What is Mycorrhiza?
- Mycorrhiza, derived from the Greek words “μύκης” (mýkēs) meaning “fungus” and “ῥίζα” (rhiza) signifying “root,” refers to the symbiotic association between specific fungi and plant roots. This relationship is predominantly mutualistic, where both organisms derive benefits.
- The fungus aids the plant by enhancing water and mineral ion uptake, thanks to its extensive hyphal network that provides a broader surface area for absorption from the soil. Conversely, the plant supplies the fungus with essential nutrients, particularly sugars and nitrogenous compounds, produced through photosynthesis.
- There are two primary modes of fungal colonization in a mycorrhizal relationship. In the case of arbuscular mycorrhizal fungi (AMF or AM), the colonization is intracellular, where the fungus penetrates the root cells.
- On the other hand, ectomycorrhizal fungi establish an extracellular association, enveloping the root tissues without intruding the cells. The nature of this association can vary based on the species involved and environmental conditions. While many mycorrhizal relationships are mutualistic, certain scenarios or specific species might lead to a parasitic interaction, where one organism benefits at the expense of the other.
- It’s noteworthy that not all plants form mycorrhizal associations. In regions where the soil is rich in water and nutrients, plants might not necessitate the services of mycorrhizal fungi.
- Similarly, under such conditions, mycorrhizal fungi might not find it conducive to germinate and proliferate. However, for certain plants, the presence of mycorrhizae is crucial. A quintessential example is the Pinus species, where mycorrhizae play an indispensable role in seed germination and establishment.
- In the broader context of ecology, mycorrhizae significantly influence nutrient cycles, impacting plant evolution, physiology, and overall soil dynamics. By facilitating nutrient exchange and enhancing soil biology, these symbiotic relationships underscore the intricate interdependencies in terrestrial ecosystems.
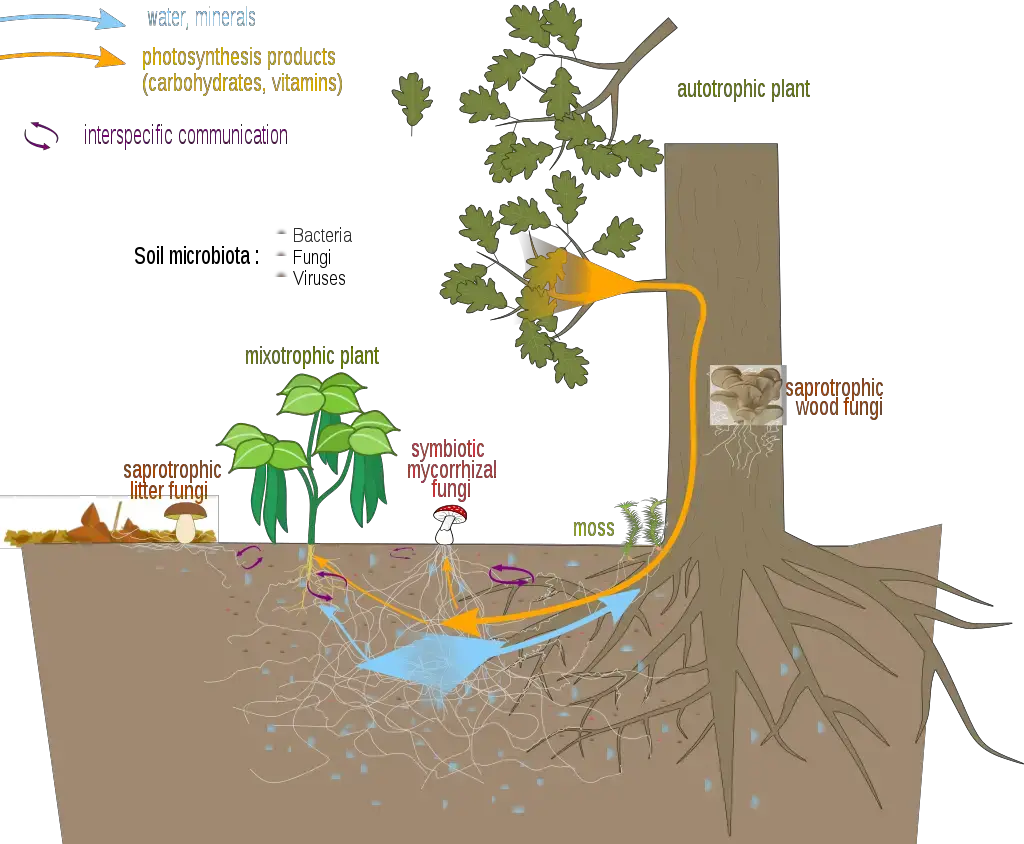
Definition of Mycorrhiza
Mycorrhiza refers to the symbiotic association between specific fungi and plant roots, where the fungus aids in nutrient and water uptake for the plant, and in return, the plant provides the fungus with essential nutrients produced through photosynthesis.
Types of Mycorrhizae
Mycorrhizas are often classified as either ectomycorrhizas or endomycorrhizas. The hyphae of ectomycorrhizal fungi do not penetrate individual root cells, but the hyphae of endomycorrhizal fungi invade the cell membrane and pierce the cell wall. Endomycorrhiza consists of arbuscular, ericoid, and orchid mycorrhiza, whereas ectoendomycorrhiza comprises arbutoid mycorrhiza. Monotropoid mycorrhizas constitute a unique category.
- Ectomycorrhiza
- Endomycorrhiza
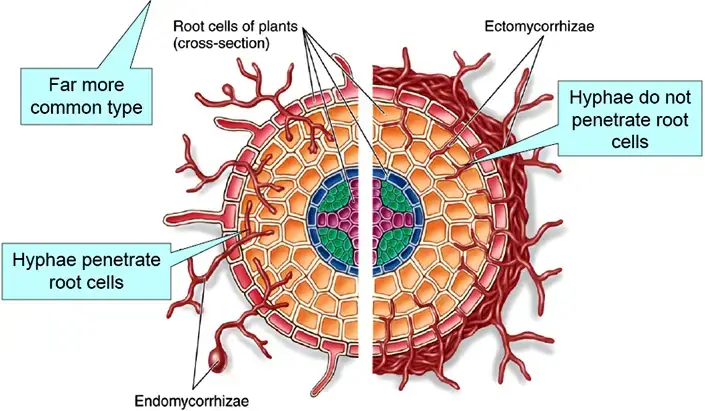
A. Ectomycorrhiza
- Ectomycorrhiza (EcM) represents a specific type of symbiotic association primarily found between certain fungi and the roots of woody plants. Notably, tree genera such as birch, beech, willow, oak, pine, spruce, fir, and eucalyptus are involved in these relationships.
- This association is characterized by the formation of a Hartig Net, an intricate intercellular network of hyphae that interfaces with the epidermal and cortical cells of the plant root. Externally, a dense sheath of hyphae, termed the mantle, envelops the root surface, distinguishing ectomycorrhizae from other mycorrhizal types.
- Intriguingly, ectomycorrhizae do not penetrate the root cells but remain external, establishing a significant portion of their fungal biomass outside the root. This external structure comprises two main components: the aforementioned mantle and the extraradical hyphae that extend into the surrounding soil.
- The mantle can vary in its complexity, ranging from a simple network of hyphae to a multilayered structure resembling plant parenchyma tissue, known as pseudoparenchymatous. The Hartig net, situated internally to the root but external to the cortical cells, serves as the primary site for nutrient and carbon exchange between the symbiotic partners.
- The colonization by ectomycorrhizal fungi often leads to suppressed root hair development and induces changes in root branching patterns. These alterations can range from simple bifurcations to extensive branching, resulting in multiple root tips enveloped by a continuous hyphal sheath. Such extensive branching is sometimes referred to as coralloid or tuberculate. The fungal hyphae also impart a distinct coloration to the mycorrhizal root surface, aiding in its identification.
- Ectomycorrhizal fungi, which include members of the Basidiomycota, Ascomycota, and Zygomycota, are often associated with forest and woodland mushrooms such as Russula, Hebeloma, and Amanita, as well as truffles like Tuber.
- While many of these fungi are generalists, associating with multiple tree species, some exhibit specificity, forming symbiotic relationships with only particular tree genera.
- A unique variant of ectomycorrhiza, termed ectendomycorrhiza, exhibits characteristics of both ectomycorrhizae and arbuscular mycorrhizae. This association is particularly observed in pines and larches and is attributed to specific fungi like those from the Pezizales order.
- In summary, ectomycorrhizae play a pivotal role in terrestrial ecosystems, facilitating nutrient exchange and enhancing the survival and growth of their host plants. Their intricate structures and diverse fungal associations underscore the complexity and significance of these symbiotic relationships in nature.
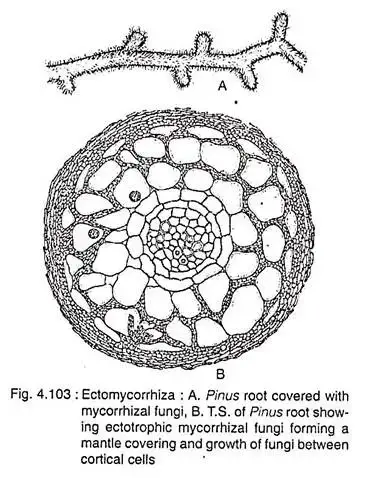
Morphology/Structure of Ectomycorrhiza
The mycosymbiont’s biomass, as the name implies, is located largely outside the plant root. The basic structure of a fungus consists of three parts: The Hartig net, composed of intraradical hyphae; the mantle, a sheath that encircles the root tip; the extraradical hyphae and associated structures, which permeate the soil.
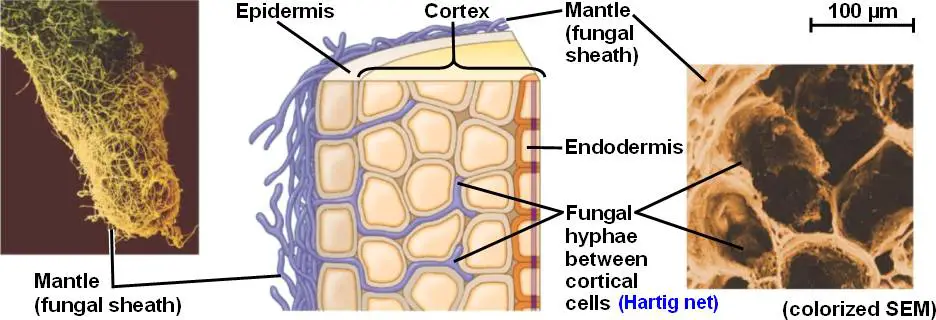
1. Hartig net
The Hartig net is a pivotal component of the ectomycorrhizal symbiosis, serving as the primary interface for nutrient and carbon exchange between the fungal and plant partners. This intricate network is formed by specialized fungal hyphae that penetrate the plant root.
- Formation and Origin: The genesis of the Hartig net is initiated by hyphae, predominantly originating from the innermost regions of the mantle. These hyphae navigate towards and subsequently infiltrate the root of the host plant.
- Structural Orientation: Upon entering the root, the hyphae establish an extensive network by interfacing with the outer cells of the root axis. This interaction is characterized by the hyphae growing in a direction that is orthogonal to the root axis, ensuring a comprehensive and efficient interface for exchange.
- Functional Significance: The Hartig net is the primary locus for the exchange of nutrients and carbon. This exchange is facilitated at the precise junctures where the fungal hyphae and root cells come into contact, underscoring the importance of this structural component in the symbiotic relationship.
- Depth of Penetration: The extent to which the Hartig net penetrates the root varies among different plant species. For instance, in species like Eucalyptus and Alnus, the Hartig net is confined to the epidermal layer. However, in many gymnosperms, this network delves deeper, reaching the cortical cells and occasionally extending to the endodermis.
- Adaptations for Enhanced Contact: To optimize the exchange of nutrients, certain adaptations are observed in the root cells. Notably, cells from various epidermal layers undergo elongation along the epidermis, increasing the surface area for interaction. Interestingly, such elongations are predominantly observed in epidermal Hartig nets, suggesting that different species might have evolved distinct strategies to enhance surface contact with the fungal partner.
2. Mantle
The ectomycorrhizal association, a symbiotic relationship between specific fungi and plant roots, features a distinct structural component known as the mantle. This element plays a pivotal role in the symbiotic interface, ensuring the effective exchange of nutrients and other essential compounds.
- Composition and Biomass: Enveloping the root is a specialized sheath, the mantle, primarily composed of fungal hyphae. Intriguingly, the biomass of the mantle often surpasses that of the Hartig net, highlighting its substantial presence in the symbiotic association.
- Structural Diversity: The mantle’s architecture can vary considerably. It can range from a loosely arranged mesh of hyphae to a meticulously structured, stratified tissue system. Such organized layers in the mantle are frequently termed “pseudoparenchymatous” due to their morphological resemblance to the plant’s parenchyma tissue.
- Impact on Root Growth: The presence of the mantle can exert certain inhibitory effects on the host root. A notable observation is the suppression of root hair development in plants that are hosts to ectomycorrhizal fungi. This phenomenon can be attributed to the encompassing nature of the mantle.
- Induction of Cytokinins and Root Branching: The mantle’s influence extends to the plant’s hormonal system, particularly inducing the production of cytokinins. This hormonal surge often culminates in enhanced root branching. In certain instances, the branching becomes so extensive that a singular, unified mantle might envelop multiple root tips simultaneously. Such specialized structures are referred to as tuberculate or coralloid ectomycorrhizas.
- Identifying Features: The mantle’s unique characteristics, such as its hue, pattern of branching, and structural intricacy, serve as distinguishing markers for different ectomycorrhizal pairings. These morphological traits, in tandem with molecular analyses, aid in ascertaining the identity of the associated fungus. While fruiting bodies of the fungus can offer additional identification cues, their sporadic presence can sometimes limit their utility.
3. Extraradical hyphae and linkage
Ectomycorrhizal symbiosis, a mutualistic association between specific fungi and plant roots, encompasses a range of structural components, one of which is the extraradical hyphae. This component plays a pivotal role in nutrient acquisition and transport, as well as interplant communication.
- Compensation for Root Hair Loss: Upon colonization by the ectomycorrhizal fungus, there’s a notable reduction in root hair development. To counteract this loss, the fungus extends its extraradical hyphae from the mantle, reaching out into the surrounding soil matrix.
- Formation of Rhizomorphs: The extraradical hyphae can either proliferate independently or aggregate to form composite structures known as rhizomorphs. These can vary from simple linear arrays of parallel-oriented hyphae to more complex configurations. Some rhizomorphs exhibit a central core of thicker hyphae, while others display tip growth reminiscent of plant meristematic activity.
- Role in Nutrient Transport: Termed as the extraradical or extramatrical mycelium, this ectomycorrhizal structure primarily functions as a conduit for nutrient transport. Its extensive reach, often spanning several yards, ensures a vast surface area remains in contact with the soil, facilitating efficient nutrient uptake.
- Correlation with Nutrient Transfer Rates: Research has unveiled a correlation between the structural intricacies of rhizomorphs and their efficiency in nutrient transfer. The unique architectural patterns and growth dynamics of rhizomorphs in various ectomycorrhizal associations serve as identifiers, aiding in discerning the fungal partner.
- Formation of Common Mycorrhizal Networks (CMNs): The extraradical hyphae possess the capability to colonize adjacent plants, leading to the establishment of CMNs. These networks act as channels for carbon and nutrient exchange between interconnected host plants. The incorporation of the rare isotope carbon-14 into a tree and its subsequent detection in neighboring plants exemplifies this interconnected nutrient flow.
- Ecological Implications of CMNs: CMNs are believed to influence a myriad of ecological processes, from seedling establishment and forest succession to intricate plant-plant interactions. Furthermore, certain mycorrhizal associations, such as arbuscular mycorrhizas, have been shown to relay signals across the network, warning plants of impending pest or pathogen threats.
4. Fruiting bodies
Ectomycorrhizal (EcM) fungi exhibit a unique reproductive strategy that markedly distinguishes them from their arbuscular mycorrhizal counterparts. Central to this reproductive process is the formation of conspicuous fruiting bodies, which play a pivotal role in the life cycle and ecological interactions of these fungi.
- Sporocarp Formation: The sporocarp, commonly referred to as the fruiting body, serves as an extension of the hyphal network emanating from the central fungal mass. This structure is integral to the sexual reproduction of EcM fungi, facilitating the generation and dispersal of spores.
- Biochemical Composition: Fruiting bodies are characterized by a rich biochemical profile. Notably, they possess high nitrogen content and are replete with complex carbohydrates, which are integral components of their cell walls and spores. This unique composition underscores their ecological significance and potential nutritional value.
- Mutualistic Interactions: The completion of the life cycle, encompassing the formation of fruiting bodies, often necessitates mutualistic interactions between different EcM fungi. This interdependence underscores the intricate web of relationships within the fungal community.
- Diversity of Fruiting Bodies: EcM fungi manifest a diverse array of fruiting bodies, ranging from epigeous mushrooms visible above the ground to hypogeous truffles concealed beneath the soil surface. These structures, despite their morphological differences, serve the common purpose of spore production and dispersal.
- Propagule Dispersal: Most fruiting bodies produce minute propagules, typically less than 10 micrometers in size. These propagules are adeptly adapted for long-distance dispersal, facilitated by various vectors, including wind currents and mycophagous animals that consume fungi.
- Ecological Significance of Hypogeous Fruiting Bodies: Hypogeous fruiting bodies, given their rich content of nitrogen, phosphate, minerals, and vitamins, are postulated to be attractive food sources for certain fauna. While the specific nutrient composition is undoubtedly a draw, some theories posit that the seasonal availability of these fruiting bodies might be a more crucial factor driving animal attraction.
- Challenges in Fruiting Body Surveys: While fruiting body surveys have been employed to gauge fungal diversity and abundance in specific locales, they present inherent challenges. The ephemeral nature of fruiting bodies, coupled with their often-cryptic presence, makes consistent detection a daunting task.
Morphology/Structure of Ectomycorrhiza
Ectomycorrhiza, often abbreviated as EcM, is a symbiotic association between specific fungi and plant roots. The structural components of this association are intricate and play a pivotal role in nutrient exchange and overall plant health. Here, we delve into the primary morphological features of ectomycorrhiza.
1. Hartig Net: The Hartig net is a network of intraradical hyphae that originates predominantly from the inner section of the mantle. These hyphae penetrate the plant root, establishing a connection between the outer cells of the root axis. This network grows perpendicularly to the root axis, facilitating the exchange of nutrients and carbon at the juncture where the fungal and plant cells meet. The depth of penetration varies among species. For instance, in plants like Eucalyptus and Alnus, the Hartig net is restricted to the epidermis. In contrast, in most gymnosperms, it extends deeper, reaching the cortical cells and occasionally the endodermis.
2. Mantle: Encasing the root is the mantle, a hyphal sheath that often possesses more biomass than the Hartig net. The mantle’s structure can range from a disorganized mesh of hyphae to a structured, layered tissue system. These layers, reminiscent of plant parenchyma tissue, are termed “pseudoparenchymatous.” The presence of the mantle often inhibits the growth of the root and suppresses the development of root hairs. The mantle’s characteristics, such as its color, branching pattern, and complexity, can be used in conjunction with molecular studies to identify the associated fungus.
3. Extraradical Hyphae and Linkage: Emerging from the mantle and extending into the soil are the extraradical hyphae. These hyphae can either grow individually or amalgamate to form structures known as rhizomorphs. Rhizomorphs can exhibit a variety of structures, from simple parallel hyphal arrangements to more complex configurations. These structures primarily serve as transport systems, often extending several meters, maintaining a vast surface area in contact with the soil. The unique structures and growth patterns of rhizomorphs can aid in identifying the symbiotic fungus.
4. Fruiting Bodies: Distinct from arbuscular mycorrhizal fungi, EcM fungi reproduce sexually, producing conspicuous fruiting bodies known as sporocarps. These fruiting bodies, which include familiar fungi forms like mushrooms and truffles, are rich in complex carbohydrates and nitrogen. Many EcM fungi require the presence of another EcM fungus to produce these fruiting bodies and complete their life cycles. These fruiting bodies serve as a means of reproduction, producing spores that can be dispersed by various vectors, including wind and animals.
Mechanism of Ectomycorrhiza
Ectomycorrhiza (EcM) is a specialized mycorrhizal association between certain fungi and plant roots. This symbiotic relationship involves a series of intricate mechanisms that facilitate nutrient exchange, protection, and mutual growth. Understanding the mechanisms of ectomycorrhiza is crucial for appreciating its ecological significance and potential applications. Here, we delve into the detailed processes involved in this fascinating symbiosis.
1. Presymbiosis:
Ectomycorrhiza begins with the fungus’ hyphae venturing into the soil, seeking out the plant’s roots to establish a symbiotic relationship. This initial phase involves several critical steps:
- Fungal Invasion: Fungal hyphae must penetrate the soil and make their way towards the plant roots.
- Root Cap Infection: To establish the symbiotic Hartig net and other associated structures, the fungus encases and infects the root cap cells.
- Gene Expression: Both the plant and the fungus follow a strict timetable of gene expression. Genes related to secretory processes, apical growth, and infection undergo changes during this pre-contact phase.
- Chemical Signaling: Chemical compounds secreted by the plant into the rhizosphere stimulate fungal growth and early stages of EcM formation.
2. Symbiosis:
Once the initial contact is made, the symbiosis progresses, leading to the formation of key structures and nutrient exchange:
- Mantle Formation: The fungal hyphae continue to grow inward from root cap cells towards the epidermal cells, creating layers that contribute to the formation of the mantle.
- Polypeptide and mRNA Synthesis: Rapid changes occur in polypeptide and mRNA synthesis following fungal invasion. Specific polypeptides, known as ectomycorrhizins, are produced only during this symbiotic phase.
- Mantle’s Role: The mantle regulates root growth, root hair production, and branching. Fungal exudates are studied as proxies for understanding the mantle’s functions.
- Hartig Net Formation: The Hartig net originates in the inner mantle layer and invades the root apoplast region. Some plant cells produce defensive proteins that can hinder Hartig net development, but the resistance often diminishes over time.
- Nutrient Uptake and Exchange: Nutrient exchange mechanisms involve nutrient flow through three interfaces: soil-fungus, fungus-apoplast, and apoplast-root cell. The fungal hyphae stimulate root cells, causing them to bulge outward, facilitating nutrient transfer between the two organisms.
3. Non-nutritional Benefits:
Ectomycorrhiza offers several non-nutritional benefits to plants, including:
- Water Transport: Extraradical hyphae, including rhizomorphs, can transport water, extending the plant’s effective watering zone.
- Protection: The hyphal sheath enveloping root tips serves as a physical barrier, protecting plant tissues from infections and predators.
- Biochemical Defense: Secondary metabolites produced by fungi act as biochemical defenses against pathogenic microorganisms that may attempt to infiltrate mycorrhizal roots.
- Tolerance to Stress: EcM fungi enhance plant tolerance to heavy metals, salts, radionuclides, and organic contaminants in soil, making them crucial for plants growing in challenging environments.
4. Ectendomycorrhiza:
In some cases, certain ectomycorrhizal fungi can behave either as typical ectomycorrhizas or as arbuscular mycorrhizas, penetrating the cortical cells of plant roots. These dual interactions are known as ectendomycorrhizas and highlight the flexibility of EcM fungi in forming diverse symbiotic relationships.
In summary, ectomycorrhiza involves a complex series of processes that benefit both the fungus and the host plant. From initial fungal invasion to nutrient exchange and non-nutritional advantages, this symbiotic association showcases the remarkable interplay between plants and fungi in terrestrial ecosystems.
Importance of Ectomycorrhiza
Ectomycorrhiza (EcM) plays a pivotal role in various ecological, environmental, and agricultural contexts. Their importance spans across multiple domains, from agriculture and forestry to pollution mitigation and climate change adaptation.
- Agricultural Implications: Modern agricultural practices, such as tilling, excessive fertilization, and fungicide application, inadvertently jeopardize the health of mycorrhizae and the broader ecosystem. Such practices can inadvertently alter local ectomycorrhizal communities, with repercussions like diminished sporocarp production due to heightened fertilization.
- Forestry and Tree Plantations: In commercial forestry, the transplantation of trees to novel regions often necessitates the concurrent introduction of an ectomycorrhizal counterpart. This is particularly pertinent for trees with specialized mycobiont requirements or those introduced to regions with unfamiliar fungal species. Obligate ectomycorrhizal trees, exemplified by species like Eucalyptus and Pinus, often necessitate the application of a local EcM fungal inoculum for optimal growth in plantations. While such plantations are occasionally proposed as climate change mitigation strategies, the potential soil carbon reduction by their associated ectomycorrhizal fungi remains a topic of debate.
- Ecosystem Restoration: Given the integral role of ectomycorrhizas in supporting host plants, there’s a burgeoning interest in leveraging EcM fungi for ecosystem restoration endeavors. The reintroduction of mycorrhizal fungi, following their loss from a habitat, emerges as a vital step in re-establishing vegetation and rejuvenating ecosystems.
- Heavy Metal Detoxification: Ectomycorrhizal fungi exhibit resilience to heavy metals, which are detrimental to most life forms. These fungi can colonize metal-contaminated soils and bind significant amounts of heavy metals. Intracellularly, these metals undergo various detoxification processes, from sequestration to complex formation. Moreover, ectomycorrhizal fungal fruiting bodies can act as concentrators of heavy metals.
- Pollution Mitigation and Phytoremediation: EcM fungi demonstrate beneficial effects in diverse contamination scenarios:
- Salinity: Certain EcM fungi enhance host plant survival in high soil salinity conditions.
- Radionuclides: Some ectomycorrhizal species, especially within the Cortinariaceae family, exhibit hyperaccumulation of radionuclides.
- Organic Pollutants: Specific EcM species can degrade persistent organic pollutants, detoxifying compounds like 2,4-dichlorophenol and tetrachloroethylene.
- Climate Change Adaptations: Ectomycorrhizal communities exhibit varied responses to the multifaceted challenges of climate change. Elevated CO2 levels can amplify fungal mycelium growth and root colonization in certain EcM associations. Temperature fluctuations elicit diverse responses, ranging from detrimental to beneficial. Notably, many EcM species bolster host plant resilience during droughts, enhancing root water uptake capabilities.
Examples of Ectomycorrhiza
Ectomycorrhizal (EcM) fungi predominantly belong to the Basidiomycota phylum and are integral to forest ecosystems. These fungi form symbiotic relationships with various tree species, facilitating nutrient exchange and enhancing tree health. Herein, we delve into some representative examples of ectomycorrhizal fungi:
- Amanita spp.: The Amanita genus is a quintessential example of ectomycorrhizal fungi. Notably, certain species within this genus, such as Amanita muscaria, exhibit a broad host range, forming symbiotic associations with over 20 different tree species. This non-specificity underscores the adaptability and versatility of Amanita muscaria in establishing mycorrhizal relationships with diverse tree hosts.
- Boletus spp.: Boletus represents another pivotal genus within the ectomycorrhizal fungi realm. While some species within this genus are non-specific in their host preferences, others, like Boletus elegans, display a high degree of specificity. Boletus elegans, for instance, predominantly associates with larch trees, highlighting the intricate and specialized nature of certain ectomycorrhizal partnerships.
- Tricholoma spp.: Tricholoma is yet another genus that contributes to the rich diversity of ectomycorrhizal fungi in woodland ecosystems. Species within this genus are known to form symbiotic relationships with various tree species, playing a pivotal role in nutrient cycling and soil health.
B. Endomycorrhiza
Endomycorrhiza represents a category of mycorrhizal associations characterized by the fungal component’s predominant internal localization within the root structure. This involves the fungal hyphae penetrating the host plant’s cortical cell walls, establishing a close and intricate relationship.
Two primary forms of endomycorrhiza are the arbuscular and ericoid mycorrhizae. However, other types, such as the dark septate endophytes, can also be classified under this category. A unifying feature across these forms is the hyphal penetration into the host cells, signifying a deep-rooted symbiotic relationship.
Remarkably, endomycorrhizae are associated with a vast majority of contemporary plant species, encompassing over 80% of them. This includes a wide range of plants from agricultural crops to greenhouse flora, such as vegetables, grasses, flowers, and fruit-bearing trees. The relationship is marked by the fungi’s formation of arbuscules and vesicles and its penetration into the cortical cells of the host. This internal exchange mechanism is complemented by the extension of the fungal hyphae outside the root, making it a more intimate association compared to ectomycorrhizae.
Further categorization of endomycorrhizae reveals specific types, including:
- Arbuscular Mycorrhizae: A widespread form, known for its arbuscules, which are tree-like structures formed within the plant cells.
- Ericaceous Mycorrhizae: Associated primarily with plants in the Ericaceae family.
- Arbutoid Mycorrhizae: Found in plants of the Arbutus genus and related species.
- Orchidaceous Mycorrhizae: Specific to orchids, aiding in their nutrient uptake.
Types/Classification of Endomycorrhiza
Endomycorrhizas are varied and have been further characterised as arbuscular, ericoid, arbutoid, monotropoid, and orchid mycorrhizas.
1. Arbuscular mycorrhiza
- Arbuscular mycorrhiza represents a specialized form of symbiotic relationship, primarily established between a select group of fungal species from the phylum Glomeromycota and a diverse array of vascular plant species. This association encompasses grasses, herbs, and notably, tropical tree species.
- The initiation of this symbiotic relationship is marked by the penetration of fungal hyphae into the plant’s epidermal cells. This infiltration is facilitated through a synergistic action of enzymatic processes and hydrostatic pressure. This process results in the formation of an appressorium, a hyphal swelling on the root’s exterior, where the pressure intensifies.
- Subsequently, the hyphae navigate through the cortical cell walls, displacing the plasma membrane in their path. This leads to the formation of intricate, tree-like structures known as arbuscules. These structures play a pivotal role in enhancing the contact surface between the fungal and plant cellular contents, thereby optimizing the exchange of nutrients and carbon between the two entities.
- Arbuscule development can be categorized into two primary types: the Arum and Paris forms. The Arum type is characterized by its multibranched hyphal formations that resemble trees, while the Paris type is marked by extensive hyphal coils within the host cells.
- A significant consequence of this fungal colonization is the suppression of root hair development. In its stead, extraradical hyphae, extending from the root’s exterior into the surrounding soil, assume the role of augmenting the root’s absorptive surface area.
- Certain fungal genera, with notable exceptions like Gigaspora and Scutellospora, may develop vesicles within the root tissue. These vesicles, which are lipid-staining, occupy the entirety of the host cell. Historically termed as vesicular-arbuscular mycorrhizae due to the presence of these vesicles, these structures are believed to serve as storage units for materials.
- Furthermore, the production of asexual spores can occur both externally and within the root. These spores, which vary in size and possess unique characteristics depending on the fungal species, are pivotal for species identification. Their dispersal mechanisms encompass air, water, and soil-dwelling organisms.
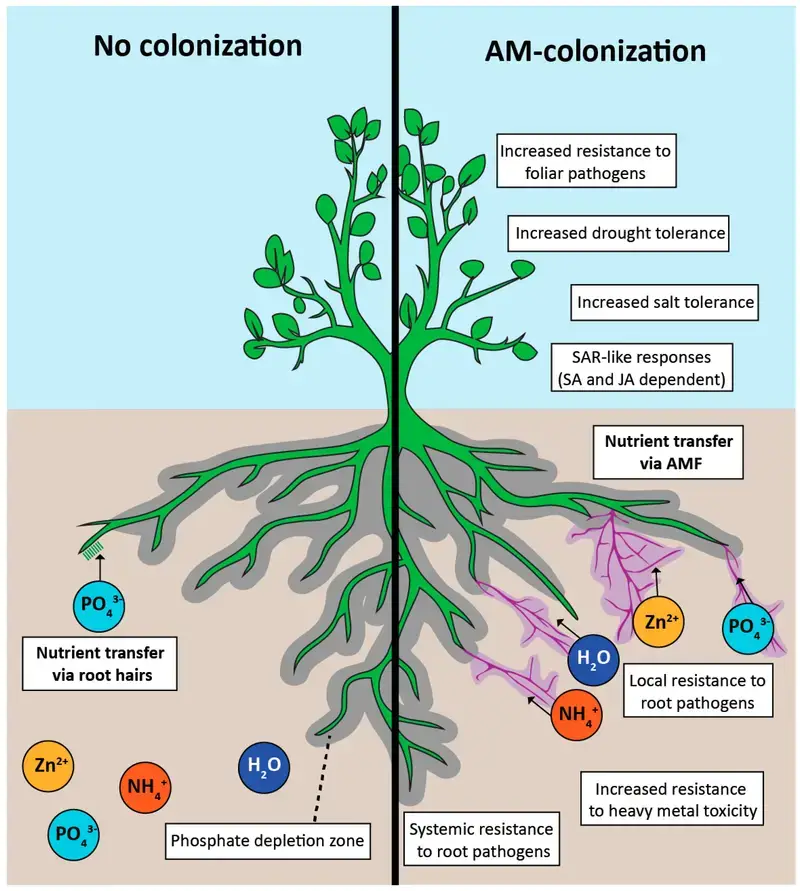
Vesicular Arbuscular Mycorrhizae (VAM)
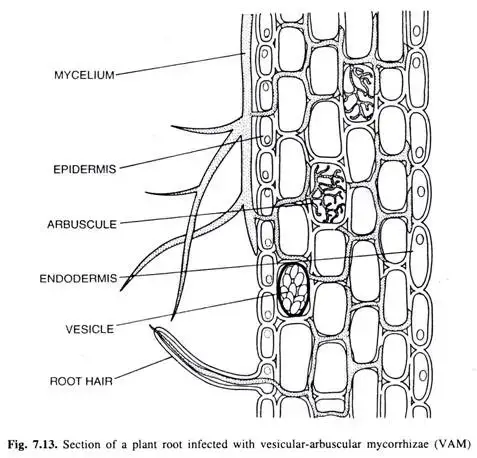
- Vesicular Arbuscular Mycorrhizae (VAM) represents a specialized form of symbiotic relationship predominantly observed in herbaceous angiosperms. Unlike ectotrophic mycorrhizae, VAM does not form a dense mantle around the roots, and its biomass is significantly less, constituting roughly 10% of the weight of the roots it colonizes.
- The colonization process of VAM is initiated as the mycelium infiltrates the host’s roots through root hairs and epidermal cells. As the hyphae navigate through the cortical cells, they form two distinct structures: vesicles and arbuscles. Vesicles are ellipsoid-shaped structures, while arbuscles exhibit a highly branched, tree-like morphology. These arbuscles are enveloped by the host’s plasma membrane, ensuring that only the cell walls are penetrated, leaving the protoplast untouched. As a result of VAM colonization, the cytoplasmic volume of the host’s cortical cells can increase by approximately 20-25%.
- The primary function of arbuscles is to amplify the contact area between the fungal hyphae and the host’s cortical cells, facilitating an efficient exchange of nutrients. Minerals can be transferred from the arbuscles to the host’s cortical cells either directly or through the disintegration of the arbuscles, releasing their contents.
- VAM derives its nomenclature from the presence of its hallmark structures: vesicles and arbuscles. Vesicles serve as storage units for polyphosphate and other minerals, while arbuscles, with their dichotomous branching, function as intracellular haustoria. After a span of about four days, arbuscles undergo lysis, liberating stored nutrients, predominantly in the form of polyphosphate.
- Externally, VAM lacks a fungal mantle but possesses a sparse network of septate hyphae that permeate the soil. These hyphae house a variety of spores, including chlamydospores, sporocarps, and zygospores. The superficial hyphae, with their penetrating branches, infiltrate the epidermis and primarily develop within the cortex.
- The evolution of this symbiotic relationship can be traced back to the early history of terrestrial plants. Evidence of VAM-like organisms has been identified in ancient plants like Rhynia and Asteroxylon. This ancient association underscores the pivotal role of mycorrhization in the evolution and sustenance of terrestrial plants.
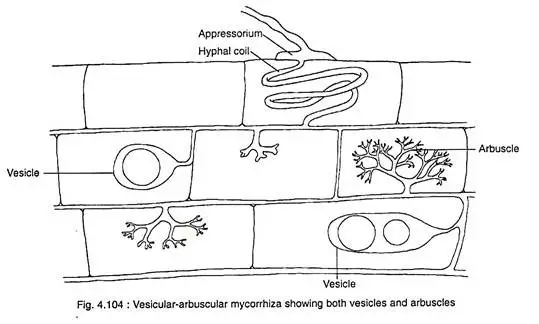
Mechanism of Arbuscular mycorrhiza
Arbuscular mycorrhiza (AM) represents a prominent mutualistic symbiosis between certain fungi and the roots of most terrestrial plants, encompassing a complex mechanism that involves presymbiosis, symbiosis, and nutrient uptake and exchange phases. Understanding the intricacies of AM is essential for elucidating its role in enhancing plant nutrition. Here, we delve into the scientific details of the mechanisms underlying AM symbiosis.
1. Presymbiosis:
The presymbiotic phase encompasses several crucial stages that lay the foundation for AM formation:
- Spore Germination: AM fungal spores, characterized by multinucleated structures with robust walls, can germinate independently of the host plant. However, host root exudates can expedite spore germination under favorable soil conditions.
- Hyphal Growth: The proliferation of AM hyphae is influenced by root exudates, particularly strigolactones, and soil phosphorus levels. Low phosphorus concentrations in soil stimulate hyphal development and branching, while high phosphorus levels can inhibit these processes.
- Host Recognition: Root exudates from AMF host plants, particularly those cultivated in phosphorus-deficient conditions, play a significant role in regulating hyphal development. These exudates guide the growth and branching intensity of AM fungal hyphae, enhancing their efficiency in seeking a suitable plant host.
- Appressorium Formation: When AM fungal hyphae contact the host plant’s root, an appressorium or ‘infection structure’ forms on the root’s epidermis. The appressorium allows hyphae to penetrate the root’s parenchyma cortex, initiating the symbiotic relationship.
2. Symbiosis:
The symbiotic phase of AM development involves the creation of arbuscules, highly branched structures within the plant root that facilitate nutrient exchange:
- Arbuscule Formation: Arbuscules are distinctive features of AM fungi, where essential nutrient exchanges occur. They come in two variations: Paris and Arum types, mainly determined by the host plant’s family. These structures serve as sites for phosphorus, carbon, water, and other nutrient exchanges.
- Host Regulation: The host plant actively regulates the growth of intercellular hyphae and arbuscule development. Cells containing arbuscules undergo significant changes, including organelle multiplication and cytoskeletal rearrangement.
- Other Hyphal Forms: Beyond arbuscules, AM fungi produce short-lived runner hyphae that extend into the soil for phosphate and micronutrient absorption. These hyphae have a higher surface-to-volume ratio than plant roots, enhancing nutrient absorption capacity. Additionally, AMF hyphae can access soil pores inaccessible to roots.
- Unique Morphologies: Four distinct forms of AMF hyphae exist, each with unique morphologies, enabling diverse functions within the symbiotic relationship.
3. Nutrient Uptake and Exchange:
Nutrient exchange and uptake are central to the AM symbiosis:
- Carbon Exchange: AM fungi depend on the host plant for their carbon source, absorbing hexoses produced by plant photosynthesis. Carbon transfer occurs through arbuscules or intraradical hyphae.
- Metabolic Pathways: Intraradical mycelium of AM fungi metabolizes hexoses into carbon storage forms like trehalose and glycogen. These compounds act as buffers for intracellular sugar concentrations.
- Lipid Production: Lipid production takes place within intraradical mycelium, and lipids are either stored or transported to extraradical hyphae. Gluconeogenesis, the conversion of lipids into hexoses, occurs in extraradical mycelium.
- Phosphorus Absorption: AMF hyphae can absorb phosphorus more efficiently than plant roots, owing to their larger surface area and enhanced transport capacity. Phosphorus can enter mycorrhizae up to six times faster than root hairs.
- Nitrogen Flow: AM symbiosis impacts nitrogen flow by affecting soil pH, enhancing solubility of phosphorus precipitates, and influencing ammonium ion absorption and release.
- Carbon Investment: Plants invest a substantial amount of carbon in the mycorrhizal network, enhancing phosphorus absorption and transfer from fungi to the plant.
- Phosphorus Absorption Variability: Different AMF species exhibit varying capacities to provide phosphorus to plants, with some acting as poor symbionts, consuming more carbon than they contribute in phosphorus.
Importance of Arbuscular mycorrhiza
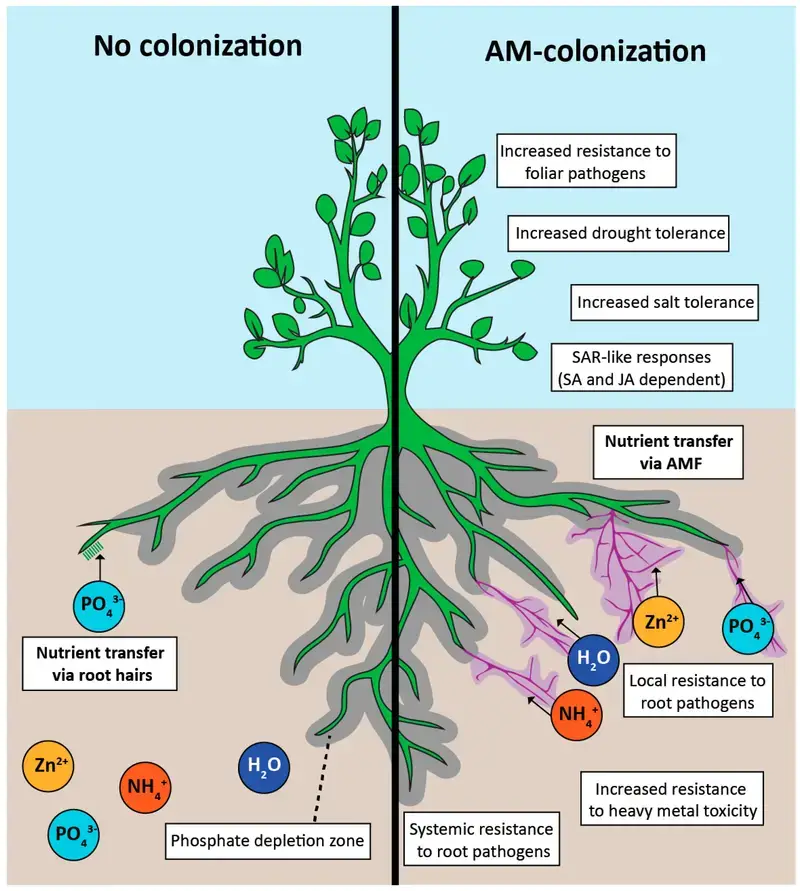
- Role in Phosphorus Uptake: Arbuscular mycorrhizal fungi (AMF) exhibit pronounced benefits in low-input systems. The presence of phosphate fertilizers can inhibit the colonization and growth of AMF. As soil phosphorus levels rise, plant tissue phosphorus content also increases, making the carbon expenditure on the AMF symbiosis less favorable for the plant. Interestingly, a decline in mycorrhizal colonization due to elevated soil phosphorus can lead to copper deficiency in plants, given that copper uptake is facilitated by mycorrhizae.
- Enhancement in Perennial Cropping Systems: Cover crops, cultivated during seasons when land typically remains barren, can bolster the inoculum potential and hyphal network of mycorrhizal fungi. Since AM fungi are biotrophic, their survival and growth are contingent on plants. By extending the growing season, cover crops promote the development of AMF, leading to a more expansive hyphal network. This network facilitates rapid colonization in the subsequent season, ensuring efficient phosphorus nutrition during the early growth stages, ultimately boosting crop yield.
- Augmentation of Soil Quality: Introducing native AM fungi can expedite the success of ecological restoration projects and hasten soil recovery. The aggregate stability of soil is enhanced by AM fungi due to the proliferation of extraradical hyphae and the production of a soil protein termed glomalin. Glomalin-related soil proteins (GRSP) have been identified using specific antibodies, underscoring their significance in soil health.
- Phytoremediation Potential: Inoculating soils with AM fungi prior to vegetation reintroduction in ecological restoration projects offers a novel approach to land rehabilitation. Such inoculation enables host plants to thrive in previously degraded soils, rejuvenating their quality. Soils inoculated with a consortium of indigenous AM fungi species exhibit marked long-term improvements in quality parameters compared to non-inoculated or singly inoculated soils. The presence of AM fungi enhances legume nodulation, leading to improved soil aeration, enhanced plant growth, and increased nutrient content. Notably, native AM fungi strains excel in extracting heavy metals from contaminated soils, rendering them suitable for agricultural use.
- Influence on Rhizosphere Ecology: The rhizosphere, the soil region surrounding plant roots, is profoundly impacted by arbuscular mycorrhizal symbiosis. The symbiosis affects the diversity and community structure of various soil species, either directly through exudate release or indirectly via alterations in the type and quantity of plant exudates.
- Implications in Global Climate Change: The dynamics of AM fungal communities and their interactions with plant hosts are susceptible to global climate change. While it’s acknowledged that organismal interactions will shape responses to climate change, predicting these outcomes remains challenging. Recent studies suggest that AM fungi can enhance plant biomass under drought conditions but may reduce it under simulated nitrogen deposition scenarios. Furthermore, AM fungi have demonstrated an increase in biomass in response to elevated atmospheric CO2 levels.
In conclusion, Arbuscular mycorrhiza plays a pivotal role in various ecological and agricultural contexts, from nutrient uptake and soil health to climate change implications. Their significance underscores the need for continued research and integration into sustainable agricultural practices.
Plants lacking arbuscular mycorrhizae
Mustard plants (Brassicaceae) including cabbage, cauliflower, canola, and crambe are unable to colonise their root systems with arbuscular mycorrhizal fungus.
3. Ericoid mycorrhizae
Ericoid mycorrhizae represent a unique form of symbiotic relationship predominantly observed in members of the Ericaceae plant family. This association is established with a select group of mycorrhizal fungi, tailored to thrive in specific environmental conditions.
- Habitat and Adaptation: Plants of the Ericaceae family, which form ericoid mycorrhizae, are typically native to acidic and nutrient-deprived soils. These environments include boggy terrains, heathlands, and boreal forests. The formation of ericoid mycorrhizae is a vital adaptation that enables these plants to survive and flourish under such challenging conditions. Historical molecular clock analyses suggest that this symbiotic relationship originated approximately 140 million years ago.
- Plant Families Involved: While the Ericaceae family is the primary participant in this symbiotic relationship, other plant families, including the Epacridaceae and Empetraceae, also engage in ericoid mycorrhizal associations. This indicates a specialized adaptation shared among these families to cope with similar environmental challenges.
- Fungal Partners: The fungal species Hymenoscyphus (Pezizella) ericae was initially identified as the primary endosymbiont forming ericoid mycorrhizae with plants of the Ericaceae family. However, subsequent research has unveiled the involvement of other fungal genera, such as Oidiodendron, Myxotrichium, and Gymnascella, in establishing mycorrhizal associations with ericoid plants.
- Morphological Characteristics: In terms of structural formation, ericoid mycorrhizae exhibit similarities with arbuscular mycorrhizae. The fungal hyphae penetrate the cortical cells of the host plants, particularly those of fine roots. Within these cells, the hyphae form intricate coils, akin to the arbuscules observed in arbuscular mycorrhizae. Notably, the fine roots of ericoid plants are characterized by a central vascular bundle surrounded by a singular layer of cortical or epidermal cells.
Structure and function of Ericoid mycorrhizae
Ericoid mycorrhizae represent a specialized form of symbiotic association predominantly observed in ericaceous plants. This relationship is characterized by distinct structural features and functional roles that facilitate nutrient exchange and survival in challenging environments.
- Structural Characteristics: The hallmark of ericoid mycorrhizae is the formation of fungal coils within the epidermal cells of the fine hair roots of ericaceous species. These coils are intricate structures formed by the fungal hyphae as they penetrate the walls of the plant’s cortical cells. While these hyphae form extensive networks around the exterior of the hair roots, they remain confined to the intercellular spaces, never breaching the plant cell’s plasma membranes. Over time, typically a few weeks, both the plant cell and the encompassed fungal hyphae undergo disintegration, marking the transient nature of these coils.
- Functional Role of Coils: The coils serve as the primary sites for nutrient exchange between the plant and the fungus. The plant provides the fungus with carbohydrates, products of photosynthesis, while in return, the fungus offers nutrients it has absorbed from the soil. This mutualistic exchange ensures the survival and growth of both partners.
- Enzymatic Capabilities: A significant attribute of ericoid mycorrhizal fungi is their enzymatic potential to break down complex organic compounds. While some evidence suggests that certain ericoid mycorrhizal fungi might exhibit saprophytic tendencies, leveraging these enzymes to derive nutrients from decaying organic matter, the primary role of these enzymes is believed to be mineral acquisition. Specifically, these enzymes enable the fungi to access organic forms of essential minerals, such as nitrogen. This capability is particularly crucial in the habitats of ericaceous plants, where mineralized forms of nutrients are scarce.
- Adaptation to Nutrient-Poor Environments: The ability of ericoid mycorrhizal fungi to tap into organic nutrient sources underscores their adaptive strategy. In the nutrient-poor environments typically inhabited by ericaceous plants, such as acidic and boggy soils, this symbiotic relationship becomes indispensable. The fungi’s enzymatic prowess complements the plants’ photosynthetic capabilities, ensuring a steady supply of essential nutrients for both partners.
Fungal symbionts of Ericoid mycorrhizae
Ericoid mycorrhizae, a specialized form of symbiotic association, is characterized by its interaction with a diverse range of fungal symbionts. These fungi play pivotal roles in the establishment and functioning of this symbiosis, especially in nutrient-poor environments where ericaceous plants predominantly thrive.
- Dominant Fungal Symbionts: Historically, much of the research on the physiology and function of ericoid mycorrhizal fungi has centered around isolates that were morphologically identified as Rhizoscyphus ericae. This fungus, belonging to the Ascomycota order Helotiales, has since been reclassified as a species of Pezoloma.
- Other Culturable Ascomycota: Apart from Rhizoscyphus ericae, several other culturable fungi from the Ascomycota phylum have been recognized as contributors to ericoid mycorrhizal formation. Notable among these are Meliniomyces, which shares a close phylogenetic relationship with Rhizoscyphus ericae, as well as Cairneyella variabilis, Gamarada debralockiae, and Oidiodendron maius.
- Diversity Revealed by DNA Sequencing: Modern molecular techniques, particularly DNA sequencing, have unveiled a broader spectrum of fungal communities associated with ericoid roots. However, it’s imperative to note that while these fungi are present, not all of them have been confirmed to form the characteristic ericoid mycorrhizal coils. Some might function as non-mycorrhizal endophytes, saprophytes, or even potential parasites, emphasizing the complexity of interactions within the ericoid mycorrhizal system.
- Basidiomycota Involvement: Beyond the realm of Ascomycota, fungi from the phylum Basidiomycota have also been identified as partners in ericoid mycorrhizal associations. Sebacina species, although not readily cultivable, are frequent inhabitants of ericoid roots and have demonstrated the capability to form ericoid mycorrhizas. Furthermore, certain basidiomycetes from the order Hymenochaetales have been implicated in the formation of this unique mycorrhizal type.
Economic significance of Ericoid mycorrhizae
- Several commercial and ornamental plant species, including blueberries, cranberries, and Rhododendron, have symbiotic relationships with ericoid mycorrhizal fungi.
- Inoculation with ericoid mycorrhizal fungus can affect plant development and nutrient absorption.
- However, ericoid mycorrhizal fungi have been the subject of significantly less agricultural and horticultural research than arbuscular and ectomycorrhizal fungi.
4. Orchid mycorrhiza
Orchid mycorrhizae represent a pinnacle of symbiotic relationships between plants and fungi, showcasing an intricate interdependence unparalleled in the realm of mycorrhizal interactions. With approximately 17,000 orchid species in existence, these plants heavily rely on their basidiomycete fungal counterparts for essential nutrient acquisition.
- Orchid-Fungal Interdependency: The degree of reliance between orchids and their fungal partners is profound. In certain orchid species, the symbiotic relationship is so deeply ingrained that the orchid seeds carry fungal propagules. This ensures that the fungus is immediately available during seed germination, facilitating nutrient uptake during the critical early stages of plant growth.
- Significance in Seed Germination: Given the diminutive size of orchid seeds, they possess limited nutrient reserves due to the absence of an endosperm. Consequently, the initial development of the protocorm post-germination is contingent upon the symbiotic fungus, not just for nutrient acquisition but also for carbohydrate provision. This symbiotic reliance persists until the orchid develops its photosynthetic capabilities—a process that can span up to a year in certain species. Remarkably, some achlorophyllous orchid species never achieve this photosynthetic capacity, rendering their dependence on the fungal partner perpetual.
- Fungal Structures: Arbuscules (Peletons): Within the cortical cells of the host orchid, the fungi form intricate, coiled structures known as arbuscules or peletons. These structures are transient in nature, and upon reaching the end of their lifespan, they leave behind deposits of cellulose and pectin within the host cell. Intriguingly, new fungal hyphae can subsequently invade these cells, tapping into the stored carbohydrates and nutrients.
5. Monotropoid mycorrhiza
Monotropoid mycorrhizae are specialized mycorrhizal associations predominantly found in the Monotropoideae subfamily within the Ericaceae family. These plants exhibit a unique adaptation, having largely lost their photosynthetic capabilities and existing as achlorophyllous plants on forest floors.
- Initial Misconceptions: Initially, plants associated with monotropoid mycorrhizae were believed to be parasitic on forest tree species. However, contemporary understanding reveals a more intricate relationship. These plants, rather than being direct parasites, leverage shared mycorrhizal symbionts common to neighboring trees.
- Carbohydrate Acquisition via Mycorrhizal Bridges: Monotropoid plants obtain essential carbohydrates from adjacent trees. This transfer is facilitated through mycorrhizal bridges, which connect the root systems of the monotropoid plants and the neighboring trees. This intricate network ensures a continuous supply of photosynthates to the achlorophyllous plants.
- Fungal Partnerships: Several fungal species, including Tricholoma, Russula, and Rhizopogon, have been identified as partners in these mycorrhizal associations. On their monotropoid hosts, these fungi exhibit characteristics similar to ectomycorrhizal associations on trees. They form fungal sheaths and Hartig nets, which are typical of ectomycorrhizal relationships. Additionally, these fungi produce unique structures called hyphal pegs, which originate from the inner sheath layer of hyphae and penetrate the tangential wall of the host’s cortical cells. Each cortical cell typically houses a single peg. The formation of these pegs is closely tied to the host plant’s growth and development, peaking during the flowering stage and subsequently diminishing.
- Nutrient Transfer and Acquisition: The pioneering work of Bjo¨rkman in the 1960s, utilizing radiotracers, provided evidence for the transfer of photosynthates from trees to Monotropa plants. Further research corroborated that this transfer is mediated through mycorrhizal bridges formed by shared fungal hyphae. While the exact mechanisms of nutrient acquisition by monotropoid mycorrhizae remain to be fully elucidated, it is postulated that the process mirrors that of ectomycorrhizal associations.
Other Mycorrhizae
Arbutoid (Ectendomycorrhizae)
Arbutoid mycorrhizae, also referred to as ectendomycorrhizae, represent a distinct type of mycorrhizal association predominantly observed between specific members of the Ericaceae family, namely Arbutus and Arctostaphylos, and several genera within the Pyrolaceae family.
- Fungal Partnerships: A variety of ectomycorrhizal fungal species have been identified to establish these arbutoid associations. These fungi play a pivotal role in facilitating nutrient exchange and enhancing the overall health and growth of their host plants.
- Structural Characteristics: The hallmark of arbutoid mycorrhizae is the formation of a delicate fungal sheath that envelops the exterior of the root. This is accompanied by the development of a paraepidermal Hartig net, which is characterized by fungal penetration between the plant’s epidermis. Furthermore, the outermost layer of cortical cells witnesses the invasion of fungal hyphae, leading to the formation of intricate hyphal coils, also known as intracellular hyphal complexes.
- Nutrient and Photosynthate Exchange: One of the primary functions of arbutoid mycorrhizae is to facilitate the exchange of essential nutrients and photosynthates. It is postulated that arbutoid plants may engage in nutrient and photosynthate exchange with neighboring tree species, further emphasizing the interconnectedness and mutualistic nature of these symbiotic relationships.
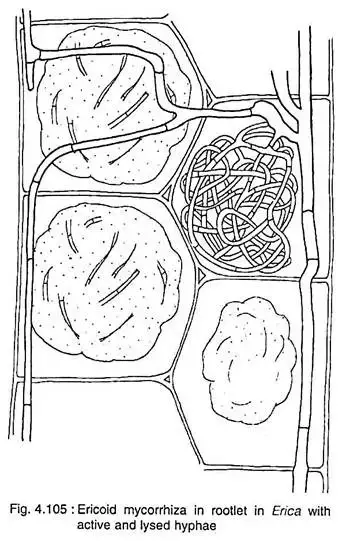
Dark septate endophytes
Dark septate endophytes (DSE) represent a group of root endophytes that have been detected in a myriad of plant species spanning almost all plant families. Their prevalence is particularly notable in environments characterized by cool temperatures and limited nutrient availability.
- Taxonomic Diversity: While a significant proportion of these fungi remain unidentified, certain genera such as Chloridium, Leptodontidium, Phialocephala, and Phialophora have been recognized in plant roots. This highlights the vast taxonomic diversity and potential ecological roles of DSE.
- Structural Adaptations: DSE fungi typically gain entry into the plant root system either through root hairs or cortical cells. Once inside, they form runner hyphae that navigate between the cortical cells. A distinctive feature of these fungi is the formation of microsclerotia, which are densely branched structures that establish themselves within the host cell. The initial hyaline hyphae of these fungi often undergo melanin deposition in their cell walls, resulting in a dark hue, which is the origin of their nomenclature.
- Functional Significance: The exact functional role of DSE remains an area of ongoing research. However, their pronounced presence in oligotrophic (nutrient-poor) and climatically challenging environments suggests a potential role in nutrient acquisition. It is postulated that DSE may possess specialized enzymatic capabilities that enable them to extract nutrients from inorganic sources, thereby benefiting their host plants in nutrient-scarce settings.
Function of Mycorrhiza/Mycorrhizal Function
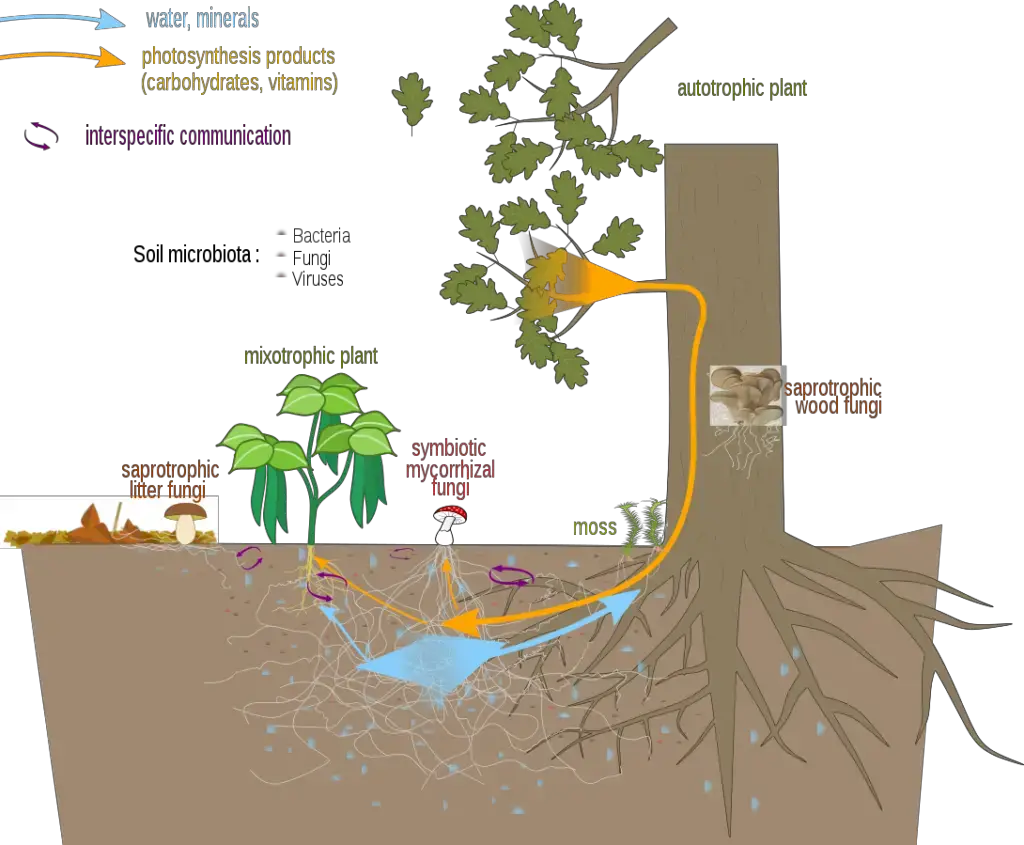
Mycorrhizae, symbiotic associations between fungi and plant roots, play a pivotal role in plant health and productivity. Their functions, however, extend beyond the traditionally understood nutrient uptake enhancement.
- Nutrient Acquisition: Central to the mycorrhizal function is the enhancement of inorganic nutrient uptake from the soil. This is evident from numerous studies indicating superior growth in plants with mycorrhizal associations compared to those without. A landmark study by Nye and Tinker, employing radioactive phosphate, illuminated the superior phosphate uptake capability of mycorrhizal roots. The extensive hyphal network, with its vast exploratory capacity, facilitates efficient nutrient uptake, especially phosphorus. This nutrient acquisition mechanism is not only energy-efficient but also genetically regulated, with specific proteins and transporters playing crucial roles.
- Carbon Exchange: Mycorrhizae receive carbon in the form of photosynthates from the host plant. This exchange is facilitated by the upregulation of hexose importer genes in both the plant and the fungus. This carbon exchange not only fuels fungal growth but also fortifies the mycorrhizal association against potential threats.
- Water Acquisition: Mycorrhizal hyphae and rhizomorphs possess the ability to translocate water, a function particularly vital in arid environments. The efficiency of this process, however, is contingent on the plant species and environmental conditions.
- Plant Defense Mechanisms: Mycorrhizae confer a dual-layered defense to plants. Firstly, the physical barrier created by the ectomycorrhizal sheath offers protection against soil-borne pathogens and grazers. Secondly, the biochemical defense, mediated by fungal secondary metabolites, shields the plant against a spectrum of pathogens. This protective function is evident in agricultural settings where mycorrhizal associations mitigate the deleterious effects of pathogens, enhancing plant growth and yield.
- Disease Prevention: Mycorrhizae play a pivotal role in disease prevention, especially in high-density agricultural settings conducive to pathogen proliferation. Their protective role extends to both fungal pathogens and nematodes. However, the extent of this defense in natural ecosystems remains an area of active research.
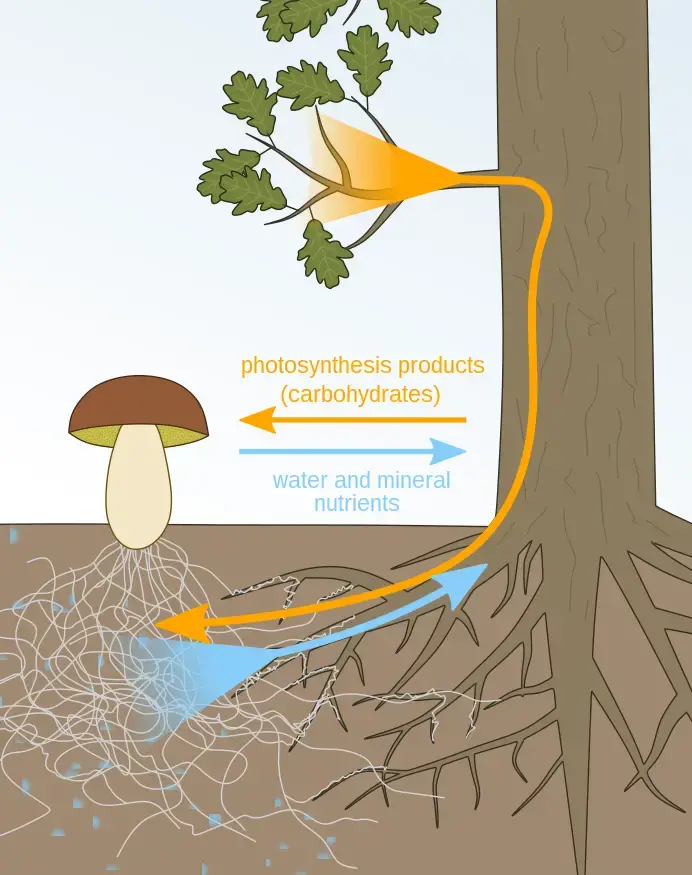
Ecology of Mycorrhizae
Mycorrhizae, symbiotic associations between fungi and plant roots, play a pivotal role in the ecological dynamics of various ecosystems. Their influence spans from nutrient cycling to plant community structuring and even extends to the food web.
- Global Distribution and Soil Nutrient Dynamics: The distribution of mycorrhizal types is intrinsically linked to the global distribution of their respective host plants. However, as postulated by Read, the relationship between mycorrhizal types and soil factors is more profound. In regions characterized by colder climates or higher altitudes, organic matter accumulation is prevalent. Here, nutrient reserves might be abundant, but their bioavailability is limited. Mycorrhizal types, like ericoid mycorrhizae, in these regions possess enzymes that allow them to access organic nitrogen forms directly. In contrast, in temperate regions, ectomycorrhizal communities can access both organic nitrogen and phosphorus. In tropical regions, where nutrient mineralization is rapid, the mycorrhizal community predominantly consists of arbuscular mycorrhizae with limited enzymatic capabilities.
- Influence on Plant Communities: Mycorrhizae play a crucial role in shaping plant communities. The ability of a plant to form mycorrhizal associations and its dependency on these associations can influence its competitive advantage within a community. Bever and Schultz (2005) highlighted that the interplay between plant-mycorrhizal strategies can lead to niche partitioning, thereby maintaining diversity within plant communities. The diversity of the mycorrhizal community often mirrors that of plant species diversity. Moreover, mycorrhizal bridges between plants can facilitate resource sharing, leading to both competitive and synergistic interactions that challenge traditional plant community theories.
- Mycorrhizae as a Food Source: Mycorrhizal fungi, particularly their fruiting bodies, serve as a rich nutrient source for various fauna. Ectomycorrhizal fungi, such as mushrooms, are consumed by invertebrates and small mammals. In Australia, for instance, fungi constitute a significant portion of the diet for several mammalian species. These fungi, especially the hypogeous ones, are nutrient-dense, making them a valuable food resource. Furthermore, the role of small mammals in dispersing fungal spores has been observed to aid in vegetation recovery in areas affected by natural disasters, such as volcanic eruptions. Below the ground, mycorrhizal mycelia serve as food for soil fauna, including nematodes and springtails. However, the impact of soil fauna grazing on mycorrhizal efficiency remains a topic of debate.
Mycorrhizae and Plant Production
Mycorrhizal associations, the symbiotic relationships between fungi and plant roots, have garnered considerable attention for their potential in various fields, including agriculture, commercial forestry, and ecosystem restoration. These associations offer several advantages, from improved plant nutrition to protection against pathogens and stressors. Here, we delve into their applications in these sectors:
- Agriculture: In agricultural settings, the growth-enhancing properties of mycorrhizae have been extensively explored. However, their impact can vary due to the widespread use of fertilizers and the presence of native fungal propagules in the soil. Mycorrhizal associations can enhance plant nutrition by increasing nutrient uptake. Additionally, they provide protective benefits, safeguarding crops against root-feeding nematodes and various bacterial and fungal pathogens. Research has also focused on optimizing methods for mycorrhizal delivery, including incorporating spores into pellets or producing them in matrix materials like rockwool and clay-brick granules. Several companies have developed commercial mycorrhizal inoculum products for use by gardeners, horticulturalists, and forest nurseries.
- Commercial Forestry: The use of mycorrhizal inoculation in commercial forestry has gained attention due to its potential to enhance tree seedling growth. Ectomycorrhizal and arbuscular mycorrhizal inocula are commercially available and are often delivered in perlite-peat mixtures or alginate-entrapped mycelial cultures. These inocula have demonstrated growth benefits in nursery settings and have contributed to the survival and growth of seedlings in challenging environments. For example, ectomycorrhizal fungi like Pisolithus tinctorius have facilitated tree seedling growth on heavy metal-contaminated mine spoils. However, the extent of growth enhancement varies depending on tree species and location, with outplanted seedlings sometimes facing competition from native mycorrhizal flora.
- Restoration: Mycorrhizal associations play a pivotal role in ecosystem restoration projects. They aid in establishing vegetation on nutrient-poor soils typically found in restoration sites. This facilitates the return of organic matter to the soil, improving its health. Moreover, mycorrhizal fungi contribute to soil stability by physically binding soil mineral particles or producing substances like glomalin, which prevent soil erosion. These actions increase soil aggregation and carbon storage within soil aggregates, potentially serving as a means of long-term carbon sequestration. In regions with saline soils resulting from frequent crop irrigation, arbuscular mycorrhizae have been instrumental in enabling crop growth under stressful conditions, allowing continued agricultural production in otherwise challenging areas.
Mycorrhizae and Pollution
Mycorrhizal associations, crucial for plant nutrient uptake and ecosystem health, can be profoundly influenced by various types of pollution. Here, we delve into how mycorrhizae respond to different pollutants, including acidifying substances, heavy metals, radionuclides, organic pollutants, and the impacts of climate change.
- Acidifying Pollutants: Research on acid rain in the late 1970s revealed its detrimental effects on mycorrhizae. Acid rain, primarily composed of sulfuric acid from dissolved SO2 in rain, can affect root growth and alter the ectomycorrhizal status of trees. Acid rain’s impact on mycorrhizae is twofold: (1) It reduces photosynthesis in the tree canopy, diminishing energy supply to roots, and (2) it increases the availability of toxic metal ions (e.g., aluminum, manganese, magnesium) in soil, leading to root damage and changes in the composition of ectomycorrhizal fungal symbionts. These effects disrupt mycorrhizal formation and the associated benefits, such as enhanced nutrient uptake.
- Heavy Metal Pollutants: Heavy metals are toxic to many organisms, including fungi. However, mycorrhizal fungi, especially ectomycorrhizae, exhibit tolerance to heavy metals. Studies have shown that mycorrhizal inoculation can enhance tree survival and growth in polluted environments, with certain fungi like Pisolithus tinctorius reducing the uptake of heavy metals by host trees. The mechanisms behind this protection involve adsorption of heavy metals onto fungal hyphae, preventing their translocation into the host plant’s vascular tissue.
- Radionuclide Pollutants: Mycorrhizal fungi, particularly ectomycorrhizae, have demonstrated an ability to accumulate radionuclides. Research following the Chernobyl nuclear disaster revealed that certain fungi had ten times the concentration of radiocesium compared to surrounding soils. This accumulation is attributed to internal translocation within the mycelium, followed by directional transport to fruiting bodies. Mycorrhizae can enhance radionuclide uptake by host plants while preventing their translocation to shoots, serving as a protective mechanism.
- Organic Pollutants: Mycorrhizal fungi have shown potential in decomposing organic pollutants, such as polychlorinated biphenyls (PCBs). Several mycorrhizal species have been found to degrade PCBs to varying degrees. While the interactions between mycorrhizal fungi, rhizospheric bacteria, and organic pollutant decomposition are still emerging fields of study, there is evidence of fungal involvement in mitigating organic pollution.
- Climate Change: Climate change factors, including increased atmospheric CO2 levels, rising temperatures, and altered rainfall patterns, can influence mycorrhizal communities. Elevated CO2 levels impact the C:nutrient ratio of plant material entering decomposition pathways, altering nutrient availability and affecting mycorrhizal community composition. Research on the responses of mycorrhizae to climate change-related factors, such as ozone, UV light, and altered rainfall, is ongoing.
- Fungal Conservation: Long-term fungal records have revealed shifts in fungal populations, with ectomycorrhizal species declining in dominance. This change has been attributed to acidifying pollutants, particularly nitrogen deposition. The decline in fungal species has raised concerns about fungal conservation, prompting efforts to maintain habitats for declining fungal species and assess the impact of mushroom harvesting on fungal decline. Forest management practices may also influence the composition of ectomycorrhizal fungal flora.
Benefits of Mycorrhiza
Mycorrhiza, the symbiotic association between fungi and plant roots, offers a wide range of benefits to both plants and ecosystems. These benefits are crucial for nutrient uptake, plant health, and overall ecosystem functioning. Here are some of the key advantages of mycorrhizal associations:
- Improved Nutrient Uptake:
- Mycorrhizal fungi extend their hyphae into the soil, increasing the root’s capacity to absorb essential nutrients such as phosphorus, nitrogen, and micronutrients.
- They can access nutrient sources that are otherwise unavailable to plants, enhancing nutrient acquisition efficiency.
- Enhanced Water Uptake:
- Mycorrhizal networks can extend deep into the soil, accessing water in deeper layers during drought conditions, which helps plants maintain adequate hydration.
- Disease Resistance:
- Mycorrhizal associations can enhance a plant’s resistance to soil-borne pathogens by forming physical barriers between pathogens and roots.
- Some mycorrhizal fungi also produce antimicrobial compounds that inhibit the growth of harmful microorganisms.
- Improved Plant Growth:
- Mycorrhizal fungi stimulate plant growth by facilitating nutrient uptake and increasing the availability of water.
- Enhanced growth can lead to improved crop yields in agriculture and healthier, more robust plants in natural ecosystems.
- Stress Tolerance:
- Mycorrhizal plants are often more resilient to environmental stressors, such as drought, salinity, and heavy metal contamination, due to their improved nutrient and water uptake capabilities.
- Ecosystem Restoration:
- Mycorrhizal fungi play a critical role in the restoration of degraded ecosystems. They help establish vegetation on poor soils, stabilize soil structure, and sequester carbon.
- Increased Biodiversity:
- Mycorrhizal associations are associated with greater plant diversity, as they enable a wider range of plant species to thrive by accessing limited soil resources.
- Carbon Sequestration:
- Mycorrhizal fungi store carbon in soil organic matter, contributing to carbon sequestration, which is essential for mitigating climate change.
- Support for Non-Photosynthetic Plants:
- Mycorrhizal networks can connect different plant species, allowing non-photosynthetic plants (mycoheterotrophs) to obtain carbon and nutrients from nearby photosynthetic plants.
- Improved Soil Structure:
- Mycorrhizal fungi produce extracellular substances like glomalin, which help bind soil particles together, enhancing soil structure, and preventing erosion.
- Increased Ecosystem Productivity:
- Mycorrhizal associations contribute to overall ecosystem productivity by promoting the growth of plants, which, in turn, support higher trophic levels.
- Reduced Fertilizer Dependency:
- In agriculture, mycorrhizal associations can reduce the need for synthetic fertilizers by enhancing nutrient uptake efficiency, potentially reducing production costs and environmental impacts.
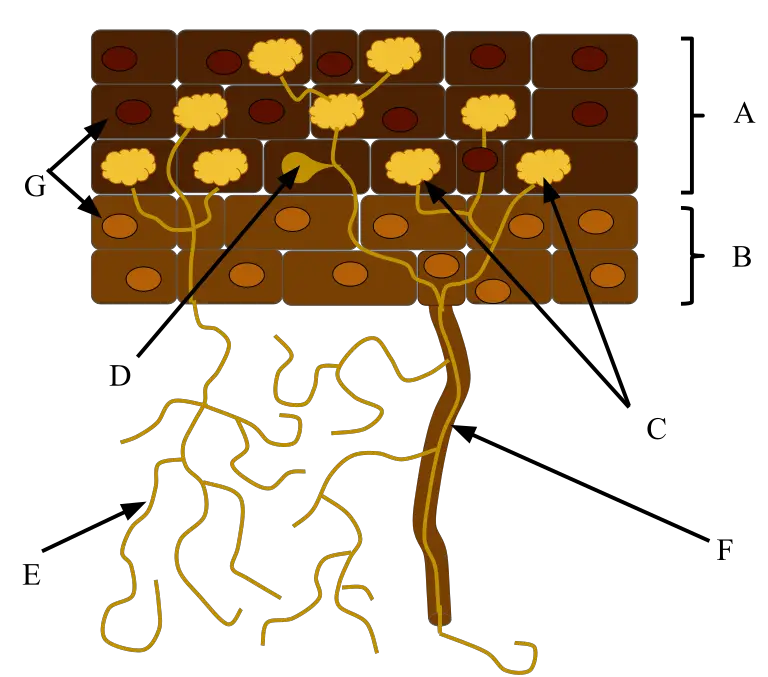
Examples of Mycorrhiza
Mycorrhiza, the symbiotic associations between fungi and plant roots, exhibit various types and are found in a range of plant species across diverse ecosystems. These associations play critical roles in nutrient uptake, plant health, and ecosystem functioning. Here are some examples of mycorrhiza:
- Orchid Mycorrhiza:
- Orchids, particularly those that are non-photosynthetic or have limited photosynthesis during their early growth stages, form mycorrhizal associations with specific fungi.
- Orchid seeds depend on fungal invasion for germination, as they cannot acquire sufficient nutrients independently.
- Orchidaceous mycorrhiza involves the formation of hyphal coils (pelotons) within the root cells, facilitating nutrient exchange between the fungus and the orchid.
- Arbuscular Mycorrhiza:
- Arbuscular mycorrhizae are among the most widespread mycorrhizal associations, commonly found in many plant species.
- Fungi involved in arbuscular mycorrhiza are typically members of the Zygomycota family.
- These fungi form arbuscules, which are intricate structures within root cells that serve as sites for nutrient exchange, particularly for phosphorus, carbon, and water.
- Arbuscular mycorrhizae are considered obligate symbionts, as the fungi cannot thrive without their plant hosts.
- Ericaceous Mycorrhiza:
- Ericaceous mycorrhizae are found in plants belonging to the Ericales order, often in acidic environments.
- These mycorrhizae help regulate the plant’s acquisition of minerals such as iron, manganese, and aluminum.
- While they do penetrate root cells, they do not form arbuscules. Instead, they create hyphal coils outside of root cells, significantly increasing root volume.
- Arbutoid Mycorrhiza:
- Arbutoid mycorrhiza is a type of endomycorrhizal association that resembles ectomycorrhizal fungi in appearance.
- Fungi involved in this association form a fungal sheath around plant roots and penetrate cortical cells.
- This differs from ectomycorrhizal fungi, which do not penetrate root cells.
- Ectotrophic Mycorrhiza:
- Ectotrophic mycorrhizae are formed between fungi belonging to the Ascomycota and Basidiomycota families and many trees in cooler environments.
- Unlike wood-rotting fungi from the same families, ectotrophic mycorrhizal fungi do not degrade plant materials.
- Instead, they derive nutrients and sugars from the living roots of their host plants.
Mycorrhiza Lecture Video
Quiz
What is mycorrhiza?
A. A type of plant disease
B. A symbiotic association between fungi and plant roots
C. A mineral-rich soil
D. A type of plant hormone
Which part of the plant is primarily involved in mycorrhizal associations?
A. Leaves
B. Stems
C. Roots
D. Flowers
What is the main benefit of mycorrhizal associations for plants?
A. Increased water absorption
B. Enhanced photosynthesis
C. Improved disease resistance
D. Enhanced nutrient uptake
Which type of mycorrhiza is known for its high affinity for phosphorus?
A. Arbuscular mycorrhiza
B. Ectotrophic mycorrhiza
C. Ericaceous mycorrhiza
D. Orchid mycorrhiza
What do arbuscules represent in arbuscular mycorrhizal associations?
A. Fungal spores
B. Root hairs
C. Sites of nutrient exchange
D. Protective structures
Which of the following mycorrhizal types is commonly found in acidic environments?
A. Ectotrophic mycorrhiza
B. Arbutoid mycorrhiza
C. Orchid mycorrhiza
D. Ericaceous mycorrhiza
What is the primary role of ericaceous mycorrhiza in plant nutrition?
A. Enhancing photosynthesis
B. Regulating water uptake
C. Facilitating mineral absorption
D. Promoting root growth
In which type of mycorrhizal association do fungi form a sheath around plant roots?
A. Ectotrophic mycorrhiza
B. Arbuscular mycorrhiza
C. Orchid mycorrhiza
D. Ericaceous mycorrhiza
Which fungal families are typically involved in ectotrophic mycorrhizal associations?
A. Zygomycota
B. Ascomycota and Basidiomycota
C. Glomeromycota
D. Chytridiomycota
What is the ecological significance of mycorrhizal associations?
A. They promote fungal diseases in plants
B. They reduce plant growth and productivity
C. They contribute to nutrient cycling and ecosystem health
D. They have no impact on ecosystems
FAQ
What is mycorrhiza?
Answer: Mycorrhiza is a symbiotic association between fungi and the roots of plants. It involves a mutually beneficial exchange of nutrients between the two organisms.
What is the role of mycorrhizal fungi in plants?
Answer: Mycorrhizal fungi help plants by enhancing their nutrient and water uptake capabilities. They also improve the plant’s resistance to diseases and environmental stress.
How does the symbiosis between mycorrhizal fungi and plants work?
Answer: Mycorrhizal fungi form a network of fine threads (hyphae) that extend into the soil, increasing the root’s surface area. This allows the plant to access more nutrients and water, while the fungi receive sugars produced by the plant through photosynthesis.
Are mycorrhizal associations beneficial for all plants?
Answer: Mycorrhizal associations are beneficial for most plants, especially in nutrient-poor soils. However, some plants have evolved to grow without mycorrhizal partners.
What are the different types of mycorrhizae?
Answer: There are several types of mycorrhizae, including arbuscular mycorrhizae, ectotrophic mycorrhizae, ericaceous mycorrhizae, and orchid mycorrhizae, each forming unique associations with plants.
Can mycorrhizal fungi protect plants from diseases?
Answer: Yes, mycorrhizal fungi can enhance a plant’s resistance to diseases by competing with harmful pathogens for resources and producing antimicrobial compounds.
How do I know if my soil has mycorrhizal fungi?
Answer: Mycorrhizal fungi are present in most soils. You can encourage their growth by maintaining healthy, diverse plant communities and avoiding excessive use of synthetic fertilizers.
Can mycorrhizal fungi be used in agriculture?
Answer: Yes, mycorrhizal inoculants are used in agriculture to improve crop yields and reduce the need for synthetic fertilizers. They can be applied to the roots of plants during transplantation.
Do mycorrhizal associations have an impact on ecosystems?
Answer: Yes, mycorrhizal associations play a crucial role in nutrient cycling, soil health, and plant diversity within ecosystems. They contribute to the overall ecological balance.
Can mycorrhizae help mitigate climate change?
Answer: Mycorrhizal fungi can assist in carbon sequestration by improving soil structure and storing carbon in soil aggregates. This has the potential to contribute to climate change mitigation efforts.
References
- Dighton, J. (2009). Mycorrhizae. Encyclopedia of Microbiology, 153–162. doi:10.1016/b978-012373944-5.00327-8
- Egerton-Warburton, L. M., Querejeta, J. I., Allen, M. F., & Finkelman, S. L. (2005). MYCORRHIZAL FUNGI. Encyclopedia of Soils in the Environment, 533–542. doi:10.1016/b0-12-348530-4/00455-0
- Egerton-Warburton, L. M., Querejeta, J. I., Allen, M. F., & Finkelman, S. L. (2013). Mycorrhizal Fungi. Reference Module in Earth Systems and Environmental Sciences. doi:10.1016/b978-0-12-409548-9.05226-x
- Bonfante, Paola & Anca, Iulia-Andra. (2009). Plants, Mycorrhizal Fungi, and Bacteria: A Network of Interactions. Annual review of microbiology. 63. 363-83. 10.1146/annurev.micro.091208.073504.
- Devi, S. H. , Bhupenchandra, I., Sinyorita, S., Chongtham, S., & Devi, E. L. (2021). Mycorrhizal Fungi and Sustainable Agriculture. In T. Ohyama, & K. Inubushi (Eds.), Nitrogen in Agriculture – Physiological, Agricultural and Ecological Aspects. IntechOpen. https://doi.org/10.5772/intechopen.99262
- https://people.unil.ch/iansanders/mycorrhizas/
- https://collegedunia.com/exams/mycorrhiza-biology-articleid-1551
- https://soils.ifas.ufl.edu/soil-microbial-ecology/mycorrhizal-services/
- https://www.gardenorganic.org.uk/mycorrhizal-fungi
- https://biologydictionary.net/mycorrhizae/
- https://mycorrhizae.com/how-it-works/
- https://www.groundworkbioag.com/mycorrhiza/
- https://www2.nau.edu/~gaud/bio300/mycorrhizae.htm
- https://untamedscience.com/biology/ecology/mycorrhizae/
- https://arboretum.harvard.edu/stories/food-poison-and-espionage-mycorrhizal-networks-in-action/
- https://www.spun.earth/networks/mycorrhizal-fungi
- https://en.wikipedia.org/wiki/Mycorrhiza#:~:text=A%20mycorrhiza%20is%20a%20symbiotic,phosphorus%2C%20taken%20from%20the%20soil.