A microscope is a scientific instrument that is used to magnify the image of an object or sample in order to study its structure or features in detail. Microscopes are commonly used in scientific and medical research, as well as in education and other applications, to study small structures and organisms that are not visible to the naked eye.
There are several different types of microscopes, including simple microscopes, compound microscopes, and electron microscopes. Simple microscopes, also known as monocular microscopes, have only one lens and are typically used for low-magnification applications. Compound microscopes have multiple lenses and are capable of higher magnifications and better resolution, and they are commonly used in scientific and medical research. Electron microscopes use a beam of electrons instead of visible light to magnify the image of an object, and they are capable of achieving extremely high magnifications and resolutions.
Microscopes are essential tools for scientific research and have made many important contributions to our understanding of the natural world. They have helped to reveal the existence of microorganisms, enabled the study of cells and tissues, and opened up new areas of research that have led to many of the scientific and medical advances we enjoy today.
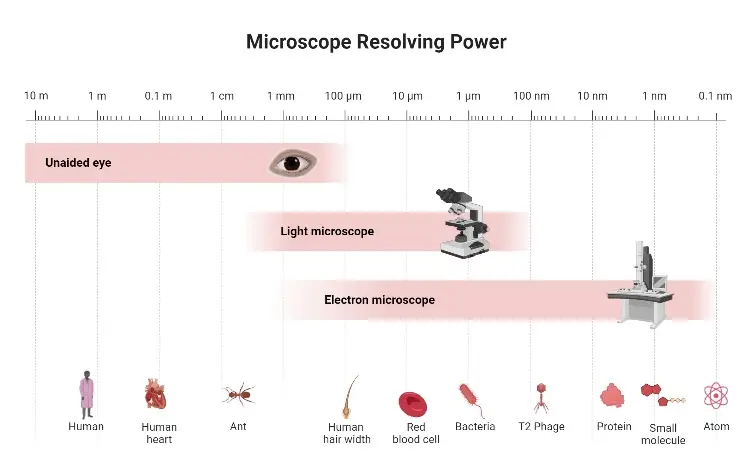
History of Microscope
The history of the microscope can be traced back to the late 16th century, when the first simple microscopes were developed. These early microscopes were simple refracting telescopes that used a converging lens to magnify the image of an object.
The first compound microscopes, which have multiple lenses and are capable of higher magnifications and better resolution, were developed in the early 17th century. The Dutch scientist Antonie van Leeuwenhoek is credited with building the first compound microscopes, which he used to study living cells in detail. His observations revolutionized our understanding of biology and laid the foundation for modern microscopy.
In the 19th century, advances in microscopy led to the development of new types of microscopes, including the polarizing microscope, the phase-contrast microscope, and the fluorescence microscope. These microscopes made it possible to study the properties of light and to examine samples in greater detail than was possible with earlier microscopes.
In the 20th century, the development of the electron microscope, which uses a beam of electrons instead of visible light to magnify the image of an object, enabled scientists to study even smaller structures and organisms with unprecedented resolution. Today, microscopes are essential tools for scientific research and have made many important contributions to our understanding of the natural world.
Different Types of Microscopes
A microscope is an optical instrument used in the laboratory for examining the small objects which we can’t see in the naked eye. There are present different types of microscope, which we are using for different purposes. Microscopes are classified based on their working principle, application.
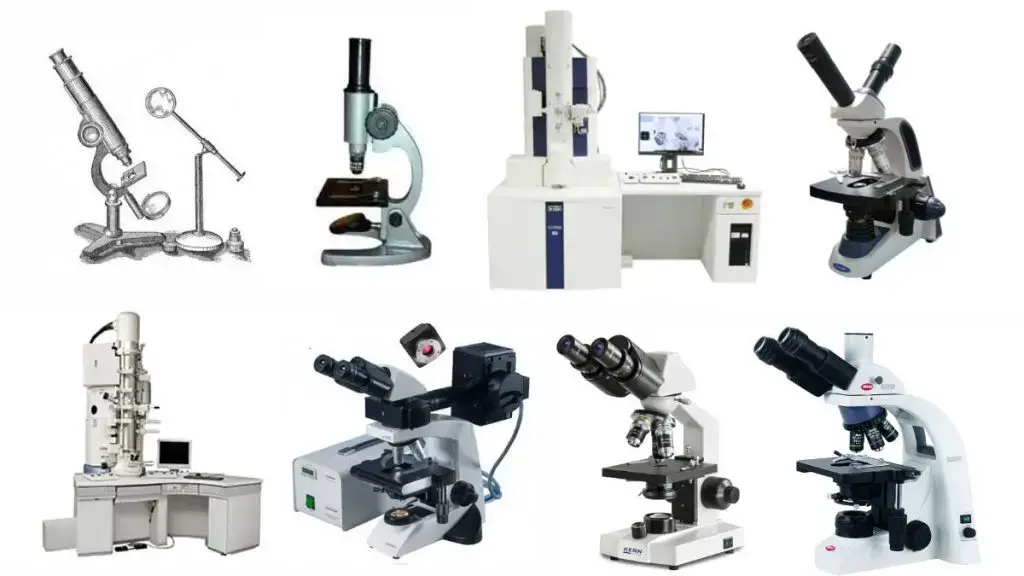
There are mainly present two types of microscope, such as;
- Simple Microscope
- Compound Microscope
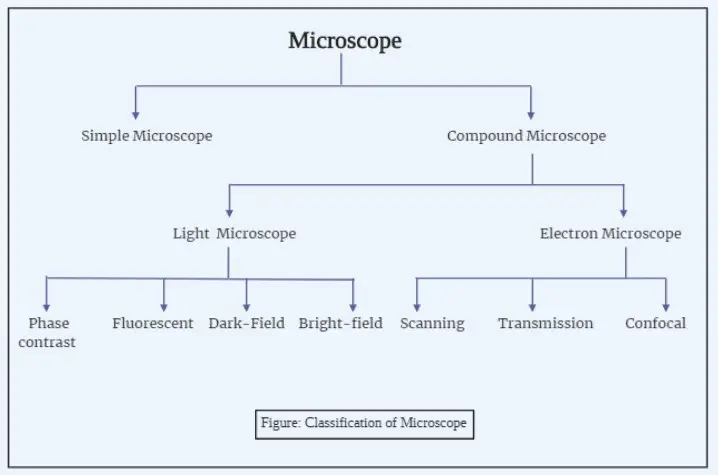
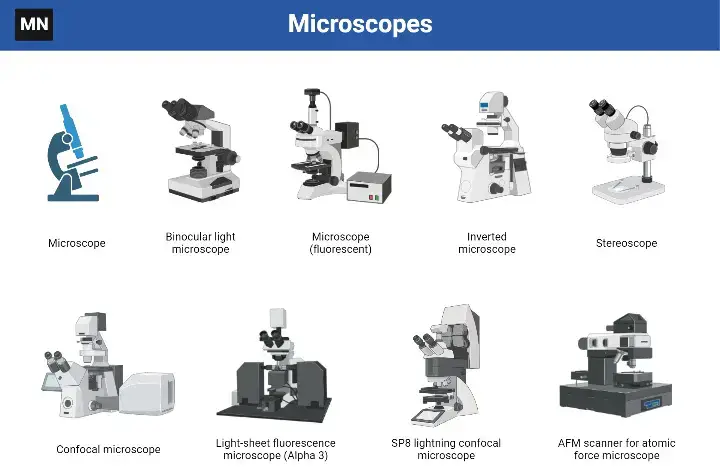
1. Simple Microscope
A simple microscope, often referred to as a magnifying instrument, is characterized by its singular double convex lens with a notably short focal length. Instruments such as the hand lens and reading lens fall under this category. Scientifically, when an object is positioned proximate to the lens, an image is generated. This image, in contrast to the original object, is both erect and magnified. It is imperative to note that the image produced is virtual in nature. Unlike real images, it cannot be projected onto a screen.
From a technical standpoint, the magnifying capability of a simple microscope can be quantified using a specific formula:
Magnifying Power=D/F
In this equation:
- D represents the minimum distance at which an object can be clearly perceived, commonly known as the least distance of distinct vision.
- F denotes the focal length of the double convex lens.
In summation, the simple microscope is a fundamental tool in the realm of optics, offering a magnified view of objects through its unique lens configuration. Its scientific relevance is underscored by the precise mathematical relationship that governs its magnifying prowess.
Working Principle of simple Microscope
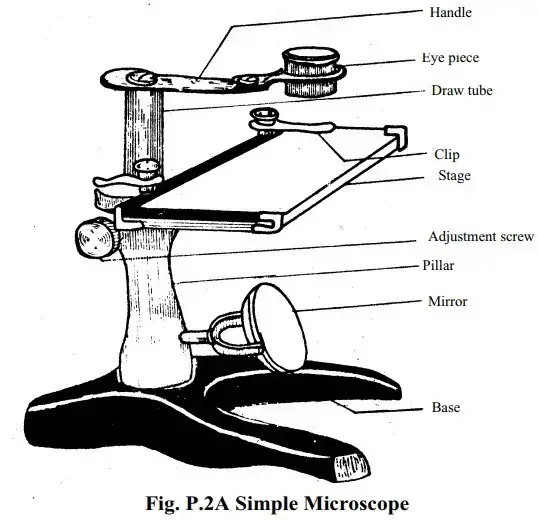
The simple microscope operates on a foundational optical principle. When a specimen is strategically positioned at the focal point of the microscope’s convex lens, the resultant image is virtual, upright, and enlarged. This image is discerned at the minimum distance wherein clear vision is attainable, often referred to as the least distance of distinct vision.
Delving into the structural components of a simple microscope, several key elements play pivotal roles in its functionality:
- Mirror as Illuminator: This component serves to direct and enhance light onto the specimen, ensuring optimal visibility and clarity during observation.
- Convex Lens: The heart of the microscope, this lens is responsible for the magnification process. Its curvature facilitates the bending of light rays, leading to the formation of a magnified virtual image of the specimen.
- Stage: A platform where the specimen is placed for examination. It provides stability and ensures the specimen remains in the optimal position relative to the lens.
- Metallic Stand with Base: This structural element provides the necessary support and stability to the entire microscope apparatus, ensuring precise and undisturbed observations.
Applications of Simple Microscope
The simple microscope, a fundamental instrument in the realm of optics, boasts a myriad of applications across diverse scientific and technical domains. Its ability to magnify minute details renders it indispensable in various fields. Herein, we delineate its primary uses:
- Morphological Studies: The simple microscope is quintessential in the study of the morphology of various organisms. It facilitates detailed examinations of insects, algae, and fungi, allowing researchers to discern their structural intricacies and adaptations.
- Pedological Analysis: In the domain of pedology, the simple microscope is employed to investigate soil types and their constituent components. Such analyses are pivotal in understanding soil health, fertility, and suitability for agricultural endeavors.
- Microdevice Repairs: Technical workshops specializing in electronics frequently utilize simple microscopes. These instruments are indispensable in the repair and maintenance of intricate devices such as watches, mobile phones, and other micro-components. Their magnifying capability ensures precision and accuracy in such delicate operations.
- Gemological Assessments: Jewelers rely on the simple microscope to ascertain the quality and authenticity of precious gemstones, including diamonds and rubies. The instrument allows for a meticulous examination of facets, inclusions, and other minute details, ensuring the gem’s value and quality are accurately determined.
- Detailed Script and Engraving Analysis: Historical manuscripts, engravings, and scripts with diminutive letters often require magnification for proper study and interpretation. The simple microscope serves as an invaluable tool in such endeavors, revealing details that might otherwise remain obscured.
In summation, the simple microscope, with its magnifying prowess, finds extensive application across a spectrum of scientific, technical, and artisanal fields. Its versatility underscores its significance in both research and practical domains.
Advantages of Simple Microscope
- Low cost: Simple microscopes are relatively inexpensive compared to more advanced models.
- Easy to use: Simple microscopes are designed to be easy to use, even for people who are new to microscopy.
- Portable: Simple microscopes are small and lightweight, making them easy to take with you wherever you go.
- Great for educational purposes: Simple microscopes are often used in schools and other educational settings to introduce students to the principles of microscopy.
Disadvantages of Simple Microscope
The simple microscope, while a foundational instrument in the field of optics, is not without its constraints. As with any scientific tool, understanding its limitations is paramount for its effective utilization. Here, we elucidate the primary constraints associated with the simple microscope:
- Restricted Magnification: One of the most pronounced limitations of the simple microscope is its constrained magnifying capability. Typically, its magnification potential is capped at approximately 10X. This limitation often precludes the detailed study of ultra-microscopic entities.
- Illumination and Stage Constraints: The simple microscope employs a mirror for illumination purposes. This rudimentary approach can sometimes result in suboptimal lighting conditions for the specimen under observation. Additionally, the absence of a mechanical stage can pose challenges in maneuvering and positioning the specimen with precision.
- Specimen Requirements: For optimal visibility under a simple microscope, specimens often need to be thinly sliced and appropriately stained. This requirement can be cumbersome and might not be feasible for all types of samples, potentially limiting the range of specimens that can be effectively observed.
- Resolution and Contrast Limitations: The resolution, which refers to the ability of the microscope to distinguish between two closely situated points, is notably low in simple microscopes. Coupled with this is the issue of diminished image contrast, which can impede the clear differentiation of specimen details.
In conclusion, while the simple microscope is a valuable tool in various applications, it is imperative for users to be cognizant of its inherent limitations. Such awareness ensures that the instrument is employed within its optimal capacity, thereby yielding the most accurate and informative observations.
2. Compound Microscope
The compound microscope, often referred to as a “biological microscope,” stands as a cornerstone in the realm of optical instruments. Distinct from its simple counterpart, the compound microscope boasts a sophisticated design encompassing multiple lenses, specifically an objective lens and an ocular lens, to achieve its magnification.
Structural and Functional Attributes:
- Multiple Objectives: A salient feature of the compound microscope is its revolving ring housing multiple objectives. This design allows users to seamlessly switch between different magnification powers, adapting to the specific requirements of the observation.
- Magnification Capabilities: The magnification range of a compound microscope is notably expansive, often spanning from 40x to 1000x. While some claims suggest even higher magnifications, it’s scientifically acknowledged that resolution tends to deteriorate significantly beyond this range.
- Magnification Formula: The magnifying power of a compound microscope is a product of the magnifying capabilities of both the objective and ocular lenses. This relationship is mathematically represented as: m=D/f0×L/fe Where:
- m denotes the magnifying power.
- D represents the least distance of distinct vision.
- L signifies the length of the tube.
- fe is the focal length of the ocular lens.
- f0 corresponds to the focal length of the objective lens.
Applications and Sample Preparation: The compound microscope finds extensive application in myriad biological disciplines, including medicine, microbiology, pathology, hematology, and molecular biology, to name a few. Given its high magnification capabilities, it is particularly adept at examining specimens invisible to the unaided eye, such as blood cells, parasites, germs, and thin tissue sections.
For effective observation, specimens are meticulously prepared on slides, often secured with a cover slip. This ensures the sample remains flat and stable during examination. Educational institutions, especially at advanced levels, frequently provide students with pre-prepared slides, embedding samples permanently between layers for ease of study.
In summation, the compound microscope, with its intricate lens system and unparalleled magnifying prowess, remains an indispensable tool in the scientific community. Its versatility and precision have cemented its status as a staple in both research and educational settings.
Working Principle of Compound Microscope
The compound microscope, a pivotal instrument in optical microscopy, operates based on a series of coordinated magnification events. Its functionality is rooted in the intricate interplay of light and lenses, resulting in a highly magnified representation of the specimen under observation. Here, we elucidate the step-by-step working principle of the compound microscope:
- Illumination and Specimen Interaction: The initial phase involves focusing light onto the specimen. This is achieved using a condenser, which directs and concentrates light onto the specimen positioned on the stage. As light interacts with the specimen, it undergoes transmission, with certain wavelengths being absorbed or refracted based on the specimen’s properties.
- Formation of the Primary Image: The transmitted light emerging from the specimen is then intercepted by the objective lens. This lens, with its specific focal length and curvature, magnifies the light’s pattern, forming an enlarged image within the body tube. This initial magnified representation is termed the primary image.
- Secondary Magnification: The primary image’s light trajectory is further manipulated as it traverses the body tube. Upon reaching the ocular lens, or the eyepiece, this image undergoes a second round of magnification. The ocular lens, designed to further enlarge the image, produces what is referred to as the secondary image.
- Final Image Projection: The culmination of these magnification events results in a highly amplified image, which is projected at the least distance of distinct vision. This ensures that the observer perceives a clear, detailed, and greatly magnified representation of the original specimen.
Compound Microscope Diagram
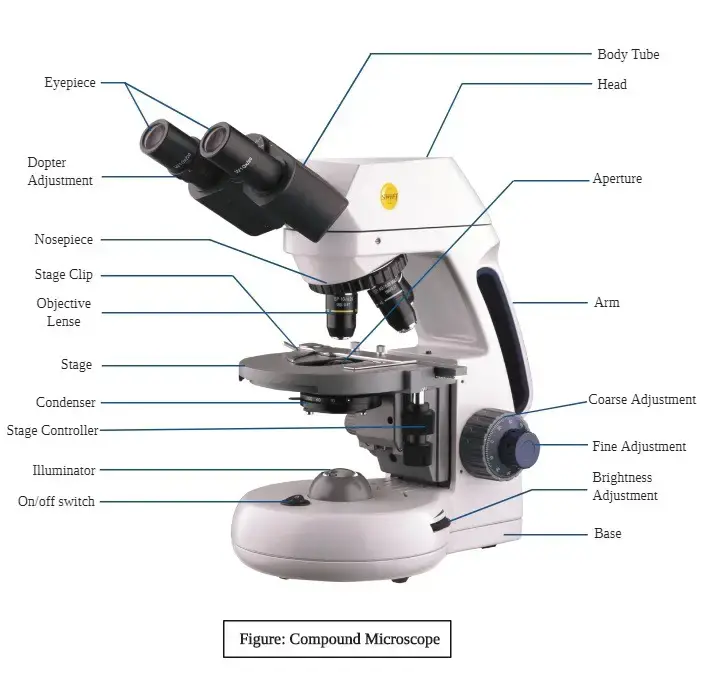
Compound Microscope Parts
The compound microscope, a sophisticated optical instrument, is composed of an array of meticulously designed components. Each part plays a pivotal role in the microscope’s functionality, ensuring precise and detailed observations. Herein, we delineate the primary components of the compound microscope:
- Illuminator (Light Source): This serves as the primary source of light, illuminating the specimen for observation. It ensures optimal visibility and clarity of the specimen under examination.
- Diaphragm (Iris): Positioned beneath the stage, the diaphragm regulates the amount of light reaching the specimen, allowing for adjustments in illumination intensity.
- Condenser: Situated below the diaphragm, the condenser focuses and directs the light onto the specimen, optimizing clarity and resolution.
- Condenser Focus Knob: This control allows for the vertical adjustment of the condenser, fine-tuning the light’s focus on the specimen.
- Rack Stop: A safety feature that prevents the objective lens from coming into direct contact with the slide, protecting both the lens and the specimen.
- Stage: The platform where the specimen slide is placed for examination. It provides stability and ensures optimal positioning relative to the lenses.
- Stage Control Knobs: These knobs facilitate the precise movement of the stage, allowing for the accurate positioning of the specimen under the objective lens.
- Nose Piece: A revolving component that houses the objective lenses, enabling users to switch between different magnification powers seamlessly.
- Objective Lens: These lenses, attached to the nose piece, are responsible for the initial magnification of the specimen.
- Tube (Head): The structural component connecting the eyepiece to the nose piece, facilitating the passage of light.
- Eyepiece (Ocular Lens): Positioned at the top of the tube, this lens further magnifies the image, providing the observer with a detailed view.
- Diopter Adjustment: This feature allows for the fine-tuning of the eyepiece lens, accommodating individual visual requirements.
- Adjustment Knobs: a. Fine Adjustment Knob: Enables minute adjustments in focus, refining the clarity of the image. b. Coarse Adjustment Knob: Facilitates larger, rapid adjustments in focus, particularly useful when initially positioning the specimen.
- Arm: The curved structure that supports the tube, providing stability and a grip for handling the microscope.
- Base: The foundational component that provides stability and support to the entire microscope apparatus.
- Light Switch: Activates or deactivates the illuminator, controlling the light source.
- Brightness Adjustment: Regulates the intensity of the illuminator, allowing for variations in illumination based on the specimen’s requirements.
In summation, the compound microscope, with its intricate assembly of parts, stands as a testament to the precision and sophistication inherent in optical instrumentation. Each component, meticulously designed and integrated, ensures the instrument’s efficacy in producing detailed and accurate observations.
Uses of Compound Microscope
The compound microscope, a paramount instrument in the realm of optical microscopy, boasts a myriad of applications across diverse scientific domains. Its ability to provide detailed magnification of minute specimens renders it indispensable in various fields of study. Herein, we delineate its primary uses:
- Microbiology: In the domain of microbiology, the compound microscope is quintessential for studying the morphology of microorganisms. It facilitates the detailed examination of the structural intricacies, adaptations, and behaviors of bacteria, viruses, fungi, and other microscopic entities.
- Histopathology: The compound microscope plays a pivotal role in histopathology, a discipline focused on the microscopic examination of tissues to study diseases. It allows pathologists to discern cytopathic effects, identify tumors, and analyze tissue samples for signs of disease or abnormalities.
- Cytology: Cytology, the study of cells, heavily relies on the compound microscope. This instrument enables researchers to delve into the cellular structure of various cell types, providing insights into cell morphology, organelle distribution, and cellular interactions.
- Biological Research: Biologists employ the compound microscope to observe and analyze slides containing cells, tissues, or specific segments of biological components. Whether studying plant cells, animal tissues, or other biological specimens, the microscope provides a magnified view, revealing details that are imperceptible to the naked eye.
In summation, the compound microscope, with its unparalleled magnifying prowess, finds extensive application in a spectrum of scientific disciplines. Its versatility and precision have cemented its status as an invaluable tool in both research and diagnostic settings, underscoring its significance in advancing our understanding of the microscopic world.
Advantages of Compound Microscope
- High magnification: Compound microscopes are capable of high magnification, making them suitable for observing very small or detailed specimens.
- Widely available: Compound microscopes are widely available and are often used in educational settings and laboratories.
- Easy to use: Compound microscopes are relatively simple and easy to use, even for people who are new to microscopy.
- Good for a variety of samples: Compound microscopes can be used to study a wide range of samples, including cells, tissues, bacteria, and small structures within materials.
Disadvantages of Compound Microscope
- Limited resolution: Compound microscopes often have a lower resolution compared to more advanced models, so they may not be able to distinguish between closely spaced objects or structures.
- Limited lighting options: Compound microscopes often have a single light source, which may not be sufficient for observing some specimens.
- Limited adjustments: Compound microscopes often have limited adjustments, such as the ability to focus or adjust the eyepieces, which may make it more difficult to obtain a clear image.
- Inaccurate measurements: Compound microscopes often have an inaccurate scale, which can make it difficult to accurately measure the size of objects or structures within the sample.
Classification of Compound Microscope
Compound Microscope is classified in two categories;
A Light Microscope
Light Microscope is further classified into four categories such as;
- Bright-field Microscope
- Dark-Field Microscope.
- Phase-contrast Microscope.
- Fluorescent Microscope.
B. Electron Microscope
Electron Microscope is further classified into three categories such as;
- Scanning Microscope
- Transmission Microscope
- Confocal Microscope
A. Light Microscope
- Light Microscopes use visible light and magnifying lenses to examine small objects not visible to the naked eye, or in finer detail than the naked eye allows. Magnification, however, is not the most important issue in microscopy.
They are classified in these following groups;
- Bright-field Microscope
- Dark-Field Microscope.
- Phase-contrast Microscope.
- Fluorescent Microscope.
1. Bright-field Microscope
- In a bright-field microscope, the specimen appears as dark against the bright background.
- A bright-field microscope is a type of light microscope that uses visible light to illuminate a sample and a system of lenses to magnify the image. In a bright-field microscope, the light source is located below the stage, and the sample is illuminated from below.
- The light passes through the transparent or semi-transparent sample and is focused by the objective lens onto the eyepiece. This produces a bright image against a dark background, which makes it easy to see the details of the sample.
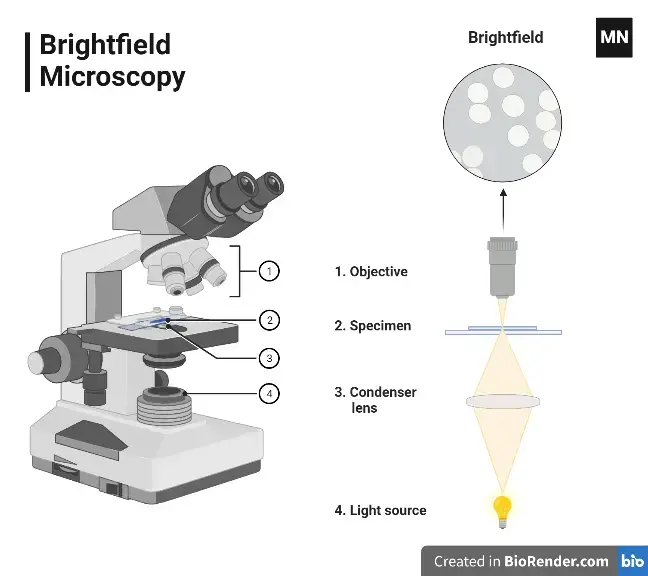
- Bright-field microscopes are the most common type of light microscope and are widely used in a variety of fields, including biology, medicine, and materials science. They are often used to study cells, tissues, and small structures within materials.
- One of the advantages of bright-field microscopy is that it is relatively simple and easy to use. It is also relatively inexpensive compared to other types of microscopes.
- However, bright-field microscopy has some limitations, including poor contrast for transparent or colorless samples, and the inability to distinguish between closely spaced objects or structures. To overcome these limitations, other types of microscopy, such as phase contrast microscopy or fluorescence microscopy, may be used.
Applications of Bright-field Microscope
- They are used in the laboratory for studying the outer structure of microorganisms.
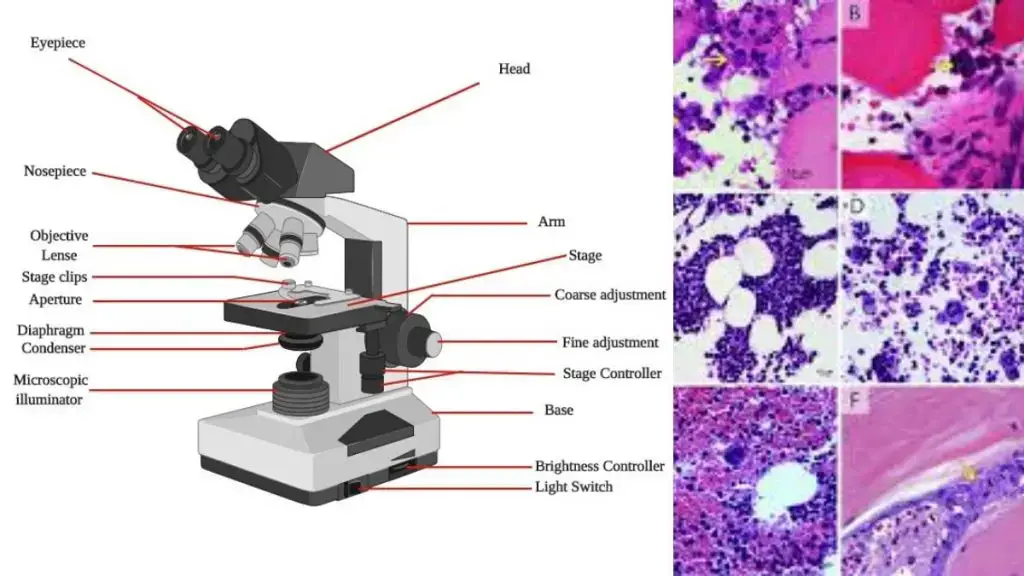
Advantages and Disadvantages of Bright-field Microscope
Advantages:
- Widely available: Bright-field microscopes are widely available and are often used in educational settings and laboratories.
- Easy to use: Bright-field microscopes are relatively simple and easy to use, even for people who are new to microscopy.
- Inexpensive: Bright-field microscopes are generally less expensive compared to other types of microscopes.
- Good for opaque or semi-transparent samples: Bright-field microscopy is well-suited for studying opaque or semi-transparent samples, such as cells, tissues, and small structures within materials.
Disadvantages:
- Poor contrast for transparent or colorless samples: Bright-field microscopy may not provide sufficient contrast for transparent or colorless samples, such as bacteria or algae.
- Limited detail: Bright-field microscopy may not be able to distinguish between closely spaced objects or structures, which limits its ability to observe fine details.
- Limited lighting options: Bright-field microscopes often have a single light source, which may not be sufficient for observing some samples.
- Limited adjustments: Bright-field microscopes often have limited adjustments, such as the ability to focus or adjust the eyepieces, which may make it more difficult to obtain a clear image.
2. Dark-Field Microscope
The Dark-Field Microscope stands as a specialized variant of the conventional light microscope, distinguished by its unique illumination technique and resultant imaging characteristics. Here, we delve into the intricacies and applications of this advanced optical instrument:
Fundamental Design and Working Principle: The Dark-Field Microscope employs a modified illumination system. Central to this system is an opaque disc positioned beneath the condenser lens. This disc serves to obstruct direct light from the source, preventing it from reaching the objective lens. Instead, only the light scattered by the specimen is captured. As a result, the specimen is illuminated laterally, from the side, at a shallow angle. The scattered light is then focused by the objective lens onto the eyepiece, producing an image where the specimen appears luminous against a contrasting dark background.
Imaging Characteristics:
- High Contrast and Resolution: One of the standout features of the dark-field microscope is its ability to produce images with heightened contrast and resolution compared to bright-field microscopy. This is particularly beneficial for observing minute details in specimens.
- Unstained Specimen Observation: A significant advantage of dark-field microscopy is the elimination of the need for staining specimens. This facilitates the observation of specimens in their natural state, preserving their inherent characteristics.
- Bright Specimen against Dark Background: The resultant images showcase specimens as bright entities juxtaposed against a dark, contrasting background, offering a clear and detailed view.
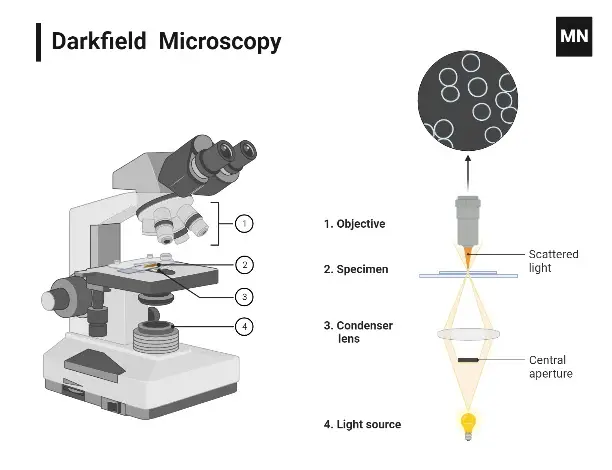
Uses of Dark Field Microscope
The Dark-Field Microscope, a specialized variant of light microscopy, boasts a myriad of applications across diverse scientific and technological domains. Its unique illumination technique, which emphasizes scattered light, renders it particularly adept at revealing details often obscured in other microscopy forms. Herein, we delineate its primary uses:
- Microbiology:
- Microbial Motility: Dark-field microscopy is invaluable in observing the motility of microorganisms, offering insights into their movement patterns and behaviors.
- Spirochetes and Thin Bacteria: The technique is especially adept at visualizing very thin bacteria, including spirochetes, which are often challenging to observe under conventional microscopy.
- Capsulated Organisms: Organisms possessing capsules, protective layers outside their cell walls, can be effectively studied using dark-field microscopy, revealing details about their structure and interactions.
- Cytology: Dark-field microscopy finds application in cytology, the study of cells. It is particularly useful for examining internal organelles, offering a detailed view of cellular components and their functions.
- Technological Applications: In the realm of technology, the dark-field microscope has been ingeniously integrated into computer mice. This allows the mouse to function effectively over transparent mediums, enhancing its versatility and adaptability.
- Nanoparticle Characterization: When coupled with hyperspectral imaging, dark-field microscopy becomes a powerful tool for characterizing nanoparticles. This combination allows for the detailed study of nanoparticle size, shape, and distribution, providing crucial information for various scientific and industrial applications.
In summation, the Dark-Field Microscope, with its distinctive imaging capabilities, finds extensive application across a spectrum of scientific and technological disciplines. Its ability to reveal minute details and structures, otherwise imperceptible, underscores its significance in both research and practical domains.
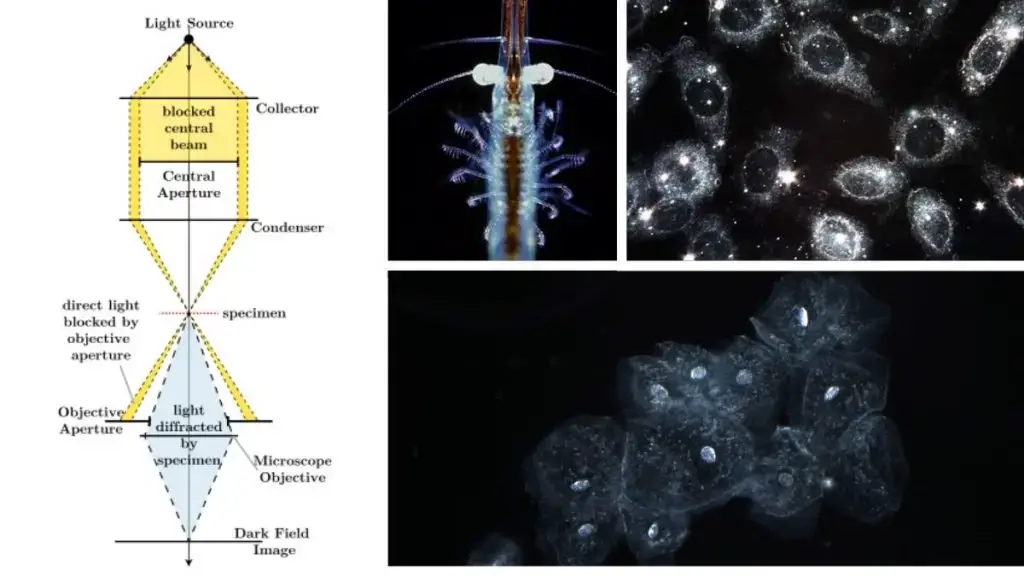
Advantages of Dark-Field Microscope
- High contrast: Dark-field microscopy produces an image with high contrast, which makes it easy to see fine details and structures that are not visible in bright-field microscopy.
- Suitable for transparent or semi-transparent samples: Dark-field microscopy is particularly well-suited for studying transparent or semi-transparent samples, such as bacteria, algae, and small crystals.
- Can observe fine details and structures: Dark-field microscopy is effective at observing fine details and structures that are not visible in bright-field microscopy, such as the flagella of bacteria or the spines of algae.
Disadvantages of Dark-Field Microscope
- Limited field of view: Dark-field microscopy has a narrow field of view compared to bright-field microscopy.
- Cannot produce a true-color image: Dark-field microscopy cannot produce a true-color image of the sample.
- Requires skilled operator: Dark-field microscopy requires a highly skilled operator to properly align the microscope and adjust the light source.
- Limited lighting options: Dark-field microscopes often have a single light source, which may not be sufficient for observing some samples.
- Limited adjustments: Dark-field microscopes often have limited adjustments, such as the ability to focus or adjust the eyepieces, which may make it more difficult to obtain a clear image.
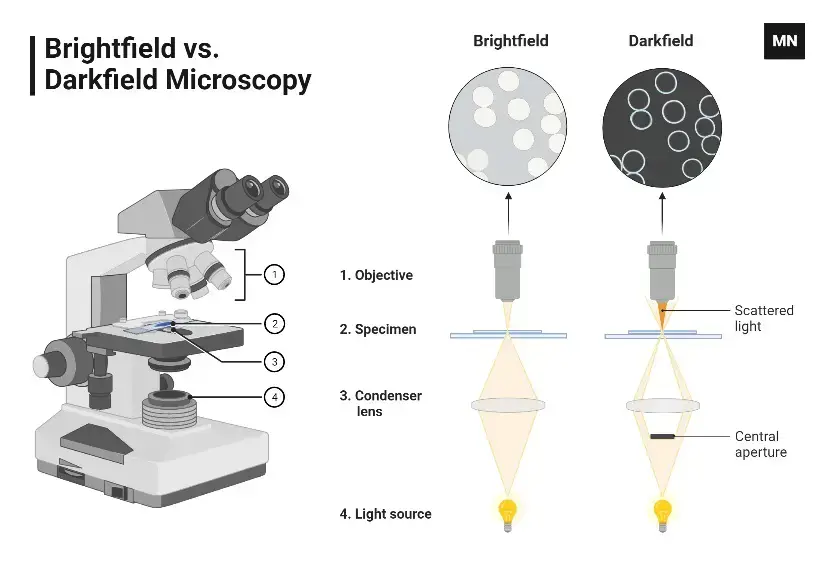
3. Phase-contrast Microscope
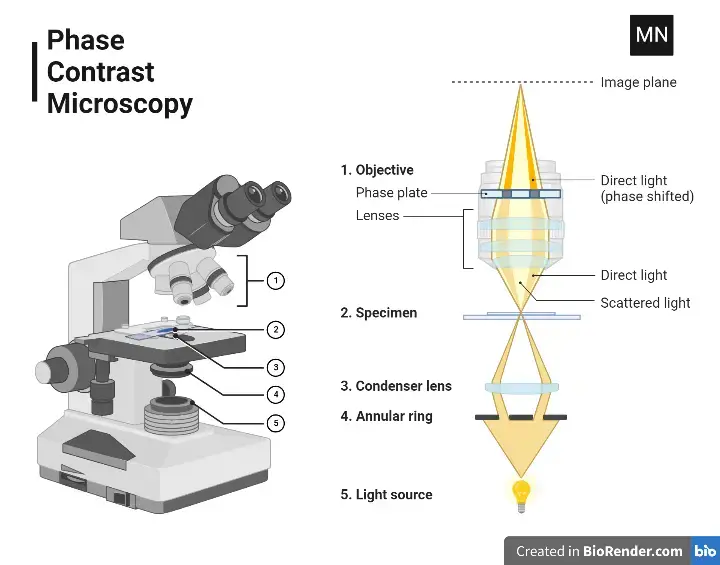
- The Phase-Contrast Microscope (PCM) stands as a pivotal advancement in the realm of optical microscopy. This instrument operates on the principle of transforming minute phase shifts in light waves into discernible variations in light intensity. Such a transformation facilitates the enhancement of contrast in the resultant images, making them more perceptible to the human eye.
- When light traverses through transparent specimens, it undergoes a subtle phase shift. This shift, albeit minuscule, is pivotal in the context of microscopy. However, this phase shift remains imperceptible to the naked eye. The ingenuity of the PCM lies in its incorporation of phase plates. These plates are adept at converting the aforementioned phase shifts into alterations in light amplitude. Consequently, these amplitude changes manifest as variations in image contrast, rendering them observable.
- One of the paramount advantages of the Phase-Contrast Microscope is its capability to observe living cells in their intrinsic state. This obviates the need for any staining or fixing procedures, which can potentially alter the specimen’s natural behavior. Furthermore, the PCM offers enhanced visibility of transparent specimens, including intricate subcellular organelles, by accentuating the contrast.
- The underlying mechanism of this contrast enhancement can be attributed to the disparities in both thickness and refractive index inherent to different segments of a specimen. As light permeates these specimens, it experiences phase shifts. The PCM ingeniously translates these shifts into variations in light intensity or brightness. This, in turn, augments the contrast in the resultant image, offering a clearer and more detailed visualization of the specimen.
- In summation, the Phase-Contrast Microscope is a testament to the confluence of optical principles and innovative design, providing researchers with a tool that captures the intricacies of transparent specimens with unparalleled clarity and contrast.
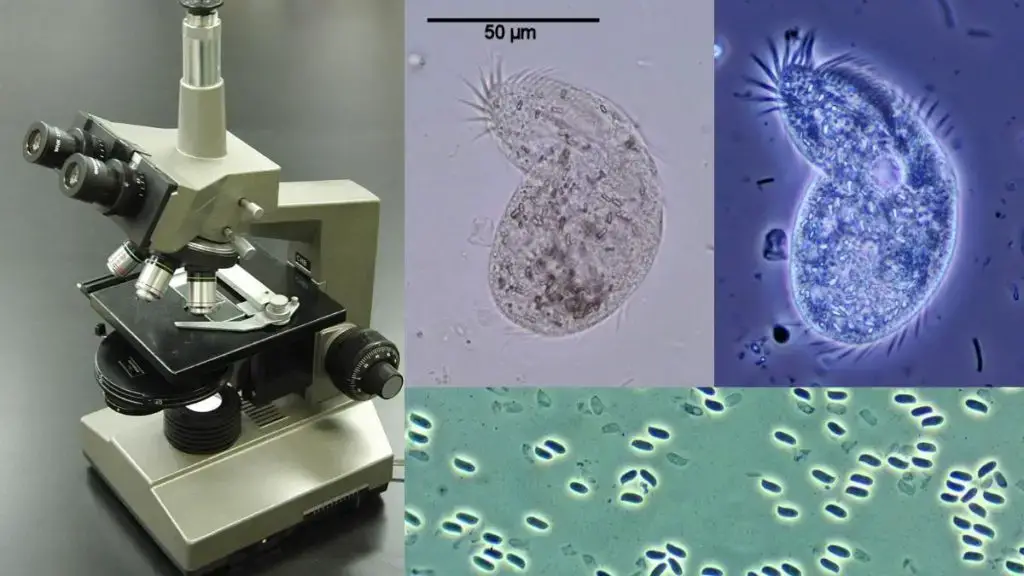
Phase Contrast Microscope Principle
- The Phase Contrast Microscope (PCM) operates on a foundational optical principle that capitalizes on the interaction of light with specimens of varying refractive indexes and thicknesses. To elucidate, when light emanating from the illuminator is channeled onto the specimen via the condenser annulus, it traverses regions of the specimen that possess distinct refractive properties and thicknesses.
- As a consequence of this interaction, light rays that permeate regions characterized by a heightened refractive index and increased thickness undergo a more pronounced phase retardation compared to those rays that navigate through regions with a diminished refractive index and reduced thickness.
- It is imperative to note that these differential phase shifts, despite their significance in microscopy, remain elusive to unaided human vision. The brilliance of the PCM lies in its integration of a specialized optical component, known as the phase plate.
- This device is meticulously designed to transmute the aforementioned phase shifts into alterations in light brightness. Such a transformation culminates in discernible contrast variations in the resultant microscopic image.
Phase Contrast Microscope Parts
The Phase Contrast Microscope (PCM) is a specialized variant of the compound microscope, augmented with specific optical components tailored for phase contrast microscopy. In addition to the standard components inherent to a compound microscope, the PCM is equipped with two pivotal optical parts: the condenser annulus and the phase plate.
- Condenser Annulus Often referred to as the phase condenser or sub-stage annular diaphragm, the condenser annulus plays a crucial role in the optical configuration of the PCM. This component is characterized by a black, light-absorbing circular plate embedded with a transparent annular ring or groove. The primary function of the condenser annulus is to direct a circumscribed hollow cone of light onto the specimen situated on the microscope stage. Positioned beneath the condenser, it ensures that only the light traversing through the annular ring illuminates the specimen.
- Phase Plate Situated above the objective’s rear focal plane, the phase plate stands as a quintessential component in the phase contrast mechanism. This optical part is tasked with the selective modification of both the phase and amplitude of the light emanating from the specimen. Structurally, the phase plate is a transparent circular plate, bifurcated into two distinct regions. The segment aligned with the condenser annulus is designated as the conjugate area, while the residual section is termed the complementary area.
The complementary area is distinctively treated with a light retarding substance, typically magnesium fluoride, to induce specific phase shifts. Furthermore, phase plates can be categorized into two primary types based on their structural attributes:
- Positive Phase Plate: Characterized by a conjugate area that is comparatively thinner.
- Negative Phase Plate: Distinguished by a conjugate area that exhibits increased thickness.
In summation, the Phase Contrast Microscope, while retaining the foundational components of a compound microscope, incorporates the condenser annulus and phase plate to achieve its unique imaging capabilities. These specialized components are instrumental in manipulating light in a manner that accentuates the contrast of transparent specimens, thereby facilitating their detailed visualization.
Application of Phase-contrast Microscope
The Phase Contrast Microscope (PCM) stands as a pivotal instrument in the domain of microscopy, offering a suite of specialized applications that capitalize on its unique imaging capabilities. The following elucidates the diverse uses of the PCM in scientific research and analysis:
- Observation of Living Cells: One of the primary advantages of the PCM is its ability to visualize living cells in their intrinsic state. This facilitates real-time observation and analysis, eliminating the need for staining or other preparative techniques that might alter the cell’s natural behavior.
- Microbiological Studies: The PCM proves indispensable in the field of microbiology. It is adept at providing detailed images of a range of microorganisms, including protozoans, diatoms, planktons, cysts, helminths, and larvae. Such capabilities are crucial for understanding the morphology, behavior, and interactions of these microscopic entities.
- Examination of Subcellular Structures and Processes: The PCM’s enhanced contrast capabilities make it an ideal tool for delving into the intricacies of subcellular structures. Researchers can gain insights into organelles, cellular dynamics, and various intracellular processes, thereby deepening our understanding of cellular biology.
- Analysis of Thin Tissue Sections: In histological studies, the PCM is employed to scrutinize thin slices of tissues. This allows for the detailed examination of cellular arrangements, tissue architecture, and other histopathological features without the need for extensive sample preparation.
- Industrial and Material Science Applications: Beyond biological applications, the PCM finds utility in the realm of material science. It is employed to study lithographic patterns, latex dispersions, and the morphology of glass fragments. Additionally, its ability to visualize crystals provides valuable insights into their structure and properties.
In conclusion, the Phase Contrast Microscope, with its distinctive optical properties, serves as an invaluable tool across diverse scientific disciplines. From biological research to material analysis, its applications underscore its versatility and the depth of insights it offers to researchers and professionals alike.
Advantages of Phase-contrast Microscope
- Improved contrast for transparent or semi-transparent samples: Phase-contrast microscopy improves the contrast of transparent or semi-transparent samples, such as cells, bacteria, and small structures within materials, making them easier to observe.
- Can observe fine details and structures: Phase-contrast microscopy is effective at observing fine details and structures that are not visible in bright-field microscopy.
- Can produce a true-color image: Phase-contrast microscopy can produce a true-color image of the sample.
- Widely used: Phase-contrast microscopy is widely used in biology, medicine, and materials science to study the structure and function of cells and other small structures.
Disadvantages of Phase-contrast Microscope
- Requires special phase plates: Phase-contrast microscopy requires the use of special phase plates, which may be expensive and may need to be replaced periodically.
- Requires skilled operator: Phase-contrast microscopy requires a skilled operator to properly align the microscope and adjust the light source.
- Limited lighting options: Phase-contrast microscopes often have a single light source, which may not be sufficient for observing some samples.
- Limited adjustments: Phase-contrast microscopes often have limited adjustments, such as the ability to focus or adjust the eyepieces, which may make it more difficult to obtain a clear image.
4. Fluorescent Microscope
The Fluorescence Microscope stands as a specialized variant of the optical microscope, distinguished by its utilization of fluorescence or phosphorescence phenomena to magnify and elucidate specimens. Unlike conventional light microscopes, the Fluorescence Microscope harnesses the unique properties of certain molecules to emit light upon excitation, thereby enabling the visualization of specific cellular components and structures.
At its core, this instrument operates by illuminating the specimen with a specific wavelength of light, which subsequently excites fluorescent molecules within the specimen. Upon returning to their ground state, these molecules emit light of a longer wavelength, which is then captured and magnified to produce a detailed image.
The applications of the Fluorescence Microscope are vast and pivotal in the realm of biological research. Some of its primary uses include:
- Cellular and Subcellular Studies: The Fluorescence Microscope is adept at visualizing living cells and intricate cell organelles. By targeting specific fluorescent markers, researchers can gain insights into cellular dynamics, structure, and function.
- Protein Identification: Given its high sensitivity, the Fluorescence Microscope is instrumental in pinpointing specific proteins within a cellular context. This is particularly valuable in understanding protein localization, interactions, and functionality.
- Immunological Studies: The instrument is frequently employed in immunofluorescence techniques, allowing for the detection of specific antigens and immunoglobulins. This has profound implications in understanding immune responses, disease mechanisms, and therapeutic interventions.
- High Sensitivity Imaging: One of the paramount attributes of the Fluorescence Microscope is its heightened sensitivity. This ensures that even minute quantities of fluorescent molecules can be detected, rendering it invaluable in studies requiring precision and detail.
In summation, the Fluorescence Microscope, with its unique optical principles and capabilities, has revolutionized the field of microscopy. Its ability to harness the properties of fluorescence and phosphorescence has opened avenues for detailed, specific, and sensitive imaging, making it an indispensable tool in modern scientific research.
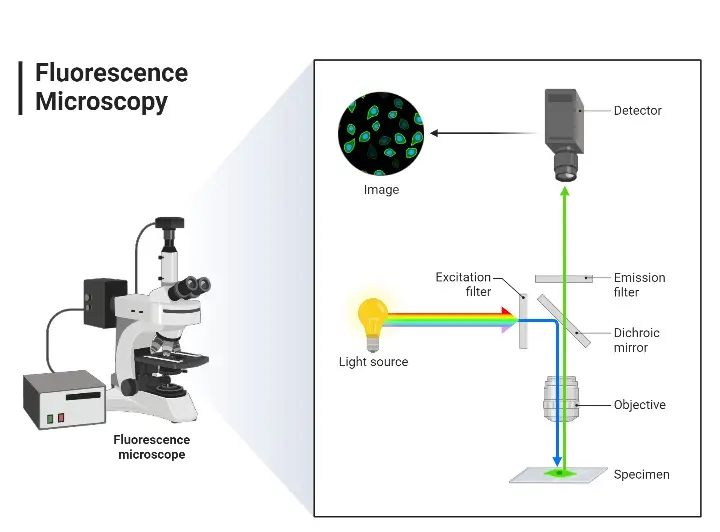
Fluorescence Microscope Principle
The Fluorescence Microscope operates on the foundational principle of fluorescence, a phenomenon wherein certain molecules, when exposed to specific wavelengths of light, absorb this energy and subsequently re-emit light of a different wavelength. The intricacies of this principle, as applied in the Fluorescence Microscope, are detailed below:
- Specimen Preparation: The specimen of interest is meticulously stained with a specific compound known as a fluorophore. This molecule possesses the unique ability to fluoresce upon exposure to certain wavelengths of light.
- Light Generation and Filtration: A source generates high-energy light, which is then channeled through an excitation filter. This filter is calibrated to permit only short-wavelength light, typically in the ultraviolet (UV) region, to traverse. This light is specifically tailored to excite the fluorophore, while concurrently blocking all other wavelengths.
- Dichroic Filter and Fluorophore Excitation: The filtered light is subsequently reflected onto the specimen using a dichroic filter. Upon interaction with the fluorophore, the light is absorbed, causing the electrons within the fluorophore to elevate to a higher energy state. As these excited electrons revert to their ground state, they release energy in the form of light, but crucially, this emitted light possesses a longer wavelength than the incident light.
- Emission Filtration and Detection: The emitted light, bearing a longer wavelength, traverses the dichroic mirror and is directed towards the emission filter. This filter is designed to obstruct the original short-wavelength light, allowing only the longer-wavelength emitted light to progress. This filtered light then advances through the ocular lenses, culminating at the detector system.
- Image Formation: Within the detector, a magnified image of the specimen is constructed. Owing to the principles of fluorescence, the background manifests as a stark contrast, appearing dark, while the fluorescent regions of the specimen are illuminated, presenting as bright entities.
Fluorescence Microscope Parts
The Fluorescence Microscope, a specialized variant of the optical microscope, is equipped with a suite of distinct components tailored to harness the phenomenon of fluorescence. The following delineates the integral parts of this sophisticated instrument:
- Fluorophore (Fluorescent Dye):
- Definition: Fluorophores are chemical entities endowed with the capability to fluoresce, meaning they absorb light at a specific wavelength and subsequently re-emit light at a longer wavelength.
- Composition: Predominantly organic in nature, fluorophores are often complex molecules comprising aromatic or planar structures enriched with multiple pi (π) bonds.
- Application: These compounds are employed to stain a plethora of biomolecules and cellular structures, with notable examples being fluorescein, rhodamine, cyanine, and acridine orange, among others.
- Light Source:
- Components: The mercury vapor lamp is a prevalent source for generating ultraviolet (UV) light. However, alternative sources such as xenon arc lamps, high-power LEDs, and lasers are also utilized.
- Function: These sources emit high-energy light pivotal for exciting the fluorophores.
- Excitation Filter:
- Position: Strategically located in the illumination path, preceding the specimen.
- Role: This band-pass filter permits the passage of short-wavelength light, which is competent in exciting the fluorophore, while obstructing other wavelengths.
- Emission Filter:
- Position: Situated in the imaging path, subsequent to the specimen.
- Function: Another band-pass filter, it allows the light emitted by the fluorophore to traverse while inhibiting light in the excitation range. This ensures a pronounced contrast between the dark background and the illuminated specimen.
- Dichroic Mirror (Beam Splitter):
- Design: A specialized mirror that can selectively reflect or transmit specific wavelengths of light.
- Positioning: Interposed between the excitation and emission filters, it is oriented at a 45° angle.
- Function: It directs the light from the excitation filter towards the fluorophore and channels the emitted light towards the emission filter.
- Additional Components:
- The Fluorescence Microscope also encompasses a detector system, objective lenses, and ocular lenses. Furthermore, it retains all the foundational components inherent to a conventional compound microscope.
In summation, the Fluorescence Microscope, with its array of specialized components, is adept at capturing the intricate dance of light and molecules. By manipulating the properties of fluorescence, this instrument offers unparalleled insights into specimens, rendering otherwise elusive details vividly discernible in a high-contrast setting.
Types of Fluorescence Microscope
Fluorescence microscopy, an advanced domain within optical microscopy, encompasses various specialized types, each tailored for distinct research and diagnostic applications. Herein, we delineate the primary types of fluorescence microscopes and their respective characteristics:
- Epifluorescence Microscope:
- Description: The Epifluorescence Microscope stands as a prevalent type of fluorescence microscope. Its defining feature is the concurrent path for both excitation and emission light, meaning both traverse through the same objective lens.
- Confocal Microscope:
- Description: Also termed the confocal laser scanning microscope, this instrument employs a spatial pinhole to eliminate out-of-focus light, thereby generating 3-D images with enhanced resolution and contrast. The microscope focuses excitation light on a specific focal plane spot, which is then optically manipulated to scan the entire specimen.
- Mechanism: Laser light illuminates the fluorophore-stained specimen. The resultant emitted fluorescent light traverses a pinhole, selectively permitting light from the focal point while blocking extraneous background light. This light is subsequently transformed into an electrical signal via a photomultiplier tube, with computer software analyzing this signal to render a 3-D image.
- Applications:
- Detection of corneal diseases and identification of fungal cells in corneal scrapings.
- Quality control in pharmaceutical products.
- Optical 3-D scanning and imaging.
- Limitations:
- Restricted excitation wavelength and narrow bands.
- High cost associated with the system.
- Multiphoton Microscope:
- Description: This microscope variant employs multiple photons to excite fluorophore molecules, leading to the generation of high-resolution 3-D images. The most prevalent forms include two-photon and three-photon excitation microscopy.
- Total Internal Reflection Fluorescence (TIRF) Microscope:
- Description: The TIRF Microscope is adept at imaging fluorophore molecules situated in an aqueous environment adjacent to a solid surface with a pronounced refractive index. This selective imaging results in high-resolution visuals.
- Advantages: The TIRF Microscope offers images with heightened contrast, reduced background interference, and enhanced clarity, making it a valuable tool for specific imaging applications.
In summation, the realm of fluorescence microscopy is vast, with each type offering unique capabilities tailored to specific research needs. From the foundational Epifluorescence Microscope to the advanced TIRF and Confocal systems, these instruments have revolutionized the field of microscopy, providing unparalleled insights into the microscopic world.
Uses of Fluorescence Microscope
The Fluorescence Microscope, a specialized instrument within the domain of optical microscopy, offers a myriad of applications across diverse scientific disciplines. Its ability to harness the properties of fluorescence provides researchers with unparalleled insights into various biological and chemical phenomena. The following elucidates the primary uses of the Fluorescence Microscope:
- Cellular and Subcellular Analysis:
- The Fluorescence Microscope is adept at visualizing both fixed and live cells, allowing researchers to delve into the intricate structures of cells and their organelles. This capability is pivotal for understanding cellular morphology, dynamics, and functionality.
- Physiological Assessment:
- The instrument is employed to gauge the physiological state of cells. By utilizing specific fluorescent markers, researchers can ascertain various cellular parameters, such as pH, ion concentrations, and metabolic activity, thereby providing insights into cellular health and behavior.
- Clinical Microbiology:
- In the realm of clinical diagnostics, the Fluorescence Microscope proves invaluable for the detection of specific pathogens. It facilitates the identification of acid-fast bacteria, malarial parasites, and a plethora of other microorganisms within clinical samples, ensuring timely and accurate diagnosis.
- Molecular Biology and Biochemistry:
- The Fluorescence Microscope is frequently employed in the study of macromolecules and nucleic acids. By tagging these molecules with specific fluorophores, researchers can visualize and analyze their distribution, interactions, and dynamics, thereby deepening our understanding of molecular biology and biochemistry.
- Fluorescent In-situ Hybridization (FISH):
- The FISH technique, integral to the study of microbial ecology, relies heavily on the Fluorescence Microscope. FISH allows for the specific labeling and visualization of microbial populations within environmental samples, providing insights into microbial diversity, distribution, and interactions.
In summation, the Fluorescence Microscope, with its unique optical capabilities, serves as an indispensable tool across a spectrum of scientific endeavors. From cellular biology to clinical diagnostics and molecular research, its applications underscore its versatility and the depth of insights it offers to the scientific community.
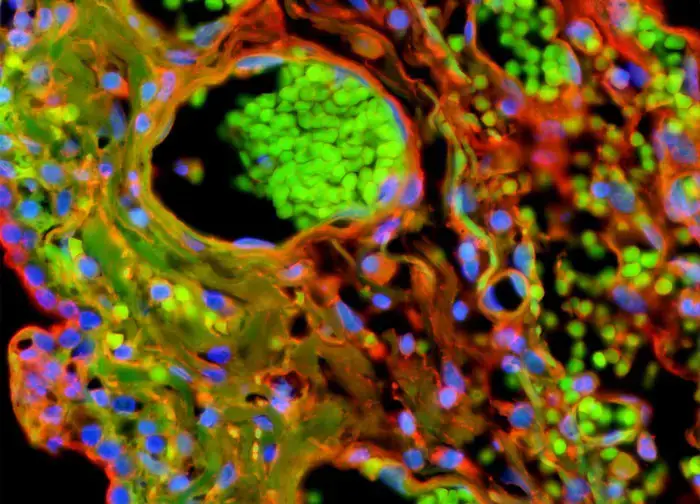
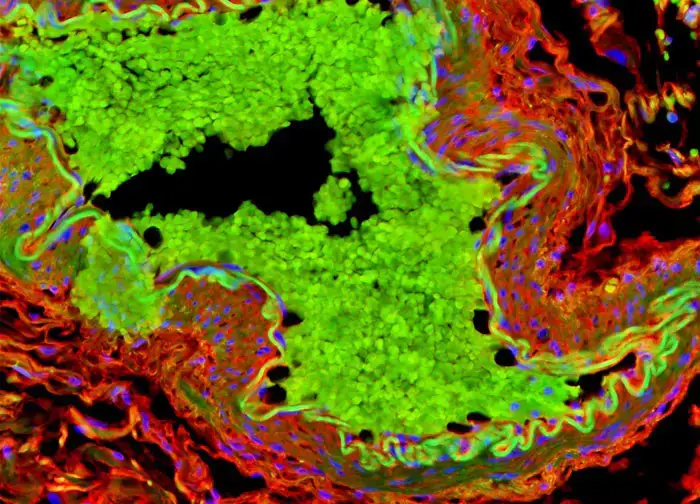
Advantages of Fluorescent Microscope
- Improved contrast and resolution: Fluorescent microscopy can produce high-contrast images with improved resolution compared to bright-field microscopy, making it easier to observe fine details and structures within the sample.
- Can identify specific molecules or structures: Fluorescent microscopy can be used to identify specific molecules or structures within the sample by using fluorescent dyes that are specific for those molecules or structures.
- Can produce a true-color image: Fluorescent microscopy can produce a true-color image of the sample.
- Widely used: Fluorescent microscopy is widely used in biology, medicine, and materials science to study the structure and function of cells and to identify specific molecules or structures within the sample.
Disadvantages of Fluorescent Microscope
- Requires specialized equipment: Fluorescent microscopy requires specialized equipment, such as a fluorescence light source and fluorescent dyes, which may be expensive and may need to be replaced periodically.
- Requires skilled operator: Fluorescent microscopy requires a skilled operator to properly align the microscope and adjust the light source.
- Limited lighting options: Fluorescent microscopes often have a single light source, which may not be sufficient for observing some samples.
- Limited adjustments: Fluorescent microscopes often have limited adjustments, such as the ability to focus or adjust the eyepieces, which may make it more difficult to obtain a clear image.
B. Electron Microscope
The Electron Microscope stands as a paradigm shift in the realm of microscopy, diverging from traditional optical microscopes by employing accelerated electron beams as the illuminating medium, rather than conventional light rays. This instrument’s design and functionality are underpinned by the following key attributes:
- Electromagnetic Lenses:
- In the Electron Microscope, traditional glass lenses are supplanted by electromagnets. These electromagnetic lenses function to focus and direct the accelerated electron beams, ensuring precision and clarity in imaging.
- High-Resolution Imaging:
- One of the paramount advantages of the Electron Microscope is its ability to produce images of exceptional resolution. This is attributed to the inherently short wavelength of electrons, which facilitates the capture of intricate details that are beyond the reach of optical microscopes.
- Magnification Capabilities:
- The Electron Microscope boasts unparalleled magnification capabilities, with the potential to magnify specimens up to an astounding 10,000,000 times their original size. This magnification prowess allows researchers to delve into the nanoscopic realm, unveiling structures and details that were previously imperceptible.
- Contrast and Detail:
- Beyond its resolution and magnification attributes, the Electron Microscope is renowned for producing images characterized by high contrast. This ensures that even minute differences in specimen composition and structure are vividly discernible, providing researchers with comprehensive insights into their samples.
- Specimen Clarity:
- The instrument’s advanced design and technology enable the clear visualization of specimens with dimensions as minute as 0.2 nm. This capability is instrumental in studies that necessitate the examination of ultra-fine structures and details.
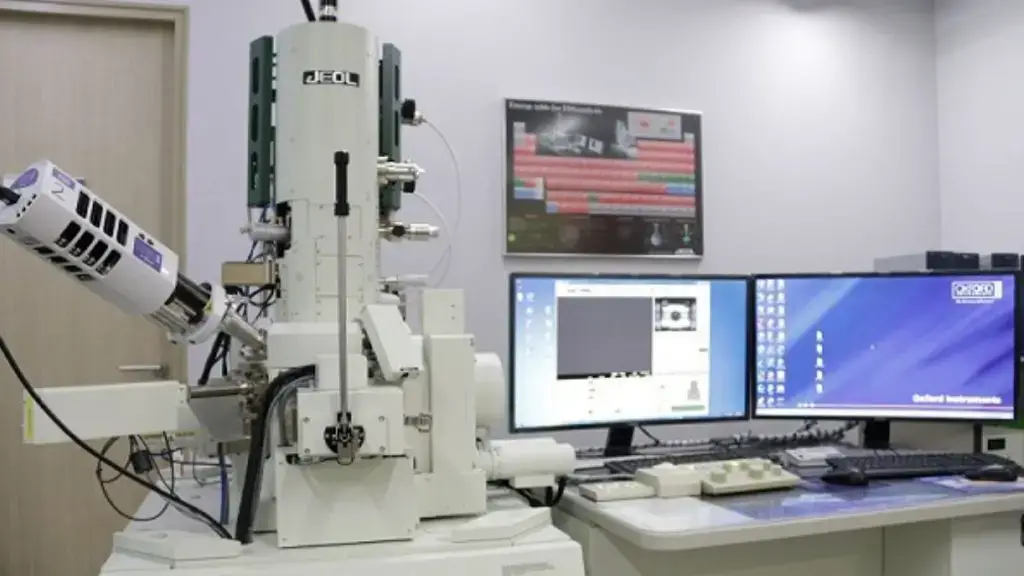
Principle of Electron Microscope
The Electron Microscope, a pinnacle in microscopy technology, operates on a distinct principle that diverges from traditional optical microscopes. The foundational principle and operational mechanism of the Electron Microscope are elucidated below:
- Electron Generation:
- At the heart of the Electron Microscope lies the electron gun, typically constituted of a heated tungsten filament or a field emission source. This component is responsible for generating a stream of electrons, which are then subjected to high voltages, typically ranging from 100 to 1000 kV.
- Electron Acceleration and Focusing:
- Within the vacuum system of the microscope, an anode plate accelerates the electrons. Subsequent to this acceleration, the electron beam is meticulously focused onto the specimen. This focusing is achieved using a combination of aperture and electromagnetic lenses, ensuring precision in electron beam direction.
- Electron-Specimen Interaction:
- As the accelerated electron beam impinges upon the specimen, it interacts with the various components of the sample. This interaction results in the scattering of electrons. Notably, the extent of this scattering is contingent upon factors such as the refractive index and thickness of the specimen. Different regions or components of the specimen scatter electrons to varying degrees, leading to contrast in the resultant image.
- Image Formation:
- The electrons scattered from the specimen are subsequently collected and channeled through a series of electromagnetic lenses, including both objective and ocular lenses. These lenses play a pivotal role in focusing the scattered electron beams.
- The magnetic lenses then detect these scattered beams and transform them into highly magnified images. The inherent short wavelength of the electrons ensures that these images are of exceptional resolution, revealing intricate details of the specimen.
Electron Microscope Parts
The Electron Microscope, a sophisticated instrument in the microscopy realm, is intricately designed with a myriad of components, each serving a specific function to ensure optimal imaging capabilities. The following delineates the primary components of a typical Electron Microscope:
- Electron Gun (Electron Source):
- Function: The electron gun is pivotal for generating the electron beams essential for specimen illumination.
- Composition: It comprises a cathode, typically a tungsten filament, and an anode. Upon heating the tungsten filament in a vacuum using high voltage, electrons are emitted. A surrounding negative cap confines these electrons, producing a focused electron beam, which is subsequently accelerated by the positive anode towards the specimen.
- Electromagnetic Lenses:
- Function: In contrast to optical microscopes that employ glass lenses, electron microscopes utilize electromagnetic lenses, which harness magnetic fields generated by electromagnets to focus and direct electron beams.
- Types:
- Condenser Lens: Focuses the accelerated electron beam onto the specimen, ensuring a precise and concentrated beam.
- Objective Lens: Collects and magnifies the electron beam post-specimen interaction.
- Projector (Ocular) Lens: Further amplifies the image produced by the objective lenses.
- Aperture System:
- Function: These microscopic pinholes serve to filter extraneous electrons from the electron beam, both pre and post-specimen interaction.
- Composition: The system encompasses thin disks with minuscule holes ranging from 2 to 100 μm in diameter. Key apertures include the condenser aperture (situated below the condenser lens) and the objective aperture (positioned between the objective and projector lenses).
- Sample Holder:
- Function: This component provides a stable platform equipped with a mechanical arm to securely position specimens.
- Composition: Typically, it consists of an ultra-thin carbon film supported by a metal grid.
- Vacuum System:
- Function: The vacuum system ensures an environment devoid of air, facilitating unhindered electron generation and travel.
- Composition: The vacuum column houses the lenses, aperture, sample holder, and specimens. High-efficiency vacuum pumps maintain the requisite vacuum, preventing electron collisions and scatter.
- Imaging System:
- Function: This system is responsible for refining, magnifying, and projecting images.
- Composition: It encompasses electromagnetic lenses, a phosphorescent screen for image visualization, and a camera for image capture and display. The resultant image, termed an electron micrograph, is either displayed on a phosphorescent plate or a monitor.
In summation, the Electron Microscope, with its intricate assembly of components, offers unparalleled imaging capabilities, enabling researchers to delve deep into the nanoscopic realm. Each component, from the electron gun to the imaging system, plays a pivotal role in ensuring the instrument’s precision and efficacy.
Application of Electron Microscope
Electron microscopy, with its unparalleled resolution and depth of imaging, has found applications across a myriad of scientific disciplines. Its ability to visualize structures at the nanometer and even atomic scale has revolutionized our understanding in various fields. Here are some of the prominent uses of electron microscopes:
- Microbiology:
- Electron microscopes play a pivotal role in microbiological research, enabling scientists to delve into the intricate details of microbial entities. They are instrumental in elucidating the structural intricacies of viruses, bacterial cells, flagella, and pili, thereby enhancing our understanding of microbial physiology and pathogenicity.
- Crystallography and Nanotechnology:
- In the realm of crystallography, electron microscopy aids in the analysis of crystal structures, revealing atomic arrangements and lattice defects.
- Furthermore, as nanotechnology seeks to manipulate materials at the atomic or molecular scale, electron microscopy provides the necessary visualization to guide this precision engineering.
- Cellular Biology:
- The morphology of cellular organelles, from mitochondria to the Golgi apparatus, can be intricately studied using electron microscopes. This has been pivotal in understanding cellular functions and dysfunctions.
- Forensic Science:
- In the domain of forensics, electron microscopy is employed for the ballistic study of gunshots. By analyzing gunshot residues and bullet fragments, investigators can derive crucial information about firearms used in criminal activities.
- Geology:
- Geologists utilize electron microscopes to study the microstructure of rocks, minerals, and gems. This aids in understanding their formation, history, and any alterations they might have undergone over time.
- Industrial Quality Control and Research:
- Electron microscopy is indispensable in industries for quality control purposes. It assists in the detection of fractures, cracks, and other structural defects in materials.
- Additionally, in the pharmaceutical sector, it aids in drug development by allowing researchers to visualize drug interactions at the molecular level and analyze atomic structures.
Limitations of Electron Microscope
Electron microscopy, while a powerful and revolutionary tool in the realm of scientific imaging, is not without its limitations. These constraints, inherent to the technology, can sometimes pose challenges to researchers and limit its applicability in certain scenarios. Here are some of the primary limitations associated with electron microscopy:
- Economic and Complexity Concerns:
- One of the foremost challenges associated with electron microscopes is their high cost. Acquiring, maintaining, and operating these instruments require significant financial investment.
- Additionally, the complexity of the system demands specialized training for operation, further adding to the costs and limiting its widespread use.
- Monochromatic Imaging:
- Electron microscopes produce images in black and white. This absence of color can sometimes limit the amount of information that can be derived from the images, especially when distinguishing between different components or structures within a specimen.
- Specimen Thickness Constraints in TEM:
- Transmission Electron Microscopy (TEM) necessitates the use of extremely thin specimens, often not more than a few nanometers thick. Preparing specimens of this thickness can be challenging and might not be feasible for all types of samples. Moreover, this limitation restricts the kind of information that can be obtained, especially from thicker specimens.
- Vacuum Requirement:
- Electron microscopes operate in a vacuum to ensure that the electron beam remains uninterrupted by air molecules. This necessitates a sophisticated vacuum system, which can be cumbersome and adds to the complexity of the setup. Furthermore, the requirement for a vacuum means that living specimens cannot be observed in their natural, hydrated state, potentially altering their native structures.
In summary, while electron microscopy offers unparalleled resolution and has revolutionized our understanding of the microscopic world, it is essential to be cognizant of its limitations. These constraints can influence the choice of microscopy technique depending on the specific requirements of a study.
Types of Electron Microscope
There are present different types of electron microscope such as;
1. Scanning Electron Microscope (SEM)
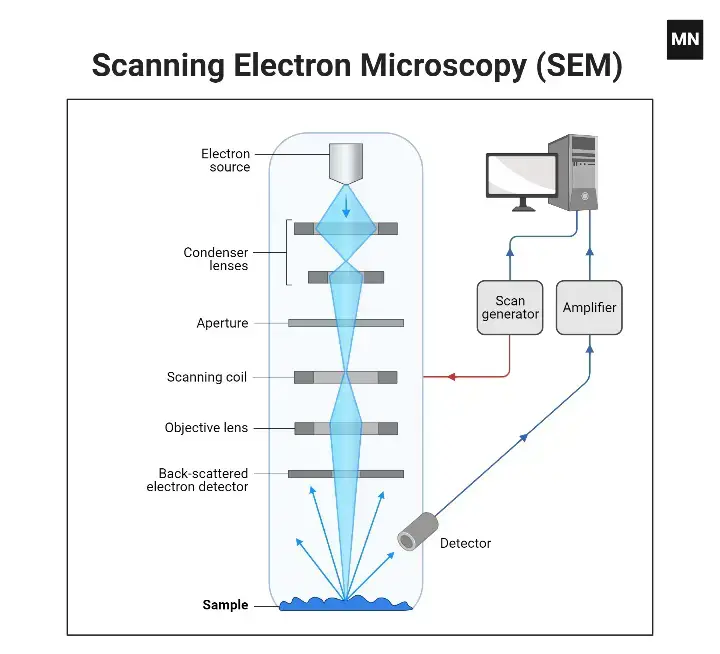
The Scanning Electron Microscope (SEM) is a specialized variant of electron microscopes, distinguished by its unique imaging approach and capabilities. This instrument offers a detailed exploration of specimen surfaces, providing three-dimensional visualizations that are both intricate and informative. The following elucidates the fundamental principles, components, and attributes of the SEM:
- Operational Mechanism:
- The SEM operates by raster scanning a specimen using a high-energy beam of electrons. This scanning pattern systematically covers the specimen’s surface, ensuring comprehensive imaging.
- Unlike the Transmission Electron Microscope (TEM) which captures transmitted electrons, the SEM primarily focuses on the electrons that are emitted, backscattered, or diffracted from the specimen upon interaction with the incident electron beam.
- Image Formation:
- The resultant images generated by the SEM are three-dimensional in nature, offering a topographical view of the specimen’s surface. These images vividly depict the morphological features and textural nuances of the specimen.
- While the SEM might not achieve the magnification levels of the TEM, it compensates with images of superior resolution and clarity. The images produced are sharp, detailed, and provide a depth perspective that is invaluable for understanding surface structures.
- Detectors in SEM:
- Integral to the SEM’s functionality are its array of detectors, each designed to capture specific types of electrons, thereby contributing to the overall image quality and detail.
- Back-Scattered Electron Detectors: These detectors capture electrons that are backscattered from the specimen. The intensity of these electrons is often related to the atomic number of the specimen, providing compositional information.
- Secondary Electron Detectors: These detectors are attuned to capture secondary electrons, which are emitted from the specimen’s surface due to the incident electron beam’s energy. These electrons provide detailed information about the specimen’s topography.
- X-ray Detectors: These detectors capture X-rays emitted from the specimen, providing elemental composition data.
- Integral to the SEM’s functionality are its array of detectors, each designed to capture specific types of electrons, thereby contributing to the overall image quality and detail.
In summation, the Scanning Electron Microscope (SEM) stands as a testament to the advancements in microscopy technology. Its ability to provide detailed, three-dimensional images of specimen surfaces, coupled with its suite of specialized detectors, renders it an invaluable tool for researchers and professionals across various scientific disciplines. Through the SEM, the intricate world of surface structures is vividly brought to life, offering insights that are both profound and enlightening.
Application of Scanning Electron Microscope (SEM)
- Used to study the surface area of microorganisms in detail.
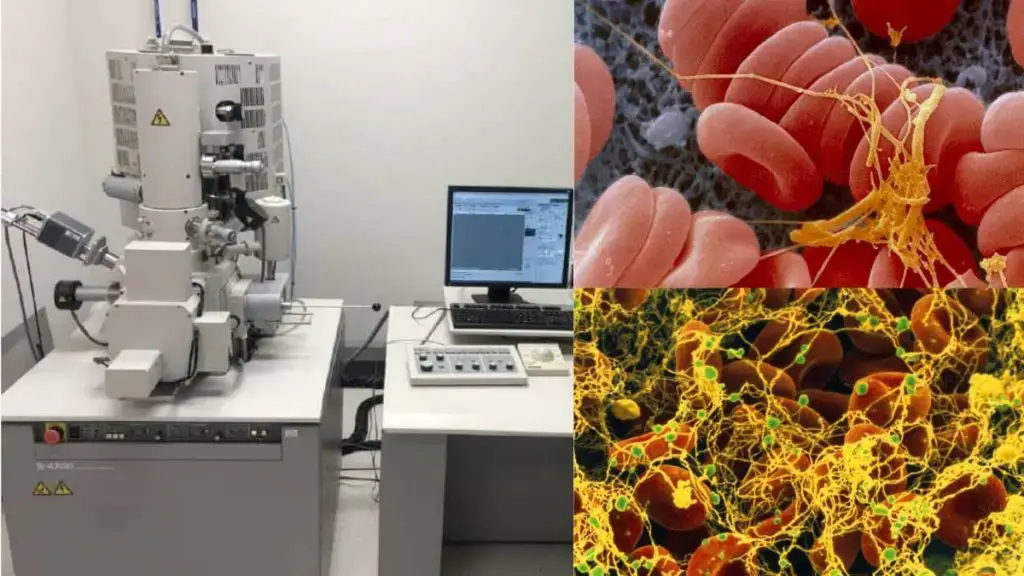
2. Transmission Electron Microscope (TEM)
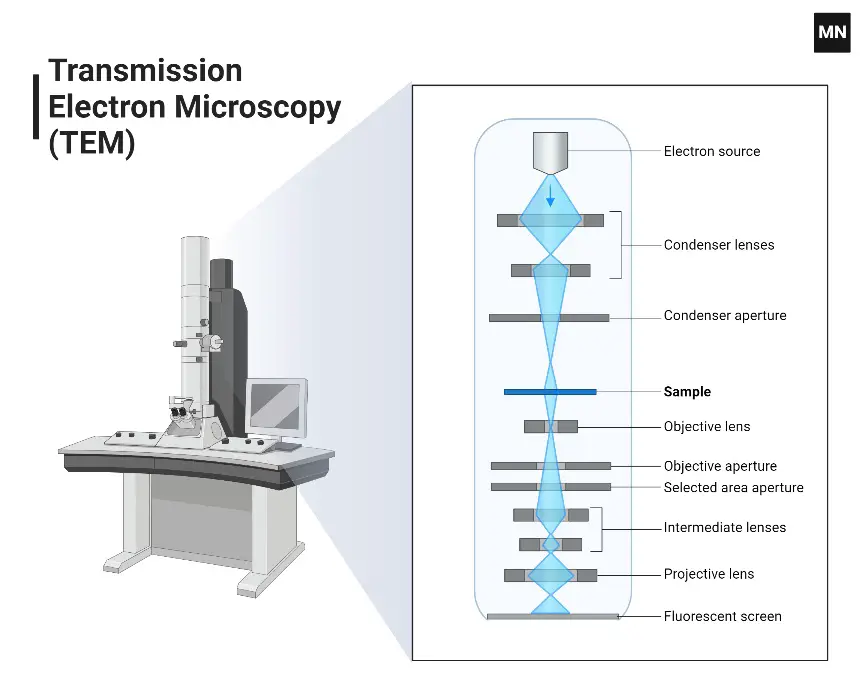
The Transmission Electron Microscope (TEM) stands as a specialized category within the broader family of electron microscopes. Its operational mechanism and imaging capabilities are distinct, offering researchers unparalleled insights into the nanoscopic realm. The following elucidates the fundamental principles and attributes of the TEM:
- Principle of Operation:
- The TEM operates by utilizing transmitted electrons to generate a magnified representation of a specimen. This transmission is facilitated by the interaction of the electron beam with the specimen’s constituents.
- Specimen Thickness:
- A quintessential aspect of TEM imaging is the requirement for extremely thin specimens. These specimens typically do not exceed a thickness of 100 nm. To put this into perspective, they are approximately 200 times thinner than those used in conventional compound microscopes.
- Electron Interaction and Imaging:
- The electron beam, focused onto the specimen by a condenser lens, interacts with the specimen’s components. Post-interaction, electrons are emitted from the specimen. These emitted electrons are subsequently channeled through a series of electromagnetic lenses, including both objective and ocular lenses.
- Upon reaching the fluorescent screen, these electrons stimulate the screen to produce an enlarged image. This image generation is a consequence of the electron-screen interaction, where the impinging electrons induce fluorescence, thereby visualizing the specimen’s details.
- Image Characteristics:
- The TEM is renowned for producing two-dimensional images. These images, while being monochromatic, are characterized by their exceptional resolution. The inherent high resolution of TEM images is a testament to the instrument’s capability to discern minute details, often at the molecular or atomic scale.
- In terms of magnification, the TEM offers a broad range, with capabilities extending from a modest 2X to a staggering 50,000X. This wide magnification spectrum ensures that researchers can tailor the imaging scale to their specific requirements.
- Prevalence in Scientific Research:
- Among electron microscopes, the TEM is notably the most ubiquitous. Its widespread adoption in scientific research can be attributed to its ability to provide detailed insights into structures that are beyond the resolution limits of optical microscopes.
In summation, the Transmission Electron Microscope (TEM) is a cornerstone in the field of electron microscopy. Its unique operational principle, coupled with its unparalleled imaging capabilities, renders it an indispensable tool for researchers endeavoring to explore the intricacies of the nanoscopic world.
Application of Transmission Electron Microscope (TEM)
- It used to study the internal structure of a specimen.
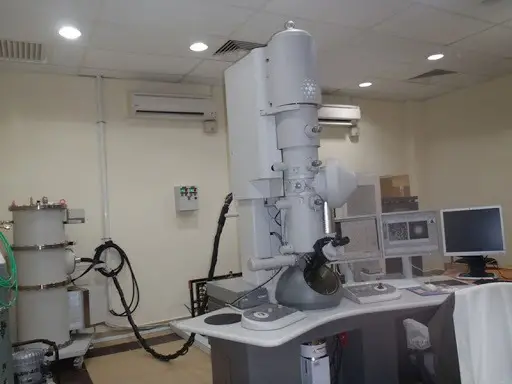
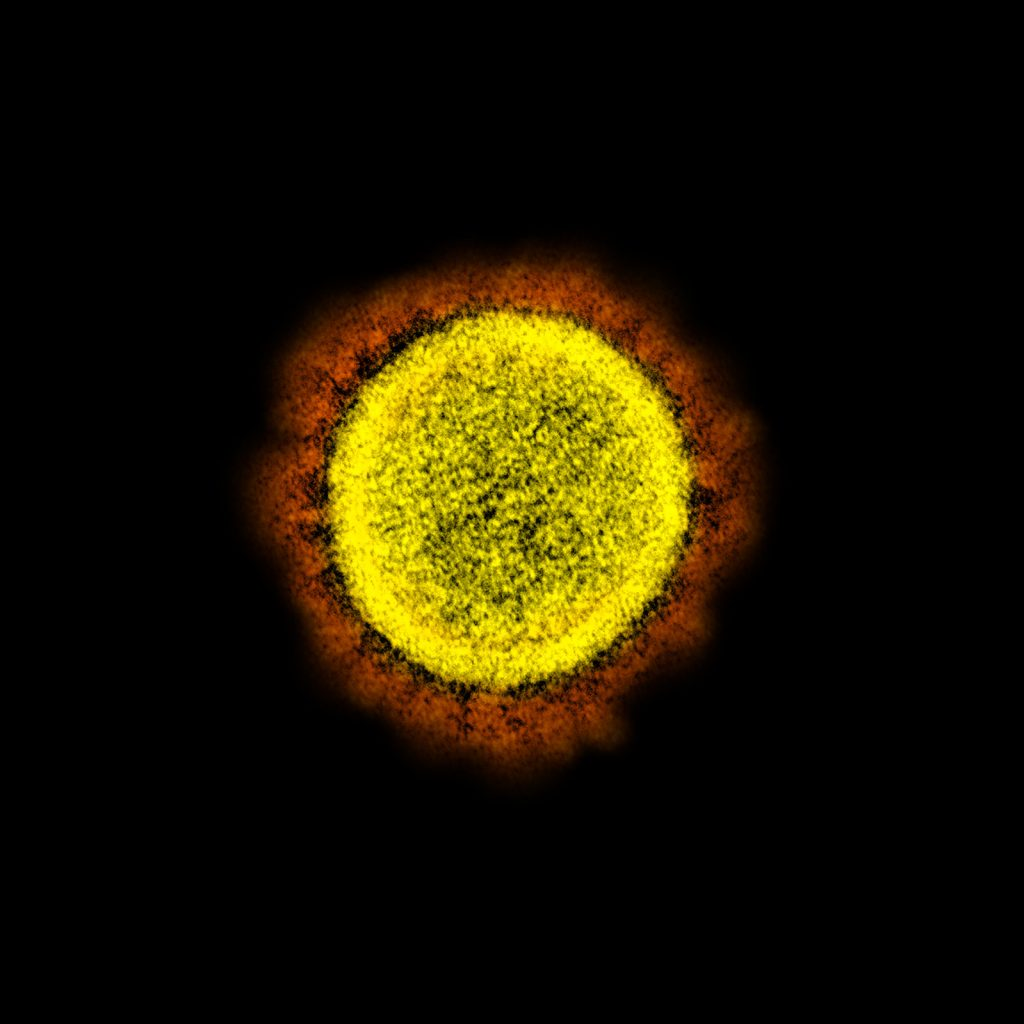
3. Confocal Microscopy
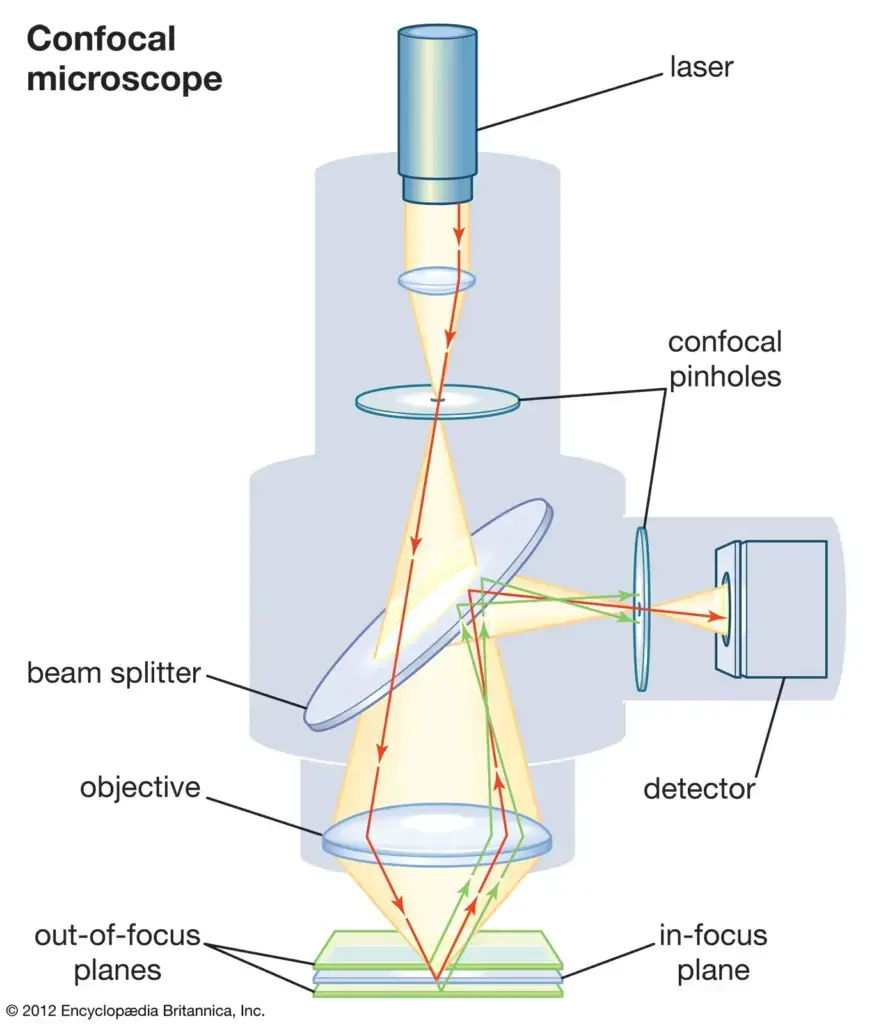
Confocal Microscopy, often referred to as Confocal Laser Scanning Microscopy (CLSM) or Laser Confocal Scanning Microscopy (LCSM), represents a pivotal advancement in the realm of optical microscopy.
- Principle of Operation:
- Central to the functionality of confocal microscopy is the strategic use of a spatial pinhole. Positioned at the confocal plane, this pinhole plays a crucial role in eliminating out-of-focus light, thereby enhancing both the optical resolution and contrast of the resultant micrograph. By selectively allowing only in-focus light to reach the detector, the confocal microscope ensures that the image is derived from a precise, thin plane within the specimen.
- Three-Dimensional Imaging:
- One of the standout features of confocal microscopy is its ability to perform optical sectioning. By sequentially capturing a series of two-dimensional images at varying depths within a specimen, the microscope facilitates the reconstruction of a comprehensive three-dimensional representation of the sample’s internal structures. This capability is invaluable for understanding the spatial relationships and intricate architectures within biological specimens, among others.
- Advantages:
- The inherent design and operational principles of confocal microscopy offer several advantages over traditional optical microscopy. Notably, the enhanced resolution and contrast, coupled with the ability to generate three-dimensional images, make it a preferred choice for detailed studies of cellular and subcellular structures.
In summation, Confocal Microscopy stands as a testament to the confluence of optics and technology, offering researchers a sophisticated tool for delving deep into the intricacies of specimens. Through its unique imaging capabilities, it provides insights that are both detailed and dimensionally comprehensive, enriching our understanding of the microscopic world.
Application
Confocal Microscopy is used extensively in the scientific and industrial communities and typical applications are in life sciences, semiconductor inspection, and materials science.
Other Types of Electron Microscopes
Electron microscopy, with its ability to provide unparalleled resolution, has evolved into various specialized forms, each tailored to specific applications and research needs. Beyond the commonly known Transmission Electron Microscope (TEM) and Scanning Electron Microscope (SEM), there exist other types of electron microscopes, each with unique functionalities:
- Reflection Electron Microscope (REM):
- The Reflection Electron Microscope operates by utilizing the beams of electrons that are reflected or scattered off the specimen’s surface to generate an image.
- This microscope integrates techniques from diffraction, imaging, and spectroscopy, offering a multifaceted approach to specimen analysis.
- Scanning Transmission Electron Microscope (STEM):
- The STEM is a hybrid instrument that amalgamates the technologies of both SEM and TEM.
- By combining the principles of these two microscopes, STEM can offer versatile imaging capabilities, capturing both surface and internal structural details of specimens.
- Scanning Tunneling Microscope (STM):
- Distinct from other electron microscopes, the STM operates based on the quantum mechanical phenomenon known as electron tunneling.
- Instead of penetrating the specimen, the STM scans the atomic and molecular structures present on the specimen’s surface. The resulting images are three-dimensional representations of these surface atoms and molecules.
- The STM’s ability to visualize atomic-scale details on surfaces has revolutionized the field of surface science, providing insights into atomic arrangements and molecular architectures.
In conclusion, the realm of electron microscopy is vast and varied, with each type of microscope offering unique capabilities and insights. These specialized instruments, from REM to STM, underscore the versatility and depth of electron microscopy, enabling researchers to delve deeper into the intricacies of materials and biological specimens.
Other Types of Microscopes
There are present other types of Microscopes such as;
- Polarizing microscopes
- Scanning Probe Microscope
- Stereo Microscope
- Inverted Microscopes
1. Polarizing microscopes
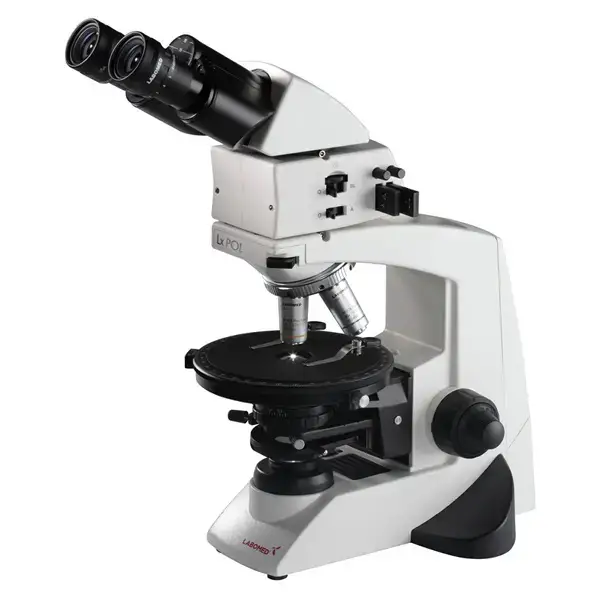
- The Polarizing Microscope stands as a specialized variant of the conventional light microscope, distinguished by its utilization of polarized light for specimen illumination. Unlike standard optical microscopes that employ natural light, the polarizing microscope harnesses light that has undergone polarization, a process that restricts the vibrations of light waves to a singular plane.
- The primary advantage of using polarized light lies in its ability to significantly enhance both the quality and contrast of the resultant image. This enhancement is particularly beneficial when examining specimens with inherent birefringent properties, as the polarized light interacts with these properties to produce distinct and detailed images.
- Often referred to as petrographic microscopes, polarizing microscopes are quintessential in the realm of geology and mineralogy. Their ability to discern between different minerals, especially in thin rock sections, makes them invaluable tools for petrologists.
Polarizing Microscope Principle
The foundational principle of the polarizing microscope revolves around the manipulation of light waves to extract detailed information from anisotropic specimens. Here’s a breakdown of the process:
- Light Source and Polarization: The microscope initiates the process by producing normal light from its illuminator. This light is then directed through a polarizer, which transforms it into plane-polarized light. This means that the light waves now oscillate predominantly in a single plane.
- Interaction with the Specimen: The plane-polarized light is subsequently focused onto an anisotropic specimen. Anisotropic substances are characterized by having multiple refractive indices, which means they interact with light in a unique manner.
- Birefringence Phenomenon: Upon interaction with the anisotropic specimen, the plane-polarized light undergoes a phenomenon known as birefringence. This results in the splitting of the light wave into two distinct waves: the ordinary wave and the extraordinary wave. These waves are orthogonal, meaning they oscillate perpendicular to each other.
- Phase Transmission and Analysis: The two waves, having been transmitted through the specimen, are out of phase due to their different interactions with the specimen’s properties. These waves then encounter an analyzer, a crucial component of the polarizing microscope. The analyzer’s role is to recombine these waves, facilitating their passage through the ocular lens.
- Image Formation: The culmination of this intricate process is the formation of an enlarged, detailed image. The image’s characteristics are influenced by the specimen’s interaction with the polarized light, revealing details that might be invisible under standard light microscopy.
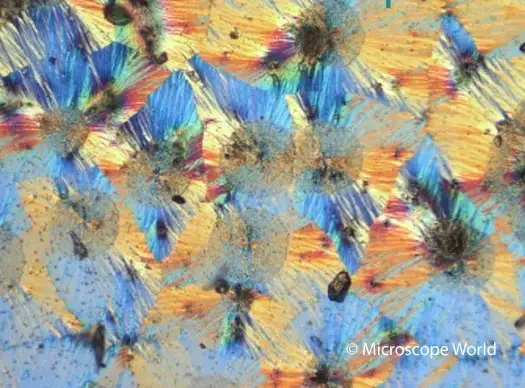
Polarizing Microscope Parts
The polarizing microscope, while sharing similarities with the conventional light microscope, is equipped with specialized components tailored for polarized light microscopy. These components are integral to the microscope’s ability to elucidate intricate details of specimens. Here’s an in-depth look at these parts:
- Polarizer: Positioned between the illuminator and the specimen stage, the polarizer serves a pivotal role. It is a filter designed to convert the unpolarized light, emitted from the light source, into plane-polarized light. This ensures that the light interacting with the specimen oscillates predominantly in a singular plane.
- Analyzer: Situated in the optical pathway above the objective lenses, the analyzer is another crucial polarizing filter. Its primary function is to recombine the light waves after they have interacted with the specimen. This recombination is vital for the formation of the final image that is observed.
- Accessory Plates: These are specialized plates, including compensators and retardation plates, which are introduced before the analyzer in the optical path. Their role is to determine the optical path difference or the relative retardation between the ordinary and extraordinary waves that arise due to birefringence. By doing so, they enhance the contrast in the images, facilitating a clearer visualization of the specimen’s internal structures.
- Specialized Stage: Unlike the fixed stages found in many conventional microscopes, the polarizing microscope is equipped with a circular stage. This stage is designed to rotate in a full 360° direction. Such a design allows for the precise orientation of the specimen, which is crucial for certain polarized light microscopy techniques.
In summation, the polarizing microscope, through its specialized components, offers a unique perspective on specimens, revealing details that remain hidden under standard microscopy. Each part, from the polarizer to the specialized stage, plays a pivotal role in ensuring the microscope’s efficacy in visualizing intricate specimen details.
Uses of Polarizing Microscope
The polarizing microscope, with its unique ability to utilize polarized light for specimen illumination, finds applications in a diverse range of scientific fields. Here are the primary uses of this specialized microscope:
- Geological Studies: One of the foremost applications of the polarizing microscope is in the realm of geology. Geologists employ this microscope to meticulously examine and characterize various geological specimens. This includes the detailed study of rocks, minerals, and the intricate components of soil. Through the polarizing microscope, the crystalline structures, mineral composition, and other microscopic features of these specimens are elucidated.
- Biological Research: Beyond the realm of geology, the polarizing microscope also finds significant utility in biological studies. It is particularly useful for investigating transparent biological entities. For instance, it aids in the detailed examination of planktons, diatoms, and protozoans. By employing polarized light, the internal structures of these organisms, which might remain obscured under conventional microscopy, are vividly revealed.
Limitations of Polarizing Microscope
The polarizing microscope, while offering unique advantages in certain scientific domains, is not without its limitations. These constraints, inherent to its design and operational principles, are outlined below:
- Necessity for Anisotropic Specimens: One of the primary limitations of the polarizing microscope is its dependency on anisotropic specimens. Anisotropic materials possess different properties in different directions, which is essential for the microscope to produce the desired birefringence effect. Consequently, isotropic materials, which exhibit uniform properties in all directions, are not suitable for examination under a polarizing microscope. This requirement restricts the range of specimens that can be effectively studied using this technique.
- Limited Scope of Application: The specialized nature of the polarizing microscope means that its applications are somewhat niche. While it excels in specific fields like geology and certain biological studies, it may not be the instrument of choice for a broad spectrum of scientific investigations. Its utility is primarily confined to those areas where the study of birefringence or the properties of anisotropic materials is paramount.
In summary, while the polarizing microscope offers unparalleled insights into certain types of specimens, its specialized nature and the requirement for anisotropic materials limit its broader applicability in the realm of microscopy.
2. Scanning Probe Microscope
A scanning probe microscope (SPM) is a type of microscope that uses a very sharp probe to scan the surface of a sample and create high-resolution images of its topography. SPMs are capable of producing images with a resolution of just a few nanometers, making them useful for studying the properties of small structures and surfaces.
There are several different types of SPMs, including atomic force microscopes (AFMs), scanning tunneling microscopes (STMs), and scanning near-field optical microscopes (SNOMs). These instruments work by using a probe to interact with the sample in various ways, such as by measuring the force between the probe and the sample, by tunneling electrons through the gap between the probe and the sample, or by measuring the near-field optical properties of the sample.
SPMs are widely used in a variety of fields, including materials science, biology, and nanotechnology, to study the surface properties of samples and to analyze the structure and composition of small structures. They are also used to fabricate and manipulate small structures, such as nanowires and nanotubes, and to study the properties of individual atoms and molecules.
Principle of Scanning Probe Microscope
The probe tip of the scanning probe microscope is affixed to the end of a cantilever. The tip is so sharp that it can glide precisely and precisely across the sample’s surface, scanning each and every atom. The tip is positioned close to the surface of the sample, causing the cantilever to deflect under the influence of forces. This distance of deflection is measured by the laser. After scanning, the final image is obtained on a computer.
Types of Scanning Probe Microscope
There are several different types of scanning probe microscopes (SPMs), each of which uses a different method to scan the surface of a sample and measure its properties. Some common types of SPMs include:
- Atomic force microscopes (AFMs): AFMs use a sharp, cantilevered probe to measure the forces between the probe and the sample as the probe is scanned across the surface. The probe is typically made of a very hard and stiff material, such as silicon, and is attached to a piezoelectric element that moves the probe in very small increments. AFMs can be used to measure the topography of a sample, as well as its electrical, mechanical, and magnetic properties.
- Scanning tunneling microscopes (STMs): STMs use a very sharp probe to measure the tunneling current between the probe and the sample as the probe is scanned across the surface. The probe is typically made of a metallic material, such as tungsten, and is mounted on a piezoelectric element that moves the probe in very small increments. STMs can be used to measure the topography of a sample and to study the electronic properties of surfaces.
- Scanning near-field optical microscopes (SNOMs): SNOMs use a probe with a very sharp tip, typically made of a metallic material such as gold, to measure the near-field optical properties of a sample. The probe is typically mounted on a piezoelectric element that moves the probe in very small increments. SNOMs can be used to study the optical properties of samples at the nanoscale, such as the absorption and scattering of light.
- Scanning capacitance microscopes (SCMs): SCMs use a probe with a sharp tip to measure the capacitance between the probe and the sample as the probe is scanned across the surface. The probe is typically made of a metallic material and is mounted on a piezoelectric element that moves the probe in very small increments. SCMs can be used to measure the topography of a sample and to study its electrical properties.
- Scanning Kelvin probe microscopes (SKPMs): SKPMs use a probe with a sharp tip to measure the electrostatic potential of a sample as the probe is scanned across the surface. The probe is typically made of a metallic material and is mounted on a piezoelectric element that moves the probe in very small increments. SKPMs can be used to measure the topography of a sample and to study its electrical properties.
Application of Scanning Probe Microscope
- It is used to examine various sample qualities, such as electrical properties.
- This microscope is used to analyse the magnetic property of the sample.
- With the use of this microscope, information on the sample can be transferred.
3. Dissecting Microscope (Stereo Microscope)
The Dissecting Microscope, commonly referred to as the Stereo Microscope, stands as a distinct category within the realm of light microscopy. Unlike traditional microscopes that transmit light through specimens, the dissecting microscope operates on the principle of reflected light microscopy.
- The core functionality of the dissecting microscope is based on the reflection of light off the surface of the specimen. This reflected light is then captured by the microscope’s optics to produce a magnified image.
- The unique design and optical arrangement of the dissecting microscope allow for the visualization of three-dimensional specimens. This is in contrast to many other light microscopes, which are designed primarily for the examination of thin, two-dimensional specimens mounted on slides.
- As the name suggests, the dissecting microscope is an invaluable tool in the realm of biological dissections. It provides researchers and students with a clear, magnified view of their specimen, facilitating precision in their work.
- Beyond dissections, the stereo microscope’s ability to visualize three-dimensional objects makes it a versatile instrument, suitable for a range of applications from examining mineral samples in geology to inspecting electronic components in technology.
In conclusion, the dissecting microscope, with its unique design and capability to produce three-dimensional images, offers a different perspective compared to traditional light microscopes. Its versatility and ease of use make it an essential tool in various scientific and industrial fields.
Stereo Microscope Diagram
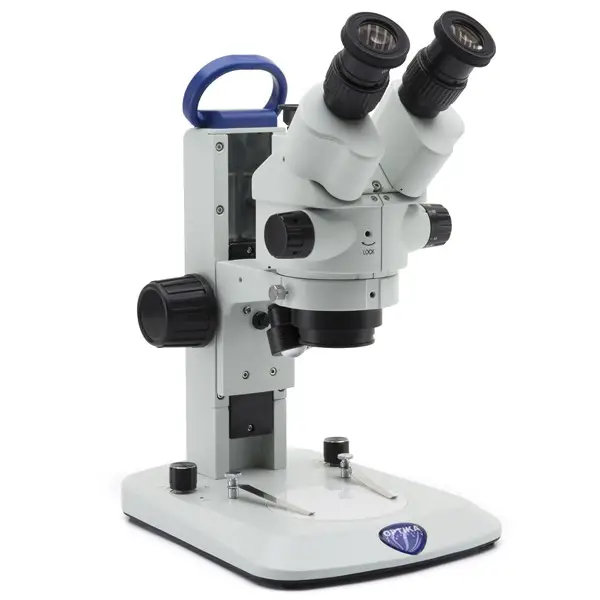
Principle of Stereo Microscope
The Dissecting Microscope, also known as the Stereo Microscope, operates on a distinct optical principle that sets it apart from traditional compound microscopes. At its core, the stereo microscope is designed to provide a three-dimensional perspective of the specimen under examination.
Optical Principle:
- The foundational principle of the stereo microscope lies in its dual optical pathways. Unlike the singular pathway in conventional microscopes, the stereo microscope employs two separate light paths, each corresponding to one of the ocular lenses. This bifurcated light path is what facilitates the generation of a stereoscopic or three-dimensional image.
- Each of the two ocular lenses focuses on a slightly different angle of the specimen, capturing two distinct images. When viewed together, these images converge to produce a depth perception, allowing the observer to discern the three-dimensional structure of the specimen.
Illumination System:
- The stereo microscope is equipped with both top and bottom illumination systems. The top light, often referred to as incident light, illuminates the surface of the specimen, making it particularly useful for dissecting or examining opaque objects.
- Conversely, the bottom light, or transmitted light, shines through the specimen, enhancing the visibility of transparent or semi-transparent samples.
Objective Lenses and Stage Design:
- The objective lenses of the stereo microscope are housed within a cylindrical cone, distinguishing it from the exposed objectives of compound microscopes. This design not only protects the lenses but also facilitates the microscope’s unique optical configuration.
- Accommodating the needs of various specimens, the stage of the stereo microscope is typically larger than that of its compound counterpart. A notable feature is the inclusion of a groove or restraining mechanism, ensuring the stability of the specimen during examination.
In summation, the Dissecting or Stereo Microscope employs a dual optical pathway system, combined with specialized illumination techniques, to render a three-dimensional visualization of specimens. This unique design and principle make it an indispensable tool in fields requiring detailed, depth-perceptive observations.
Applications of Stereo Microscope
The Dissecting Microscope, commonly referred to as the Stereo Microscope, is a versatile instrument with a wide range of applications across various scientific and technical domains. Its unique design, which offers a three-dimensional view of specimens, makes it particularly suited for tasks requiring intricate detail and depth perception. Here are some of the primary uses of the Dissecting or Stereo Microscope:
- Biological Procedures:
- Dissection and Microsurgery: The stereo microscope is an indispensable tool in biological laboratories, especially during dissection procedures. Its ability to provide a detailed, three-dimensional view allows for precise manipulation and examination of small biological specimens, tissues, and organs. Additionally, it is employed in microsurgical procedures where precision and depth perception are paramount.
- Archaeological and Geological Examination:
- The microscope is frequently used in the fields of archaeology and geology. Archaeologists utilize it to closely examine and analyze artifacts, ensuring that every detail, no matter how minute, is observed. Similarly, geologists employ the stereo microscope to study rock samples, minerals, and other geological specimens, gaining insights into their composition, structure, and history.
- Electronics and Precision Engineering:
- Nano Electric Appliance Manufacturing and Repair: The intricate nature of modern electronics, from microchips to circuit boards, necessitates tools that offer precision and clarity. The stereo microscope is invaluable in the manufacturing, inspection, and repair of such components. Its ability to magnify and provide a three-dimensional view ensures that even the smallest of components can be accurately placed, soldered, or repaired.
- Watchmaking: The world of horology, or watchmaking, requires a keen eye and a steady hand. The stereo microscope aids watchmakers in assembling the tiny components of a watch mechanism, ensuring its accurate and reliable function.
- Mobile Phone Repair: With the increasing complexity of mobile phones, repairing them requires a tool that can provide a clear view of their intricate internal components. The stereo microscope is often used to inspect and repair the minute parts of mobile phones, from circuitry to connectors.
In conclusion, the Dissecting or Stereo Microscope, with its unique optical capabilities, serves a multitude of purposes across diverse scientific and technical fields. Its ability to offer a detailed, three-dimensional perspective makes it an essential instrument for tasks that demand precision and depth of view.
Limitations of Dissecting Microscope or Stereo Microscope
The Dissecting Microscope, also known as the Stereo Microscope, is a valuable tool in various scientific and technical domains due to its ability to provide a three-dimensional view of specimens. However, like all scientific instruments, it comes with its own set of limitations. Here are some of the inherent constraints associated with the Dissecting or Stereo Microscope:
- Limited Applicability:
- While the stereo microscope is indispensable for specific tasks requiring depth perception and detailed observation of three-dimensional objects, its utility is restricted to these particular applications. It may not be the preferred choice for tasks that require high magnification or the examination of cellular and sub-cellular structures.
- Magnification Constraints:
- One of the primary limitations of the stereo microscope is its relatively low magnification capabilities. Unlike compound microscopes or electron microscopes, which can magnify specimens to a much higher degree, the stereo microscope’s magnification range is comparatively limited. This constraint makes it unsuitable for observing ultra-fine details or structures at the cellular or molecular level.
- Economic Considerations:
- The stereo microscope, especially high-quality models with advanced features, can be a significant investment. The cost associated with acquiring, maintaining, and upgrading these microscopes might be prohibitive for some institutions, researchers, or hobbyists, particularly when their usage might be infrequent or specialized.
In summary, while the Dissecting or Stereo Microscope offers unique advantages in terms of depth perception and the observation of three-dimensional specimens, it is essential to be aware of its limitations. Users must carefully consider the specific requirements of their tasks and weigh them against the capabilities and constraints of the microscope to ensure optimal results.
4. Inverted Microscopes
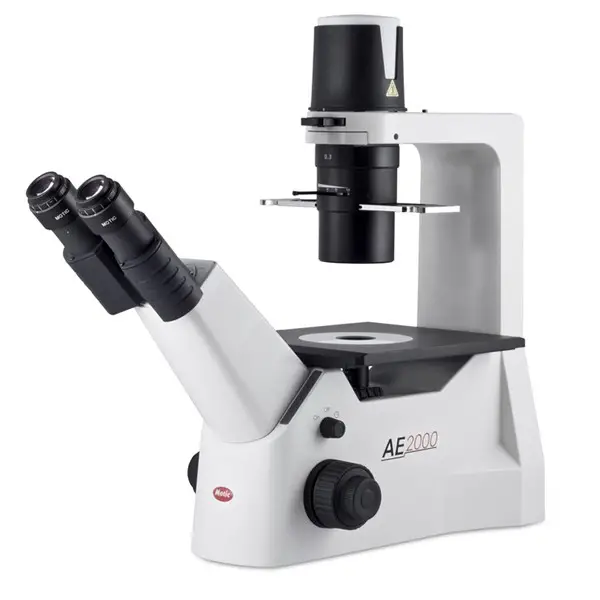
The Inverted Microscope, as the name suggests, is a unique variation of the conventional light microscope, characterized by an inverted design where the positions of key components are reversed. This specialized microscope is tailored to cater to specific observational needs, offering distinct advantages in certain research and clinical applications.
- Design and Configuration: The primary distinction of the Inverted Microscope lies in its structural configuration. Unlike the traditional upright microscope, where the light source and condenser are positioned below the specimen and the objective lenses are above, the Inverted Microscope flips this arrangement. The objective lenses and turret are situated beneath the stage, while the illuminator and condenser are positioned above it. This inversion means that observers must direct their gaze upwards to visualize the specimen.
- Operational Principle: Fundamentally, the Inverted Microscope operates on the same principles as its upright counterpart. It harnesses the properties of light to magnify and elucidate the intricate details of specimens. However, the inverted design facilitates specific observational scenarios, particularly when examining specimens in larger containers or when observing living cells in culture dishes.
- Instrumentation: While the core components of the Inverted Microscope mirror those of standard microscopes, their arrangement is what sets it apart. The light source, typically located at the base in traditional microscopes, is positioned at the top in the inverted design. Conversely, the turret and objective lenses, which are usually above the stage in upright microscopes, are located below the stage in the Inverted Microscope. This design allows for more direct access to the specimen, especially when using larger or unconventional containers.
- Digital Enhancements: With the advent of technology, many modern Inverted Microscopes are equipped with digital cameras. These cameras enable researchers and clinicians to capture high-resolution images or videos of their specimens, facilitating detailed analysis, documentation, and sharing of findings.
In conclusion, the Inverted Microscope is a testament to the adaptability and evolution of microscopy, designed to meet specific observational challenges. Its unique configuration offers researchers and clinicians a versatile tool, especially beneficial for studies involving living cells and tissues.
Uses of Inverted Microscope
The Inverted Microscope, characterized by its unique design with objective lenses positioned below the stage, has found a myriad of applications in various scientific domains. Its specialized configuration facilitates the observation of specimens that might be challenging to examine under conventional upright microscopes. Here, we delve into some of the primary uses of the Inverted Microscope in scientific research.
- Metallurgical Analysis: In the realm of metallurgy, the Inverted Microscope is an indispensable tool. It is employed to scrutinize the microstructure of metals and minerals. By illuminating these specimens from above and observing them from below, researchers can gain insights into the grain structure, phase distribution, and other microstructural features of metallic samples. This information is pivotal in understanding the properties and performance of metals, aiding in the development of new alloys and improving existing ones.
- Cytological Studies: The field of cytology, which focuses on the study of cells, also benefits from the capabilities of the Inverted Microscope. Researchers utilize this instrument to closely monitor the process of cell division, allowing them to observe the intricate stages of mitosis and meiosis in real-time. The microscope’s design is particularly advantageous when studying cells in culture dishes, as it provides unobstructed access to the specimen.
- Microbiological Investigations: In microbiology, the Inverted Microscope proves to be a valuable asset, especially in the identification and study of specific microorganisms. For instance, it is employed in the detection of Mycobacterium tuberculosis, the causative agent of tuberculosis. Additionally, it facilitates the observation of the growth and behavior of certain pathogens, such as Phytophthora spp., in culture. By providing a clear view of these microorganisms, the Inverted Microscope aids in understanding their morphology, life cycle, and interactions with their environment.
In summary, the Inverted Microscope, with its distinct design and features, serves as a versatile instrument in various scientific disciplines. From metallurgy to microbiology, its applications are vast, underscoring its significance in advancing research and enhancing our understanding of the microscopic world.
Limitations of Inverted Microscope
The Inverted Microscope, while a valuable tool in various scientific applications, is not without its limitations. These constraints, inherent to its design and functionality, can sometimes pose challenges to researchers and impede certain microscopic investigations. Here, we elucidate some of the primary limitations associated with the Inverted Microscope.
- Limited Availability and High Cost: One of the primary constraints of the Inverted Microscope is its limited availability in many research settings. Unlike conventional upright microscopes, which are more ubiquitous in laboratories, the Inverted Microscope is less commonly found. Moreover, the specialized design and features of the Inverted Microscope often come with a higher price tag, making it a significant investment for many institutions. This cost factor can be a deterrent for some research facilities, especially those operating with constrained budgets.
- Specimen Thickness Impediments: The design of the Inverted Microscope, which necessitates the placement of specimens above the objective lenses, introduces challenges when working with slides or petri dishes of varying thicknesses. The thickness of the slide or dish can influence the quality of imaging. Specifically, if the slide or dish is too thick, it can introduce aberrations or distortions in the captured images. This limitation necessitates careful consideration of the specimen holder’s dimensions to ensure optimal imaging results.
In conclusion, while the Inverted Microscope offers unique advantages, especially for observing living cells and tissues in culture dishes, it is essential to be cognizant of its inherent limitations. Researchers must weigh these constraints against the microscope’s benefits to determine its suitability for specific applications.
5. Metallurgical Microscopes
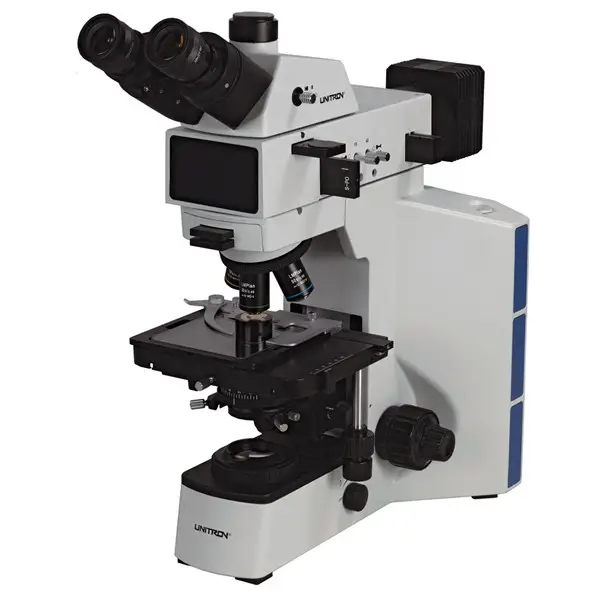
The realm of microscopy has been enriched by the advent of the Metallurgical Microscope, a specialized instrument tailored for the meticulous examination of metals and their intricate structures. This microscope stands distinct from its counterparts, primarily due to its reliance on reflected light as opposed to transmitted light, a feature that enables the detailed observation of opaque specimens.
- Fundamental Design and Instrumentation: At its core, the Metallurgical Microscope shares similarities with the conventional optical microscope in terms of its fundamental design and components. However, the distinguishing feature lies in its illumination system. Instead of allowing light to pass through the specimen, as is the case with transmitted light microscopy, the Metallurgical Microscope employs a method where light is reflected off the surface of the specimen. This approach is crucial, given the opaque nature of the materials it is designed to study.
- Applications in Metallography: The primary domain of the Metallurgical Microscope is metallography, the science of analyzing the physical structure and components of metals and alloys. Through this microscope, scientists and researchers can delve into the microstructures of metals, gaining insights into grain boundaries, inclusions, phases, and other metallurgical phenomena. Such detailed examinations are pivotal in understanding the properties, behavior, and performance of metals under various conditions.
- Scope of Study: While the primary focus of the Metallurgical Microscope is metals and alloys, its utility extends beyond this. The microscope is adept at examining other opaque materials such as ceramics, certain minerals, and rocks. This versatility makes it an invaluable tool in diverse fields, from materials science to geology.
In summation, the Metallurgical Microscope is a testament to the advancements in microscopy, offering a specialized lens through which the intricate world of metals and other opaque materials can be explored with precision and clarity.
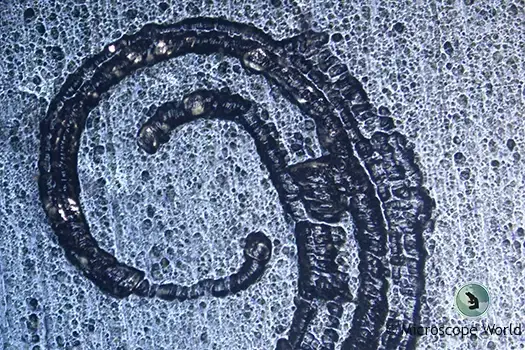
6. The Digital Microscope
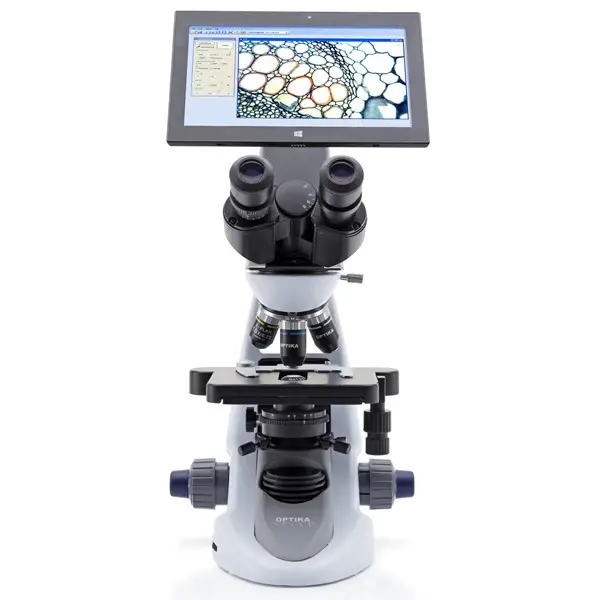
In the ever-evolving realm of microscopy, the Digital Microscope stands as a testament to the seamless integration of traditional optical techniques with modern digital technology. This innovative instrument has revolutionized the way microscopic observations are conducted, offering enhanced visualization, analysis, and sharing capabilities.
- Core Design and Features: Distinct from traditional microscopes, the Digital Microscope eschews the conventional ocular lens. Instead, it incorporates a digital camera, which captures and relays images directly to a screen for digital display. This design is underpinned by a sophisticated computerized system that amalgamates the microscope, camera, display monitor, and specialized software. The absence of eyepieces is compensated by the camera, which serves as the primary medium for capturing microscopic images.
- Image Processing and Analysis: One of the standout features of the Digital Microscope is its advanced image processing capabilities. The integrated software facilitates a myriad of functions, from basic magnification and focusing to intricate analyses such as size measurement and color correction. Furthermore, the software enables users to capture both still images and videos, offering flexibility in documentation and analysis. The ability to manipulate images, adjust color contrast, control brightness, and even produce graphic recordings underscores the versatility of this instrument.
- Three-Dimensional Visualization: Beyond two-dimensional imaging, certain advanced Digital Microscopes are equipped to project three-dimensional images, providing a more comprehensive view of specimens and enhancing depth perception.
- Applications Across Domains: The Digital Microscope’s prowess extends across a multitude of fields. Its precision and advanced imaging capabilities make it an invaluable tool in microbiology, pathology, and cytology. Surgeons leverage its capabilities during intricate procedures, while researchers in nanotechnology benefit from its detailed imaging. Furthermore, its role in forensics and various industrial sectors underscores its versatility. The instrument’s ability to process and display images digitally positions it as a preferred choice over traditional compound microscopes in many scenarios.
In conclusion, the Digital Microscope represents a paradigm shift in microscopy, bridging the gap between optical techniques and digital technology. Its multifaceted capabilities, ranging from advanced image processing to diverse applications, underscore its significance in contemporary scientific and industrial endeavors.
7. USB Microscope
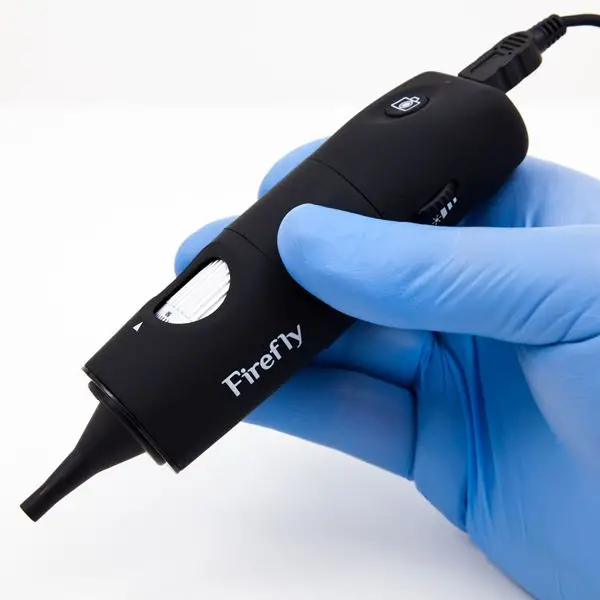
The realm of microscopy has witnessed numerous advancements, and the USB Microscope stands as a testament to the integration of digital technology with traditional microscopic techniques. This instrument, characterized by its simplicity and portability, offers a unique approach to microscopic observation, leveraging the capabilities of modern computers.
- Design and Instrumentation: The USB Microscope is fundamentally a low-power digital microscope, distinguished by its ability to interface directly with a computer via a USB port. At its core, it employs a digital camera equipped with a high-power macro lens, capable of achieving magnifications up to 200X. The design is streamlined, comprising primarily of a light source, typically an LED, the aforementioned digital camera, and the integral USB port for connectivity.
- Operational Mechanism: The operational principle of the USB Microscope is straightforward. The LED illuminates the specimen, and the light reflected from the specimen is captured by the digital camera. This image is then transmitted digitally to the connected computer, where it is displayed on the screen. The digital nature of the microscope allows for the captured images or videos to be saved, edited, or processed using specialized computer software.
- Applications and Utility: The USB Microscope finds its niche in a variety of applications. It is adept at examining objects such as insects, coins, gemstones, jewelry, intricate scripts, and crystalline structures. Beyond these, it also finds utility in medical examinations, particularly in endoscopic and ENT procedures, offering a detailed view of internal structures.
- Advantages and Limitations: One of the standout features of the USB Microscope is its affordability and portability. Its compact design and direct computer interface make it a convenient tool for both professionals and hobbyists. However, it’s worth noting that while it offers several advantages, it does come with its set of limitations, primarily its relatively low magnification when compared to more advanced microscopes.
In summation, the USB Microscope represents a fusion of traditional microscopy with digital technology, offering a unique and accessible means of microscopic exploration. While it may not replace high-end microscopes in specialized applications, its versatility and ease of use make it a valuable tool for a wide range of users.
8. The Pocket Microscope
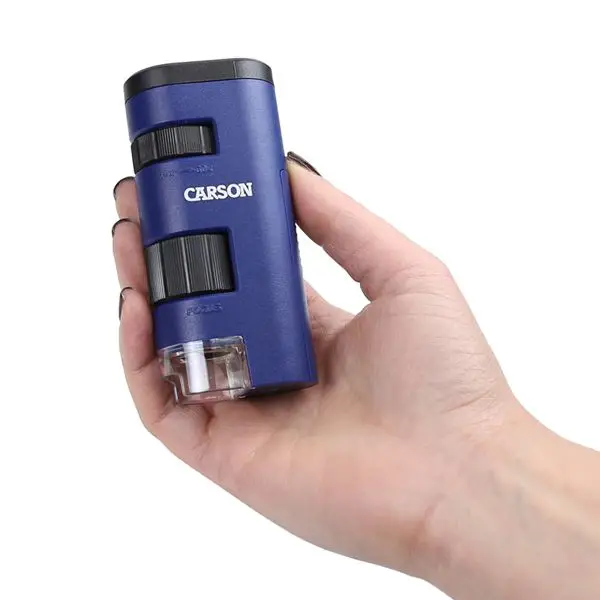
The realm of microscopy, traditionally dominated by large, stationary instruments, has seen the emergence of compact, portable devices designed for on-the-go observations. The Pocket Microscope stands as a testament to this evolution, offering a blend of convenience and functionality.
- Design and Components: The Pocket Microscope is characterized by its diminutive size, designed specifically for easy portability. Despite its compact nature, it houses essential components integral to its operation. At its core, it features an eyepiece for direct observation. Illumination is provided by an LED, powered by an onboard battery. To aid in the reflection and direction of light onto the specimen, a mirror is incorporated into the design. Furthermore, a stage is present to securely hold and position the sample for observation. Some advanced variants of the Pocket Microscope also come equipped with a digital camera, allowing users to capture and store images of their observations.
- Applications and Utility: Given its design and magnification capabilities, the Pocket Microscope is best suited for general observational purposes. It excels in examining objects that are millimeter-scale in size. This makes it particularly useful for inspecting jewelry, gemstones, intricate components in watchmaking, electronic circuits, insects, and other similar objects.
- Limitations: While the Pocket Microscope offers the advantage of portability and ease of use, it does come with inherent limitations. Its magnification capability, typically capped at around 100X, restricts its ability to observe truly microscopic entities, such as microorganisms. As such, it cannot replace the functionality of more advanced, high-magnification microscopes in specialized applications.
In conclusion, the Pocket Microscope represents a significant stride in making microscopy accessible and convenient for a broader audience. While it may not cater to the needs of advanced scientific research, its versatility and portability make it an invaluable tool for a myriad of general observational purposes.
9. The Acoustic Microscope
Acoustic microscopy stands as a distinctive branch within the realm of microscopy, diverging from traditional methods by harnessing the power of high-frequency ultrasound waves, rather than light or electrons, to generate detailed images of specimens. This innovative approach offers a unique perspective, particularly in visualizing the internal structures of objects.
Fundamental Principle
Central to the operation of an acoustic microscope is the principle of ultrasonic wave propagation and reflection. A transducer, acting as the heart of the system, plays a dual role: it transforms electrical signals into ultrasonic waves and vice versa. When these sound waves, typically ranging from 5 MHz to 400 MHz, are directed towards a specimen, they interact with it, leading to both reflection and transmission of waves. The nature of these interactions, whether it’s the amplitude, phase, or time of return of the reflected sound, is meticulously processed to generate an image.
Imaging Modalities
Two primary imaging techniques are employed in acoustic microscopy:
- Pulse-Echo Mode: Utilizing a singular transducer, this method focuses on analyzing the reflected sound waves or echoes.
- Transmission Mode: This technique employs a pair of transducers, with one capturing transmitted sound waves and the other detecting reflected waves. In both methodologies, meticulous scanning of the specimen, pixel by pixel, results in the formation of a two-dimensional image.
Instrumentation
Beyond the pivotal transducer, which acts both as a loudspeaker and microphone, the acoustic microscope relies on computer software to interpret the transformed electrical signals into coherent images. This system’s prowess lies in its ability to delve into the internal intricacies of specimens, capturing even submicron-level features without compromising the integrity of the sample.
Applications
The acoustic microscope’s capabilities have found resonance across various sectors:
- Electronics: Instrumental in quality assurance and production, especially in imaging circuit boards and identifying microchip defects.
- Industrial: Employed across the chemical, pharmaceutical, and ceramic industries for product quality assessments.
- Biomedical: Offers insights into cellular structures, motility, and elasticity, proving invaluable for cytological and histological examinations.
Limitations
Despite its innovative approach and diverse applications, acoustic microscopy is not without its challenges:
- Sound propagation necessitates a medium.
- Sound manipulation poses difficulties.
- The image processing time can be protracted.
- The associated costs can be prohibitive.
In summation, acoustic microscopy, with its unique sound-based imaging technique, offers a fresh perspective in the microscopy domain. While it presents certain challenges, its potential applications across industries underscore its significance in modern microscopy.
10. X-Ray Microscopes
The X-Ray Microscope, distinct from traditional optical microscopes, employs X-rays to illuminate specimens, yielding magnified images. This technique’s inherent advantage lies in X-rays’ ability to penetrate most materials, eliminating the need for specialized sample preparation or staining. Typically, X-rays ranging from 100 to 1,000 eV, equivalent to a wavelength of approximately 1 nm, are utilized. However, contemporary X-Ray microscopes have evolved to use wavelengths between 0.1 to 10 nm.
Underlying Principle
The foundational principle of X-Ray microscopy is rooted in the interaction between matter and X-rays. When molecules encounter X-rays, ionization ensues. This interaction excites the electrons of the atom to a higher energy state. As these electrons revert to their ground state, they release energy in the form of X-rays, which possess a specific energy and wavelength characteristic of the element in question.
Upon illuminating the specimen with high-energy X-rays, various interactions occur: some X-rays scatter, others penetrate the sample, and a fraction is absorbed. The ionized molecules subsequently emit X-rays, which are captured either photographically or through a detection system, culminating in the formation of an image.
Instrumentation
- X-ray Tube: This component, reminiscent of cathode ray tubes, is responsible for X-ray generation. It houses a vacuum system with a metallic anode and a tungsten filament cathode. When subjected to high voltage, the cathode emits electron beams that, upon striking the anode, release X-ray photons.
- Collimator: This device focuses the generated X-rays into a parallel beam, a process termed collimation. It comprises two sets of metallic plates, allowing only a narrow beam to pass, resulting in a collimated X-ray beam.
- Monochromator: This optical apparatus polarizes an unpolarized X-ray beam. It can either be a filter or a crystal, such as quartz or NaCl.
- Detection System: This encompasses various detectors:
- Imaging Detectors: Utilize photographic plates or X-ray films.
- Scintillator Detectors: Comprise a scintillator and a photomultiplier tube. Upon X-ray interaction, scintillators emit visible light, which is then converted into electric pulses.
- Semiconductor Detectors: These employ silicon and lithium plates. X-ray interaction produces an electron and a hole, which are detected and processed to form images.
- Other detectors, such as the Geiger-Muller tube and Proportional counter, are occasionally employed.
Applications
X-Ray microscopes have found utility across various domains:
- Crystal and polymer identification and characterization.
- Quality control in metallurgy, petroleum, ceramics, and glass manufacturing sectors.
- Geological studies encompassing rock, mineral, and soil composition analysis.
Limitations
Despite its potential, X-Ray microscopy is not devoid of challenges:
- Imaging can be intricate.
- The process is costly, time-intensive, and necessitates intricate instrumentation.
11. Near-infrared microscopes
- Near-infrared microscopes (NIR microscopes) are a type of microscope that uses near-infrared light to produce images of samples. Near-infrared light has a wavelength that is slightly longer than visible light and is therefore not visible to the human eye. NIR microscopes are often used to study the structure and function of tissues and cells, as well as the distribution and interaction of molecules within these systems.
- NIR microscopes work by illuminating the sample with near-infrared light and detecting the light that is scattered or absorbed by the sample. The resulting image can provide information about the distribution and concentration of various molecules within the sample. NIR microscopes are particularly useful for studying biological samples, as many biological molecules absorb near-infrared light and can therefore be visualized using this technique.
- NIR microscopes are commonly used in fields such as biology, materials science, and medicine to study the structure and function of tissues and cells, as well as the distribution and interaction of molecules within these systems. They are also used to study the properties of materials and to analyze the chemical composition of samples.
12. Raman microscopes
- Raman microscopes are a type of microscope that uses lasers to excite vibrations in the bonds of molecules, which can then be detected and used to identify the molecules present in a sample. Raman microscopes work by shining a laser beam onto the sample and detecting the light that is scattered by the sample. The intensity and wavelength of the scattered light are unique for each type of molecule, and can be used to identify the specific molecules present in the sample.
- Raman microscopes are commonly used in fields such as materials science, biology, and chemistry to study the chemical composition of samples and to analyze the properties of materials. They are particularly useful for studying samples that are difficult to analyze using other techniques, such as samples that are too small or too complex to be studied with other types of microscopes.
- Raman microscopes are also useful for studying samples that are too weak or too unstable to be studied using other techniques, such as samples that are sensitive to heat or other forms of damage. They are also used to study the structure and function of tissues and cells, and to analyze the distribution and interaction of molecules within these systems.
Differences between Simple Microscope and Compound Microscope
Simple microscopes and compound microscopes are both types of optical microscopes that use lenses and light to magnify and analyze samples. However, there are several key differences between these two types of microscopes:
- Magnification: Simple microscopes have a lower magnification compared to compound microscopes. Simple microscopes typically have a magnification range of 2x to 10x, while compound microscopes can have a magnification range of up to 1000x or more.
- Objective lenses: Simple microscopes have a single objective lens, while compound microscopes have multiple objective lenses that can be used to achieve different levels of magnification.
- Illumination: Simple microscopes typically use natural light or an external light source, such as a lamp, to illuminate the sample. Compound microscopes often have an internal light source, such as a bulb or a LED, that is used to illuminate the sample.
- Eyepieces: Simple microscopes have a single eyepiece, while compound microscopes have two eyepieces that are used to provide a three-dimensional view of the sample.
- Image quality: Simple microscopes often produce lower quality images compared to compound microscopes due to their lower magnification and less sophisticated optical system. Compound microscopes are capable of producing higher resolution images with greater detail.
- Applications: Simple microscopes are often used for basic observations and are suitable for examining larger, more transparent samples. Compound microscopes are more powerful and are commonly used for more advanced studies and for examining smaller, more opaque samples.
Differences between Phase-contrast Microscope and Fluorescent Microscope
Phase-contrast microscopes and fluorescent microscopes are two different types of microscopes that are used to observe and study samples at a very small scale.
Phase-contrast microscopes use a special type of objective lens that is designed to enhance the contrast of samples that are transparent or have low refractive indices. This makes it easier to see and study the details of these types of samples. Phase-contrast microscopes do not require the use of dyes or stains, as the contrast is created through the interaction of light with the sample itself.
Fluorescent microscopes, on the other hand, use special dyes or stains that are applied to the sample and emit light when exposed to a specific wavelength of light. This allows researchers to visualize specific structures or molecules within the sample. Fluorescent microscopes require the use of a special light source, called a fluorescence lamp, to excite the fluorophores in the sample and emit light.
There are a few key differences between phase-contrast microscopes and fluorescent microscopes:
- Image contrast: Phase-contrast microscopes enhance the contrast of transparent or low-index samples through the interaction of light with the sample, while fluorescent microscopes use dyes or stains to create contrast.
- Sample preparation: Phase-contrast microscopes do not require the use of dyes or stains, while fluorescent microscopes require the application of special dyes or stains to the sample.
- Light source: Phase-contrast microscopes use standard light sources, while fluorescent microscopes require a special light source, called a fluorescence lamp, to excite the fluorophores in the sample.
- Image resolution: Fluorescent microscopes typically have higher resolution than phase-contrast microscopes, as the use of dyes or stains can help to enhance the contrast and clarity of the sample.
Overall, phase-contrast microscopes and fluorescent microscopes are both useful tools for studying samples at a very small scale, but they are used in different ways and for different purposes.
Differences between Scanning Electron Microscope (SEM), Transmission Electron Microscope (TEM) and Confocal Microscopy
Scanning electron microscopes (SEM), transmission electron microscopes (TEM), and confocal microscopes are all types of microscopes that are used to observe and study samples at a very small scale. However, they use different techniques and have different capabilities.
- Scanning electron microscopes (SEM): SEMs use a focused beam of electrons to scan the surface of a sample and create an image of its surface features. They are particularly useful for studying the surface features of samples, such as the topography and composition of materials. SEMs can achieve high resolution and can be used to study samples at a variety of scales, from micrometers to nanometers.
- Transmission electron microscopes (TEM): TEMs use a beam of electrons that is transmitted through a sample to create an image of the internal structure of the sample. They are particularly useful for studying the microstructure and internal features of samples, such as the arrangement of atoms and molecules. TEMs can achieve very high resolution and are often used to study samples at the nanoscale.
- Confocal microscopes: Confocal microscopes use a laser to scan a sample and create an image by focusing the laser onto a small, specific area of the sample. They are particularly useful for studying samples with a high degree of depth or thickness, as they can create detailed images of the sample’s structure at different depths. Confocal microscopes are often used to study biological samples, such as cells and tissues.
There are a few key differences between these types of microscopes:
- Image contrast: SEMs create images based on the interaction of electrons with the sample surface, while TEMs create images based on the interaction of electrons with the internal structure of the sample. Confocal microscopes create images based on the reflection of a laser off the sample.
- Sample preparation: SEMs and TEMs typically require samples to be prepared in a specific way, such as by coating them with a thin layer of metal or by embedding them in a resin. Confocal microscopes typically do not require any special sample preparation.
- Resolution: TEMs can achieve the highest resolution of the three types of microscopes, followed by SEMs and confocal microscopes.
- Scale: SEMs and TEMs can be used to study samples at a variety of scales, from micrometers to nanometers, while confocal microscopes are typically used to study samples at the micrometer scale.
Differences Between Bright-field Microscope and Dark-Field Microscope
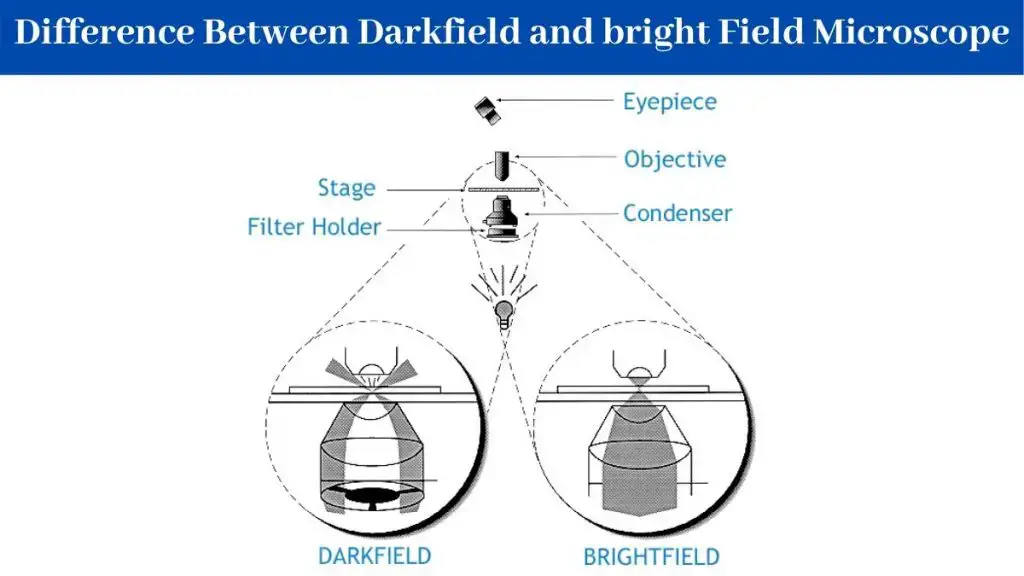
There are several key differences between bright-field microscopy and dark-field microscopy:
- Illumination: In bright-field microscopy, the sample is illuminated from below, and the light passes through the transparent or semi-transparent sample and is focused by the objective lens onto the eyepiece. In dark-field microscopy, the light source is located below the stage and the sample is illuminated from the side at a shallow angle. The light that is scattered by the sample is focused by the objective lens onto the eyepiece.
- Image contrast: In bright-field microscopy, the sample appears bright against a dark background. In dark-field microscopy, the sample appears bright against a dark background.
- Sample types: Bright-field microscopy is best suited for studying opaque or semi-transparent samples, while dark-field microscopy is best suited for studying transparent or semi-transparent samples.
- Details and structures: Bright-field microscopy is not as effective at observing fine details and structures as dark-field microscopy.
- Color: Bright-field microscopy can produce a true-color image of the sample, while dark-field microscopy cannot.
- Field of view: Bright-field microscopy has a wider field of view compared to dark-field microscopy.
- Operator skill: Dark-field microscopy requires a highly skilled operator to properly align the microscope and adjust the light source.
Differences between Scanning Probe Microscope and Stereo Microscope
Scanning probe microscopes (SPMs) and stereo microscopes are two different types of microscopes that are used to observe and study samples at a small scale. They have different capabilities and are used for different purposes.
- Scanning probe microscopes (SPMs): SPMs use a very small probe, often just a few nanometers in size, to scan the surface of a sample and create an image of its surface features. SPMs are particularly useful for studying the surface features of samples at the nanoscale, such as the topography and composition of materials. There are several different types of SPMs, including atomic force microscopes (AFMs), scanning tunneling microscopes (STMs), and scanning near-field optical microscopes (SNOMs).
- Stereo microscopes: Stereo microscopes, also known as dissecting microscopes or low-power microscopes, use two objective lenses to create a three-dimensional image of the sample. They are particularly useful for studying samples that have a high degree of depth or thickness, such as biological samples or small mechanical parts. Stereo microscopes are often used for dissection, inspection, and assembly tasks.
There are a few key differences between these types of microscopes:
- Image contrast: SPMs create images based on the interaction of the probe with the sample surface, while stereo microscopes create images based on the refraction of light through the sample.
- Sample preparation: SPMs typically do not require any special sample preparation, while stereo microscopes may require samples to be mounted on a slide or in a special holder.
- Resolution: SPMs can achieve very high resolution, often at the atomic scale, while stereo microscopes typically have lower resolution.
- Scale: SPMs are typically used to study samples at the nanoscale, while stereo microscopes are typically used to study samples at the micrometer scale.
Overall, SPMs and stereo microscopes are both useful tools for studying samples at a small scale, but they are used for different purposes and have different capabilities.
Differences between Electron Microscope and Compound Microscope
Electron microscopes and compound microscopes are two different types of microscopes that are used to observe and study samples at a small scale. They have different capabilities and are used for different purposes.
- Electron microscopes: Electron microscopes use a beam of electrons to create an image of the sample. There are two main types of electron microscopes: transmission electron microscopes (TEMs) and scanning electron microscopes (SEMs). TEMs create an image of the internal structure of the sample by transmitting a beam of electrons through the sample, while SEMs create an image of the surface features of the sample by scanning the surface with a beam of electrons. Electron microscopes can achieve very high resolution and are often used to study samples at the nanoscale.
- Compound microscopes: Compound microscopes use light to create an image of the sample. They have two main components: an objective lens that is located close to the sample and a eyepiece lens that is located near the observer’s eye. Compound microscopes are often used to study biological samples, such as cells and tissues, as well as small mechanical parts. They can achieve high resolution, but are typically limited to studying samples at the micrometer scale.
There are a few key differences between these types of microscopes:
- Image contrast: Electron microscopes create images based on the interaction of electrons with the sample, while compound microscopes create images based on the refraction of light through the sample.
- Sample preparation: Electron microscopes often require samples to be prepared in a specific way, such as by coating them with a thin layer of metal or by embedding them in a resin. Compound microscopes typically do not require any special sample preparation.
- Resolution: Electron microscopes can achieve very high resolution, often at the atomic scale, while compound microscopes typically have lower resolution.
- Scale: Electron microscopes are typically used to study samples at the nanoscale, while compound microscopes are typically used to study samples at the micrometer scale.
Overall, electron microscopes and compound microscopes are both useful tools for studying samples at a small scale, but they are used for different purposes and have different capabilities.
Types of Microscopes with their applications ppt
Types of Microscopes with their applications Video
FAQ
Q1. What are two types of electron microscopes?
There are two main types of electron microscopes: transmission electron microscopes (TEMs) and scanning electron microscopes (SEMs).
- Transmission electron microscopes (TEMs): TEMs use a beam of electrons to create an image of a thin sample by transmitting the electrons through the sample and recording the resulting image on a fluorescent screen or a digital detector. TEMs are capable of producing high-resolution images of samples with a resolution of just a few nanometers, making them useful for studying the internal structure of materials and small biological specimens.
- Scanning electron microscopes (SEMs): SEMs use a beam of electrons to create an image of the surface of a sample by scanning the surface with the beam and recording the resulting image on a fluorescent screen or a digital detector. SEMs are capable of producing high-resolution images of the surface features of a sample, with a resolution of just a few nanometers. They are commonly used to study the surface features of materials and biological specimens.
Q2. What are the types of microscopes?
There are several different types of microscopes, each of which uses a different method to magnify and analyze samples. Some common types of microscopes include:
- Optical microscopes: These use lenses and light to magnify and analyze samples, and include microscopes such as compound microscopes, stereo microscopes, and polarizing microscopes.
- Electron microscopes: These use a beam of electrons to create an image of a sample and include transmission electron microscopes (TEMs) and scanning electron microscopes (SEMs).
- Scanning probe microscopes (SPMs): These use a very sharp probe to scan the surface of a sample and create high-resolution images of its topography. Examples include atomic force microscopes (AFMs), scanning tunneling microscopes (STMs), and scanning near-field optical microscopes (SNOMs).
- Confocal microscopes: These use lasers and specialized optics to produce high-resolution images of samples by selectively illuminating small areas of the sample at a time.
- Fluorescence microscopes: These use fluorescent dyes to highlight specific structures or molecules in a sample and are commonly used in biological research.
- X-ray microscopes: These use X-rays to produce images of the internal structure of samples and are often used to study materials and biological specimens.
- Near-infrared microscopes: These use near-infrared light to produce images of samples and are often used to study the structure and function of tissues and cells.
- Raman microscopes: These use lasers to excite vibrations in the bonds of molecules, which can then be detected and used to identify the molecules present in a sample.
Q3. which of the following types of microscopes reveals the surface features of small molecules?
Scanning electron microscopes (SEMs) are capable of revealing the surface features of small molecules. SEMs use a beam of electrons to create an image of the surface of a sample by scanning the surface with the beam and recording the resulting image on a fluorescent screen or a digital detector. SEMs are capable of producing high-resolution images of the surface features of a sample, with a resolution of just a few nanometers. They are commonly used to study the surface features of materials and biological specimens, including small molecules.
Q4. Which of the following types of microscopes can magnify more than 2000x?
Transmission electron microscopes (TEMs) are capable of magnifying samples by more than 2000x. TEMs use a beam of electrons to create an image of a thin sample by transmitting the electrons through the sample and recording the resulting image on a fluorescent screen or a digital detector. TEMs are capable of producing high-resolution images of samples with a resolution of just a few nanometers, and are capable of magnifying samples by more than 2000x. They are commonly used to study the internal structure of materials and small biological specimens.
Q5. Explain why the several different types of microscopes are all necessary?
There are several different types of microscopes because each type of microscope is designed to analyze samples in a specific way and is best suited to certain types of applications. Different types of microscopes use different techniques to magnify and analyze samples, and each type has its own unique capabilities and limitations.
For example, optical microscopes, such as compound microscopes and stereo microscopes, use lenses and light to magnify and analyze samples, and are best suited for studying samples that are transparent or moderately opaque. These types of microscopes are commonly used in fields such as biology, materials science, and engineering to study the surface features of samples and to perform tasks such as dissection, assembly, and inspection.
On the other hand, electron microscopes, such as transmission electron microscopes (TEMs) and scanning electron microscopes (SEMs), use a beam of electrons to create an image of a sample and are best suited for studying samples that are too small or too dense to be studied with an optical microscope. These types of microscopes are commonly used in fields such as materials science, biology, and nanotechnology to study the internal structure of materials and small biological specimens.
Scanning probe microscopes (SPMs), such as atomic force microscopes (AFMs) and scanning tunneling microscopes (STMs), use a very sharp probe to scan the surface of a sample and create high-resolution images of its topography. SPMs are best suited for studying the surface properties of samples at the nanoscale, such as the electrical, mechanical, and magnetic properties of materials.
In summary, the different types of microscopes are all necessary because they allow researchers to study samples in different ways and to analyze a wide range of properties, including the surface and internal structure of materials, the electrical and optical properties of samples, and the topography of surfaces at the nanoscale.
Q6. what types of microscopes are used to study viruses?
There are several different types of microscopes that can be used to study viruses, depending on the properties of the virus and the specific type of analysis that is being performed. Some common types of microscopes that are used to study viruses include:
- Optical microscopes: These use lenses and light to magnify and analyze samples, and can be used to study the size and shape of viruses, as well as their surface features. Examples of optical microscopes that are commonly used to study viruses include compound microscopes, stereo microscopes, and polarizing microscopes.
- Electron microscopes: These use a beam of electrons to create an image of a sample and can be used to study the internal structure of viruses and other small biological specimens. Examples of electron microscopes that are commonly used to study viruses include transmission electron microscopes (TEMs) and scanning electron microscopes (SEMs).
- Scanning probe microscopes (SPMs): These use a very sharp probe to scan the surface of a sample and create high-resolution images of its topography. Examples of SPMs that are commonly used to study viruses include atomic force microscopes (AFMs) and scanning tunneling microscopes (STMs).
- Confocal microscopes: These use lasers and specialized optics to produce high-resolution images of samples by selectively illuminating small areas of the sample at a time. Confocal microscopes can be used to study the structure and distribution of viruses in tissues and cells.
- Fluorescence microscopes: These use fluorescent dyes to highlight specific structures or molecules in a sample and can be used to study the distribution and interaction of viruses with host cells.
- X-ray microscopes: These use X-rays to produce images of the internal structure of samples and can be used to study the internal structure of viruses and other small biological specimens.
- Near-infrared microscopes: These use near-infrared light to produce images of samples and can be used to study the distribution and interaction of viruses with host cells.
- Raman microscopes: These use lasers to excite vibrations in the bonds of molecules, which can then be detected and used to identify the molecules present in a sample. Raman microscopes can be used to study the chemical composition of viruses and other small biological specimens.
Quiz
Which type of microscope uses a beam of electrons to produce an image?
a) Light microscope
b) Transmission Electron Microscope (TEM)
c) Polarizing microscope
d) Acoustic microscope
Which microscope is primarily used for observing live cells in culture?
a) Inverted Microscope
b) Scanning Electron Microscope (SEM)
c) Polarizing microscope
d) X-Ray Microscope
Which microscope uses polarized light to enhance image quality and contrast?
a) Acoustic microscope
b) Digital microscope
c) Polarizing microscope
d) USB microscope
Which microscope is designed for portability and can easily fit in a pocket?
a) Transmission Electron Microscope (TEM)
b) Digital microscope
c) USB microscope
d) Pocket microscope
Which microscope uses sound waves for imaging?
a) Acoustic microscope
b) X-Ray Microscope
c) Polarizing microscope
d) Digital microscope
Which microscope is used to study the internal structures of metals and alloys?
a) Metallurgical Microscope
b) Inverted Microscope
c) Scanning Electron Microscope (SEM)
d) Transmission Electron Microscope (TEM)
Which microscope lacks an ocular lens and displays images digitally?
a) Polarizing microscope
b) Digital microscope
c) Acoustic microscope
d) X-Ray Microscope
Which microscope is used for observing objects at the atomic and molecular level on specimen surfaces?
a) Scanning Tunneling Microscope (STM)
b) Scanning Electron Microscope (SEM)
c) Transmission Electron Microscope (TEM)
d) Acoustic microscope
Which microscope uses X-rays to illuminate samples?
a) Digital microscope
b) Acoustic microscope
c) X-Ray Microscope
d) Polarizing microscope
Which microscope is connected to a computer via a USB port and is used for observing larger objects like insects, coins, and jewelry?
a) Digital microscope
b) USB microscope
c) Polarizing microscope
d) Acoustic microscope
FAQ
What is a microscope?
A microscope is a scientific instrument used to magnify and visualize objects or specimens that are too small to be seen with the naked eye.
What are the main types of microscopes?
The main types of microscopes include optical microscopes (such as compound and stereo microscopes), electron microscopes (such as TEM and SEM), and other specialized microscopes like polarizing microscopes and atomic force microscopes.
How does an optical microscope work?
Optical microscopes use visible light to illuminate specimens. They contain lenses that magnify and focus the light to produce an enlarged image of the specimen.
What is the difference between a compound microscope and a stereo microscope?
Compound microscopes are used for high magnification and are suitable for viewing thin specimens on slides. Stereo microscopes provide lower magnification but offer a 3D view of larger, solid objects.
How do electron microscopes differ from optical microscopes?
Electron microscopes use a beam of electrons instead of visible light to magnify specimens. They offer much higher resolution and can visualize objects at the nanoscale.
What is the purpose of a polarizing microscope?
A polarizing microscope uses polarized light to enhance image quality and contrast, making it particularly useful for studying minerals, geological samples, and materials with birefringent properties.
What are some common applications of electron microscopes?
Electron microscopes are used in various fields, including biology, materials science, and nanotechnology, to study the structure and composition of materials at the microscopic and nanoscopic levels.
Can you explain the working principle of a scanning electron microscope (SEM)?
SEM uses a focused beam of electrons to scan the surface of a specimen. By detecting emitted and backscattered electrons, it creates a 3D image with high resolution.
What are the limitations of optical microscopes compared to electron microscopes?
Optical microscopes have limited resolution and cannot visualize objects smaller than the wavelength of visible light. Electron microscopes can achieve much higher resolution.
Are there portable microscopes available for field use?
Yes, there are portable microscopes like pocket microscopes and USB microscopes designed for fieldwork and on-the-go observations. They are often used for tasks like inspecting jewelry, insects, or small objects.
Reference
- http://www.biologyreference.com/La-Ma/Light-Microscopy.html
- https://en.wikipedia.org/wiki/Scanning_electron_microscope
- https://opticsmag.com/types-of-microscopes/
- https://www.microscopeworld.com/p-3658-types-of-microscopes.aspx
- https://microscopeinternational.com/types-of-microscopes/