Contents
What is Gene Interactions?
- Gene interactions, a fundamental concept in genetics, refer to the intricate relationships between genes that collectively influence the phenotype of an organism. This process is characterized by the mutual influence of two or more genes on the expression of a single trait. Contrary to the initial assumptions of early geneticists like Mendel, the expression of most traits in living organisms is not determined by a singular gene but is instead orchestrated by multiple genes.
- Historically, Mendel’s pioneering work on inheritance postulated that individual traits were governed by single genes. However, subsequent research revealed that many traits are influenced by two or more genes, leading to the realization that inheritance patterns are more complex than initially believed. These genes can modify the expected dihybrid (9:3:3:1) or trihybrid (27:9:9:9:3:3:3:1) ratios, thereby affecting the manifestation of the associated traits in diverse ways.
- At the core of gene interactions is the principle that the expression of one gene can be modulated by the presence or absence of another gene’s expression. This interplay between genes is what gives rise to the vast diversity of phenotypic traits observed in nature.
- The significance of gene interactions was further underscored following the rediscovery of Gregor Mendel’s work on inheritance. While Mendel’s observations laid the foundation for the field of genetics, it became evident that inheritance is not merely a linear one-gene, one-trait relationship. Instead, it encompasses the interactions of multiple genes, and the cumulative effects of these interactions cannot be deduced by examining each gene in isolation.
- Building on Mendel’s findings, Bateson and Punnett conducted breeding experiments with chickens. Their research offered early indications that multiple genes could influence traits. They observed that the inheritance patterns of certain traits deviated from the expected Mendelian ratios, signifying the interaction of genes.
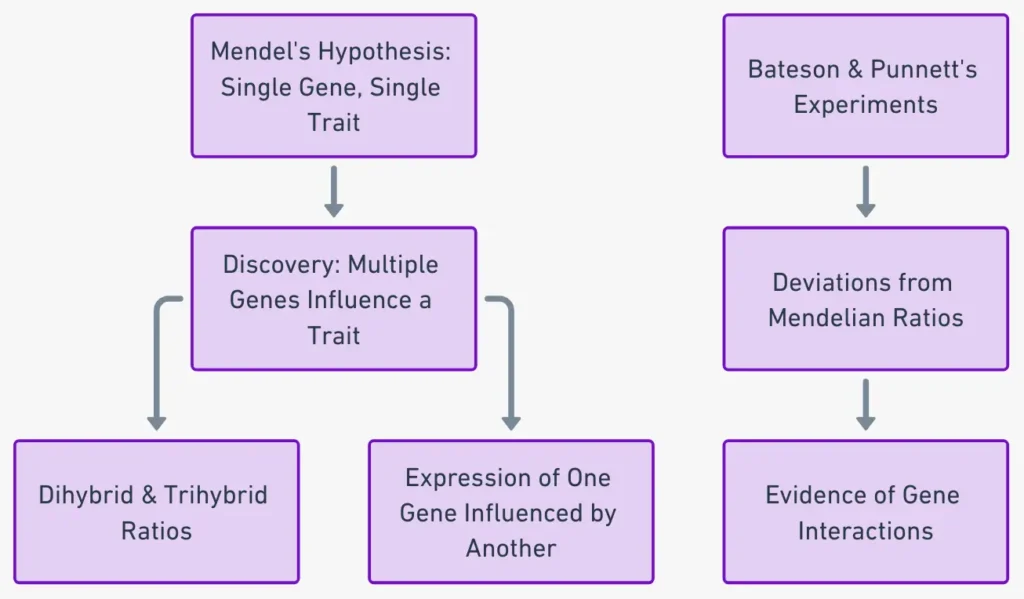
Definition of Gene Interactions
Gene interactions refer to the relationships between multiple genes where the expression of one gene can influence, or be influenced by, the expression of another gene, collectively determining specific phenotypic traits in an organism.
Types of Gene Interaction
Gene interactions, pivotal in shaping the phenotypic traits of organisms, can be classified into two primary categories based on the nature of the genes involved and their locational attributes on chromosomes:
- Allelic or Non-epistatic Gene Interaction:
- Definition: This type of interaction transpires between the alleles of a singular gene.
- Characteristics: In this interaction, the alleles of the same gene influence each other’s expression, leading to variations in the phenotypic manifestation of the trait they control. Such interactions are foundational to Mendelian genetics, where dominant and recessive alleles of a gene determine the phenotype.
- Non-allelic or Epistatic Gene Interaction:
- Definition: This interaction pertains to the relationships between genes located on the same or different chromosomes.
- Characteristics: In epistatic interactions, one gene (the epistatic gene) masks or modifies the expression of another gene (the hypostatic gene). The outcome of this interaction can lead to phenotypes that deviate from expected Mendelian ratios, showcasing the complexity of genetic interplay.
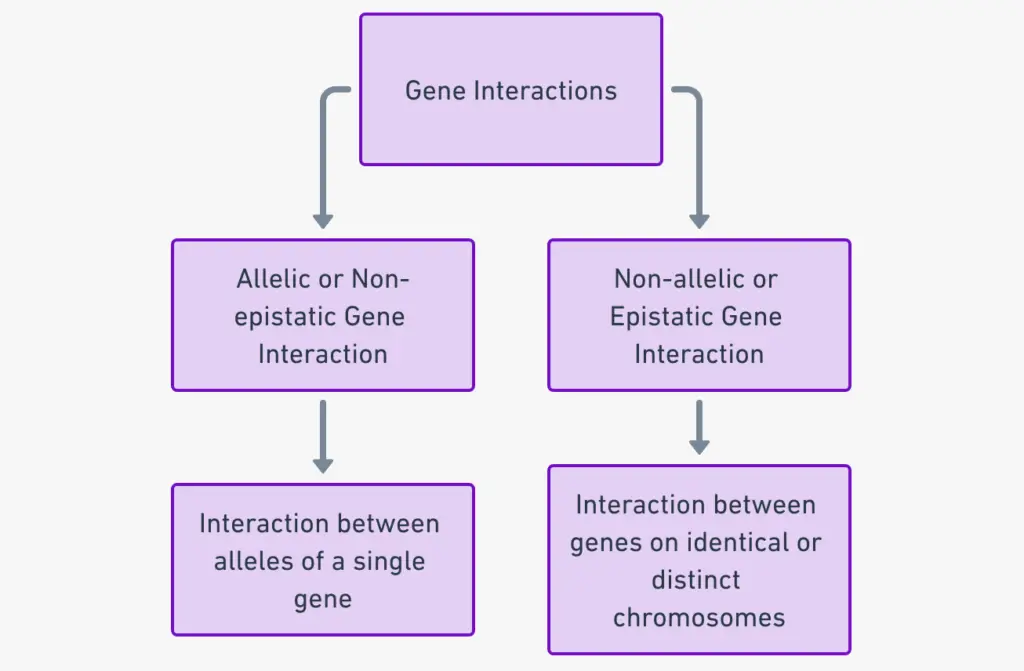
Allelic or Non-epistatic Gene Interaction
In the realm of genetics, allelic or non-epistatic gene interactions play a pivotal role in determining phenotypic outcomes. These interactions are characterized by the interplay between alleles of a singular gene. Unlike the straightforward dominant-recessive relationships postulated by Mendelian genetics, allelic interactions can be more intricate, leading to phenotypic ratios that deviate from the expected Mendelian patterns. Some of the types of allelic gene interactions are discussed below:
1. Complete Dominance
Complete dominance is a fundamental concept in genetics that adheres to Mendel’s laws of inheritance. Within this framework, each gene possesses two alleles. Among these alleles, one is categorically dominant over its counterpart. This dominance ensures that the presence of the dominant allele completely overshadows or masks the effects of the recessive allele. Consequently, only the phenotype associated with the dominant allele is manifested, even if the recessive allele is present.
A seminal discovery that underscored the principle of complete dominance was made by Archibald Garrod in 1903. Garrod’s research centered on alkaptonuria, a metabolic disorder marked by the excretion of homogentisic acid in urine. This condition manifests as a darkening of the urine when exposed to air. Delving into the family histories and analyzing urine samples from affected individuals, Garrod discerned a phenotypic ratio of 3:1 in affected families. This ratio was in alignment with Mendelian inheritance, where three individuals exhibited the dominant phenotype (absence of the disease) for every one individual with the recessive phenotype (presence of the disease).
Example: In the context of alkaptonuria:
- Dominant allele (A): Does not lead to alkaptonuria.
- Recessive allele (a): Leads to alkaptonuria when present in a homozygous state (aa).
Individuals with genotypes AA or Aa will not exhibit alkaptonuria, as the dominant allele masks the effects of the recessive allele. Only individuals with the genotype aa will manifest the disease.
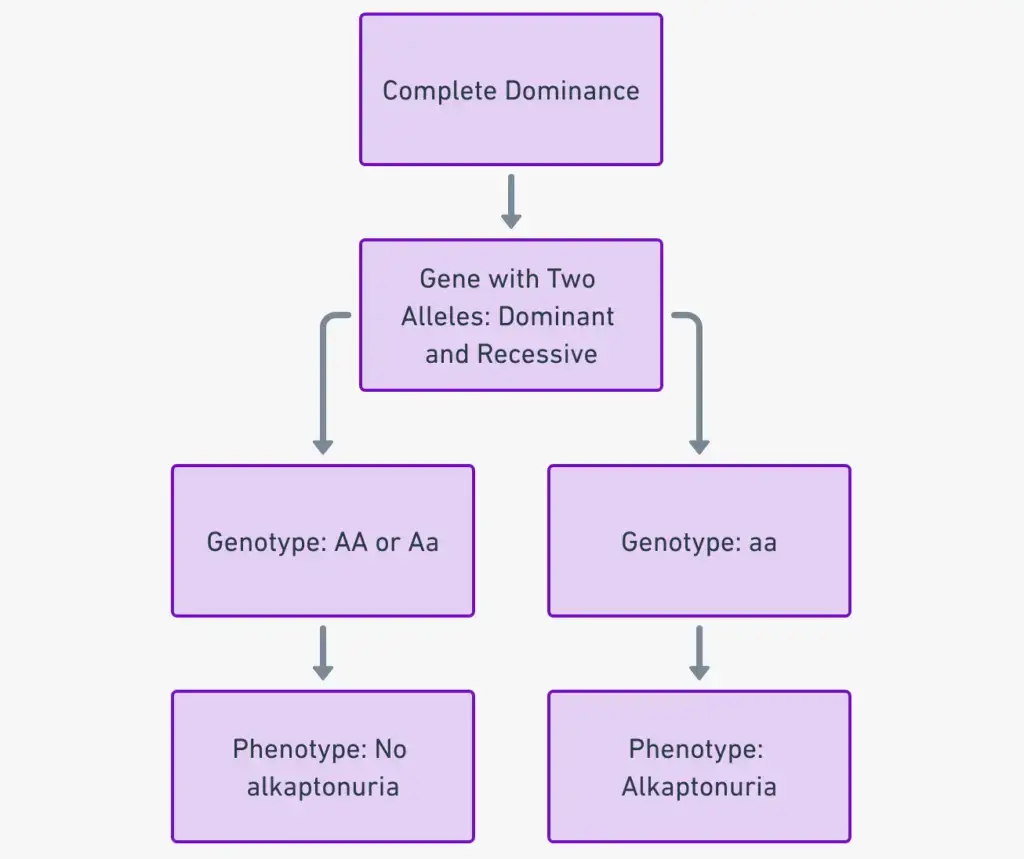
2. Incomplete Dominance
- Incomplete dominance is a genetic phenomenon where neither allele for a specific trait is completely dominant over the other. When two organisms possessing distinct traits are interbred, their progeny exhibit an intermediate phenotype, which is a blend of the parental traits.
- This deviation from the conventional dominant-recessive relationship is attributed to the fact that a dominant allele cannot entirely suppress the expression of the other allele.
- As a result, a heterozygote manifests a phenotype that is distinct from either homozygote, presenting an intermediate expression.
- A classic illustration of incomplete dominance is observed in the breeding of snapdragon plants (Mirabilis jalapa). When a pure-bred red-flowered snapdragon is crossed with a pure-bred white-flowered snapdragon, the resulting F1 hybrid generation produces pink-flowered plants. This pink coloration is an intermediate phenotype, deviating from both parental phenotypes. Further, when these F1 hybrids undergo self-fertilization, the subsequent F2 generation presents a phenotypic ratio of 1 red: 2 pink: 1 white. This ratio diverges from the typical 3:1 Mendelian ratio, underscoring the nuances of incomplete dominance.
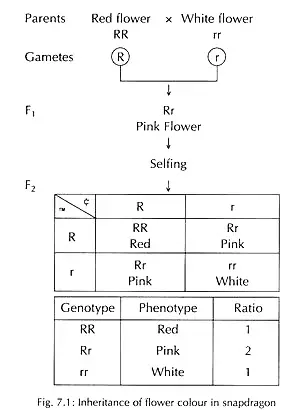
3. Codominance
- Codominance is a distinctive genetic phenomenon characterized by the concurrent and independent expression of two different alleles within an individual. In situations governed by codominance, neither allele asserts dominance over the other.
- Instead, both alleles are equivalently expressed, leading to the manifestation of both associated traits without any intermediate blending.
- This mode of inheritance stands in contrast to complete dominance, where the presence of one dominant allele completely overshadows or masks the expression of its recessive counterpart. In codominance, both alleles are conspicuously visible in the organism’s phenotype.
- A quintessential example of codominance is observed in the ABO blood group system in humans. Within this system, individuals can inherit an A, B, or O allele from each parent.
- Those inheriting an A allele from one parent and a B allele from the other exhibit both A and B antigens on the surface of their red blood cells. This results in the AB blood type, where both the A and B antigen alleles are co-dominantly expressed without one masking the other.
4. Lethal Allele
Lethal alleles are a subset of mutant alleles that, when expressed, disrupt vital physiological processes, culminating in the premature death of the organism. These alleles are of paramount significance in the realm of genetics, especially when studying human diseases, as they are often linked to critical genes whose malfunction can have dire consequences.
Lethal alleles can be categorized based on their dominance:
- Dominant Lethal Alleles: These alleles, when present, lead to the death of the organism irrespective of their zygosity. However, their persistence in populations is contingent upon their time of action:
- If they act early in life, carriers succumb before reaching reproductive age, leading to the allele’s eradication within a single generation.
- If they manifest later in life, post-reproduction, they can be transmitted to subsequent generations.
- Recessive Lethal Alleles: These alleles, when homozygous, result in lethality. However, when heterozygous, their deleterious effects are masked by the normal allele, allowing them to persist across generations. This phenomenon is due to the protective effect of the normal gene, which conceals the detrimental impact of the mutant allele.
A classic exemplar of a recessive lethal allele is observed in mice, specifically the yellow-lethal mutation, denoted as AY. This mutation exhibits a dual nature:
- Dominant for Coloration: Mice carrying the AY allele have a distinct yellow fur, a deviation from the standard gray-brown (agouti) coloration.
- Recessive for Lethality: Mice homozygous for the AY allele (AYAY) do not survive past early embryonic stages due to the mutation’s lethal effect.
When heterozygous yellow mice (AYA+) are interbred, the resulting offspring comprise yellow (AYA+) and gray-brown (A+A+) mice, with a phenotypic ratio of 2:1. Notably absent are the AYAY homozygotes, a testament to the lethal nature of the mutation when present in a homozygous state.
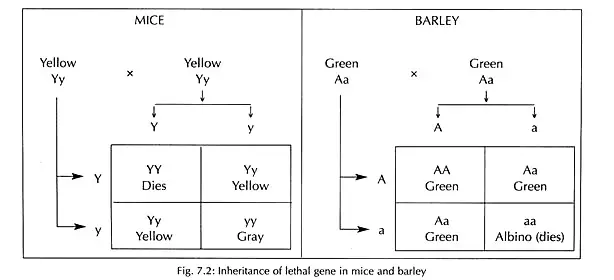
5. Multiple Alleles
In the realm of genetics, while Mendel’s foundational work posited the existence of two alleles for a given gene, subsequent research has unveiled a more intricate picture. Some genes, rather than being limited to just two allelic forms, possess multiple alleles. These alleles, though distinct, occupy the same chromosomal locus. The presence of multiple alleles for a single gene within a population adds layers of complexity to inheritance patterns and phenotypic outcomes.
A quintessential example underscoring the concept of multiple alleles is observed in rabbits, specifically in the gene governing coat color. This gene, denoted by the symbol “c,” is not restricted to a binary allelic system. Instead, it boasts four distinct alleles, each contributing differently to the rabbit’s coat coloration:
- c (Albino): This allele results in a complete absence of pigmentation, giving the rabbit an albino appearance.
- ch (Himalayan): Rabbits with this allele exhibit a unique coloration pattern reminiscent of the Himalayan mountains, with darker pigmentation on the extremities and a lighter body.
- cch (Chinchilla): This allele imparts a chinchilla-like coloration to the rabbit’s fur, characterized by a muted gray hue.
- c+ (Wild-type): Representing the standard or “wild” form, this allele produces the typical gray-brown coat observed in wild rabbit populations.
The presence of these multiple alleles within the rabbit population exemplifies the vast genetic diversity and the myriad phenotypic outcomes that can arise from a single gene. It underscores the fact that genetic inheritance is not always a straightforward binary system but can encompass a spectrum of allelic variations.
Non-Allelic Gene Interaction
In the realm of genetics, non-allelic gene interaction, also known as epistatic or inter-allelic interaction, is a phenomenon wherein two or more genes influence each other’s expression patterns, culminating in the manifestation of a singular phenotypic character. This interaction is distinct from the typical allelic interactions that occur within a single gene locus. Instead, non-allelic gene interactions involve genes that may be situated on the same chromosome or on different chromosomes.
Mechanism of Interaction
The underlying mechanism of non-allelic gene interaction is rooted in the intricate web of genetic regulation. When multiple genes are involved in determining a particular phenotype, the expression of one gene can mask or modify the expression of another. This masking effect, termed epistasis, can lead to phenotypic outcomes that deviate from the expected Mendelian ratios.
Example: Complementary Gene Interaction in Flower Color
A classic example of non-allelic gene interaction can be observed in the determination of flower color in certain plants. Consider two genes, A and B. Both genes are essential for the synthesis of a purple pigment in flowers. If a plant possesses at least one dominant allele (A) and one dominant allele (B), it will produce purple flowers. However, if either gene is homozygous recessive (aa or bb), the synthesis pathway is disrupted, resulting in white flowers. Thus, even though two separate genes are involved, they interact in a complementary manner to produce a single phenotypic outcome.
In this scenario, neither gene A nor gene B can independently produce the purple pigment. Their combined presence and interaction are crucial for the manifestation of the purple flower phenotype.
Some of the types of non-allelic gene interactions are discussed below:
1. Simple Interaction (9:3:3:1)
In the intricate tapestry of genetic inheritance, the 9:3:3:1 ratio represents a quintessential example of a dihybrid cross involving two non-allelic gene pairs. This interaction is emblematic of the Mendelian principles of independent assortment and segregation.
Mechanism of Interaction
The 9:3:3:1 interaction arises when two non-allelic gene pairs influence the same phenotypic character. Each gene pair, when acting independently, produces a distinct phenotype in the presence of its dominant allele. However, when both dominant alleles from the two gene pairs are concurrently present, they interact to produce a unique phenotype. Conversely, the absence of both dominant alleles culminates in yet another distinct phenotype.
Example: Seed Shape and Color in Pea Plants
Consider two gene pairs in pea plants: one governing seed shape (R for round and r for wrinkled) and the other determining seed color (Y for yellow and y for green). When subjected to a dihybrid cross, the following phenotypic ratio emerges:
- 9/16 of the offspring exhibit round and yellow seeds (RRYY, RRYy, RrYY, RrYy).
- 3/16 of the offspring display round and green seeds (RRyy, Rryy).
- 3/16 of the offspring manifest wrinkled and yellow seeds (rrYY, rrYy).
- 1/16 of the offspring show wrinkled and green seeds (rryy).
Here, the presence of dominant alleles R and Y independently results in round and yellow seeds, respectively. When both dominant alleles R and Y coexist, the seeds are both round and yellow. In the absence of both dominant alleles (rryy), the seeds are wrinkled and green.
2. Complementary Factor (9:7)
In the realm of genetics, the concept of gene interaction plays a pivotal role in determining phenotypic outcomes. One such interaction is the complementary gene interaction, where multiple genes collaborate to manifest a specific trait. The term “Complementary Factor (9:7)” refers to a specific phenotypic ratio that arises due to this interaction.
Definition: Complementary gene interaction involves two or more genes located at distinct loci, inherited from different parents, that work in tandem to produce a particular phenotype. Intriguingly, these genes, when present individually, remain silent and do not express the trait. Their expression is contingent upon their coexistence, achieved through appropriate genetic crossing.
Mechanism: The underlying mechanism of complementary gene interaction is rooted in the collaborative function of the genes involved. Each gene in the interaction might be responsible for a specific step or component in a biochemical pathway. The absence of any one gene disrupts the pathway, preventing the manifestation of the phenotype. Only when all the requisite genes are present can the pathway function optimally, leading to the expression of the desired trait.
Example: Consider a hypothetical plant species where flower color is determined by two genes, A and B. For the flower to exhibit a red color, both genes A and B must be functional. If either gene is non-functional or absent, the flower will be white.
In a dihybrid cross involving these genes, the phenotypic ratio observed would be 9:7, where:
- 9 represents the offspring with both functional genes A and B, resulting in red flowers.
- 7 represents the offspring where at least one of the genes (A or B) is non-functional, leading to white flowers.
This 9:7 ratio is emblematic of the complementary gene interaction, highlighting the collaborative nature of genes A and B in determining flower color.
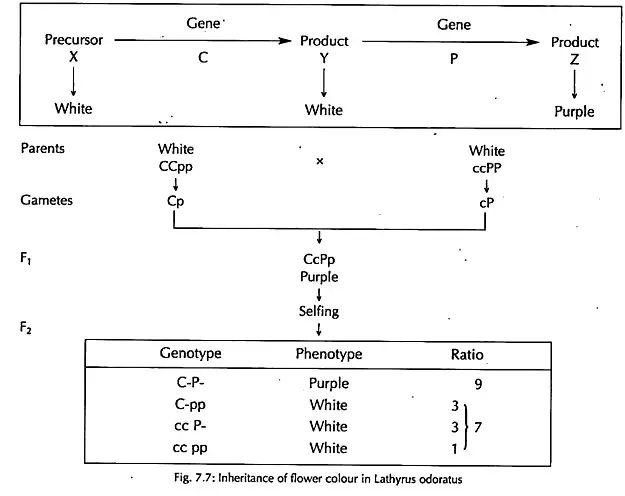
3. Epistasis
In the intricate tapestry of genetics, epistasis stands out as a phenomenon where the expression of one gene is influenced by another, non-allelic gene. This interaction results in the modification or complete masking of the phenotypic effect of one gene by another.
Definition and Origins: Epistasis is derived from the Greek word “epistasis,” meaning “stopping” or “standing upon.” The term was introduced by the pioneering geneticist William Bateson in 1907 to elucidate deviations from the expected Mendelian inheritance patterns. In this context, the gene that exerts the masking effect is termed the “epistatic gene,” while the gene whose effect is masked is referred to as the “hypostatic gene.”
Types of Epistasis:
- Dominant Epistasis: In this form of epistasis, a dominant allele at one gene locus suppresses the phenotypic expression of alleles at a second locus.Example: The flower coloration in foxgloves (Digitalis purpurea) serves as a classic illustration. Here, two genes play a pivotal role: one (with alleles d and D) influences the intensity of the red pigment, while the other (with alleles w and W) dictates the regions of pigment synthesis in the petals. The dominant allele W restricts pigment synthesis to specific throat spots, leading to predominantly white petals with red spots. When examining the F2 generation from a cross involving D/d and W/w alleles, it becomes evident that the W allele exhibits dominant epistasis, permitting red pigment synthesis only in the throat spots.
- Recessive Epistasis: Here, a recessive allele at one gene locus masks the phenotypic effects of alleles at another locus.Example: The coat color of Labrador retriever dogs provides a tangible example of recessive epistasis. While the B (black coat) and b (brown coat) alleles typically determine coat color, the presence of the recessive e allele (epistatic) alters this dynamic. Dogs with the e allele, regardless of their B or b genotype, exhibit a yellow coat phenotype.
Diverse Interpretations: It’s noteworthy that the term “epistasis” has garnered varied interpretations across different genetic disciplines. While Mendelian and molecular geneticists employ the term to denote specific gene interactions where one locus masks another, evolutionary and quantitative geneticists adopt a broader perspective, using “epistasis” to encompass any form of gene interaction.
Mapping Gene Interactions
Genetic interaction mapping is a pivotal technique in the realm of genetics, offering a deep dive into the intricate web of gene interactions. By understanding these interactions, we can gain insights into gene functions and the biological pathways they define. This knowledge is crucial for understanding the underlying mechanisms of various biological processes and diseases.
How Does Genetic Interaction Mapping Work?
The core principle behind genetic interaction mapping is observing the changes in an organism’s phenotype (observable traits) when specific genes are altered. This alteration can be achieved in several ways:
- Gene Deletion: By removing or “knocking out” a particular gene, researchers can observe the resulting changes in the phenotype. This helps in understanding the direct role of that gene in the organism’s biology.
- Gene Expression Inhibition: Instead of completely deleting a gene, its expression can be inhibited or reduced. This allows scientists to study the effects of reduced gene activity on the phenotype.
- High-throughput Screening: This method involves studying multiple genes simultaneously using automated systems. It’s particularly useful for mapping interactions between several genes and understanding complex genetic networks.
Example: Mapping Yeast Gene Interactions
Let’s consider an example using yeast, a commonly used model organism in genetics.
Researchers are interested in understanding the interaction between two genes, Gene A and Gene B. Individually, when either Gene A or Gene B is deleted, the yeast grows normally, indicating that neither gene is essential for growth. However, when both genes are deleted simultaneously, the yeast fails to grow.
This suggests a synthetic lethal interaction between Gene A and Gene B. In simpler terms, while the absence of either gene doesn’t affect the yeast, the absence of both is detrimental. This interaction indicates that these genes might be involved in parallel or compensatory pathways. When one pathway is disrupted, the other can compensate. But when both are disrupted, the organism cannot survive.
Such insights from genetic interaction mapping can be invaluable. For instance, in the context of drug development, if a disease-causing gene has a synthetic lethal partner, targeting that partner gene could be a potential therapeutic strategy.
Powerful Tools for a Complex Task
Techniques like Pairwise deletion, gene expression inhibition, and high-throughput screening are powerful tools in the geneticist’s arsenal. They allow for a comprehensive understanding of gene interactions, paving the way for advancements in various fields, from basic biology to therapeutic drug development.
Classification of Epistasis Gene Interaction
Epistasis is a phenomenon in genetics where the expression of one gene is influenced by the presence or absence of one or more other genes. This intricate interplay of genes is pivotal in determining the phenotypic outcomes in organisms. The classification of epistatic gene interactions is based on the manner in which the genes in question influence each other’s expression. The following elucidates the various types of epistatic interactions:
- Supplementary Gene Interaction:
- This interaction is characterized by the dominant allele of one gene producing a phenotypic effect, while the dominant allele of another gene lacks an independent phenotypic effect. However, when present alongside the dominant allele of the first gene, it modifies the latter’s phenotypic expression. An exemplification of this is the Agouti (grey) coat coloration in mice.
- In the F2 generation, the phenotypic ratio manifests as 9 (Agouti):3 (coloured):4 (Albino).
- Complementary Gene Interaction:
- Here, both gene loci, when homozygous, yield the same phenotype, altering the expected F2 ratio to 9:7 from 9:3:3:1. The genes are termed “complementary” because a unique phenotype emerges only when both dominant alleles coexist. For instance, in sweet pea, certain homozygous gene combinations prevent chromogen production, resulting in a white coloration, which is altered in heterozygous conditions.
- Inhibitory Gene Interaction:
- This interaction is observed when the presence of a homozygous recessive trait at one gene locus inhibits the phenotypic expression of other genes. The F2 ratio is altered to 13:3 from the conventional 9:3:3:1.
- Duplicate Gene Interaction:
- In this interaction, both gene loci’s dominant alleles yield the same phenotype without any additive effect. The resultant phenotypic ratio is 15:1 instead of 9:3:3:1. An example is observed in the shepherd’s purse plant, where seed capsules can be either triangular or oval based on the gene combinations.
- Masking Gene Interaction:
- This interaction is characterized by the dominant allele of one gene (epistatic locus) overshadowing or “masking” the effects of another gene’s allele (hypostatic locus). The expression of the hypostatic locus is only evident when the epistatic locus allele is in a homozygous recessive state.
- Polymeric Gene Interaction:
- This interaction involves two dominant alleles that collaboratively enhance the phenotype or yield an intermediate variation. The presence of each dominant allele individually results in a distinct phenotype, but their combined presence leads to a third unique phenotype. Neither of the dominant alleles overshadows the other.
In conclusion, the realm of epistatic gene interactions is vast and intricate, with each type playing a crucial role in the phenotypic manifestation of organisms. Understanding these interactions is pivotal for advancements in genetics and evolutionary biology.
Importance of Gene Interactions
1. Deciphering Functional Relationships
By studying gene interactions in model organisms, we can unravel the functional relationships between genes and their gene products. Model organisms, like fruit flies or yeast, serve as simplified systems that allow scientists to dissect genetic interactions in a controlled environment.
2. Unveiling Genetic Pathways
Gene interactions are the roadmap to understanding the key components and mechanisms involved in genetic pathways. These pathways are like circuits, where each gene plays a role in ensuring the system functions correctly. By understanding how genes interact, we can pinpoint where and how these circuits might break down in diseases.
3. Understanding Phenotypic Variation
The vast diversity in traits among organisms, known as phenotypic variation, is largely due to gene interactions. Different genes collaborate to express various phenotypic traits. For instance, the color of a flower might not be determined by a single gene but by the interaction of several genes working in tandem.
Example: The Height of an Organism
Consider height in humans. While it might be tempting to think there’s a “height gene,” the reality is far more complex. Multiple genes interact to determine an individual’s height, and these interactions can be influenced by environmental factors like nutrition. A mutation in one gene might have a minimal effect, but when combined with mutations in other height-related genes, the result could be a significant change in stature.
4. Evolution of Genetic Systems
Gene interactions offer a lens into the evolutionary history of organisms. By studying how genes interact and change over time, we gain insights into how complex genetic systems have evolved. This evolutionary perspective can help explain why certain genetic interactions exist and how they’ve shaped the diversity of life on Earth.
5. Decoding Complex Molecular Mechanisms
The world of gene interactions is intricate, governed by a myriad of molecular mechanisms. These interactions are not just about genes turning on or off; they involve various processes like gene regulation, protein-protein interactions, and feedback loops. Understanding these complex interactions is foundational for grasping how genes collectively influence biological processes.
In conclusion, gene interactions are a cornerstone in the study of genetics. They offer profound insights into the functional, phenotypic, evolutionary, and molecular aspects of biology, driving our understanding forward and paving the way for innovations in medicine, agriculture, and beyond.
FAQ
What is a gene interaction?
Gene interaction refers to the phenomenon where multiple genes influence a single phenotype or trait. It’s the interplay between genes that determines how they express themselves in an organism.
How do gene interactions differ from Mendelian inheritance?
While Mendelian inheritance focuses on the inheritance patterns of single genes, gene interactions consider the combined effects of two or more genes on a single phenotype.
What is epistasis?
Epistasis is a type of gene interaction where one gene masks or modifies the effect of another gene. It can alter expected Mendelian phenotypic ratios in the offspring.
How is complementary gene interaction different from supplementary gene interaction?
In complementary gene interaction, two non-allelic genes work together to produce a phenotype. Both genes are required for the trait to manifest. In supplementary gene interaction, one gene modifies the phenotype produced by another gene.
What is meant by the term “masking” in gene interactions?
“Masking” refers to the phenomenon where the effect of one gene (the epistatic gene) hides or overshadows the effect of another gene (the hypostatic gene).
Why are gene interactions important in understanding phenotypic outcomes?
Gene interactions provide a more comprehensive understanding of how traits are expressed. They explain why some phenotypic ratios deviate from expected Mendelian ratios and offer insights into the complexity of genetic control in traits.
Can gene interactions be influenced by environmental factors?
Yes, the expression of genes and their interactions can be influenced by environmental conditions. This interplay between genes and the environment can lead to varied phenotypic outcomes.
What is a polygenic trait?
A polygenic trait is controlled by multiple genes, each contributing a small effect to the overall phenotype. Height, skin color, and intelligence are examples of polygenic traits.
How do duplicate gene interactions work?
In duplicate gene interaction, two genes control a trait, and the presence of a dominant allele in either of the genes can produce the same phenotype. The combined effect of both genes doesn’t enhance the trait further.
Are gene interactions the same in all organisms?
While the basic principles of gene interactions are consistent across organisms, the specific interactions and outcomes can vary based on the organism’s genetic makeup and evolutionary history.
References
- Moore, J. H. (2013). Gene Interaction. Brenner’s Encyclopedia of Genetics, 200–201. doi:10.1016/b978-0-12-374984-0.00592-1
- Goodnight, C. J. (2016). Gene Interactions in Evolution. Encyclopedia of Evolutionary Biology, 104–109. doi:10.1016/b978-0-12-800049-6.00048-2
- https://www.slideshare.net/harshrajshinde1/gene-interaction
- https://www.turito.com/learn/biology/gene-interactions-grade-10
- https://www.ndsu.edu/pubweb/~mcclean/plsc431/mendel/mendel6.htm
- https://www.biologydiscussion.com/genetics/gene-interactions/gene-interactions-allelic-and-non-allelic-cell-biology/38795