- The structure of hydrocarbon (HC) compounds consists of hydrogen and carbon.
- The environment is especially polluted by unintended leaks of petroleum products. Some HC compounds can be carcinogenic and neurotoxic to various organisms.
- Bioremediation is a potential method for the remediation of HC-contaminated sites since it is economical and can result in total mineralization.
- Utilizing the metabolic pathways of living creatures (mostly microbes) for the biodegradation of organic contaminants, resulting in their partial or total mineralization into carbon dioxide, water, and inorganic chemicals.
- Degrading organisms may utilise pollutant molecules as a source of energy and as precursors for the creation of their cellular components.
- Complex organic pollutants are transformed into simpler (perhaps less hazardous) forms that can then be utilised by other organisms.
- HC decomposition frequently necessitates the presence of oxygen, as the initial degradation is initiated by oxygenase enzymes; however, nitrate or sulphate may function as a terminal electron acceptor in following phases.
- Various businesses and daily activities rely on petroleum-based goods as their primary source of energy.
- During the transportation, production, exploration, refining, and storage of petroleum and its derivatives, leaks and accidental spills occur often.
- The annual volume of natural crude oil seepage was estimated to be 600,000 metric tonnes, with a margin of error of 200,000 metric tonnes.
- The release of HC into the marine environment has the potential to significantly contaminate the water and cause considerable harm to marine life.
- The buildup of these contaminants in the tissues of marine plants and animals may result in death or mutation.
- In addition, when these animals and plants become a part of the food chain, the biomagnification of their detrimental impacts may increase.
Contents
Types of Hydrocarbon
Crude petroleum HCs can be categorised as alkanes, cycloalkanes, aromatics, polycyclic aromatics, asphaltines, and resins.
Alkanes
- Among petroleum hydrocarbons, n-alkanes are the most biodegradable. As solvents, alkanes in the range of C5 to C10 are normally inhibitory to the majority of HC degraders at higher concentrations because they break lipid membranes.
- Alkanes in the range of C20 to C40, commonly known as waxes, are less biodegradable due to their limited solubility in water as hydrophobic solids.
- During decomposition, oxygenase enzymes target the terminal methyl group of alkanes and convert them to alcohol. The alcohol is oxidised further into aldehyde and finally into fatty acids.
- Aliphatic chain undergoes -oxidation in order to further utilise fatty acids. The more methyl branching there is, the less -oxidation there is.
Cycloalkanes
- Cycloalkanes and alicyclic hydrocarbons degrade more slowly than alkanes. Here, the biodegradability diminishes as the number of ring structures increases.
- Alkyl-substituted cycloalkanes degrade more readily than unsubstituted cycloalkanes.
- By the action of oxidases, cycloalkanes are degraded to cyclic alcohol, which is then dehydrogenated to ketone.
- Primary products of cycloalkane metabolism include cycloketones and cycloalkane-carboxylic acids.
Aromatic HCs
- Aromatic HCs molecules have a structure based on benzene. aromatic compounds are more stable than most other cyclic compounds due to the sharing of delocalized electrons by pi bonds.
- Comparatively, BTEX (benzene, toluene, ethylbenzene, xylene) chemicals are more mobile and miscible with water.
- Biodegradation of an aromatic molecule involves two key steps: (1) ring activation and (2) ring cleavage.
- Oxygenases are responsible for the incorporation of molecular oxygen into the aromatic ring, resulting in dihydroxylation of the aromatic nucleus, which is necessary for activation.
- Fungi and other eukaryotes include monooxygenases, which catalyse the incorporation of a single oxygen atom to generate an epoxide, which can subsequently undergo hydration to yield transdihydrodiols.
- Dioxygenases catalyse the incorporation of two oxygen atoms simultaneously to generate a dihydrodiol. It has been demonstrated that these dioxygenase reactions occur with benzene, halogenated benzenes, toluene, para-chlorotoluene, xylenes, biphenyls, napthelene, anthracene, etc.
- These dihydrodiols undergo further oxidation to produce catechols, which are precursors of ring cleavage.
- Catechol can be oxidised either via the ortho-cleavage pathway, which cleaves the bond between the carbon atoms of the two hydroxyl groups to produce muconic acid, or via the metacleavage pathway, which cleaves the bond between a carbon atom with a hydroxyl group and the adjacent carbon atom to produce 2-hydroxymuconic semi aldehyde.
- These chemicals are further degraded into organic acids, which microbes employ for cell formation and energy production.
Polycyclic aromatic HC
- Polycyclic aromatic hydrocarbons (PAH) and polynuclear aromatic hydrocarbons (PNA) are byproducts of high-temperature industrial processes such petroleum refining, coke manufacture, and wood preservation.
- This class of chemicals, which include two or more benzene rings, has sixteen contaminants of high concern. Some of these substances are thought to be cancer-causing.
- PAHs are less soluble and volatile as their molecular weight and number of ring structures grow, whereas their adsorption capacity rises.
- PAHs are destroyed by the same mechanism as monoaromatic chemicals. It is vital for the environment that PAHs are degraded by fungi, as some of the compounds have been linked as hazardous to higher life forms.
Asphaltines and resins
- Asphaltines and resins are high-molecular-weight compounds composed of nitrogen, sulphur, and oxygen.
- These substances are resistant to biodegradation because they are extremely insoluble and include functional units that are protected from microbial attack by an extensive aromatic ring structure.
Effects of Hydrocarbon
The ecological and/or economic effects of direct or indirect exposure of live creatures to hydrocarbon molecules can vary.
- Direct exposure to oil spill: When an oil spill happens, a range of negative consequences may arise, particularly for those who live and work in affected areas. Through breathing, they may come into touch with gaseous oil compounds or oil compounds adsorbed on particulate matter (distributed in the air). Another exposure route may involve activities conducted on contaminated ground (soil) or through direct skin absorption upon contact with spilled contaminated material.
- Indirect exposure: This occurs when consuming contaminated food or water, which is especially pertinent in the event of consuming fish from an oil spill-polluted region. As some oil components can collect in living beings, if a fish lives in a polluted environment, it will continue to accumulate in its body (without excretion) some oil components whose concentrations may be several orders of magnitude higher than those of the surrounding waters. By consuming such filthy fish meat, humans may be exposed to higher concentrations of oil components than in the surrounding environment or when compared to consuming polluted water or bathing in polluted water.
- Economic impact: Numerous businesses, such as fishing, tourism, oil industries, etc., have been harmed by oil spills. The estimated economic impact of the British Petroleum Gulf of Mexico oil disaster was a massive loss of 17,000 jobs by the end of the year. According to the National Wildlife Federation, more than 150 threatened or endangered sea turtles have already perished. In addition, 316 seabirds, primarily brown pelicans and northern gannets, have been discovered dead along the shore of the Gulf of Mexico as a result of the oil spill.
Principles of Bioremediation
- Crude oil is a complicated mixture of various hydrocarbons, and each type of oil has a particular chemical composition. There are a variety of microbes and plants that can be used to treat crude oil.
- The process of bioremediation can occur naturally or with the assistance of bacteria and fertilisers. First, biosurfactants and bio emulsifiers in the soil recognise the oil and its contents; then, microorganisms attach and utilise the hydrocarbon present in the petroleum as a source of energy.
- Due to their low solubility and adsorption, high molecular weight hydrocarbons are inaccessible to microorganisms.
- Biosurfactants enhance the solubility and removal of these pollutants, hence accelerating the biodegradation of oil.
- The volatility and susceptibility to biodegradation of oil components varied considerably, with certain chemicals being readily biodegradable and others being resistant to microbial breakdown.
- Various components of petroleum undergo simultaneous biodegradation, with distinct microbial species attacking different molecules at varying speeds.
- Enzymes produced by microbes in the presence of a carbon source are responsible for the biodegradation of petroleum hydrocarbons, but the absence of the proper enzyme inhibits the hydrocarbon degradation process.
Oil Spill Cleaning Methods
To safeguard the flora and wildlife from further harm and extinction, many strategies, including physical, chemical, and biological treatments, are utilised to clean oil-contaminated places. However, cleaning up contaminated surroundings is frequently challenging due to the influence of a number of factors. The physical or chemical method could be the first option immediately after oil spills, despite the disadvantages of both techniques, such as the need for numerous auxiliary materials, the inability to completely eliminate the leaking oil, and the additional environmental damage caused by the chemicals used in treatments. Due to the drawbacks of physical and chemical treatments, biodegradation and bioremediation have emerged as viable alternatives for eliminating oil from contaminated settings.
Biodegradation
- Biodegradation of hydrocarbons is a crucial technique in which microorganisms are used to enhance the natural cleansing processes of hydrocarbon pollutants.
- However, the biodegradation of hydrocarbons is subject to a number of factors and a series of vital and frequently unique interactions between microorganisms and other factors, which primarily occur on the surface of contaminated water, as well as in the sediments, beaches, and water column of the marine ecosystem.
- This process ultimately relies on bacteria consuming hydrocarbons to produce food, energy, and carbon dioxide. Biodegradation begins immediately following an oil spill in an aquatic setting.
- Microbes naturally breakdown oil in water, and due to the availability of food, they thrive in a contaminated environment.
- This process continues with the presence of biodegradable hydrocarbons in the contaminated environment, culminating in the first month of the oil spill and diminishing over time as the environment’s nutrients are depleted.
- Nutrients (N, P, and K) play a crucial role in the growth of robust microbial communities, which accelerates the pace of hydrocarbon breakdown along the contaminated shoreline.
- However, effective biodegradation of petroleum hydrocarbons by microorganisms is dependent on certain physicochemical factors, such as temperature, pH, dissolved oxygen in water, and nutrients, which regulate the growth of the microorganisms, as well as some biological factors, such as an enzyme’s activity, which control the potency of biodegradation.
- In addition to these, numerous additional variables affect biodegradation rates, such as the chemical composition and physical state of the oil, the kind and quantity of oil spilt, the characteristics of the damaged habitat, etc.
Bioremediation
- Bioremediation is one of the alternative strategies for the treatment of pollutants. It is an innovative technique that uses microorganisms to convert hazardous pollutants into innocuous chemicals such as H2O, CO2, CH4, and biomass without affecting the ecology of the damaged area.
- There are numerous species of microorganisms with the ability to bioremediate hydrocarbon contaminants. Included among these are yeast, fungi, algae, and bacteria.
- Bioremediation can be accelerated by supplementing oil-contaminated habitats with components such as nitrogen.
- Even if oil-polluted marine areas are cleansed, some substances may persist for decades on beaches and in surroundings, posing a harm to the creatures inhabiting these ecosystems.
- The bioremediation process can be divided into two forms, in situ bioremediation and ex situ bioremediation, based on the application technique.
- In situ bioremediation techniques are the most attractive alternative due to their low cost and the fact that they eliminate the need for drilling and transporting toxins.
- Due to the difficulty of extracting toxins from water or soil, ex-situ bioremediation, which is the removal of the contaminant’s physical material for treating contaminated environments, is both expensive and challenging. This method entails drilling and the removal of polluted soil and water, as well as composting, land farming, bioreactors, and biopiles.
- In addition, bioremediation procedures can be divided into two primary categories: I Biostimulation and ii) Bioaugmentation.
a. Biostimulation
- Biostimulation is the process of modifying the environment to stimulate the growth of existing bacteria and the biodegradation of pollutants to be treated in the transformed environment.
- This process is implemented by adding nutrients or substances, such as carbon, nitrogen, and phosphorus, to the environment to be treated, as well as by ensuring appropriate environmental conditions, such as moisture and oxygen content, to promote microbial growth and the natural biodiversity of microorganisms.
- The quantities of nitrogen, phosphorus, and molecular oxygen in seawater are significant examples of abiotic factors.
- Therefore, Nitrogen and Phosphate are utilised to decrease the limit of these nutrients, which promotes the growth of bacteria that degrade petroleum hydrocarbons.
- Due to their high water solubility, nitrogen and phosphate-based fertilisers such as nitrates, ammonium phosphate, urea, and phosphate can be employed in such situations.
- In addition, uric acid, which is utilised as a nitrogen source by numerous bacterial species, including Alcanivorax strains, can serve as a potent biological catalyst for the bioremediation of oil spills.
b. Bioaugmentation
- Bioaugmentation is the employment of specific strains of microbial organisms, which may be isolated or genetically manipulated to boost their capacity for pollution control, such as cleaning up oil spills in polluted marine habitats.
- This technique relies on the ability of bacteria to digest contaminants and convert them into chemicals that are less poisonous and less hazardous.
- Biodegradation occurs naturally in contaminated settings by native microorganisms, which typically have a limited capacity to digest these substances due to the compounds’ complicated structure and harsh environmental conditions.
- Therefore, bioaugmentation is necessary under these conditions so that these complicated compounds can be thoroughly digested by specialist microorganisms.
- Bioaugmentation, also known as the planting of microorganisms in contaminated locations, is employed in oil-polluted marine ecosystems to encourage the breakdown of spilled oil. This results in the proliferation of bacteria that use hydrocarbons as their sole source of energy and carbon.
- These microorganisms are referred to as hydrocarbonoclastic microorganisms, and they consist primarily of bacteria, including numerous types including Cycloclasticus, Thalassolituus, Alcanivorax, Oleispira, and Oleiphilus.
- Cycloclasticus strains favour aromatic hydrocarbons, such as anthracene, phenanthrene, and naphthalene, for growth, whereas Oleispira sp. and Oleiphilus prefer aliphatic hydrocarbons, alkanoates, and alkanols.
- Similarly, Alcanivorax sp. strains can only grow on branched alkanes and n-alkanes.
What is Aerobic and Anaerobic Bioremediation?
- In the absence of oxygen (anaerobic), the rate of bioremediation of hydrocarbon pollutants is slower than when oxygen is present (aerobic).
- Most species of bacteria, algae, and fungi have the capability to breakdown under aerobic conditions, making aerobic bioremediation of hydrocarbons faster and simpler.
- Adding oxygen to contaminated habitats multiplies the rate of biodegradation by several orders of magnitude compared to the rate at which biodegradation occurs naturally in these situations.
- In aerobic conditions, microorganisms often degrade hydrocarbon pollutants by attaching an unsaturated ring of polycyclic aromatic hydrocarbons or a hydroxyl group to the alkanes, thereby generating alcohol.
- These chemicals are therefore readily soluble in water with oxygen. In addition, aerobic microorganisms are more capable of degrading a wide variety of hydrocarbons than anaerobic microbes, leading in efficient bioremediation of hydrocarbon contaminants under aerobic circumstances.
- Despite the sluggish rates of anaerobic bioremediation, this is essential for the complete breakdown of certain hydrocarbons in the absence of oxygen, such as the 90-day degradation of polycyclic aromatic hydrocarbons and the 120-week degradation of benzene.
Biodegradation And Bioremediation Of Hydrocarbons (oil spills) By Microorganisms
1. Biodegradation by Bacteria
- The decomposition of oil occurs in a succession of distinct and consecutive stages. Bacteria are the first group of microorganisms to combat oil spills by producing medium compounds, which are subsequently utilised by a variety of other microbes.
- More than 200 species and 100 genera of microorganisms breakdown petroleum hydrocarbons in marine environments; the majority are bacteria.
- Oil-degrading bacteria include Oleiphilus, Cycloclasticus, Alcanivorax, Neptunomonas, Marinobacter, etc. These bacteria are responsible for the breakdown of alkanes and aromatic hydrocarbons.
- Acinetobacter, Achromobacter, Actinomycetes, Arthrobacter, Alcaligenes, Nocardia, Bacillus, Flavobacterium, Corynebacterium sp, and Pseudomonas sp are the other probable bacterial families capable of degrading hydrocarbons in seawater and soil.
- Similarly, Rhodococcus, Corynebacterium, Mycobacterium, Pseudomonas sp., Brevibacterium, Arthrobacter, and Nocardia are genera of bacteria that decompose gaseous hydrocarbons, particularly propane and/or butane.
- Bacillus sp., Pseudomonas sp., Acinetobacter calcoaceticus, Micrococcus sp., Nocardia erythropolis, Candida Antarctica, Ochrobactrum sp., Serratia marcescens, Acinetobacter sp., Alcaligenes odorans, Candida tropicalis, and Arthrobacter sp. were utilised for alkane degradation.
- Pseudomonas sp., Brevibacillus sp., B. stearothermophilus, Bacillus sp., Corynebacterium sp., Vibrio sp., Ochrobactrum sp., and Achromobacter sp. are capable of degrading mono-aromatic hydrocarbons.
- Burkholderia cepacia, Alcaligenes odorans, Achromobacter sp., Mycobacterium sp., Sphingomonas paucimobilis, Mycobacterium flavescens, Pseudomonas sp., Arthrobacter sp., Bacillus sp., Rhodococcus sp., Xanthomonas sp., Alcaligene
- Resin breakdown was facilitated by Vibrionaceae, Pseudomonas sp., Moraxella sp., and Enterobacteriaceae.
Examples of bacteria that degrade petroleum hydrocarbons in marine environments.
- Aeromonas hydrophila
- Ochrobactrum anthropi
- Acinetobacter lwoffii
- Stenotrophomonas maltophilia
- Erythrobacter citreus
- Pseudomonas aeruginosa
- Pseudomonas xanthomarina
- Rhodococcus corynebacterioides
- Neisseria elongata
- Vibrio fischeri
- Bacillus megaterium
- Enterobacter cloacae, Brevibacillus parabrevis B-1
- Bacillus pumilus
- Chromobacterium violaceum
- Bacillus cereus
- Klebsiella pneumoniae
- Achromobacter, Acinetobacter, Alcaligenes, Actinomycetes, Achromobacter, Acinetobacter, Alcaligenes, Actinomycetes, Archrobacter, Cycloclasticus, Coryneforms, Chromobacterium, Flavobacterium, Micrococcus, Microbacterium, Mycobacterium, Nocardia, Pseudomonas, Sarcina, Serratia, Streptomyces, Vibrio, Xanthomonas
2. Biodegradation by Algae
- Phytoremediation, a type of biological treatment that uses micro-algae or macro-algae to remove solid, liquid, or gaseous pollutants from polluted soil, wastewater, and air, is an essential step in the treatment of petroleum hydrocarbons.
- Nevertheless, the utilisation of micro-algae in the bioremediation of petroleum hydrocarbons remains a significant topic of investigation.
- Some algae, including green algae, red algae, and brown algae, have the ability to breakdown hydrocarbons into less hazardous molecules, showing their capacity to handle crude oil contamination.
- For instance, the algae Prototheca zopfi and Chlorella vulgaris are capable of hydrocarbon biodegradation, resulting in a decrease in alkanes, iso-alkanes, and aromatic hydrocarbons in the contaminated environment.
- In addition, the dry weight of Chlorella vulgaris increased as the concentration of pollutants increased, demonstrating that crude oil promotes the growth of algae species.
Examples of Algae that degrade petroleum hydrocarbons in marine environments.
- Agmenellum, Amphora, Anabaena,
- Aphanocapsa, Chlorella, Chlamydomonas,
- Coccochlorise, Cylindrotheea, Dunaliella,
- Microcoleus, Nostoc, Oscillatoria, Petalonema,
- Porphyridium.
- Prototheca zopfii
3. Biodegradation by Fungi
- In polluted aquatic habitats, fungi play a crucial role in the biodegradation of petroleum hydrocarbons.
- In addition, sand contaminated with spilled hydrocarbons is one of these fungi’s favoured habitats for extracting carbon from the hydrocarbons.
- Fungi are capable of degrading total petroleum hydrocarbons alongside a broader array of other microorganisms, such as bacteria.
Examples of Fungi that degrade petroleum hydrocarbons in marine environments.
- Aureobasidium, Candida
- Rhodotorula
- Acremonium, Aspergillus, Cladosporium, Mortierella, Saccharomyces, Trichoderma, Verticillium
- Fusarium solani
- Aspergillus fumigatus
- Aspergillus niger
- Aspergillus versicolor
- Cochliobolus lutanus
- Aspergillus saprophyticus
4. Yeast for Biodegradation of Petro-Hydrocarbons
- Yeast may utilise aliphatic hydrocarbons in addition to a number of petroleum products.
- Yeasts that utilise aliphatic hydrocarbons include Rhodotorula aurantiaca, C. tropicalis, Candida lipolytica, Aureobasidium, Trichosporon, Rhodotorula aurantiaca, and C. ernobii.
- Melanogenum and Aureobasidium pullulans are categorised as lipolytic yeasts since they are capable of degrading diesel oil. By generating laccase, they were effective at degrading anthracene in rats by 37.3%, naphthalene in rats by 24.4%, benzo(a)pyrene in rats by 45.95%, and pyrene in rats by 27.0%.
- In addition, yeast strain (AEH) degrades naphthalene at 5.36 mg L1 in 2 days, chrysene at 1.54 mg L1 in 10 days, and phenanthrene at 5.04 mg L1 in 10 days effectively. AEH decomposed a mixture of phenanthrene and naphthalene containing 4.20 and 3.79 mg L1, respectively, in 10 days.
- In 10 days, this strain can also digest benzo(a)pyrene and chrysene at concentrations of 1.91 and 3.37 mg L1, respectively.
- Further research revealed that the co-metabolic biodegradation of benzo (a) pyrene and naphthalene in the environment can be readily utilised as the best carbon source.
Examples of Yeast that degrade petroleum hydrocarbons in marine environments.
- Aureobasidium, Pichia, Candida maltosa, Candida tropicalis, Candida apicola, Candida, Debaryomyces, Monilia, Rhodotorula, Toruplopsis
- Exophiala xenobiotics
- Candida tropicalis
- Candida lipolytica
- Candida maltose
- Candida tropicalis RETL-Cr1
- Pichia ohmen YH-41
- Trichosporon asahii
5. Protozoa for the Biodegradation of Petroleum Hydrocarbons
- Compared to bacteria, algae, and fungi, protozoa have been found as a detrimental agent of biodegradation.
- On the other hand, its communities demonstrated a considerable decrease in the number of bacteria capable of eliminating hydrocarbons.
- This suggests that its presence in the ecosystem is detrimental to biodegradation. However, the bacteria feed on protozoa that feed on the breakdown of organic contaminants.
- Therefore, the interaction between protozoa and bacteria will undoubtedly influence the biodegradation of these bacteria.
- Infusoria of protozoa accelerates the decomposition of diverse compounds in the ecosystem, including PAHs. For instance, the value of naphthalene degradation has quadrupled since its inception.
- Chen et al. (2007) provided some probable assumptions on protozoa accelerating organic pollutant degradation pathways. Essentially, they include the following:
- Enhanced nutrient circulation through mineralization of the nutrient.
- Activation of bacteria that regulates the number of obsolete cells through feeding or production of efficient matter.
- The feeding options reduce competition for food and surface area. Consider it advantageous for the proliferation of microorganisms involved in biodegradation.
- Physically occurring diseases increase both the oxygen concentration and surface area of substances subjected to biodegradation.
- Direct biodegradation results in the secretion of an enzyme integral to the biodegradation process.
- Co-metabolic biodegradation is the best source of energy and carbon for bacteria.
Aerobic degradation Mechanism
- Hydrocarbons breakdown easily in aerobic circumstances. Bacteria, fungi, and algae are all capable of hydrocarbon breakdown in an aerobic environment.
- Alkenes (hydrocarbons with double bonds) and short-chain alkanes (hydrocarbons with just single bonds) are the most quickly degraded hydrocarbons, followed by branched alkanes (alkanes with side chains) and aromatics (hydrocarbons in a stable ring structure).
- However, degradation rates vary according to environmental factors and decrease as the complexity of hydrocarbons rises.
- Hydrocarbon composition depends on the source of the oil and the age of the spill, therefore reported degradation rates vary significantly. For instance, compound degradation ranges from 5% to 30% in 28 days, whereas nitrogen addition causes up to 100% degradation.
- According to reports, degradation rates by fungal species range from 30% to 100% in less than 28 days.
- As noted previously, oxygen transport is the key rate-limiting component in aerobic biodegradation.
- Oxygen availability is based on oxygen’s ability to travel or diffuse across the site environment as well as microorganisms’ uptake rate.
- The addition of oxygen can boost decomposition rates by several orders of magnitude compared to their natural values.
Aliphatic hydrocarbons
- Alkanes with less than 14 carbon atoms are prone to volatilization, but alkanes with more carbon atoms are less volatile.
- With the exception of cyclic alkanes (alkanes with a ring structure), alkanes and alkenes are the most readily degraded hydrocarbons, with recorded degradation of alkanes containing up to 44 carbons.
- With the addition of molecular oxygen, alkanes and alkenes are destroyed. The rate-limiting factors include oxygen availability and the beginning step of degradation.
- In the earliest stages of aerobic alkane degradation, oxygenases add molecular oxygen to hydrocarbon molecules, generating alcohols, which are then oxidised to fatty acids, which are then metabolised to acetyl-CoA, and lastly to CO2 and H2O.
- Numerous mono- and di-oxygenases and other oxidases have a broad substrate range and readily act on various hydrocarbons.
Aromatic Hydrocarbons
- Due to their greater toxicity, aromatic hydrocarbons are typically more difficult to decompose than shorter alkanes and alkenes. However, they are rapidly digested aerobically by a wide variety of bacteria and fungi.
- Due to an increase in hydrophobicity and sorption capacity, the degradability decreases as the number of rings and molecular size rise.
- The principal degradation rate of benzene, toluene, ethylbenzene, and xylene (BTEX compounds) is between 0.05 and 0.2 day-1 on average.
- To decompose 1 mg/L of BTEX, 3.1 mg/L of dissolved oxygen (DO) is required. When DO levels fall below 2 mg/L, biodegradation slackens.
- The general mechanism for aromatic compound breakdown begins with the addition of oxygen by mono- and di-oxygenases. Benzyl alcohol, phenol or catechol, protocatechuate, and gentisate are some of the intermediate chemicals produced.
- Also through a number of oxygenases, these intermediates undergo ring cleavage, resulting in carboxylic acids.
- The degradation then proceeds to acetyl-CoA and succinyl-CoA, which enter the central metabolic pathway.
- Degradation by fungi is mediated by non-specific extracellular oxidising enzymes that generate radical intermediates, despite the fact that many of the processes resemble those of bacteria.
Anaerobic Degradation Mechanism
- Due to less favourable reaction energies with alternative electron acceptors, hydrocarbon degradation under anaerobic circumstances is frequently slower compared to aerobic degradation.
- Despite this restriction, it is known that both facultative and obligate anaerobic bacteria and archaea may breakdown hydrocarbons in the absence of oxygen.
- Due to the quick consumption of oxygen by these microorganisms at hydrocarbon-impacted locations, anaerobic processes have a considerable impact on the fate of hydrocarbons in the environment.
- Initial rate-limiting steps in anoxic hydrocarbon breakdown entail the addition of an oxidised functional group to activate the molecule. Anaerobic hydrocarbon degraders have doubling times ranging from days to months.
- In the absence of oxygen, many distinct forms of hydrocarbons degrade completely despite moderate growth rates. For instance, it has been claimed that polycyclic aromatic hydrocarbons (compounds with numerous aromatic rings) degrade within 90 days, but benzene degrades over a period of 120 weeks.
- According to reports, under methanogenic conditions linear alkanes breakdown in less than 200 days.
- In respiration, anaerobic microorganisms employ electron acceptors other than oxygen, such as nitrate, sulphate, carbon dioxide, oxidised metals, and even certain organic molecules.
- At a contaminated site, bacteria often use electron acceptors in the order of decreasing reduction potential: oxygen, nitrate, ferric iron, sulphate, and hydrogen.
- In a few instances, it has been demonstrated that specific species of denitrifying or sulfate-reducing bacteria entirely metabolise some hydrocarbons to CO2 and water.
- However, anaerobic degradation of hydrocarbons happens more frequently via syntrophy, in which the breakdown of a substrate by one microbe is dependent on the activity of another bacterium responsible for maintaining low concentrations of intermediate products such as formate and H2.
- Low product concentrations promote otherwise unfavourable thermodynamic processes. Syntrophy is more prevalent in anaerobic environments because the utilisation of oxygen as a terminal electron acceptor is energetically advantageous.
- Since methanogens (archaea that make methane) can only metabolise simple substrates such as acetate and hydrogen, total breakdown to methane and carbon dioxide requires the utilisation of syntrophic activities.
- Multiple syntrophic interactions may exist in any ecosystem, depending on the availability of substrates and environmental conditions.
- In methanogenic conditions, where all other electron acceptors are depleted, primary degraders like as Peptococcaceae and Clostridium convert hydrocarbons into intermediates such as H2 and acetate that are eaten by methanogens.
- Anaerobic microorganisms use a variety of ways to activate hydrocarbons without oxygen (O2). Each technique is explained individually and is applicable to both aliphatic and aromatic molecules.
- The main approach is to incorporate a higher oxidised group into the molecule in order to make it more reactive to subsequent transformation to more common intermediates (usually fatty acids and other carboxylic acids) that can enter major metabolic pathways.
- The vast majority of aromatic molecules are activated and directed toward the primary anaerobic intermediate, benzoate, or, more precisely, its coenzyme A (CoA) thioester derivative, benzoyl-CoA.
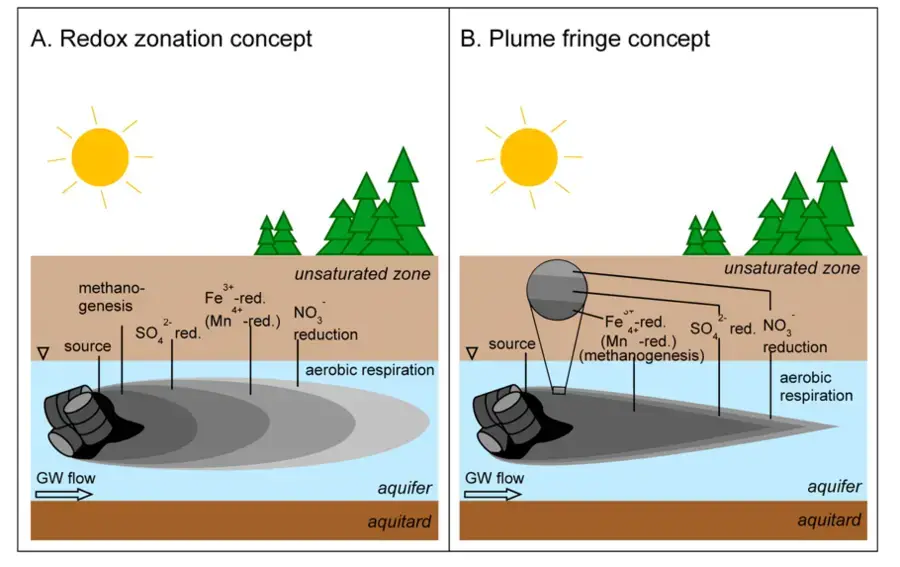
Strategies For Activating Hydrocarbons Without Oxygen
1. Fumarate Addition
- Bacteria use fumarate addition to activate alkanes with 3 to 20 carbons and alkyl-substituted aromatics such as toluene, xylenes, and methylnaphthalene.
- Common cellular metabolite containing two carboxylic acid groups and a double bond. Over the double bond of fumarate, the terminal or subterminal methyl group of alkanes or alkyl benzenes is added.
- This process is performed by alkylsuccinate synthase for alkanes and resulting in 2-(1-methylalkyl)succinates or 2-alkylsuccinates as intermediates.
- Carbon rearrangement, decarboxylation, and -oxidation are other pathways for the degradation of these molecules.
- Toluene, xylene, and 2-methylnaphthalene are aromatic hydrocarbons that breakdown upon fumarate addition.
- This reaction is facilitated by benzylsuccinate synthase.
- Through fumarate addition, numerous anaerobic bacteria, including pure cultures of sulfate- and nitrate-reducing bacteria, rapidly convert toluene to (R)-benzylsuccinate.
2. Oxygen-Independent Hydroxylation
- Oxygen-independent hydroxylation can denitrogenate ethylbenzene-metabolizing bacteria.
- In this process, ethylbenzene dehydrogenase adds a hydroxyl (-OH) group to the C1 carbon (carbon atom closest to the aromatic ring) on the side chain to generate S-1-phenylethanol, which is then oxidised to acetophenone and transformed into benzoyl-CoA and acetyl-CoA.
- The first step is catalysed by a hydroxylase containing a molybdenum cofactor. The oxidation of alkyl side chains on aromatic rings to their respective alcohols has also been attributed to flavocytochrome c hydrolases.
- On the subterminal carbon of alkanes, a similar mechanism of hydroxylation has been postulated to produce an alcohol functionality that is then more vulnerable to subsequent oxidation.
3. Carboxylation
- All processes documented to date only apply to hydrocarbons containing alkyl groups, not aromatic hydrocarbons such as benzene or naphthalene.
- In actuality, the anaerobic decomposition of benzene is far slower than that of toluene or xylenes, and it may not occur at all sites.
- Carboxylation is a proposed mechanism for naphthalene breakdown and anaerobic benzene degradation. CO2 is introduced directly to aliphatic and aromatic hydrocarbons in this procedure.
- The process is believed to be somewhat akin to the mechanism of anaerobic phenol (=hydroxybenzene) degradation, in which phenol is first activated by ATP to phosphophenol before being carboxylated into para-hydroxybenzoate.
- The mechanism by which carboxylases catalyse the addition of a carboxyl (-COOH) group to their substrate is still under investigation.
- Under iron- and nitrate-reducing circumstances, benzene is believed to be activated through carboxylation to benzoate.
- Due to the ring’s durability and concomitant high dissociation energy, benzene ring activation is challenging. Although there is currently no scientific evidence for benzene carboxylation, gene expression and proteome studies link the expression of the putative anaerobic benzene carboxylase (named AbcDA) to benzene breakdown.
- The carboxylation of naphthalene has been observed in crude cell extracts. It is believed that the active sites of aromatic ring carboxylases resemble those of the UbiD family of carboxylases engaged in aromatic metabolism in general.
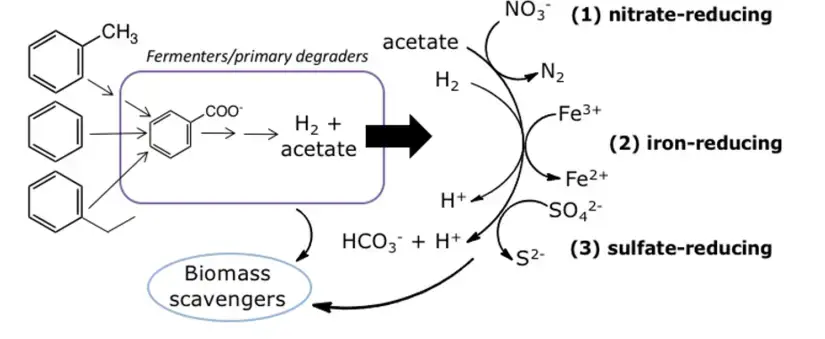
Factors Affecting the Hydrocarbon Degradation by Microorganisms
1. Effect of Temperature on Hydrocarbon Biodegradation
- Microorganisms that breakdown petroleum hydrocarbons are exceptionally effective at temperatures that regulate the enzyme-producing process.
- In addition, three species of enzyme-producing organisms have been identified based on their ideal temperature ranges: thermophiles (above 50 °C), mesophiles (15 °C–45 °C), and psychrophiles (below 20 °C).
- The majority of microorganisms are most effective at degrading petroleum at temperatures between 20 °C and 35 °C (mesotherm), where they produce the highest degradation rates.
- Initially, biodegradation is sluggish in cold water. Due to low temperatures, the viscosity of crude oil increases, the volatility of simple hydrocarbons decreases, and the solubility of these hydrocarbons increases. This renders the crude oil very poisonous and resistant to degradation by microorganisms. The saltwater temperature ranges from 2 and 35 degrees Celsius.
- The reduction in temperature from 25 °C to 5 °C reduces degradation levels by almost a factor of 10.
- The highest rates of hydrocarbon biodegradation occurred between 30 and 40 °C in soil habitats, between 20 and 30 °C in some freshwater environments, and between 15 and 20 °C in marine environments.
2. Effect of pH on Hydrocarbon Biodegradation
- The selection of pH as a regulating factor is determined by the types of microorganisms used in petroleum hydrocarbon breakdown.
- At pH 2, the local microorganisms, including certain bacteria, fungus, and yeast, are kept alive.
- Bacteria that grow rapidly at pH values greater than 9 and slowly at pH values of 6.5 are known as alkaliphiles. They existed in alkaline lakes with pH levels between 7.5 and 10.
- The pH of the growing medium is the most important factor in determining the efficiency of the BA2 strain of Sphingomonas paucimobilis. At pH 5.2, anthracene and phenanthrene biodegrade much more slowly. In the meantime, PAH biodegradation was found in a highly acidic, pH 2 soil contaminated with coal tar.
- Due to the high pH levels, the effectiveness of the bacteria decomposing naphthalene, such as Pseudomonas fluorescens (DSM6506) and Pseudomonas frederiksbergensis (DSM 13022), was drastically reduced.
3. Number, Activity, and Catabolism Evolution of the Microorganisms
- The major factors that influence the rate of petroleum hydrocarbon degradation by microbes are the accessibility of microbes to the targeted pollutants that they are capable of degrading, the number of microbes capable of degrading these pollutants, the active action of these microbes, and the molecular structure of the hydrocarbon pollutant.
- For successful biodegradation, the microbes’ catabolism must be enhanced, which can be accomplished by inducing the specified active enzymes, enhancing novel metabolism by modifying genes, and enriching the selective microorganisms with the capacity to transform the targeted hydrocarbon contaminants.
4. Effect of Microbial Consortium on Hydrocarbon Biodegradation
- A consortium for hydrocarbon biodegradation consists of two or more groups of symbiotic microorganisms.
- The primary component of petroleum is hydrocarbons with varying aliphatic and aromatic carbon chain lengths.
- In general, microbe-produced enzymes attack the molecules of hydrocarbons. It is common knowledge that enzymes are protein complexes responsible for converting petrohydrocarbons into simpler compounds. Some of these microbes are capable of producing enzymes that are able to break down virtually all hydrocarbon kinds and sizes.
- In the meantime, other bacteria have produced enzymes that break and attack specific sizes or types of hydrocarbon molecules. As soon as petroleum hydrocarbons are cracked, the remaining hydrocarbon molecules require more enzymes to be broken.
- Biodegradation cannot be completed in the absence of enzymes that are specific for cracking the remaining hydrocarbon molecules.
- Thus, the operation will be halted until a new element is added to the combination. These intricate sequences of biodegradation-related events constitute a metabolic pathway.
- There is not a single microbe species capable of biodegrading so many different petroleum hydrocarbon molecules.
- Therefore, multiple diverse enzymes and metabolic pathways are required for the biodegradation of such a large number of hydrocarbon species, including crude oil constituents.
- Due to the vast availability of biodegradable hydrocarbons in the environment, specific microorganisms will proliferate rapidly in the event of a spill of crude oil. By reducing the availability of nutrients and oxygen inside these communities, these microorganisms with rapid development may inhibit the activity of other species.
- While such readily degradable hydrocarbons have been consumed, the mortality of these bacteria is caused by a shortage in the microbial enzyme essential for decomposing the leftover hydrocarbons.
- On the other hand, other bacteria that are capable of degrading the leftover hydrocarbon are eager to develop rapidly and thrive inside their communities. This cycle continues, with microbial types thriving, regressing, and multiplying due to the availability of degradation, and then dying and so on.
- Microorganisms that reside in crude oil and degrade hydrocarbon molecules more readily are frequently identified near surface water and soil.
- Hydrocarbons close to water and soil surfaces, which are the primary sources of oxygen, moisture, and food, are responsible for the development of these microorganisms at these locations.
- The majority of microorganisms decomposing hydrocarbon compounds required oxygen for development (aerobic). Some microorganisms, however, are anaerobic, meaning they do not require oxygen.
5. Bioavailability of Hydrocarbons
- Physical qualities, water-repellent properties, adsorption on soil particles, evaporation from the medium, and the ability of hydrocarbons to dissolve have been used to determine the availability of petroleum hydrocarbons for biodegradation.
- All of these variables have a substantial impact on the rate of their breakdown by microbes. Even when all parameters affecting hydrocarbon degradation by microorganisms have been maintained at optimal levels, it has been demonstrated that a component of non-degradable hydrocarbons remains in the field.
- After hydrocarbons enter the soil environment as an organic pollution, they may be removed by microbial decomposition, vaporisation, or leaching. They may also be collected by soil micro- and macrobiota, adsorbed by soil particles, or incorporated into the soil’s organic matter or minerals.
- Various physicochemical processes, including adsorption, diffusion, and dissolution, are utilised to calculate the degradation values of hydrocarbons by microorganisms.
- The availability of microorganisms is determined by the total transit of a particular contaminant. Biological availability refers to the proportion of organic compounds in soil that are utilised or transformed by microbes. The biological availability of chemical substances is expressed as a percentage of mass transit and living organism substance actions in the soil.
- Major soil contaminants have a two-phase characteristic. In the initial phase of petroleum hydrocarbon breakdown by microorganisms, the rates of degradation have increased. In the second phase of hydrocarbon biodegradation, the hydrocarbon degradation rate has slowed due to adsorption limitations. It is important to note that the processes of weathering have a significant impact on the breakdown of hydrocarbons that polluted the soil over time (i.e., reduction of the contaminant availability to the microbes).
- The physical, chemical, and biological processes of weathering have significantly altered the surviving petroleum hydrocarbon types in the soil environment.
6. Salinity: Effect on the Biodegradation of Hydrocarbons
- It is well known that microorganisms are compatible with the extensive salinity of the world’s oceans. A little amount of evidence suggests that microorganisms are influenced by a saline environment other than one with hyperactive salinity, such as the saline water of a petroleum reservoir.
- However, estuaries may present a variety of conditions due to the fact that their salinity levels vary according to distinct scales when compared to seas and oceans.
- When adding microorganisms to the marine environment for hydrocarbon biodegradation, it should be determined whether or not these microorganisms are compatible with the salt level of this aquatic habitat.
7. Effect of Petroleum Surface Area
- Most hydrocarbon breakdown by microorganisms occurs at or near the interface between air and water in marine environments and between air and soil in soil environments.
- Consequently, the biodegradation rate will be influenced and controlled by the value of the petroleum’s interface surface area. Therefore, as the area exposed to air grows, petroleum degrades more rapidly.
- The oil surface area influences the transformation of the leftover petroleum hydrocarbons in the marine environment. Oil puddles, thick rafts, blankets, and other petroleum compounds with a high concentration and a small surface area have a low biodegradation rate.
- This is due to the fact that the availability of active sites broken by microbes decreased. Here, petroleum acts as a blanket, blocking the microbe from regenerating oxygen and nutrients.
- Thus, when concentricity increases, the substances that are easier to disintegrate are broken down by bacteria, leaving the more resistant substances towards the back.
- The latter combine to form more recalcitrant solids, such as star circles with finite moisture content and limited surface area. However, the hydrocarbon concentration ranged from 1 to 100 g/ml of water and 1 to 100 g/g of soil (as dried mass) and is not deemed toxic to the prevalent bacteria and fungi.
8. Characteristics of the Contaminants
- Typically, petroleum hydrocarbons consist of a mixture of complex compounds. Every hydrocarbon compound cannot be decomposed with a same ratio.
- The rate of degradation of petroleum hydrocarbon compounds by microorganisms is mostly determined by the chemical structure and concentration of these molecules.
- The compounds of petroleum hydrocarbons were categorised as saturated aromatics, resins, and asphaltenes.
- Among the many petroleum products with an average chain length between C10 and C25, the n-alkanes are the most preferred by microbes and the most readily biodegradable.
- In contrast, petroleum compounds with a shorter chain are both more toxic and less biodegradable.
- In contrast, alkanes with long chains ranging from C25 to C40 are water-repellent solids in water. As a result, they are difficult to breakdown due to the compounds’ inability to dissolve in water and their lack of biological availability.
- Similarly, alkanes having branched and cyclic chains are biodegraded far more slowly than symmetric n-alkanes.
- In contrast, highly condensed aromatics, tars, cycloparaffinic structures, asphaltic materials, and bitumen have a higher boiling point and provide the greatest resistance to microbial breakdown. Consequently, it was claimed that these residues from the biodegradation of petroleum are comparable to humic materials.
- It is crucial to note that the pace at which microorganisms degrade petroleum hydrocarbon compounds depends on the solubility of these molecules in the hosting medium.
- Thus, the biodegradation rate of highly soluble petroleum hydrocarbon compounds in an aqueous media is nearly proportional to their concentration.
- The opposite is true for hydrocarbons with less aqueousness. In general, a petroleum concentration of greater than 5% in the medium inhibits microbial activity.
- In addition, the carbon: nitrogen: phosphorus ratio and oxygen consumption limitations can prevent the oil’s concentration from increasing.
9. Availability of Dissolved Oxygen
- For respiration, the availability of dissolved oxygen is of paramount importance to microorganisms.
- Dissolved molecular oxygen is consumed extensively across all biodegradation pathways. Typically, 3 to 4 ml of dissolved oxygen are required to convert 1 ml of petroleum hydrocarbon compounds into CO2 and water.
- Due to the increasing concentration of hydrocarbons in petroleum and the extremely low concentration of dissolved oxygen, this is a relatively difficult target to achieve.
- Surface water, including seas, oceans, lakes, and ports, has virtually infinite oxygen due to the constant interaction between air and water as well as wind and wave activity.
- Therefore, surface waters are dissolved oxygen-rich.
- In contrast, dissolved oxygen diminishes with depth, and as depth increases, the rate of biodegradation slows down.
- When the oxygen supply in the deepest water and sediment is reduced, the hydrocarbon material biodegrades anaerobically, without oxygen.
- Consequently, it takes microorganisms a very long time to decompose petroleum hydrocarbon compounds that disperse and reach the deepest seas and oceans, which are typically covered by silt.
10. Availability of Nutrients
- Due to the constant introduction of hydrocarbon pollutants, it has been established that the organic content of petroleum-polluted areas is extraordinarily high.
- Due to the high levels of carbon in hydrocarbons and the lack of other nutrient components essential for the growth of microorganisms, the rate and value of biodegradation have been constrained by the limited availability of phosphorus and nitrogen.
- As a result, the intake of nitrogen and phosphorus for hydrocarbon degradation significantly boosted the growth of bacteria that digest hydrocarbon compounds. The carbon: nitrogen: phosphorus ratio should be maintained at 120:10:1 for the cleanup.
- The types and amounts of nutritional materials present in the hydrocarbon biodegradation process played a crucial role in lowering the hydrocarbon biodegradation rate.
References
- Leahy JG, Colwell RR. Microbial degradation of hydrocarbons in the environment. Microbiol Rev. 1990 Sep;54(3):305-15. doi: 10.1128/mr.54.3.305-315.1990. PMID: 2215423; PMCID: PMC372779.
- Ghazali, F. M., Rahman, R. N. Z. A., Salleh, A. B., & Basri, M. (2004). Biodegradation of hydrocarbons in soil by microbial consortium. International Biodeterioration & Biodegradation, 54(1), 61–67. doi:10.1016/j.ibiod.2004.02.002
- Rabus, R., Boll, M., Heider, J., Meckenstock, R. U., Buckel, W., Einsle, O., … Wilkes, H. (2016). Anaerobic Microbial Degradation of Hydrocarbons: From Enzymatic Reactions to the Environment. Journal of Molecular Microbiology and Biotechnology, 26(1-3), 5–28. doi:10.1159/000443997
- Ławniczak, Ł., Woźniak-Karczewska, M., Loibner, A. P., Heipieper, H. J., & Chrzanowski, Ł. (2020). Microbial Degradation of Hydrocarbons—Basic Principles for Bioremediation: A Review. Molecules, 25(4), 856. doi:10.3390/molecules25040856
- Leahy JG, Colwell RR. Microbial degradation of hydrocarbons in the environment. Microbiol Rev. 1990 Sep;54(3):305-15. doi: 10.1128/mr.54.3.305-315.1990. PMID: 2215423; PMCID: PMC372779.
- Xu, X., Liu, W., Tian, S., Wang, W., Qi, Q., Jiang, P., … Yu, H. (2018). Petroleum Hydrocarbon-Degrading Bacteria for the Remediation of Oil Pollution Under Aerobic Conditions: A Perspective Analysis. Frontiers in Microbiology, 9. doi:10.3389/fmicb.2018.02885
- Das, N., & Chandran, P. (2011). Microbial Degradation of Petroleum Hydrocarbon Contaminants: An Overview. Biotechnology Research International, 2011, 1–13. doi:10.4061/2011/941810
- Abdel-Shafy, H.I., Mansour, M.S.M. (2018). Microbial Degradation of Hydrocarbons in the Environment: An Overview. In: Kumar, V., Kumar, M., Prasad, R. (eds) Microbial Action on Hydrocarbons. Springer, Singapore. https://doi.org/10.1007/978-981-13-1840-5_15
- Kothari, Vijay & Panchal, Meera & Srivastava, Namrata. (2014). Microbial Degradation of Hydrocarbons. National Science Digital Library.
- Sierra-Garcia, I. N. , & de Oliveira, V. M. (2013). Microbial Hydrocarbon Degradation: Efforts to Understand Biodegradation in Petroleum Reservoirs. In R. Chamy, & F. Rosenkranz (Eds.), Biodegradation – Engineering and Technology. IntechOpen. https://doi.org/10.5772/55920
- Al-Hawash, A. B., Dragh, M. A., Li, S., Alhujaily, A., Abbood, H. A., Zhang, X., & Ma, F. (2018). Principles of microbial degradation of petroleum hydrocarbons in the environment. The Egyptian Journal of Aquatic Research, 44(2), 71–76. doi:10.1016/j.ejar.2018.06.001
- https://journals.asm.org/doi/pdf/10.1128/mr.54.3.305-315.1990
- https://www.enviro.wiki/index.php?title=Biodegradation_-_Hydrocarbons
- https://biomedgrid.com/pdf/AJBSR.MS.ID.002182.pdf
- https://i1432.shushida.cn/index4.html
- http://dzumenvis.nic.in/Microbes%20and%20Soil%20Fertility/pdf/Microbial%20Degradation%20of%20Hydrocarbons%20in%20the%20Environment.pdf
- https://www.slideshare.net/ssmvjunwani/biodegradation-of-hydrocarbon
- https://www.slideshare.net/sajjadmughal3344/biodegradation-of-xenobiotics-75556312