materials, researchers can study the organization, replication, and transcription processes of genes within cells.
- Selective Visualization using FISH
- Fluorescence in situ hybridization (FISH) is a specialized application of fluorescence microscopy.
- FISH allows researchers to detect and locate specific DNA sequences within chromosomes. Besides, it can be used to view specific cells within a larger population, making it invaluable in genetic and cytogenetic research.[1, 2, 3]
Advantages of Fluorescence Microscope
Fluorescence microscopy has emerged as a pivotal tool in the field of life sciences due to its unique capabilities and advantages over traditional optical imaging techniques. Here are the primary benefits of using fluorescence microscopy:
- High Specificity in Live-Cell Imaging
- Fluorescence microscopy stands out as the preferred method for studying dynamic behaviors in live-cell imaging.
- The technique’s ability to target and visualize specific proteins or structures within a cell in real-time provides researchers with invaluable insights into cellular processes and dynamics.
- Isolation of Individual Proteins
- One of the significant strengths of fluorescence microscopy is its ability to isolate individual proteins with high specificity amidst non-fluorescing material.
- This level of precision ensures that only the desired targets are visualized, reducing background noise and enhancing image clarity.
- High Sensitivity
- The sensitivity of fluorescence microscopy is remarkably high, with the capability to detect as few as 50 molecules per cubic micrometer.
- This high sensitivity allows for the visualization of minute quantities of specific molecules, making it possible to study low-abundance proteins or structures within cells.
- Multicolor Staining
- Fluorescence microscopy offers the advantage of multicolor staining, where different molecules can be labeled with different fluorophores.
- This capability allows for the simultaneous tracking of multiple types of molecules within a single sample, providing a comprehensive view of cellular interactions and processes.
- Superiority Over Other Optical Imaging Techniques
- When compared to other optical imaging methods, fluorescence microscopy offers clear advantages in terms of specificity, sensitivity, and versatility.
- Both in vitro (outside a living organism) and in vivo (within a living organism) imaging benefit from the enhanced capabilities of fluorescence microscopy, making it a preferred choice for many researchers.[1, 2, 3, 10, 8, 9, 11]
Limitations of Fluorescence Microscope
While fluorescence microscopy offers numerous advantages in biological research, it is not without its limitations. Understanding these constraints is crucial for researchers to make informed decisions and interpret results accurately. Here are the primary limitations of using fluorescence microscopy:
- Photobleaching
- One of the significant challenges faced in fluorescence microscopy is photobleaching. As fluorophores are illuminated, they tend to lose their ability to fluoresce.
- This loss of fluorescence is due to the accumulation of chemical damage from the electrons excited during the fluorescence process. Therefore, over time, the intensity of the fluorescence signal diminishes, which can affect the accuracy and reliability of the observations.
- Phototoxicity
- Cells, especially when exposed to short-wavelength light, are susceptible to phototoxicity. This means that the light used in fluorescence microscopy can potentially harm or damage the cells being observed.
- Besides, fluorescent molecules have a propensity to generate reactive chemical species when under illumination. This enhances the phototoxic effect, further increasing the risk of cellular damage during imaging.
- Limited Observation of Specific Structures
- Unlike other microscopy techniques that rely on transmitted and reflected light, fluorescence microscopy is limited to observing only those structures that have been specifically labeled for fluorescence.
- This means that any structure or component within a sample that has not been labeled with a fluorophore will remain invisible under fluorescence microscopy. As a result, researchers might miss out on observing certain crucial aspects of a specimen that are not fluorescently labeled.
- Reactive Chemical Species Generation
- The illumination of fluorescent molecules can lead to the generation of reactive chemical species. These reactive species can interact with other molecules within the sample, potentially altering their properties or functions.
- This can introduce artifacts or errors in the observations, especially if the reactive species interfere with the biological processes being studied.[1, 2, 3, 10, 8, 9, 11]
Resolution of fluorescence microscope
- The resolution of a fluorescence microscope refers to its ability to distinguish fine details within a sample. Resolution is typically measured in terms of the smallest distance between two points that can be distinguished as separate entities by the microscope.
- In fluorescence microscopy, the resolution is often limited by the wavelength of the excitation light and the numerical aperture (NA) of the objective lens. The shorter the wavelength and the higher the NA, the higher the resolution of the microscope.
- There are several factors that can affect the resolution of a fluorescence microscope, including the quality of the objective lens, the quality of the fluorophore, and the quality of the imaging system.
- There are several techniques that can be used to improve the resolution of a fluorescence microscope, including superresolution microscopy techniques such as stimulated emission depletion (STED) microscopy, structured illumination microscopy (SIM), and single-molecule localization microscopy (SMLM). These techniques use specialized optics and image processing algorithms to achieve resolution beyond the diffraction limit of conventional microscopes.
- Overall, the resolution of a fluorescence microscope can range from several micrometers for low-resolution microscopes to a few nanometers for high-resolution microscopes using superresolution techniques.[1, 2, 3, 10, 8, 9, 11]
Fluorescence microscope magnification
- The magnification of a fluorescence microscope refers to the size of the image of a sample as it appears through the microscope compared to its actual size. Magnification is typically expressed as a ratio, such as 10x or 100x.
- In fluorescence microscopy, the magnification of the microscope is determined by the combination of the objective lens and the eyepiece. The objective lens is responsible for collecting and focusing light from the sample, while the eyepiece magnifies the image produced by the objective lens.
- The magnification of a fluorescence microscope can be adjusted by changing the objective lens or the eyepiece. Most fluorescence microscopes come with a range of objective lenses with different magnifications, such as 4x, 10x, 40x, and 100x, allowing the user to choose the appropriate magnification for their sample.
- The magnification of a fluorescence microscope is an important consideration when choosing a microscope, as it determines the level of detail that can be resolved in the image of the sample. Higher magnification microscopes can provide more detailed images, but may have a smaller field of view and may be more susceptible to chromatic aberrations. It is important to choose a microscope with the appropriate magnification for your specific research needs.[1, 2, 3, 10, 8, 9, 11]
Fluorescence Microscopy Images
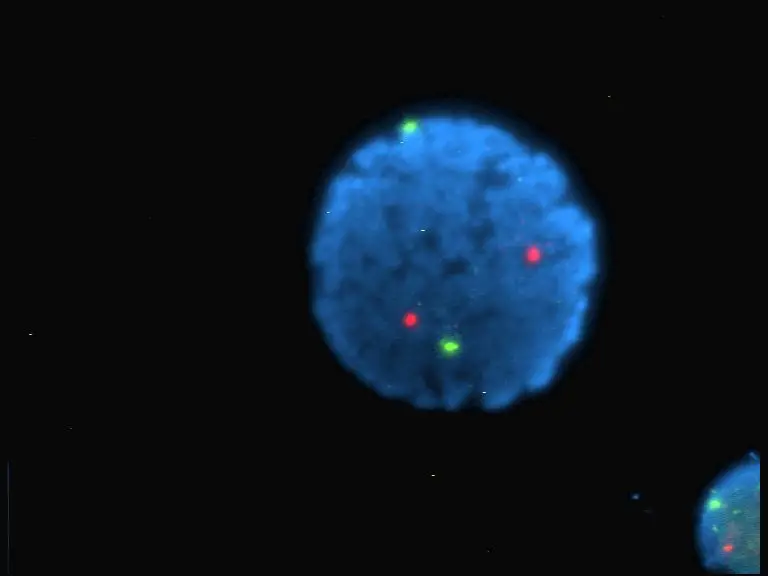
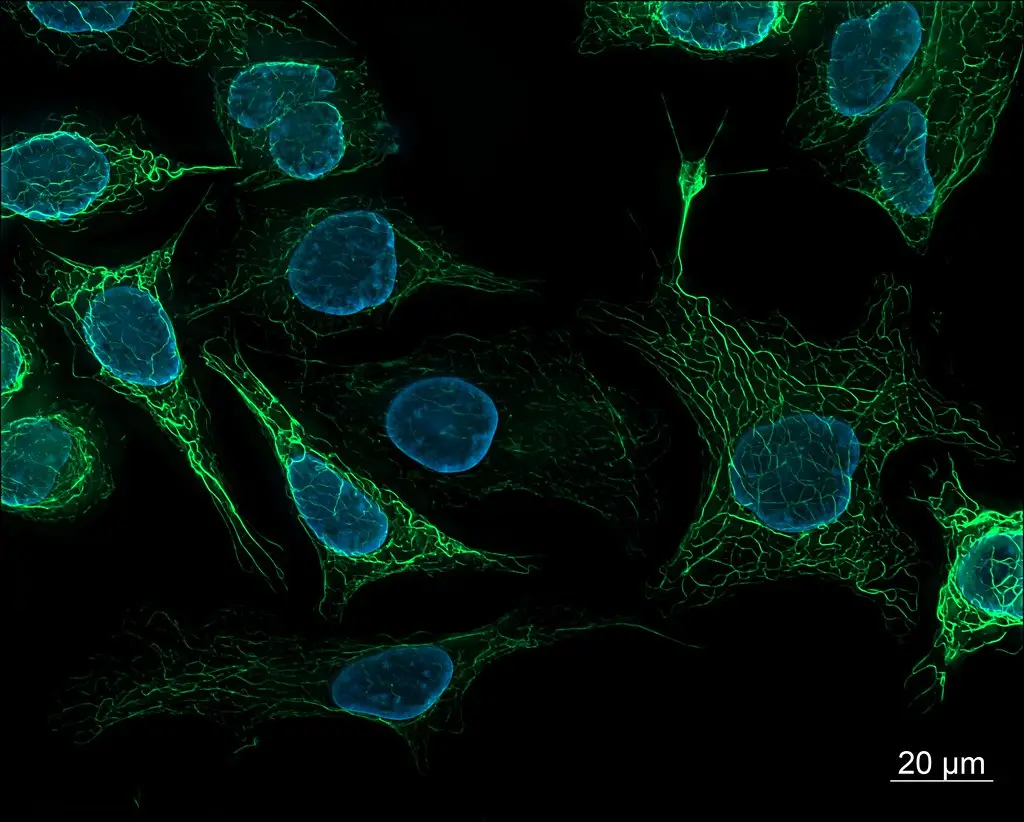
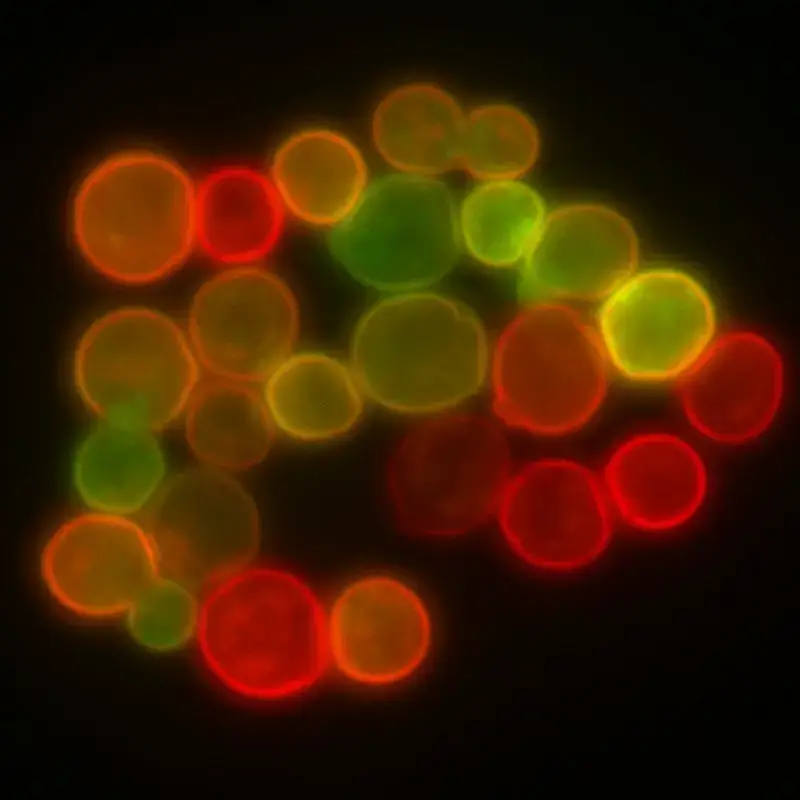
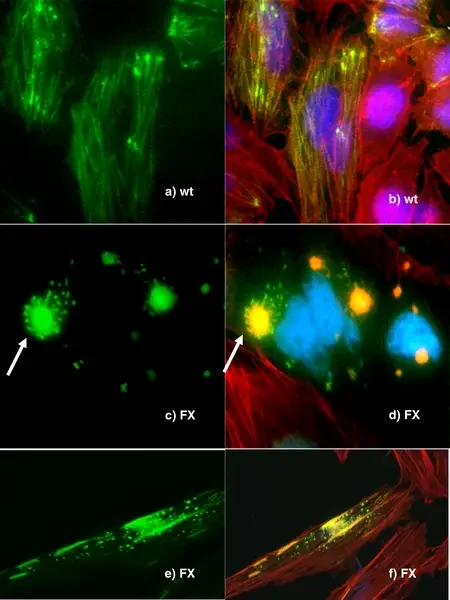
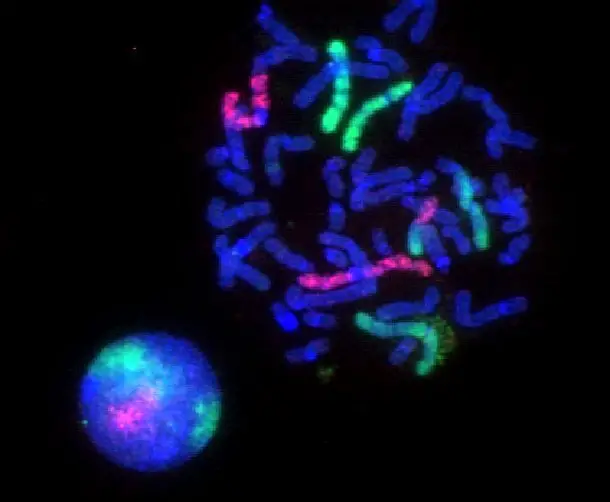
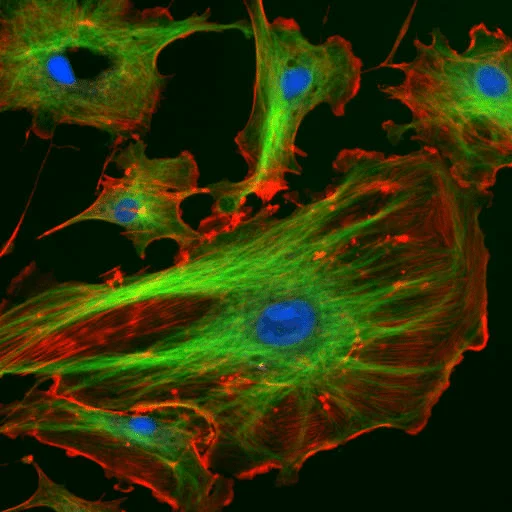
Examples
Zeiss fluorescence microscope
- Zeiss is a well-known manufacturer of high-quality optical instruments, including fluorescence microscopes. Zeiss fluorescence microscopes are widely used in many different fields, including biology, medicine, and materials science.
- Zeiss fluorescence microscopes typically use epifluorescence or total internal reflection fluorescence (TIRF) techniques to visualize fluorescence in samples. They offer high sensitivity and resolution, and can be used to visualize a wide range of fluorophores, including dyes, proteins, and nanoparticles.
- Zeiss fluorescence microscopes are also equipped with advanced features such as automated focusing, high-speed imaging, and multi-channel imaging capabilities, which allow for the simultaneous visualization of multiple fluorophores.
- Zeiss fluorescence microscopes are available in a range of configurations, including upright, inverted, and superresolution models, to suit the needs of different research applications. They are also compatible with a wide range of imaging software and accessories, such as camera systems and stage-mounted incubators, which allow for the acquisition and analysis of high-quality fluorescence images.
Nikon fluorescence microscope
- Nikon is a well-known manufacturer of high-quality optical instruments, including fluorescence microscopes. Nikon fluorescence microscopes are widely used in many different fields, including biology, medicine, and materials science.
- Nikon fluorescence microscopes typically use epifluorescence or total internal reflection fluorescence (TIRF) techniques to visualize fluorescence in samples. They offer high sensitivity and resolution, and can be used to visualize a wide range of fluorophores, including dyes, proteins, and nanoparticles.
- Nikon fluorescence microscopes are also equipped with advanced features such as automated focusing, high-speed imaging, and multi-channel imaging capabilities, which allow for the simultaneous visualization of multiple fluorophores.
- Nikon fluorescence microscopes are available in a range of configurations, including upright, inverted, and superresolution models, to suit the needs of different research applications. They are also compatible with a wide range of imaging software and accessories, such as camera systems and stage-mounted incubators, which allow for the acquisition and analysis of high-quality fluorescence images.
Evos fluorescence microscope
- The EVOS fluorescence microscope is a brand of microscope produced by Thermo Fisher Scientific, a leading manufacturer of scientific instrumentation. The EVOS fluorescence microscope is a versatile, high-performance microscope that is widely used in many different fields, including biology, medicine, and materials science.
- The EVOS fluorescence microscope uses epifluorescence or total internal reflection fluorescence (TIRF) techniques to visualize fluorescence in samples. It offers high sensitivity and resolution, and can be used to visualize a wide range of fluorophores, including dyes, proteins, and nanoparticles.
- The EVOS fluorescence microscope is equipped with advanced features such as automated focusing, high-speed imaging, and multi-channel imaging capabilities, which allow for the simultaneous visualization of multiple fluorophores. It is also compatible with a range of imaging software and accessories, such as camera systems and stage-mounted incubators, which allow for the acquisition and analysis of high-quality fluorescence images.
- Overall, the EVOS fluorescence microscope is a powerful and versatile tool for studying the structure and function of cells, tissues, and molecules at the microscopic level.
Confocal vs Fluorescence Microscopy
- Basic Principle:
- Fluorescence Microscopy: Utilizes fluorescent dyes or proteins to visualize specific structures within a sample. The sample is illuminated with a specific wavelength of light, causing the fluorescent molecules to emit light of a different wavelength, which is then detected to form an image.
- Confocal Microscopy: A specialized form of fluorescence microscopy. It uses point illumination and a pinhole in an optically conjugate plane in front of the detector to eliminate out-of-focus light, which results in sharper images.
- Depth of Field:
- Fluorescence Microscopy: Has a larger depth of field, capturing both in-focus and out-of-focus light, which can result in blurry images especially in thick specimens.
- Confocal Microscopy: Has a shallow depth of field, allowing for optical sectioning. Only the light from a specific focal plane is detected, providing clear images of thin sections within thicker specimens.
- Resolution:
- Fluorescence Microscopy: Offers good resolution but can be limited due to the collection of out-of-focus light.
- Confocal Microscopy: Provides enhanced resolution and contrast compared to standard fluorescence microscopy because it eliminates out-of-focus light.
- Applications:
- Fluorescence Microscopy: Suitable for observing the location and movement of fluorescently labeled structures or molecules within cells.
- Confocal Microscopy: Ideal for imaging thicker specimens, three-dimensional reconstructions, and capturing detailed structures within cells or tissues.
- Complexity and Cost:
- Fluorescence Microscopy: Generally simpler and less expensive than confocal systems.
- Confocal Microscopy: More complex due to the need for additional components like the pinhole and laser scanning system, making it more expensive.
- Sample Damage:
- Fluorescence Microscopy: The entire specimen is illuminated, which can lead to more extensive photobleaching and photodamage.
- Confocal Microscopy: Only the focal plane is illuminated, reducing photobleaching and photodamage in out-of-focus regions.
- Image Processing:
- Fluorescence Microscopy: Images might require post-processing to enhance contrast or reduce blur in some cases.
- Confocal Microscopy: Produces clearer images with less need for post-processing, and allows for the reconstruction of three-dimensional images from serial optical sections.
FAQ
Q1. When do we use a fluorescence microscope?
Fluorescence microscopy is a widely used technique in many different fields, including biology, medicine, and materials science. Some common applications of fluorescence microscopy include:
- Cell biology: Fluorescence microscopy is frequently used to study the structure and function of cells and their components, such as organelles, proteins, and nucleic acids.
- Molecular biology: Fluorescence microscopy can be used to visualize and study specific molecules within a sample, such as DNA, RNA, or proteins.
- Developmental biology: Fluorescence microscopy is commonly used to study the development and differentiation of cells and tissues in living organisms.
- Neuroscience: Fluorescence microscopy is used to study the structure and function of neurons and the connections between them.
- Drug discovery and development: Fluorescence microscopy can be used to study the mechanisms of action of drugs and to identify potential new drugs.
- Materials science: Fluorescence microscopy is used to study the properties and behavior of materials at the microscale.
- Environmental science: Fluorescence microscopy is used to study the presence and distribution of contaminants in water, soil, and air.
Overall, fluorescence microscopy is a powerful and versatile tool for studying the structure and function of cells, tissues, and molecules at the microscopic level.
Q2. Fluorescence microscope price?
The price of a fluorescence microscope can vary widely depending on the manufacturer, model, and features. Entry-level fluorescence microscopes may cost several thousand dollars, while more advanced models with additional features and capabilities can cost tens of thousands of dollars or more.
Some factors that can affect the price of a fluorescence microscope include:
- Type of microscope: Upright microscopes are generally less expensive than inverted microscopes, which are typically more expensive due to their more complex design.
- Magnification: Higher magnification microscopes are generally more expensive than those with lower magnification.
- Resolution: Higher resolution microscopes are generally more expensive than those with lower resolution.
- Fluorophores: Fluorescence microscopes that can visualize a wide range of fluorophores are generally more expensive than those that are limited to a specific range of fluorophores.
- Additional features: Fluorescence microscopes with advanced features such as automated focusing, high-speed imaging, or multi-channel imaging capabilities may be more expensive than those without these features.
Overall, the price of a fluorescence microscope can range from several thousand dollars for an entry-level model to tens of thousands of dollars or more for a high-end model with advanced features. It is important to consider the specific requirements and budget of your research when choosing a fluorescence microscope.
References
- Sanderson MJ, Smith I, Parker I, Bootman MD. Fluorescence microscopy. Cold Spring Harb Protoc. 2014 Oct 1;2014(10):pdb.top071795. doi: 10.1101/pdb.top071795. PMID: 25275114; PMCID: PMC4711767.
- https://conductscience.com/fluorescence-microscopy/
- https://serc.carleton.edu/microbelife/research_methods/microscopy/fluromic.html
- https://www.thermofisher.com/in/en/home/life-science/cell-analysis/cellular-imaging/evos-cell-imaging-systems.html
- https://microscopeinternational.com/fluorescence-microscopy/
- https://www.microscopyu.com/techniques/fluorescence/introduction-to-fluorescence-microscopy
- https://www.semrock.com/introduction-to-fluorescence-filters.aspx
- https://www.slideshare.net/VIVEKKUMARSINGH109/fluorescence-microscopy-72205439
- https://www.slideshare.net/doctorrao/fluorescent-microscopy
- https://en.wikipedia.org/wiki/Fluorescence_microscope
- http://www.scholarpedia.org/article/Fluorescent_proteins
- https://pubmed.ncbi.nlm.nih.gov/18228363/
- https://www.ncbi.nlm.nih.gov/pmc/articles/PMC4711767/