Contents
What is Plant Growth Promoting Rhizobacteria (PGPR)?
- Plant growth-promoting rhizobacteria are bacteria that invade plant roots and encourage plant development (PGPR).
- The rhizosphere is the zone of soil that is specifically influenced by a plant’s root system.
- Compared to the bulk soil, this zone is nutrient-rich because to the buildup of plant exudates, such as amino acids and sugars, which provide a rich source of energy and nutrition for bacteria.
- This is mirrored by the fact that the number of bacteria found near the roots of plants is often 10 to 100 times more than in the bulk of the soil.
- The microorganisms inhabiting the rhizosphere are known as rhizobacteria. The rhizosphere is inhabited by a wide variety of microorganisms.
- On the basis of their influence on plant growth, bacteria associated with plants can be divided into useful, harmful, and neutral categories.
- Rhizobacteria are the common name for plant growth-promoting free-living soil bacteria.
- PGPRs populate the rhizosphere, rhizoplane (root surface), or the root itself, independent of the processes that promote vegetative development (within radicular tissues).
- It is well-established that only 1% to 2% of bacteria in the rhizosphere promote plant growth.
- Diverse genera of bacteria have been discovered as PGPR, with Bacillus and Pseudomonas species predominating.
- The effects of PGPR on plant growth might be either indirect or direct. The direct encouragement of plant growth by PGPR involves either supplying the plant with a substance generated by the bacteria, such as phytohormones, or aiding the plant’s uptake of specific nutrients from the environment.
- When PGPR reduce or eliminate the detrimental impacts of one or more phytopathogenic organisms, they indirectly promote plant growth.
- This can occur by generating antagonistic chemicals or inducing pathogen resistance.
- A specific PGPR may affect plant growth and development by one or more of the processes listed above.
- As biocontrol agents, PGPR can function through a variety of mechanisms, regardless of their role in direct growth promotion, such as the generation of auxin phytohormone, the reduction of plant ethylene levels, and nitrogen fixation associated with root systems.
- The economic use of PGPR and its interactions with plants holds enormous promise for sustainable agriculture.
- The applications of these connections in corn, wheat, oat, barley, peas, canola, soy, potatoes, tomatoes, lentils, radicchio, and cucumber have been examined.
Mechanism of action of Plant Growth Promoting Rhizobacteria (PGPR)
The synthesis of different chemicals alters the entire microbial community in the rhizosphere niche to facilitate PGPR-mediated plant growth promotion. In general, PGPR stimulates plant growth either directly by enabling resource acquisition (nitrogen, phosphorus, and critical minerals) or indirectly by reducing the inhibitory effects of various diseases on plant growth and development via biocontrol agents.
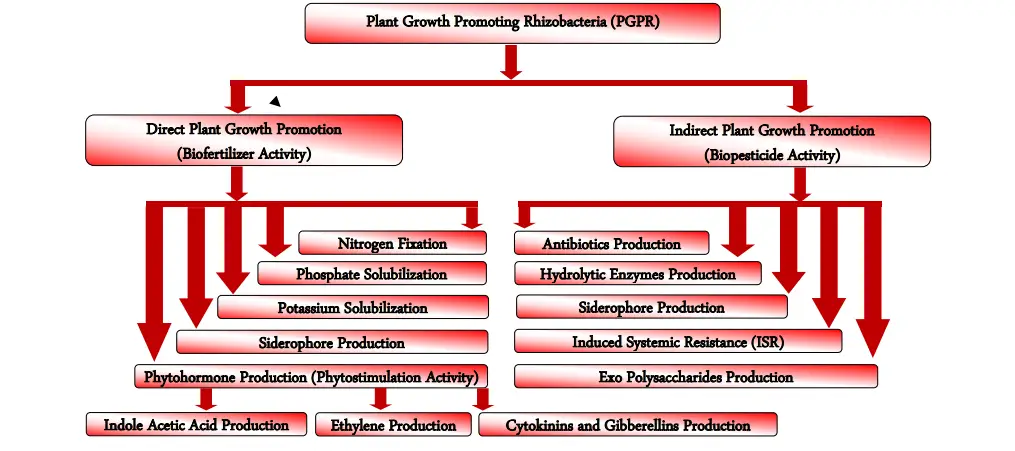
A. Direct mechanisms
1. Nitrogen fixation
- Nitrogen is the most important nutrient for plant growth and yield, and it is necessary for all forms of life.
- Even while nitrogen makes up about 78 percent of the air, it can’t be used by plants.
- Unfortunately, no plant species can convert atmospheric dinitrogen into ammonia and use it directly for growth.
- Biological nitrogen fixation (BNF) converts nitrogen gas in the air into usable forms for plants by using a complicated enzyme system called nitrogenase to catalyse the conversion of nitrogen to ammonia by nitrogen-fixing bacteria.
- Rhizobia that aid plant development can either symbiotically or non-symbiotically fix air nitrogen, making it available to the host plant.
Symbiotic nitrogen fixation
- When a bacterium and a plant work together to fix nitrogen in the soil, they are engaged in a symbiotic interaction.
- The bacterium invades the root and then develops into a nodule, where nitrogen is fixed.
- The rhizobacteria in the phylum Rhizobia colonise the roots of leguminous plants, forming nodules where nitrogen is converted to ammonia and made available to the plant.
- Rhizobium, Bradyrhizobium, Sinorhizobium, and Mesorhizobium are common symbionts found in leguminous plants; Frankia is found in non-leguminous trees and shrubs.
Non-symbiotic nitrogen fixation
- Meanwhile, free-living diazotrophs do non-symbiotic nitrogen fixation, which can promote the development of non-legume plants like radish and rice.
- Bacteria such as Azoarcus, Azotobacter, Acetobacter, Azospirillum, Burkholderia, Diazotrophicus, Enterobacter, Gluconacetobacter, Pseudomonas, and cyanobacteria, which are not symbiotic, are found in the rhizosphere and fix nitrogen (Anabaena, Nostoc).
- Both symbiotic and free-living systems have the nif genes necessary for nitrogen fixing.
- The manufacture and function of nitrogenase (nif) entail the expression of structural genes, such as those responsible for activating the Fe protein, synthesis of the iron molybdenum cofactor, electron donation, and the expression of regulatory genes.
- Crop inoculation using biological nitrogen-fixing plant growth-promoting rhizobacteria offers an all-encompassing method of controlling pests and stimulating healthy growth, all while keeping the nitogen level in the soil where the crops are grown at a steady and sustainable level.
2. Phosphate solubilization
- Phosphorus is the second most essential element for plant nourishment, behind nitrogen (N).
- It plays a crucial part in practically all key plant metabolic activities, such as photosynthesis, energy transfer, signal transduction, macromolecular biosynthesis, and respiration.
- It is abundantly present in both organic and inorganic forms in soils. Phosphate cannot be utilised by plants because 95-99% of it exists in an insoluble, immobilised, and precipitated state.
- The only soluble forms of phosphate that plants may absorb are monobasic (H2PO4) and dibasic (HPO42-) ions.
- Rhizobacteria in the soil that promote plant growth use a variety of techniques to utilise inaccessible types of phosphorus and, in turn, assist in making phosphorus available for plant absorption.
- As soil inoculums to promote plant growth and yield, phosphate-solubilizing PGPR from the genera Arthrobacter, Bacillus, Beijerinckia, Burkholderia, Enterobacter, Erwinia, Flavobacterium, Microbacterium Pseudomonas, Rhizobium, Rhodococcus, and Serratia have drawn the interest of agriculturists.
- The primary phosphate solubilization processes utilised by rhizobacteria that promote plant growth are as follows:
- Release of chemicals capable of complexing or dissolving minerals, such as organic acid anions, protons, hydroxyl ions, and CO2.
- The discharge of extracellular enzymes (biochemical phosphate mineralization).
- Phosphate released during substrate breakdown (biological phosphate mineralization).
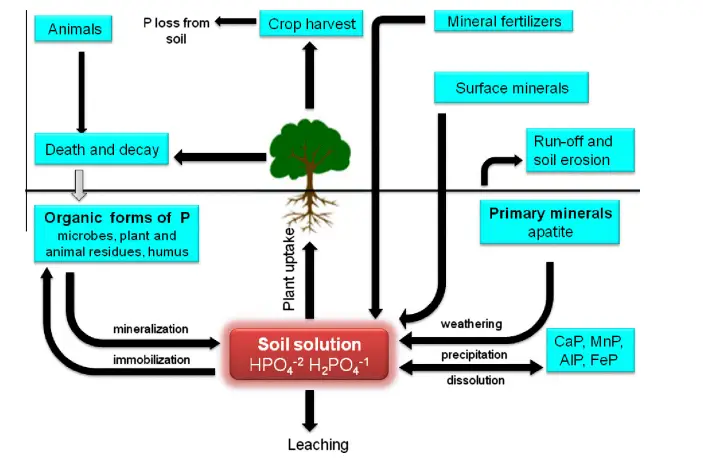
3. Potassium solubilization
- For healthy plant development, potassium (K) is the third most important macronutrient.
- More than 90% of the soil’s potassium is present as insoluble rocks and silicate minerals, while the remaining 10% is found in soluble salts and organic matter.
- However, potassium deficit is becoming one of the greatest restrictions in agricultural productivity because of uneven fertiliser use.
- Low potassium levels result in stunted plant growth, tiny seeds, and fewer harvests.
- As a result, efforts have been redoubled to identify a domestic source of potassium that can be used by plants and used by soils to keep potassium levels high enough to support crop yields.
- Beneficial rhizobacteria can dissolve potassium rock because they produce and secrete organic acids.
- Acidothiobacillus ferrooxidans, Bacillus edaphicus, Bacillus mucilaginosus, Burkholderia, Paenibacillus sp., and Pseudomonas sp. are only a few of the potassium-solubilizing plant growth-promoting rhizobacteria that have been shown to liberate potassium from potassium-bearing minerals in soils.
- Therefore, the usage of agrochemicals can be decreased, and environmentally friendly crop production can be supported, by the application of potassium-solubilizing plant growth-promoting rhizobacteria as biofertilizer for agriculture development.
4. Siderophore production
- The micronutrient iron is crucial for the survival of nearly every living thing in the biosphere.
- As the ferric ionor Fe+3, the predominant form in nature, is only sparingly soluble, the amount of iron available for assimilation by living organisms is extremely low in aerobic soils despite iron being the fourth most abundant element on earth. This is because iron is not readily assimilated by either bacteria or plants.
- In order to absorb iron, microorganisms have developed specific mechanisms, such as the synthesis of low molecular weight iron-chelating molecules called siderophores.
- The characteristic functional group allows us to classify siderophores into one of three families: hydroxamates, catecholates, or carboxylates. Over 500 unique siderophores have been identified, with 270 of those having had their structures determined.
- Indirectly and directly, siderophores have been linked to rhizobacterial plant growth promotion.
- Using radiolabeled ferric siderophores as a sole source of iron showed that plants are able to take up the labelled iron by a wide variety of plant growth promoting rhizobacteria, such as Aeromonas, Azadirachta, Azotobacter, Bacillus, Burkholderia, Pseudomonas, Rhizobium, Serratia, and Streptomyces sp., and increased chloro
5. Phytohormone production
- Microorganisms in the rhizosphere can create chemicals that control plant growth and development.
- Auxins, cytokinins, gibberellins, and ethylene, all of which are produced by plant growth-promoting rhizobacteria, influence cell proliferation in the root architecture, leading to an increase in the number of lateral roots and root hairs and, consequently, the plant’s ability to absorb nutrients and water.
6. Indole Acetic Acid (IAA)
- Natural indole acetic acid (IAA) is the most abundant auxin in plants and promotes root development.
- Colonization of the seed or root surface by the 80% of rhizobacteria that can synthesis indole acetic acid (IAA) is hypothesised to work in tandem with endogenous IAA in the host plant to increase cell proliferation and mineral absorption.
- The plant hormone indole acetic acid plays a role in photosynthesis, pigment formation, biosynthesis of various metabolites, resistance to stressful conditions, and more. It also stimulates seed and tuber germination, accelerates xylem and root development, regulates vegetative growth, and initiates the formation of lateral and adventitious roots.
- It has been determined that tryptophan, an amino acid typically found in root exudates, is the major precursor molecule for production of IAA in bacteria.
- Most bacteria, including Pseudomonas, Rhizobium, Bradyrhizobium, Agrobacterium, Enterobacter, and Klebsiella, biosynthesize indole acetic acid via the production of indole-3- pyruvic acid and indole-3-acetic aldehyde.
- There is evidence linking the low level of IAA secretion to the root development stimulation by free-living PGPR such as Alkaligenes faecalis, Enterobacter cloacae, Acetobacter dizotrophicous, species of Azospirillum, Pseudomonas, and Xanthomonas sp.
- The threshold between inhibitory and stimulatory amounts of chemically manufactured hormones is minimal, but the continual gradual release of microbial hormones makes them more effective.
7. Cytokinins and gibberellins
- Some rhizobacteria are known to stimulate plant development. Plant growth can be stimulated by cytokinins or gibberellins, or both, produced by bacteria such as Azotobacter sp., Rhizobium sp., Pantoea agglomerans, Rhodospirillum rubrum, Pseudomonas fluorescens, Bacillus subtilis, and Paenibacillus polymyxa.
- Additionally, several phytopathogens have the ability to produce cytokinins. In contrast to the inhibitory effect of cytokinins from pathogens, the action of plant growth-promoting rhizobacteria appears to be stimulatory.
- To name just a few of its many functions, ethylene stimulates seed germination, promotes decreased withering, stimulates fruit ripening, promotes leaf abscission, and even activates the creation of other plant hormones.
- Defoliation and other cellular processes are induced by the high concentration of ethylene, which may affect crop performance.
- To produce ethylene, ACC oxidase must first convert 1-aminocyclopropane-1 carboxylic acid (ACC) into ethylene.
- Reduced ethylene production through inoculation with plant growth promoting strains of Pseudomonas sp. expressing ACC deaminase and R. leguminosarum increased the number of nodules, nodule dry weight, fresh biomass, grain yield, straw yield, and nitrogen content in grains of lentil.
- Many different types of bacteria, including Acinetobacter, Achromobacter, Agrobacterium, Alcaligenes, Azospirillum, Bacillus, Burkholderia, Enterobacter, Pseudomonas, Ralstonia, Serratia, and Rhizobium, have been found to possess ACC deaminase activity.
8. 1-Aminocyclopropane-1-carboxylate (ACC) deaminase
- The metabolite ethylene plays a crucial role in typical plant development.
- Important in generating a wide variety of physiologic changes in plants, this plant growth hormone is produced endogenously by nearly all plants and also by several biotic and abiotic processes in soils.
- Ethylene is well-known as a plant growth regulator and stress hormone.
- The endogenous level of ethylene increases dramatically under stress conditions as those induced by salt, drought, water logging, heavy metals, and pathogenicity, and this has a negative impact on plant growth as a whole.
- For instance, defoliation and other cellular processes may be induced by the high quantity of ethylene, leading to diminished crop performance.
- Rhizobacteria that produce 1-aminocyclopropane-1-carboxylate (ACC) deaminase promote plant growth and development by lowering ethylene levels, inducing salt tolerance, and lessening the effects of drought stress.
- Numerous bacterial species, including Acinetobacter, Achromobacter, Agrobacterium, Alcaligenes, Azospirillum, Bacillus, Burkholderia, Enterobacter, Pseudomonas, Ralstonia, Serratia, and Rhizobium, have been found to have isolates with ACC deaminase activity.
- In order to produce ethylene, these rhizobacteria take up the ethylene precursor ACC and convert it into 2-oxobutanoate and NH3.
- Producing ACC deaminase helps plants deal with a wide variety of adversities, including those caused by phytopathogenic microorganisms (viruses, bacteria, fungi, etc.), as well as polyaromatic hydrocarbons, heavy metals, radiation, wounding, insect predation, high salt concentration, draught, extremes of temperature, high light intensity, and flooding.
- Therefore, plant root elongation, encouragement of shoot growth, and enhancement in rhizobial nodulation and N, P, and K uptake, in addition to mycorrhizal colonisation, are the most notable impacts of seed/root inoculation with ACC deaminase-producing rhizobacteria in a variety of crops.
In-direct mechanisms
- Phytopathogenic microorganisms are a major and chronic danger to sustainable agriculture and ecosystem stability globally; they subvert soil ecology, disrupt the environment, damage soil fertility, and have negative impacts on human health as well as contaminate ground water.
- Indirectly achieving sustainable soil fertility and plant growth using plant growth-promoting rhizobacteria is a promising, sustainable, and environmentally beneficial method.
- This strategy has led to a reduction in the use of agrochemicals (fertilisers and pesticides) for improving soil fertility through a variety of processes, including the synthesis of antibiotics, siderophores, HCN, hydrolytic enzymes, etc.
1. Antibiosis
- Antibiotic synthesis is regarded as one of the most effective and well-studied biocontrol methods of plant growth-promoting rhizobacteria against phytopathogens. Our understanding of this biocontrol process has improved significantly over the past two decades.
- Antibiotics that inhibit the proliferation of plant pathogens include amphisin, 2,4-diacetylphloroglucinol (DAPG), oomycin A, phenazine, pyoluteorin, pyrrolnitrin, tensin, tropolone, and cyclic lipopeptides produced by pseudomonads and oligomycin A, kanosamine, zwittermicin A, and (Generally fungi).
- Some phytopathogens may develop resistance to specific antibiotics as a result of the increased usage of antibiotic-producing plant growth boosting rhizobacteria as biocontrol agents.
- Some researchers have deployed biocontrol strains that produce one or more antibiotics to prevent this from occurring. Antibiotic 2, 4-diacetylphloroglucinol (2, 4- DAPG)-producing Pseudomonas sp. was identified in soils as a biocontrol agent for wheat diseases caused by the fungus Gaeumyces graminis var. tritici.
- In approximately 60% of field studies, bacterization of wheat seeds with P. fluorescens strains producing the antibiotic phenazine-1-carboxylic acid (PCA) significantly suppressed take-all.
- Bacillus amyloliquefaciens is well-known for the generation of lipopeptides and polyketides for biological control and plant growth promotion against soil-borne diseases.
- In addition to manufacturing antibiotics, certain rhizobacteria can also produce the volatile chemical hydrogen cyanide (HCN) for the biocontrol of black root rot of tobacco caused by Thielaviopsis basicola.
- Pseudomonas’ synthesis of DAPG and HCN contributes to the biological control of tomato bacterial canker.
2. Lytic enzymes
- Rhizobacteria that promote plant development also utilise enzymatic activity to promote plant growth.
- Rhizobacterial strains that promote plant growth can create enzymes such as chitinases, dehydrogenase, -glucanase, lipases, phosphatases, proteases, etc. that demonstrate hyperparasitic activity by excreting cell wall hydrolases and attacking pathogens.
- By inhibiting pathogenic fungi such as Botrytis cinerea, Sclerotium rolfsii, Fusarium oxysporum, Phytophthora sp., Rhizoctonia solani, and Pythium ultimum, plant growth promoting rhizobacteria play a crucial role in promoting plant growth, especially in protecting plants from biotic and abiotic stresses.
- Pseudomonas fluorescens CHA0 suppresses black root rot of tobacco caused by the fungus Thielaviopsis basicola, Pseudomonas putida against Macrophomina phaseolina in chickpea, and Azotobacter chroococcum against Fusarium oxysporum in Sesamum indicum in field conditions, according to a number of reports.
- In recent years, Pseudomonas fluorescens has been proposed as a potential biological control agent due to its ability to colonise rhizosphere and protect plants against a broad spectrum of important agronomic fungal diseases, such as black root-rot of tobacco, root-rot of mustard, and damping-off of sugar beet in field conditions.
- It has been established that arbascular mycorrhiza injection enhances plant growth. Some Trichoderma strains have been extensively used as biological control agents and plant growth enhancers.
- Inoculation with Trichoderma sp. has been the biocontrol agent of choice against Aspergillus niger, the causal agent of peanut collar rot.
- The use of multistrain inoculants is also an effective method for enabling organisms to survive and thrive in communities.
3. Siderophore
- Iron is a necessary component for growth in all living creatures.
- At neutral and alkaline pH, the availability of soluble ferric ion to soil microorganisms is restricted.
- By sequestering Fe3+ in the root zone, siderophore-producing plant growth-promoting rhizobacteria can limit the multiplication of harmful microbes.
- These siderophores connect with ferric ion to form a siderophore-ferric complex, which then binds with iron-limitation-dependent receptors on the surface of bacterial cells.
- Subsequently, the Ferric ion is liberated and becomes active in the cytoplasm as the Ferrous ion. Numerous plants can utilise diverse bacterial siderophores as iron sources, although the total concentrations are likely too low to significantly contribute to plant iron uptake.
- In numerous research, siderophore-producing bacteria of the Bradyrhizobium, Pseudomonas, Rhizobium, Serratia, and Streptomyces genera were isolated from the rhizosphere.
4. Induced systemic resistance (ISR)
- Induced resistance is a physiological condition of improved defensive capacity induced in response to certain environmental stimuli; as a result, the plant’s intrinsic defences are strengthened against subsequent biotic threats.
- Biopriming plants with certain plant growth promoting rhizobacteria can also confer systemic resistance against a wide range of plant diseases.
- After the application of plant growth boosting rhizobacteria, plant diseases of fungal, bacterial, and viral origin, and in some cases, insect and worm damage, can be decreased.
- In addition, induced systemic resistance involves jasmonate and ethylene signalling within the plant, and these hormones increase the host plant’s defence responses against numerous plant diseases.
- Numerous bacterial components, such as lipopolysaccharides (LPS), flagella, siderophores, cyclic lipopeptides, 2, 4-diacetylphloroglucinol, homoserine lactones, and volatiles such as acetoin and 2, 3-butanediol, generate induced systemic resistance.
5. Exo polysaccharides production or biofilm formation
- Certain bacteria produce a broad range of multifunctional polysaccharides, including internal, structural, and extracellular polysaccharides.
- Root colonisation can impact the interaction of bacteria with root appendages.
- Effective colonisation of plant roots by EPS-producing microorganisms assists in separating free phosphorous from insoluble phosphorous in soils, providing vital nutrition to the plant for healthy growth and development, and protecting it from the attack of foreign pathogens.
- Other numerous tasks performed by EPS-producing bacteria in plant–microbe interactions include protection against desiccation, protection against stress, adhesion to plant invasion surfaces, and plant defence response.
- Exo polysaccharides produced by plant growth-promoting rhizobacteria are crucial for encouraging plant growth because they function as an active signal molecule during beneficial interactions and provide a defence response during the infection phase.
- Some exo polysaccharides produced by plant growth-promoting rhizobacteria can bind cations, including Na+, suggesting a function in mitigating salt stress by decreasing the amount of Na+ available for plant absorption.
References
- Beneduzi A, Ambrosini A, Passaglia LM. Plant growth-promoting rhizobacteria (PGPR): Their potential as antagonists and biocontrol agents. Genet Mol Biol. 2012 Dec;35(4 (suppl)):1044-51. doi: 10.1590/s1415-47572012000600020. Epub 2012 Dec 18. PMID: 23411488; PMCID: PMC3571425.
- Bhattacharyya PN, Jha DK. Plant growth-promoting rhizobacteria (PGPR): emergence in agriculture. World J Microbiol Biotechnol. 2012 Apr;28(4):1327-50. doi: 10.1007/s11274-011-0979-9. Epub 2011 Dec 24. PMID: 22805914.
- Backer, R., Rokem, J. S., Ilangumaran, G., Lamont, J., Praslickova, D., Ricci, E., … Smith, D. L. (2018). Plant Growth-Promoting Rhizobacteria: Context, Mechanisms of Action, and Roadmap to Commercialization of Biostimulants for Sustainable Agriculture. Frontiers in Plant Science, 9. doi:10.3389/fpls.2018.01473
- Ahemad, M., & Kibret, M. (2014). Mechanisms and applications of plant growth promoting rhizobacteria: Current perspective. Journal of King Saud University – Science, 26(1), 1–20. doi:10.1016/j.jksus.2013.05.001
- Zheng, W., Zeng, S., Bais, H., LaManna, J. M., Hussey, D. S., Jacobson, D. L., & Jin, Y. (2018). Plant Growth-Promoting Rhizobacteria (PGPR) Reduce Evaporation and Increase Soil Water Retention. Water Resources Research, 54(5), 3673–3687. doi:10.1029/2018wr022656
- Jha, Dr. Chaitanya & Saraf, Meenu. (2015). Plant growth promoting Rhizobacteria (PGPR): a review. 10.13140/RG.2.1.5171.2164.
- Kenneth, O. C., Nwadibe, E. C., Kalu, A. U., & Unah, U. V. (2019). Plant Growth Promoting Rhizobacteria (PGPR): A Novel Agent for Sustainable Food Production. American Journal of Agricultural and Biological Sciences, 14(1), 35–54. doi:10.3844/ajabssp.2019.35.54
- https://www.ijcmas.com/abstractview.php?ID=1843&vol=6-3-2017&SNo=220
- https://iopscience.iop.org/article/10.1088/1755-1315/709/1/012079/pdf
- https://ijabe.org/index.php/ijabe/article/view/5762
- https://microbiologyjournal.org/plant-growth-promoting-rhizobacteria-pgpr-prospective-and-mechanisms-a-review/
- https://en.wikipedia.org/wiki/Rhizobacteria
- https://www.walshmedicalmedia.com/open-access/plant-growth-promoting-rhizobacteria-pgpr-current-and-future-prospects-for-development-of-sustainable-agriculture-1948-5948-1000188.pdf
- https://www.ias.ac.in/public/Volumes/reso/018/03/0275-0281.pdf
- https://agupubs.onlinelibrary.wiley.com/doi/full/10.1029/2018WR022656