Contents
What is Recombinant DNA Technology?
- The process of creating artificial DNA by the mixing of various genetic substances (DNA) from various sources is known as Recombinant DNA technology. This technology has become commonly described as genetic engineering.
- Recombinant DNA technology was first discovered from an investigation into restriction enzymes in years 1968, through Swiss microbiologist Werner Arber,
- Inserting the desired gene in the host’s genome isn’t as easy as it seems. It requires the selection of the gene you want to administer into the host . This is followed by the selection of the best vector which the gene needs to be integrated, and the formation of recombinant DNA.
- So, the recombinant DNA is been introduced to the host. Then it must remain within the host and passed through to offspring.
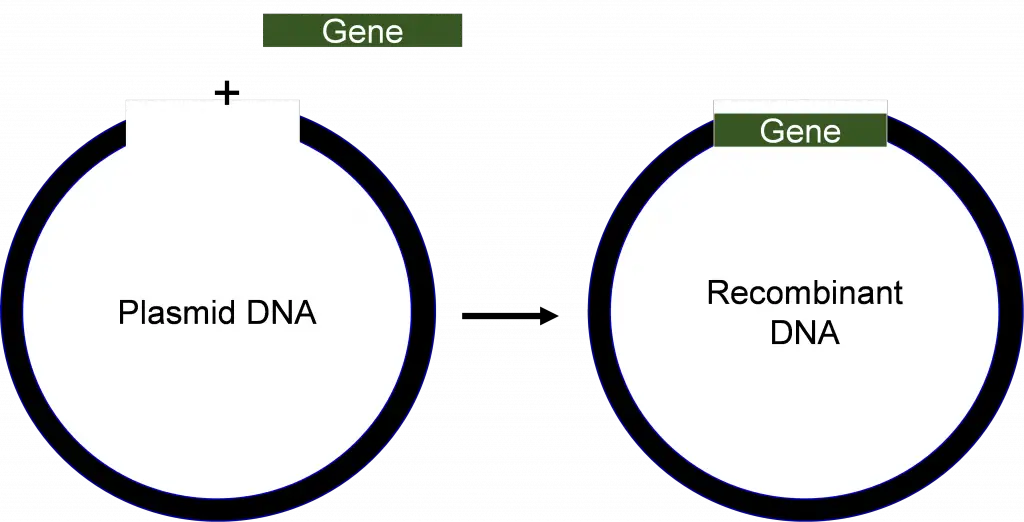
Subject of Recombinant DNA Technology
Over the past few decades, new and powerful ways to study and change DNA have changed the field of genetics in a big way. Biologists can now change the genes of organisms directly for the first time thanks to these techniques.
So, this is called recombinant DNA technology or gene technology. In nature, genetic recombination happens during meiosis, which is a process that both plants and animals go through during the process of making gametes. This is where genetic variation comes from, and this gives us a wide range of natural differences between people in the same population.
In other words, all of the differences between you and me are due to this process of genetic recombination that happened in our parents’ chromosomes before they fused together to make us. Today, we have more information from all areas of biology, such as cytology, genetics, molecular biology, and biochemistry. This means that we can create artificial genetic recombination in the lab, which results in living things with interesting traits. The technology can also be called gene manipulation, gene cloning, genetic modification, and the new genetics, among other things.
Definitions of Recombinant DNA Technology
Here are some ways to describe recombinant DNA technology:
- Recombinant DNA technology, also called genetic engineering, is the controlled manipulation of an organism’s genes to make it better in some way.
- Recombinant DNA technology includes all the different ways to do experiments that change the genes of an organism. It uses molecular cloning, transformation, and recombinant DNA.
- In recombinant DNA technology, the structure of genetic material in a living thing is changed by science. It involves making and using recombinant DNA, which has been used to make bacteria that make insulin and other human proteins.
- Recombinant DNA technology is the branch of biology that deals with splicing and recombining specific genetic units from the DNA of living organisms to make new species or biochemicals.
- Recombinant DNA technology is the development and use of scientific methods, procedures, and technologies that allow direct manipulation of genetic material to change the traits that a cell, organism, or population gets from its genes.
- Recombinant DNA technology includes a number of methods for making unlimited amounts of biological products that would otherwise be hard to find or not available at all. These methods involve putting DNA from animals or plants into bacteria and then taking the biological product from a colony of bacteria, such as human insulin made in bacteria by the human insulin gene.
Basic Principle of Recombinant DNA Technology
Even though there are a lot of different and complicated techniques involved, genetic manipulation is based on fairly simple ideas. The technology is based on the idea that genetic information, which is stored in DNA and organised into genes, is a resource that can be used in different ways to achieve goals in both basic and applied science and medicine.
Every living thing is made up of one or more cells. Each cell’s DNA contains the instructions for making thousands of proteins.
A piece of DNA with the instructions for making a certain protein (called a “gene of interest”) and the right control codes (like “promoter,” “operator,” and “regulator”) can be put into a host cell, where it becomes part of the genome of the host cell.
The protein of interest is then made by growing a lot of the recombinant cell. This recombinant protein, which is stored inside the cell or released into the culture medium, can be recovered, purified, and made into a product that can be used in healthcare, industry, or agriculture.
The host cell can be made up of bacteria, fungi, yeast, or cells from animals. In some cases, we just want to make multiple copies of the inserted gene of interest and don’t want it to be turned into proteins. In this case, we take these copies of the gene that have been made, clean them, and store them.
Goals of RDNA Technology
Here are a few of the goals of this technology:
- Identifying and isolating genes.
- Desired changes in isolated genes.
- Synthesis of new genes in a lab.
- Changing the genome of an organism.
- Diseases that run in families and how to treat them are explained.
- The human genome is being improved.
Tools Of Recombinant DNA Technology
The enzymes that comprise the restriction enzymes aid in cutting the polymerases, they aid in the production of synthesized proteins, and the ligaseshelp to connect. The restriction enzymes that are used in Recombinant DNA technology play a significant role in determining the place where the desired gene will be inserted into the genome of the vector. There are two kinds of them of restriction enzymes, which are Endonucleases as well as Exonucleases.
- The Endonucleases cut through the DNA strand , while the Exonucleases eliminate nucleotides from both ends. The restriction endonucleases are specific to sequences that typically are palindrome sequences that cut DNA at specific places. They examine how long DNA is and then make cuts at a specific location, which is known as”the restriction” site. This results in sticky ends within the sequence. The desired genes as well as their vectors will be cut with similar restriction enzymes that produce the sticky notes in the opposite direction and make the job of ligases straightforward to attach that desired gene with the appropriate vector.
- The vectors assist in carrying and connecting the desired gene. They are a crucial component of the tools used in Recombinant DNA technology since they are the most powerful vehicles to carry forward the desired gene into the host. Plasmids and bacteriophages are among the most frequently used vectors used in the field of recombinant technology and are employed because they have an extremely high copy number. The vectors consist of the replication’s origin. It is a sequence of nucleotides that are the place where replication begins and a marker that can be selected are genes that exhibit resistance to specific antibiotics, such as ampicillin and cloning spots that are identified by the restriction enzymes that are where the desired DNAs are put in.
- Host organisms – where the recombinant genome is introduced. Host is the most powerful device of recombinant DNA technology that takes in the engineered DNA vector that has been crafted with the desired DNA, with the aid of enzymes.
There are many methods by which these DNA recombinants are introduced into the body, including microinjection, biolistics, gene gun alternate heating and cooling, the using calcium ions and so on.
Steps of Genetic Recombination Technology

1. Isolation of Genetic Material
The initial stage in rDNA technique is to separate that desired DNA from its original form i.e. completely free of other macromolecules. DNA is found in the cell membrane with other macromolecules, such as polysaccharides (RNA), polysaccharides as well as lipids, DNA needs to be separated from and purified. This requires enzymes such as cellsulase and lysozymes as well as proteases like ribonuclease and proteases. Other macromolecules can be removed using other treatments or enzymes. In the end, the presence of ethanol causes DNA to separate into fine threads. This is then spun out to create pure DNA.
2. Restriction Enzyme Digestion
Restriction enzymes function like molecular scissors which cut DNA at certain locations. These reactions are known as’restriction enzyme digestions’. They involve incubating the DNA purified with the specific restriction enzyme at the conditions that are optimal for the enzyme in question. The method ‘Agarose Gel Electrophoresis shows the progression of digestion using the restriction enzyme. This method involves separating the DNA using the agarose gel. When current is applied the DNA that is negatively charged moves through the electrodes to the positive, and is separated out according to size. This permits cutting out DNA fragments that have been digested. The DNA from the vector is processed with the same method.
3. Amplification Using PCR
Polymerase Chain Reaction, also known as PCR is a technique for creating multiple copies of DNA sequences with the help of DNA Polymerase, in vitro. It allows you to multiply one copy or couple of copies of DNA into millions to thousands of copies. PCR reactions are performed by ‘thermal cycles’ that use the following elements:
- Template – DNA to be amplified
- Primers – small, chemically synthesized oligonucleotides that are complementary to a region of the DNA.
- Enzyme – DNA polymerase
- Nucleotides – needed to extend the primers by the enzyme.
The DNA fragments that have been cut can be amplified with PCR before being ligated to cutting vector.
4. Ligation of DNA Molecules
The purified DNA as well as the interest vector are cut using this same enzyme. This results in the DNA fragment that has been cut and the cut vector which is now unlocked. The method of joining these two pieces with the enzyme ‘DNA Ligase”‘ is called ligation. The resultant DNA molecule will be an amalgamation made up of two DNA molecules: both the interest and vector. In the field of genetics, the intermixing of multiple DNA strands is referred to as the process of recombination. Therefore, this hybrid DNA molecule can also be called a recombinant DNA mole and is also called the recombinant DNA technique.
5. Insertion of Recombinant DNA Into Host
In this process the recombinant DNA is transferred into a host cell. It is usually bacteria cells. This is known as “Transformation”. Bacterial cells cannot readily accept DNA from other species. So, they undergo treatment to be ‘competent’ for accepting new DNA. The methods used could be thermoshock, CaIon Treatment, Electroporation, etc.
6. Isolation of Recombinant Cells
The transformation process creates the transformation process to create a mixed number of transformed and non-transformed host cells. The process of selection involves filtering transformed host cells to the extent that they are. To distinguish recombinant cells from non-recombinant cells the marker gene in the vector plasmid is used. For examples, PBR322 plasmid vector contains different marker gene (Ampicillin resistant gene and Tetracycline resistant gene. When pst1RE is utilized, it eliminates Ampicillin resistance gene in the plasmid in order for the recombinant cells to becomes susceptible to Ampicillin.
Application of Recombinant DNA technology
- Recombinant DNA is extensively used in medicine, biotechnology and research.
- The most widely-used application of DNA recombinant is in basic research, and the technology is crucial to the majority of current research in biomedical and biological sciences.
- Recombinant DNA can be used to determine, map the sequence of genes as well as to identify their functions.
- Recombinant proteins are used extensively in laboratory experiments as reagents as well as to make antibodies to study the synthesis of proteins in cells and in organisms.
A variety of other applications for Recombinant DNA can be found in food production, industry Human and veterinarian medicine, agriculture and bioengineering.
- Recombinant chymosins: Recombinant chymosinis found in rennet, chymosin can be described as an enzyme that is required for the production of cheese. This was the very first food additive that was genetically engineered and that was commercially used. Traditionally, processors sourced the chymosin ingredient from rennet which is a product derived from the stomach of the fourth calves that are fed milk. Scientists have engineered an unpathogenic kind of strain (K-12) from E. E. coli to allow large-scale lab production of this enzyme. The is a microbiologically produced recombinant enzyme that is similar in structure to the calf-derived enzyme, is less expensive and is manufactured in huge quantities.
- Recombinant human insulin: Recombinant human insulin has almost entirely substituted insulin from animals (e.g. cattle and pigs) to treat insulin-dependent diabetes. A range of different insulin preparations made from recombinant sources are widely used. Recombinant insulin is produced through the introduction of human insulin genes into E. coli, or yeast (Saccharomyces cerevisiae) which produces insulin that is suitable for human consumption.
- Human growth hormone recombinan: Human growth hormone recombinant (HGH, Somatotropin) is administered to patients whose pituitary glands produce insufficient amounts to allow the normal development and growth. Prior to the time that the recombinant HGH was made readily available HGH to treat ailments was obtained from the pituitary glands from cadavers. This unwise practice resulted in the development of CreutzfeldtJakob disease in a few patients. Recombinant HGH has eliminated this issue and is utilized to treat patients.
- Recombinant blood-clotting factor VIII: A protein used to clot blood. It is given to patients suffering from types of hemophilia bleeding disorder who are not able to produce factor VIII in sufficient quantities to ensure the normal process of blood coagulation. Prior to the creation of recombinant factor VIII the protein was derived by processing large amounts of blood donated by multiple people which carried a significant chance of transmission of blood-borne infections, such as HIV and hepatitis B. The entry in DrugBank.
- Recombinant hepatitis B vaccine: Hepatitis B infection is prevented by using the reconjugation hepatitis B vaccine. It is a type of hepatitis B surface antigen of the virus that is created in yeast cells.
- Diagnosis of infection with HIV: The diagnosis of an infection caused by HIV The three most commonly used methods to diagnose HIV disease has been created with the recombinant DNA. The test for antibodies (ELISA also known as western blot) utilizes an Recombinant HIV protein to determine any antibodies the body produces in response to HIV infection.
- Golden rice: A recombinant type of rice engineered to express enzymes involved in the biosynthesis of b-carotene.
- Herbicide-resistant plants: Commercial varieties of key agriculture crops have been developed which contain a recombinant genetic that confers resistance the herbicide called glyphosate (trade trade name Roundup) and makes it easier to manage the control of weeds through glyphosate application.
- Insect-resistant crops: Bacillus thuringeiensis is a bacteria which naturally produces an enzyme (Bt toxin) that has insecticidal properties. The bacterium has been added to crops to control insects as a method for years and is widely used in gardening and agriculture. Recently, plants were created that have a recombinant expression of the protein bacterial that can effectively control certain insects. Environmental concerns associated with the transgenic crops are not completely addressed.
Limitations of Recombinant DNA technology
- The destruction of native species within the habitat that genetically altered species are introduced into.
- Resilient plants could in theory give rise to resistant weeds that can be difficult to manage.
- Migration of DNA that is proprietary between different organisms.
- Recombinant organisms are contaminating the natural ecosystem.
- The recombinant organisms consist of clones, which are susceptible to disease in the exact same way. One single pest or disease can decimate the entire population in a short time.
- Superbugs are believed to be the cause of the superbug.
- Concerns about the ethical implications of humans trying try to be God and interfere with natural process of choosing. This is exacerbated due to the fear of what can be made with the help of technology and how it is going to affect our civilization.
- A system like this could cause people to have their genetic data stolen and used without their permission.
- Many are concerned about the security of altering foods and medications with recombinant DNA.
What is DNA Cloning?
A clone can be described as a collection of cells which originate from a parent. Clones are genetically identical to the cell it’s parent from which it reproduces.
DNA cloning takes place by inserting DNA fragments inside DNA molecules. The replicating molecule is the keeper of DNA vectors. A clone is an assortment of cells or individuals that are derived from one progenitor. Clones are genetically similar because each time a cell reproduces it creates the same daughter cells. Scientists have found a method to create multiple copies of one DNA fragment, a gene that could be used to produce identical duplicates of a DNA clone. The process of DNA cloning involves inserting DNA fragments inside a tiny DNA molecule. The molecule is made to reproduce inside living cells such as bacteria. The carrier of DNA vector is a tiny replicating molecules. Plasmids, yeast cells as well as viruses, are some of the main examples of rDNA technology’s vectors. Plasmids are DNA molecules that have circular shapes which bacteria introduce into human bodies. They’re not part of the cell’s genome. They carry genes that confer favorable characteristics on the host cell, for instance the ability to mat and drug resistance. They are easily controlled because they are tiny and carry additional DNA which has been integrated into their.
Importance of DNA Cloning:
- Agriculture is among the fields in which genetic cloning is utilized. Nitrogen fixation happens by cyanobacteria. The desired genes are able to boost the yield of crops and enhance the health of the soil. In the process of this technique, the use of fertilizers is decreased, and chemicals-free products are created.
- In the realm of medicine, gene cloning has become very important. Vitamins, hormones, and even medicines are all created by it.
- It is able to determine and recognize the presence of an individual gene that could be altered by a process of growth in a controlled atmosphere.
- It is used in the field of gene therapy, where damaged genes are replaced by a healthy gene. This method can be utilized to treat illnesses such as sickle cell and leukaemia.
Recombinant DNA Technology Examples
Recombinant DNA technology can be found in a variety of ways such as food products, vaccines as well as diagnostic tests, pharmaceutical products and genetically engineered plants.
Vaccines
The vaccines that contain viral proteins created from yeast or yeast-derived from genes recombined from viral DNA are believed to be more secure than those made using more conventional methods and also contain virus particles.
Other Pharmaceutical Products
As we mentioned previously in the past, insulin is a further example of the application of recombinant DNA technology. Prior to that, insulin was extracted from animals, mostly the pancreas in pigs and cows. However, using the technology of recombinant DNA to introduce the insulin gene from humans into yeast or bacteria can make it easier to create greater amounts.
Many other pharmaceutical products, such as antibacterial drugs or human proteins replacements, are made through similar methods.
Food Products
Many food items are made using recombinant DNA technology. A typical example is the chymosin enzyme. It is an enzyme utilized in the production of cheese. Typically, it’s present in rennet, which is made using the stomachs from calves however, the production of chymosin by genetic engineering is much simpler and quicker (and doesn’t necessitate the slaughter of animals that are young). Nowadays, the large portion of the cheese that is produced by the United States is made with genetically modified chymosin.
Diagnostic Testing
Recombinant DNA technology can also be employed in the diagnostic field. The genetic testing of a diverse spectrum of ailments, including muscular dystrophy and cystic fibrosis benefitted from the application the rDNA technology.
Crops
Recombinant DNA technology is employed to create herbicide and insect-resistant plants. The most popular herbicide-resistant crops are not affected by the use of glyphosate which is a commonly used herbicide. The production of these crops isn’t unproblematic, as many doubt the long-term safety of genetically engineered plants.
What is The Future of Genetic Manipulation?
Scientists are enthusiastic about the possibilities that genetic manipulators will be able to create. While the methods on the horizon differ, they all have in common the accuracy with which genomes can be altered.
CRISPR-Cas9
One example of this is CRISPR-Cas9. It is a protein that allows for the insertion and elimination of DNA a highly precise way. CRISPR is an abbreviation for “Clustered Regularly Interspersed Short Palindromic Repeats” and Cas9 can be a shorthand term for “CRISPR related proteins 9”. Since the beginning of the year scientists have been excited about the potential of its application. These processes are speedier and more precise. They are also cheaper than other methods.
Ethical Questions
While many of the technological advances enable more precise methods however, ethical questions are being asked. For instance, if we have the ability to do something, does it implies that we must take it on? Are there ethical concerns with more exact genetic testing, specifically with regard to human genetic disorders?
From the initial work of Paul Berg who organized the International Congress on Recombinant DNA Molecules in 1975, and to the current guidelines laid out through The National Institutes of Health (NIH) There are a variety of ethical issues were raised and addressed.
NIH Guidelines
The NIH guidelines state that they “detail precautionary practices and containment protocols for clinical and basic research involving synthetic or recombinant DNA molecules as well as the creation and usage of organisms as well as viruses that contain synthetic or recombinant DNA molecules.” The guidelines were created to provide researchers with appropriate conduct guidelines to conduct research in this field.
Bioethicists argue that science must constantly be ethically balanced so that progress is beneficial to humanity, instead of causing harm.
FAQ
What is recombinant dna technology?
Recombinant DNA Technology is genetic engineering where the gene from one organism is inserted into another. This process enables scientists to modify the characteristics of organisms to produce useful proteins for medical purposes, such as insulin production.
Recombinant DNA Technology is used for cloning organisms. Cloning is the process of producing genetically identical copies of an organism from a single parent. Most commonly, this occurs when two different parents produce offspring which contain genetic traits from both parents. The first successful cloning was performed on sheep in 1978 by Bruce Beutler at Stanford University.
Which is most analogous to the role of gfp in recombinant dna technology?
The role of GFP in recombinant DNA technology is to give researchers the ability to detect small quantities of certain proteins. By expressing them from a plasmid, they are able to use fluorescence microscopy techniques to visualize these proteins at high resolution. They also provide new opportunities for studying protein-protein interactions.
The GFP gene is responsible for making green fluorescent protein. This gene was first isolated from Aequorea victoria jellyfish about 35 years ago. Nowadays, many scientists use GFP to study how individual cells behave inside living organisms. For example, biologists use GFPs to tag specific proteins inside cells, then watch how they move around and interact with other molecules.
which of the following tools of recombinant dna technology is incorrectly paired with its use?
a. Reverse transcriptase-production of.cDNA from mRNA
b. DNA polymerase-used in polymerase chain reaction to amplify section of DNA
c. DNA ligase-enzyme that cuts DNA, creating the sticky ends of restriction fragments
d. Restriction enzyme-production of RFLPs
Restriction enzymes cut DNA creating sticky ends of restriction fragments. While DNA ligase joins these sticky ends to form recombinant DNA.
So, the correct option is ‘DNA ligase-enzyme that cuts DNA, creating the sticky ends of restriction fragments’.
Which of the following is an example of “recombinant DNA technology?”
introducing a human gene into a bacterial plasmid
11. Which of the following was first produced commercially using recombinant DNA technology?
A. Human growth hormone.
B. Interleukins.
C. Hepatitis B vaccine.
D. Human insulin.
D. Human insulin.
Recombinant DNA technology does not rely on which of the following enzymes?
A. restriction endonucleases
B. RNA methylase
C. DNA ligase
D. reverse transcriptase
B. RNA methylase
During recombinant dna technology which structure is used to cut the dna at a specific site?
The Recombinase Enzyme has a nuclease domain, a DNA binding domain, and a catalytic domain. The enzyme cuts one strand of double stranded DNA and inserts new strands.
Why are bacteria used in recombinant dna technology
Bacteria are used in recombinant technology due to various reasons. They contain extrachromosomal DNA called plasmid, which can replicate independently. They are easier to manipulate and replicate rapidly in a medium. Transformants can be easily screened, selected and transferred to the target cells.
Related Posts
- DNA Library – Types, Construction, Applications
- Emulsion PCR – Principle, Procedure, Advantages, Limitations, Uses
- TaqMan Probe – Definition, Principle, Applications
- Differences Between Sensitivity, Specificity, False positive, False negative
- Protein Synthesis (Translation)- Definition, Steps, Sites, Machinery