Contents
What is Downstream processing?
Downstream processing refers to the procedures required to recover and purify fermentation-produced products. In the step of downstream processing, particles, mostly cells, are separated from the liquid using filtration, centrifugation, and flocculation.
- Downstream processing refers to the recovery and purification of biosynthetic products, notably pharmaceuticals, from natural sources such as animal tissue, plant tissue, or fermentation broth, as well as the recycling of salvageable components and the proper disposal of waste.
- It is a crucial stage in the production of antibiotics, hormones (such as insulin and human growth hormone), antibodies (such as infliximab and abciximab), and vaccines; diagnostic antibodies and enzymes; industrial enzymes; and natural fragrance and flavour chemicals.
- Typically, downstream processing is seen as a subfield of biochemical engineering, which is itself a subfield of chemical engineering.
- Many of the fundamental technologies for laboratory-scale separation of biological and synthetic products were invented by chemists and biologists, whereas the duty of biochemical and chemical engineers is to improve these technologies for greater production capacities.
- Downstream processing and analytical bioseparation both relate to the separation or purification of biological products, but for distinct purposes.
- Analytical bioseparation refers to purification for the sole purpose of measuring a component or components of a mixture, and may involve sample sizes as small as a single cell. Downstream processing refers to the manufacture of a purified product fit for a specific use, typically in marketable quantities, whereas analytical bioseparation refers to purification for the sole purpose of measuring a component or components of a mixture.
Steps Of Downstream Processing
Five stages in downstream processing after Fermentation:
- Solid-Liquid Separation
- Release of Intracellular Products
- Concentration
- Purification by Chromatography and
- Formulation.
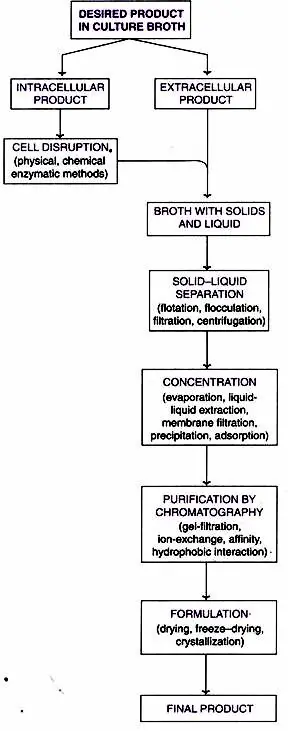
Stage 1: Solid-Liquid Separation
- The initial step in product recovery involves the separation of entire cells (cell biomass) and other insoluble components from the culture broth (Note: If the desired product is an intracellular metabolite, it must be released from the cells before subjecting to solid-liquid separation).
- Some publications refer to the separation of microbial cells from the culture media as harvesting.
- Several techniques are employed for solid-liquid separation, including:
- Flotation
- Flocculation
- Filtration
- Centrifugation
1. Flotation
- When a gas is put into a liquid, bubbles are formed.
- On gas bubbles, cells and other solid particles are adsorbed.
- These bubbles ascend to the foam layer, where they can be collected and eliminated.
- Certain compounds, known as collector substances, encourage the development of stable foam, such as long-chain fatty acids and amines.
2. Flocculation
- In flocculation, the cells (or cell detritus) form huge clumps so that they can be easily removed.
- The flocculation process is dependent on the type of the cells and the ionic composition of the medium.
- Often, flocculation requires the addition of flocculating agents (inorganic salt, organic polyelectrolyte, mineral hydrocolloid).
3. Filtration
- The most used method for separating biomass and culture filtrate is filtration.
- Numerous variables affect the efficacy of filtration, including the size of the organism, the presence of other organisms, the viscosity of the medium, and the temperature.
- There are numerous filters in use, including depth filters, absolute filters, rotary drum vacuum filters, and membrane filters.
a. Depth Filters
- They consist of a matrix of filaments, such as glass wool, asbestos, or filter paper.
- The particles are captured by the matrix, while the fluid escapes.
- By utilising depth filters, filamentous fungi can be removed.
b. Absolute Filters
- The particular pore diameters of these filters are smaller than the particles to be removed.
- Absolute filters are able to remove bacteria from culture media.
c. Rotary Drum Vacuum Filters
- These filters are widely used to separate broth containing 10-40% solids (by volume) and 0.5-10m-sized particles.
- Utilizing rotary drum vacuum filters for the filtering of yeast cells and filamentous fungus has proven effective.
- The technology is straightforward, consumes little energy, and is simple to operate. The filtration apparatus is comprised of a revolving drum partially submerged in a broth tank.
- As the drum rotates, the biomass is collected and deposited as a cake on its surface. This filter cake is simple to remove.
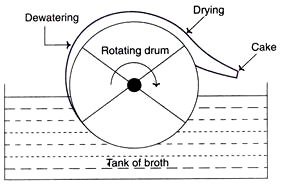
d. Membrane Filters
- In this sort of filtration, certain pore size membranes can be utilised. However, filter clogging is a significant constraint.
- There are two distinct forms of membrane filtration: static and cross-flow.
- During cross-flow filtration, the culture broth is pumped across the membrane in a transverse direction.
- This decreases clogging and is therefore superior to static filtration.
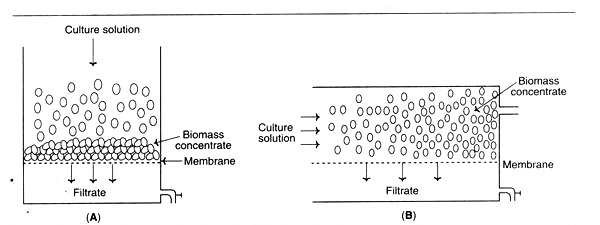
Types of membrane filter
Based on particle sizes and other characteristics, there are three major types of filtration. These include:
- Microfiltration: These are 0.1 – 10 um in diameter. It used to separate cells or cell fractions, viruses.
- Ultrafiltration: 0.001um – 0.1um in diameter size. It used to separate compounds with molecular weight greater than 1000 (e.g. enzyme).
- Reverse osmosis (hyperfiltration): These are 0.0001um to 0.001um in diameter. It used to separate compounds with molecular weight less than 1000 (e.g. lactose).
4. Centrifugation
- The separation of particles by centrifugation relies on density differences between the particles to be separated and the medium.
- Therefore, centrifugation is predominantly employed to separate solid particles from liquid phase (fluid/particle separation).
- Contrary to laboratory-scale centrifugation, industrial-scale centrifugation is restricted by specific constraints.
- However, continuous flow industrial centrifuges have been created in recent years. There is continuous feeding of the slurry and collection of cleared fluid, while occasional removal of deposited solids is possible.
- The various types of centrifuges are detailed in detail below.
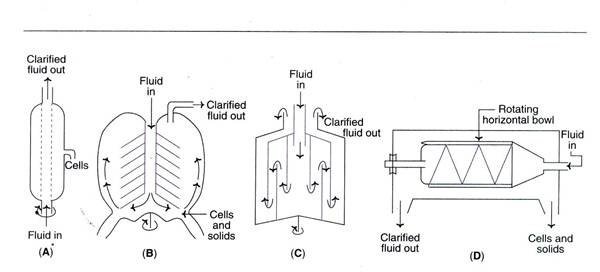
a. Tubular bowl centrifuge
- This is a simple and compact centrifuge that is frequently employed in pilot plants.
- It is possible to operate a tubular bowl centrifuge at a high centrifugal speed and in either batch or continuous mode.
- The solids are manually extracted.
b. Disc centrifuge
- It is composed of a number of discs that divide the bowl into settling zones.
- The feed/slurry is introduced via a central tube.
- The purified fluid rises as the sediments settle to the bottom of the container.
c. Multi-chamber centrifuge
- This is a variation on the tubular bowl type of centrifuge.
- It comprises of many chambers interconnected in such a way that the feed moves in a zigzag pattern.
- There are differences in centrifugal force between chambers.
- As a result of the much greater force in the peripheral chambers, the smallest particles settle in the outermost chamber.
d. Scroll centrifuge or decanter
- It consists of a spinning horizontal bowl with a tapered end.
- Typically, the decanter is used to concentrate fluids with a high solid concentration (biomass content between 5 and 80 percent).
- The solids are deposited on the bowl’s wall and can be scraped off and removed through the narrow end.
Stage # 2. Release of Intracellular Products
- As noted previously, there are several biotechnological products (vitamins, enzymes) contained within cells.
- Such compounds must initially be released (in their most active state) prior to further processing and isolation.
- Physical, chemical, or enzymatic procedures can disintegrate or disrupt the bacteria or other cells.
- The many strategies for breaking apart cells are described.
- The selection of a particular approach depends on the nature of the cells, as the property of cell disruption or breaking varies widely.
- Gram-negative bacteria and filamentous fungi, for instance, are easier to destroy than Gram-positive bacteria and yeasts.
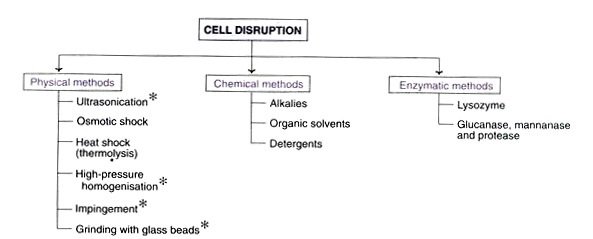
A. Cell Disruption
1. Physical methods of cell disruption
Certain physical procedures can disturb bacteria or cells to liberate intracellular products.
i. Ultra sonication
- In the laboratory, ultrasonic disintegration is widely utilised. However, due to its high cost, it is not appropriate for industrial application on a broad scale.
ii. Osmotic shock
- This technique involves suspending cells (devoid of growth media) in 20% buffered sucrose.
- The cells are subsequently transferred to roughly 4°C water.
- Gram-negative bacteria utilise osmotic shock to release hydrolytic enzymes and binding proteins.
iii. Heat shock (thermolysis)
- By subjecting cells to heat, their destruction is relatively simple and inexpensive.
- However, this method can only be applied to a small number of heat-stable intracellular substances.
iv. High pressure homogenization
- This method involves forcing a high-pressure cell suspension through a very narrow orifice to atmospheric pressure.
- This abrupt release of high pressure generates a liquid shear capable of rupturing the cells.
v. Impingement
- In this method, a stream of suspended cells with high velocity and pressure is forced to strike a stationary surface or a second stream of suspended cells (impinge literally means to strike or hit).
- At the point of contact, forces are generated that disrupt the cells. The microfluidizer is a device based on the impingement principle.
- It has been utilised successfully for destroying E. coli cells.
- The benefit of the impingement technique is that it can be used to effectively disrupt cells at low concentrations.
vi. Grinding with glass beads
- In a reaction vessel, cells combined with glass beads are treated to a very high speed.
- As the beads press the cells against the vessel wall, the cells rupture. Size and number of the glass beads, concentration and age of the cells, temperature and agitator speed all influence cell lysis.
- Under optimal conditions, approximately 80% of the cells may be destroyed.
- A schematic illustration of a cell disruptor utilising glass beads.
- It has a cylindrical body with an inlet, an outlet, and a motor-driven centre shaft. Attached to this shaft are radial agitators.
- The cylinder incorporates glass beads. The cell suspension is introduced by the inlet, while the disrupted cells exit via the outlet.
- During operation, the body of the cell disruptor is kept at a cold temperature.
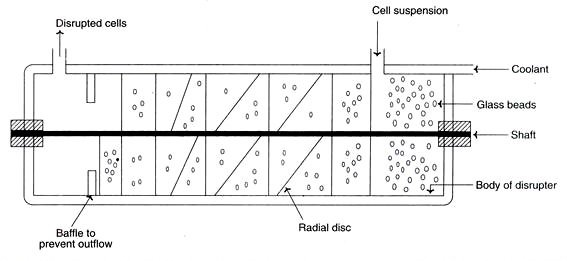
vii. Mechanical and non-mechanical methods
- Ultrasonication, high-pressure homogenization, impingement, and grinding with glass beads are mechanical methods of cell disruption, whereas osmotic shock and heat shock are non-mechanical.
- The chemical and enzymatic processes (described below) are non-mechanical.
B. Chemical methods of cell disruption
Alkalies, organic solvents, and detergents can be used to lyse cells and liberate their contents.
i. Alkalies
- Some bacterial proteins have been extracted using an alkaline solution. However, the alkali stability of the desired product is critical to the success of this procedure; for instance, recombinant growth hormone can be effectively extracted from E. coli by treating the bacteria with sodium hydroxide at a pH of 11.
ii. Organic solvents
- Several water-miscible organic solvents, such as methanol, ethanol, isopropanol, and butanol, can be employed to disturb the cells.
- These compounds are combustible, necessitating the use of specialised fire safety equipment. Toluene, an organic solvent, is often utilised.
- Toluene is thought to breakdown membrane phospholipids and form membrane holes for intracellular content release.
iii. Detergents
- Ionic detergents, such as cationic-cetyl trimethyl ammonium bromide or anionic-sodium lauryl sulphate, have the ability to denature membrane proteins and lyse cells.
- Non-ionic detergents, such as Triton X-100 or Tween, are also utilised to some extent, despite being less reactive than ionic detergents.
- The difficulty with using detergents is that they interfere with purifying processes, namely salt precipitation.
- Purification by ultrafiltration or ion-exchange chromatography can circumvent this constraint.
iv. Enzymatic methods of cell disruption
- Cell lysis happens under mild conditions and in a selective manner when cells are disrupted by enzymatic techniques.
- This is really beneficial for product recovery. Lysozyme is the most widely utilised and commercially available enzyme (produced from hen egg white).
- It hydrolyzes β-1, 4-glycosidic linkages in bacterial cell wall mucopeptides. Gram-positive bacteria (those with a high concentration of cell wall mucopeptides) are more vulnerable to lysozyme’s activity.
- In conjunction with EDTA, lysozyme can kill Gram-negative bacteria by destroying their cells. As lysozyme digests the cell wall, the osmotic effects rupture the periplasmic membrane, releasing the intracellular contents.
- Additionally, but less frequently, additional enzymes are utilised for cell disruption. In conjunction with proteases, glucanase and mannanase are used to lyse yeast cell walls.
v. Combination of methods
- A combination of physical, chemical, and enzymatic processes are utilised to increase the efficiency of cell disintegration in a cost-effective manner.
Stage # 3. Concentration
- Typically, 80-98% of the filtrate that is free of suspended particles (cells, cell debris, etc.) is water.
- The desired substance is a minor component. Water must be extracted to get the desired product concentration.
- These are the most typical ways for concentrating biological products:
- evaporation.
- liquid-liquid extraction.
- membrane filtration.
- precipitation.
- adsorption.
- Actual process depends on the nature of the desired product (quality and quantity to be preserved to the greatest extent possible) and the cost element.
1. Evaporation
- The broth filtrate can be dehydrated using a simple evaporation method. Evaporators typically include a heating device for steam supply, a unit for the separation of concentrated product and vapour, a condenser for condensing vapour, as well as accessories and control equipment.
- The equipment’s capacity is vary, ranging from small laboratory scale to industrial scale. Several of the most prevalent evaporator types are briefly described.
a. Plate evaporators
- Over plates, the liquid to be concentrated flows. As steam is introduced, the liquid becomes concentrated and viscous.
b. Falling film evaporators
- In this instance, the liquid flows through lengthy tubes and is dispersed over the heating surface as a thin coating.
- Falling film evaporators are appropriate for extracting water from thick fermentation products.
c. Forced film evaporators
- These devices are suited for the production of dry product concentrates and are mechanically driven to create liquid films.
d. Centrifugal forced film evaporators
- This device evaporates the liquid very rapidly (in seconds), making it suited for concentrating even heat-sensitive compounds.
- For instantaneous evaporation, these evaporators use centrifugal force to move liquid over heated plates or conical surfaces.
2. Liquid-Liquid Extraction
- Liquid-liquid extraction is a process that allows for the concentration of biological products by moving the target product (solute) from one liquid phase to another.
- This method is also beneficial for the partial purification of a product, in addition to concentration.
- Dependent on the partition coefficient, or the proportionate distribution of a substance between the two liquid phases, extraction efficiency is determined.
- The method of liquid-liquid extraction can be characterised roughly as extraction of low molecular weight products and extraction of high molecular weight products.
a. Extraction of low molecular weight products
- By utilising organic solvents, lipophilic substances can be extracted easily. However, it is extremely challenging to remove hydrophilic chemicals. Following are some strategies for extracting lipophilic substances.
b. Physical extraction
- On the basis of its physical properties, the compound is distributed between two liquid phases.
- This method is utilised to extract non-ionizing compounds.
c. Dissociation extraction
- This approach is suitable for ionisable chemical extraction.
- Several antibiotics can be retrieved using this method.
d. Reactive extraction
- In this instance, the intended product is caused to react with a carrier molecule (e.g., a phosphorus compound or an aliphatic amine) and then extracted with an organic solvent.
- Extraction of some substances that are extremely soluble in water (aqueous phase), such as organic acids, is facilitated by the reactive extraction technique.
e. Supercritical fluid (SCF) extraction
- This approach varies from the preceding ones since the extraction materials are supercritical fluids (SCFs). SCFs are between gases and liquids and exist as fluids above their temperature and pressure critical points.
- In extraction, supercritical CO2 with a low critical temperature and pressure is widely utilised. Therefore, supercritical fluid extraction is not utilised frequently (SCF has been used for the extraction of caffeine from coffee beans, and pigments and flavour ingredients from biological materials).
f. Extraction of high molecular weight compounds
- In fermentation industries, proteins are the most prevalent high molecular weight compounds generated.
- Organic solvents cannot be utilised to extract proteins because they lose their biological activity.
- They are extracted via aqueous two-phase systems or micelle formation in reverse.
g. Aqueous two-phase systems (ATPS)
- Mixing a polymer (e.g., polyethylene glycol) with a salt solution (ammonium sulphate) or two distinct polymers can produce them.
- The primary component of ATPS is water, although the two phases are incompatible. While cells and other solids remain in one phase, proteins are transported to another.
- The distribution of the intended product is determined by its surface and ionic properties as well as the nature of its phases.
- ATPS separation takes significantly longer.
h. Reverse miceller systems
- In organic solvents, reverse micelles are stable aggregation of surfactant molecules and water.
- By producing reverse micelles, it is possible to remove the proteins from the aqueous medium. Enzymes can be extracted with no loss of biological activity using this method.
Membrane Filtration
- In industrial biotechnology, membrane filtration has become a standard separation method.
- It is useful for the separation of biomolecules and particles, as well as the concentration of fluids.
- Membrane filtration entails the use of a semipermeable membrane that selectively holds particles/molecules larger than the pore size while allowing smaller molecules to pass through the membrane pores.
- Filtration membranes are composed of polymeric materials such as polyethersulfone and polyvinylidene fluoride.
- It is challenging to sterilise membrane filters. In recent years, ceramic and steel micro-filters and ultrafiIters have been available.
- Cleaning and sterilising these filters is simple. The different membrane filtration processes are briefly described.
i. Membrane adsorbers
- They consist of microporous or macroporous membranes containing ion exchange groups and/or affinity ligands.
- Membrane adsorbers can bind and hold proteins.
- These proteins can be eluted using chromatography solutions.
ii. Pervaporation
- Permeation via a membrane paired with evaporation is a technique for separating volatile substances.
- Pervaporation is highly beneficial for extracting, recovering, and concentrating volatile substances.
- However, this process has a disadvantage in that it cannot be utilised on a wide scale to separate volatile substances because of the cost involved.
iii. Perstraction
- This is an advanced approach based on the membrane filtration and solvent extraction principles.
- This approach can be used to recover and concentrate hydrophobic substances.
3. Precipitation
- In industry, precipitation is the most prevalent method for the concentration of macromolecules such as proteins and polysaccharides.
- In addition, the precipitation procedure can be used to remove undesirable byproducts such as nucleic acids and pigments.
- Precipitation utilises neutral salts, organic solvents, high molecular weight (ionic or non-ionic) polymers, as well as changes in temperature and pH.
- In addition to these non-specific (i.e., the type of the protein is irrelevant) protein precipitation reactions, there are some protein-specific precipitations, such as affinity precipitation and ligand precipitation.
i. Neutral salts
- Ammonium sulphate is the most popular salt because it is highly soluble, non-toxic to proteins, and inexpensive.
- Ammonium sulphate enhances the hydrophobic interactions between protein molecules, which precipitates the proteins.
- Protein precipitation is reliant on a number of variables, including protein content, pH, and temperature.
ii. Organic solvents
- For protein precipitation, ethanol, acetone, and propanol are the most widely employed organic solvents.
- They decrease the dielectric constant of the medium and increase the electrostatic contact between protein molecules, hence promoting precipitation.
- Since organic solvents denature proteins, the precipitation procedure must be conducted below 0°C.
iii. Non-ionic polymers
- Polyethylene glycol (PEG) is a non-ionic polymer with a high molecular weight that can precipitate proteins.
- It decreases the amount of accessible water for protein solvation and precipitates protein.
- PEG does not denature proteins, besides being non-toxic.
iv. Ionic polymers
- Utilized are charged polymers such polyacrylic acid and polyethyleneimine.
- They form compounds with negatively charged protein molecules, which neutralises the charge and produces precepitation.
v. Increase in temperature
- Increasing the temperature can precipitate the heat-sensitive proteins.
vi. Change in pH
- pH changes can also result in protein precipitation.
vii. Affinity precipitation
- For protein precipitation, the affinity interaction (e.g., between antigen and antibody) is utilized.
viii. Precipitation by ligands
- Utilizing ligands with specific protein binding sites has been effective for selective precipitation.
4. Adsorption
- Utilizing solid adsorbent particles, the biological products of fermentation may be concentrated.
- Activated charcoal was employed as the adsorbent material in the past.
- In recent years, cellulose-based adsorbents have been used to concentrate proteins.
- In addition, polystyrene, methacrylate, and acrylate-based matrices are utilised for the concentration of low molecular weight substances (vitamins, antibiotics, peptides).
- Adsorption can be accomplished by constructing a bed of adsorbent column and pouring culture broth through it.
- The desired product can be eluted from the adsorbent.
Stage 4 – Purification by Chromatography
- Chromatography efficiently purifies the biological products of fermentation (proteins, medicines, diagnostic chemicals, and research materials).
- Essentially, it is an analytical approach for separating closely related chemicals from a mixture.
- Typically, chromatography involves a stationary phase and a mobile phase.
- On the stationary phase is loaded the mixture of compounds to be separated. A mobile phase is responsible for eluting the chemicals.
- A single mobile phase can be employed continually, or it can be altered to assist the release of specific chemicals.
- The column’s eluate can be continually monitored (for instance, protein elution can be measured by UV absorption at 280 nm) and collected in fractions of specific volumes.
- The various types of chromatography techniques used for separation (mostly proteins) and their underlying concepts. For protein purification, numerous matrices are commercially available, such as agarose, cellulose, polyacrylamide, porous silica, cross-linked dextran, and polystyrene.
- Several key characteristics of a selection of chromatographic techniques are briefly presented.
1. Gel-filtration chromatography
- This method is also known as size-exclusion chromatography. In this method, molecules are separated based on their size, shape, and molecular weight.
- The holes in the sponge-like gel beads serve as molecular sieves to separate smaller and larger molecules.
- A solution combination containing molecules of varying sizes (such as different proteins) is applied to and eluted from the column.
- The smaller molecules penetrate the pores of the gel beads and become stuck.
- On the other hand, bigger molecules are unable to pass through the pores and are consequently the first to escape with the mobile liquid.
- On an industrial scale, gel-filtration is beneficial for removing salts and low molecular weight chemicals from high molecular weight products.
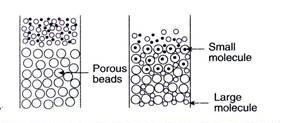
2. Ion-exchange chromatography
- It entails separating molecules according to their surface charges.
- There are two types of ion-exchangers (cation- exchangers with negatively charged groups such as carboxymethyl and sulfonate, and anion- exchangers with positively charged groups such as diethylaminoethyl) (DEAE).
- Dowex HCR and Amberlite IR are the most popular cation-exchangers, whereas Dowex SAR and Amberlite IRA are the most popular anion-exchangers.
- In ion-exchange chromatography, the pH of the medium is critical, as pH affects the net charge. In other words, pH determines both the effective charge of the target molecule and the ion-exchanger.
- The ionic-bound molecules can be eluted from the matrix by adjusting the pH of the eluant or increasing the salt concentration.
- In addition to proteins, ion-exchange chromatography is beneficial for the purification of antibiotics.
3. Affinity chromatography
- This is a sophisticated approach for separating proteins from a complicated mixture. The basis of affinity chromatography is the interaction between a protein and an immobilised ligand.
- The ligand may be a particular antibody, substrate, substrate analogue, or inhibitor.
- The ligand that has been immobilised on a solid matrix can be used to successfully extract complimentary structures.
- Reducing their connection allows for the release of the protein-ligand complex. This might be accomplished by adjusting the buffer’s pH, altering the ionic strength, or employing an additional free ligand molecule.
- The employed ligand must be eliminated in the future procedures.
4. Hydrophobic interaction chromatography (HIC)
- This is based on the weak hydrophobic interactions between hydrophobic ligands (alkyl and aryl side chains on the matrix) and hydrophobic amino acids of proteins.
- The compositional differences of hydrophobic amino acids in proteins can be exploited to separate them.
- The elution of proteins can be accomplished by reducing the salt concentration, the polarity of the medium, or the temperature.
Stage # 5. Formulation
- Formulation broadly refers to the preservation of biotechnology products’ activity and stability during storage and delivery.
- Low molecular weight products (solvents, organic acids) can be formulated by concentrating them and removing the majority of their water content.
- Certain tiny molecules (antibiotics, citric acid) can be synthesised via crystallisation with the addition of salts.
- Proteins are particularly vulnerable to losing their biological activity, necessitating stringent formulation precautions. Certain stabilising chemicals are added to protein to extend its shelf life.
- Sugars (sucrose, lactose), salts (sodium chloride, ammonium sulphate), polymers (polyethylene glycol), and polyhydric alcohols are protein formulation stabilisers (glycerol). Proteins may be manufactured as solutions, suspensions, or dry powders.
Drying
- Drying is a crucial component of product development. Essentially, it entails applying heat to a moist product in order to remove moisture.
- Most biological products of fermentation are heat-sensitive, necessitating moderate drying techniques.
- Drying equipment are classified as contact, convection, or radiation dryers based on their heat transmission technique. These three types of dryers are offered commercially.
Spray drying
- Spray drying is utilised to dry vast quantities of liquids.
- Spray drying involves passing small droplets of liquid containing the product through a nozzle that directs them over a stream of hot gas.
- The water evaporates, leaving behind the solid particles.
Freeze-drying
- Freeze-drying or lyophilization is the preferred procedure for drying and formulating a vast array of items, including medications, foodstuffs, diagnostics, bacteria, and viruses.
- This is mostly due to the fact that freeze-drying does not typically result in the loss of biological activity in the intended product.
- The principle underlying lyophilization is the sublimation of a liquid from a frozen condition. In the actual procedure, the product-containing liquid is frozen and then vacuum-dried in a freeze-dryer.
- Now that the vacuum can be released, the vials containing the product can be sealed; for instance, penicillin can be freeze-dried directly in ampules.
References
- Clarke, K. G. (2013). Downstream processing. Bioprocess Engineering, 209–234. doi:10.1533/9781782421689.209
- Johansson, M. I., Östling, M., & Jagschies, G. (2018). Downstream Processing Equipment. Biopharmaceutical Processing, 477–492. doi:10.1016/b978-0-08-100623-8.00025-6
- https://www.slideshare.net/amitcasi1/downstream-processing-group-ppt
- https://orbitbiotech.com/downstream-processing-downstream-processing-flocullation-centrifuge-purification/
- https://www.biologydiscussion.com/biotechnology/downstream-processing/stages-in-downstream-processing-5-stages/10160
- https://www.mt.com/in/en/home/applications/L1_AutoChem_Applications/fermentation/downstream-processing-in-biotechnology.html
- https://www.deshbandhucollege.ac.in/pdf/resources/1587179880_BT(H)-VI-Industrial_and_Environmetal_Microbiology-I.pdf
- https://bioprocessing.weebly.com/downstream-processing.html
- https://ijshr.com/IJSHR_Vol.1_Issue.3_Aug2016/IJSHR0002.pdf
- https://professional.mit.edu/course-catalog/downstream-processing
- http://ecoursesonline.iasri.res.in/mod/page/view.php?id=5158
- https://www.biotechnologynotes.com/industrial-biotechnology/fermentation-process/downstream-process-in-fermentation-with-methods-industries-biotechnology/13671