What is glycolysis?
- Glycolysis, a fundamental metabolic pathway, is responsible for the catabolism of glucose, a six-carbon sugar molecule, into pyruvate, a three-carbon compound. This intricate process comprises ten distinct enzymatic reactions, occurring within the cytosol of cells. While glycolysis is universally present in both aerobic and anaerobic organisms, its regulatory mechanisms and the eventual metabolic fate of the resultant pyruvate can vary among species.
- In organisms that respire aerobically, glycolysis serves as an introductory phase to the citric acid cycle and the electron transport chain. These subsequent pathways, in conjunction with glycolysis, facilitate the extraction of the majority of energy stored within glucose molecules. The Embden-Meyerhof-Parnas (EMP) pathway, named in recognition of its pioneering researchers, is the most prevalent form of glycolysis. However, it’s worth noting that other glycolytic pathways, such as the Entner–Doudoroff pathway, do exist, though this discussion primarily focuses on the EMP pathway.
- The glycolytic process can be conceptually divided into two main phases. The initial phase, termed the ‘Investment phase’, involves the consumption of ATP. This is followed by the ‘Yield phase’, where the amount of ATP generated surpasses the amount initially consumed. The energy liberated during glycolysis is harnessed to synthesize high-energy molecules, specifically adenosine triphosphate (ATP) and reduced nicotinamide adenine dinucleotide (NADH).
- Given its ubiquity across diverse species, glycolysis is believed to be an evolutionarily ancient pathway. Some theories even suggest that the reactions constituting glycolysis, along with the pentose phosphate pathway, could have transpired in the anoxic conditions of early Earth’s oceans, driven by metal ions and devoid of enzymatic action. This lends credence to the idea that glycolysis could have played a role in abiogenesis, the origin of life from non-living matter.
- In summary, glycolysis is a pivotal biochemical pathway that facilitates the conversion of glucose into pyruvate, releasing energy that is crucial for various cellular functions. Its widespread presence across organisms underscores its evolutionary significance and central role in cellular metabolism.
Definition of glycolysis
Glycolysis is a metabolic pathway that converts glucose into pyruvate, releasing energy used to form molecules of ATP and NADH, primarily occurring in the cytosol of cells.
Salient features of glycolysis
- Ubiquity: Glycolysis is a universal metabolic pathway, occurring in all cells.
- Cellular Location: The enzymes facilitating glycolysis are localized in the cytosol of cells.
- Oxygen Dependency: Glycolysis can function under both anaerobic and aerobic conditions. In the absence of oxygen (anaerobic), the end product is lactate. Conversely, in the presence of oxygen (aerobic), pyruvate is produced, which subsequently undergoes oxidation to yield CO2 and H2O.
- ATP Synthesis: In cells lacking mitochondria, such as erythrocytes, the cornea, and the lens, glycolysis serves as the primary means of ATP generation.
- Brain Energy Source: The brain predominantly relies on glucose as its energy source. For glucose to be fully oxidized to H2O and CO2, it must first undergo glycolysis.
- Net Reaction: In anaerobic conditions, glycolysis can be summarized by the equation: Glucose + 2ADP + 2Pi → 2Lactate + 2ATP.
- Metabolic Significance: Glycolysis stands as a cornerstone in metabolism. Many of its intermediates act as junctions, branching off to other metabolic pathways. For instance, glycolytic intermediates can be channeled towards the synthesis of amino acids and fats.
- Reversibility: While glycolysis primarily breaks down glucose, the synthesis of glucose is also feasible by reversing this pathway. However, certain irreversible steps necessitate alternate mechanisms.
In essence, glycolysis is a fundamental metabolic process, intricately linked with various other pathways, underscoring its central role in cellular energy production and biosynthesis.
Site of Glycolysis
Glycolysis is a metabolic process that predominantly occurs in the cytosol, the liquid matrix within cells. This intracellular compartment houses the necessary enzymes and substrates that facilitate the glycolytic reactions. Notably, certain tissues, such as erythrocytes, the cornea, and the lens, lack mitochondria, the primary site for energy production in many cells. In these mitochondria-devoid tissues, the cytosol becomes the principal site for ATP synthesis via glycolysis. Thus, the cytosol plays a pivotal role in ensuring energy production, especially in cells where alternative energy-generating pathways are absent.
Types of Glycolysis
Glycolysis, a fundamental metabolic pathway, can function under varying oxygen conditions, leading to its classification into two primary types:
- Aerobic Glycolysis: This form of glycolysis transpires in the presence of ample oxygen. The end product of this pathway is pyruvate. Concurrently, there is the synthesis of adenosine triphosphate (ATP) molecules, which serve as the primary energy currency of the cell.
- Anaerobic Glycolysis: In conditions where oxygen is limited or absent, glycolysis proceeds anaerobically. Under such circumstances, lactate is produced as the terminal product. Similar to its aerobic counterpart, ATP molecules are generated during this process.
In essence, the adaptability of glycolysis to function in both oxygen-rich and oxygen-deprived environments underscores its versatility and central role in cellular energy metabolism.
Glycolysis equation
The glycolytic pathway can be succinctly represented by the following chemical equation:
C6H12O6+2ADP+2Pi+2NAD+→2C3H4O3+2H2O+2ATP+2NADH+2H+
Interpreting the equation:
- Reactants:
- C6H12O6: Glucose, the primary substrate for glycolysis.
- 2ADP: Adenosine diphosphate, which gets phosphorylated to produce ATP.
- 2Pi: Inorganic phosphate, involved in the phosphorylation process.
- 2NAD+: Nicotinamide adenine dinucleotide, an electron acceptor that gets reduced during glycolysis.
- Products:
- 2C3H4O3: Pyruvate, the end product of glycolysis.
- 2H2O: Water molecules.
- 2ATP: Adenosine triphosphate, the main energy currency of the cell.
- 2NADH: Reduced form of NAD^+, which carries electrons to subsequent metabolic pathways.
- 2H+: Hydrogen ions, indicating the release of protons during the process.
This equation provides a comprehensive overview of the molecular transformations that occur during glycolysis, highlighting the conversion of glucose into pyruvate and the associated energy transfer processes.
Enzymes of Glycolysis
Glycolysis is a sequence of enzymatically catalyzed reactions that convert glucose into pyruvate. The enzymes responsible for these reactions predominantly reside in the cytosol, the liquid matrix of cells, outside the mitochondria. A noteworthy feature of these enzymes is their dependency on magnesium ions (Mg^2+) for their activity. Here is a detailed overview of the enzymes involved in glycolysis and their specific roles:
- Hexokinase: Initiates glycolysis by phosphorylating glucose, a crucial step in glucose metabolism.
- Phosphoglucoisomerase (Phosphohexose isomerase): Transforms glucose-6-phosphate into its isomer, fructose-6-phosphate.
- Phosphofructokinase: Utilizing ATP, this enzyme phosphorylates fructose-6-phosphate, introducing another phosphate group.
- Aldolase: Cleaves the six-carbon fructose-6-phosphate into two three-carbon molecules: dihydroxyacetone phosphate (DHAP) and glyceraldehyde-3-phosphate (GAP).
- Phosphotriose isomerase (Isomerase): Converts DHAP into its isomer, glyceraldehyde-3-phosphate.
- Glyceraldehyde 3-phosphate dehydrogenase: This enzyme both dehydrogenates GAP and phosphorylates it, transforming it into 1,3-biphosphoglycerate.
- Phosphoglycerate kinase: Facilitates the transfer of a phosphate group to ADP.
- Phosphoglycerate mutase: Modifies the position of the phosphate group, converting 3-phosphoglycerate into 2-phosphoglycerate.
- Enolase: Extracts a water molecule from 2-phosphoglycerate, producing phosphoenolpyruvate (PEP).
- Pyruvate kinase: Transfers the phosphate from phosphoenolpyruvate, culminating in the formation of pyruvate.
It’s worth noting that the nomenclature of enzymes typically ends with the suffix “-ase,” indicating their enzymatic nature. The meticulous orchestration of these enzymes ensures the efficient breakdown of glucose, a pivotal process in cellular energy production.
Enzyme | Reaction |
Hexokinase | Glucose + ATP → Glucose-6-phosphate + ADP |
Phosphofructokinase (PFK) | Fructose-6-phosphate + ATP → Fructose-1,6-bisphosphate + ADP |
Aldolase | Fructose-1,6-bisphosphate → DHAP + G3P |
Triose Phosphate Isomerase | DHAP ⇌ G3P |
Glyceraldehyde-3-Phosphate Dehydrogenase (GAPDH) | G3P + NAD+ + Pi → 1,3-BPG + NADH + H+ |
Phosphoglycerate Kinase | 1,3-BPG + ADP → 3-PG + ATP |
Phosphoglycerate Mutase | 3-PG ⇌ 2-PG |
Enolase | 2-PG → PEP + H2O |
Pyruvate Kinase | PEP + ADP → Pyruvate + ATP |
Glycolysis steps
During glycolysis, one molecule of 6-carbon glucose is split into two molecules of 3-carbon pyruvate through a series of 10 enzymatic reactions. These reactions are categorized into two phases: Phase I and Phase II. Phase I, also known as the “preparatory” phase, encompasses non-redox reactions that don’t release energy but lead to the creation of a vital intermediate for the pathway. This phase covers the initial five steps of glycolysis. Conversely, Phase II involves redox reactions where energy is stored as ATP, and two pyruvate molecules are generated. This phase includes the concluding five steps of the glycolysis process.
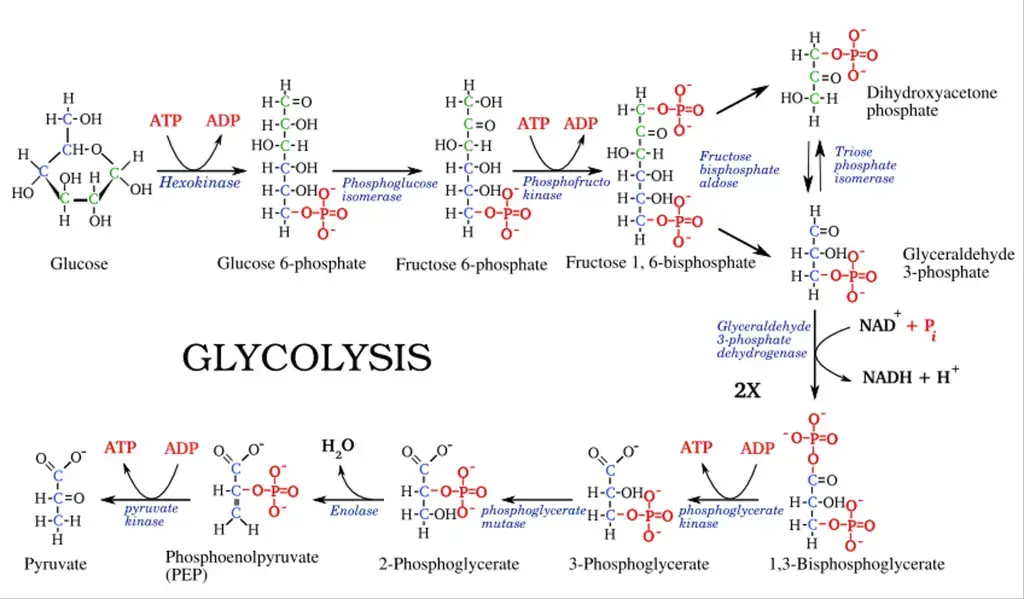
A. Phase 1: Preparatory Phase of Glycolysis
The “Preparatory Phase,” often referred to as the glucose activation phase, initiates the glycolysis process. During this phase, glucose undergoes a series of transformations: it is first phosphorylated, then isomerized, followed by another phosphorylation, and finally cleaved, resulting in two triose molecules. Specifically, the preparatory phase encompasses the initial five reactions of glycolysis, labeled as reactions 1 through 5. These reactions collectively lead to the conversion of glucose into two molecules of Glyceraldehyde-3-Phosphate. Notably, this phase requires an energy investment, consuming 2 ATPs for each glucose molecule processed.
1. Step 1 – Phosphorylation of glucose (Glucose → Glucose-6-phosphate)
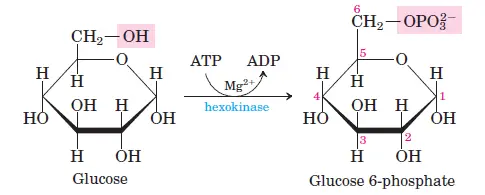
The initial step in glycolysis involves the phosphorylation of glucose at its C6 carbon. This pivotal reaction results in the formation of Glucose-6-phosphate. The enzymatic catalysts facilitating this transformation are hexokinase, which is prevalent in both liver and extrahepatic tissues, and glucokinase, which is specific to liver cells in animals and microbes. During this process, a phosphate group is transferred from an ATP molecule to glucose, converting ATP to ADP. This reaction is not only exothermic, releasing a significant amount of heat, but it is also irreversible, marking it as a regulatory step in glycolysis. Furthermore, this step is recognized as the flux-generating phase of the glycolytic pathway. It’s essential to note that the presence of Mg^2+ as a cofactor is crucial for this reaction to proceed.
2. Step 2 – Isomerization of Glucose-6-phosphate (Glucose-6-phosphate → Fructose-6-phosphate)
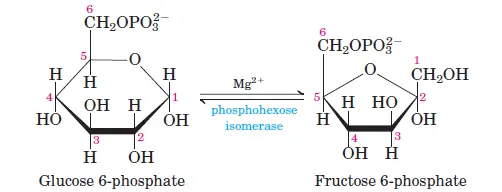
The second step of glycolysis encompasses the isomerization of Glucose-6-phosphate into Fructose-6-phosphate. This transformation is facilitated by the enzyme phosphohexoisomerase, also known as phosphoglucoisomerase. During this reaction, the carbonyl oxygen undergoes a positional shift from the C1 carbon to the C2 carbon, leading to the conversion of an aldose sugar into a ketose sugar. This process is characterized by Aldose-Ketose Isomerism. Notably, this isomerization reaction is reversible, allowing for the interconversion between Glucose-6-phosphate and Fructose-6-phosphate.
3. Step 3 – Phosphorylation of fructose-6-phosphate (fructose-6-phosphate → fructose-1,6-bisphosphate)
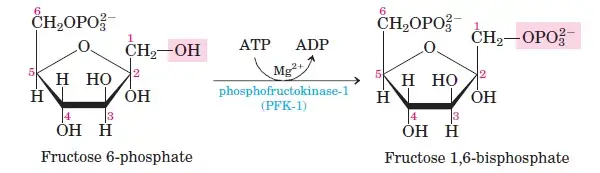
In the third step of glycolysis, fructose-6-phosphate undergoes an additional phosphorylation to form fructose-1,6-bisphosphate. This transformation is mediated by the enzyme phosphofructokinase. Similar to the initial phosphorylation step, an ATP molecule donates a phosphate group during this reaction. As fructose-6-phosphate is phosphorylated, energy is expended and subsequently dissipated as heat. This step serves as a crucial preparatory phase, priming the sugar for subsequent breakdown in the glycolytic pathway.
4. Step 4 – Cleavage of fructose 1, 6-diphosphate (fructose 1,6-bisphosphate → glyceraldehyde 3-phosphate, an aldose, and dihydroxyacetone phosphate, a ketose)
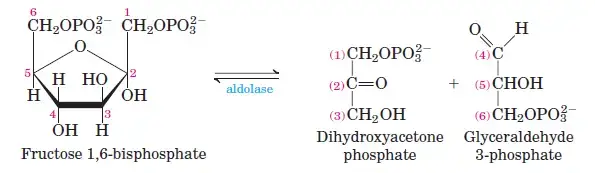
In the fourth stage of glycolysis, the six-carbon compound fructose 1,6-bisphosphate undergoes a distinctive cleavage, splitting it into two distinct three-carbon molecules. This cleavage is facilitated by the enzyme known as fructose diphosphate aldolase. As a result of this enzymatic action, two triose phosphates are produced: glyceraldehyde 3-phosphate, an aldose, and dihydroxyacetone phosphate, a ketose. From this point onward in the glycolytic pathway, the reactions are centered around these three-carbon molecules, marking a shift from the initial six-carbon glucose molecule.
5. Step 5 – Isomerization of dihydroxyacetone phosphate (Dihydroxyacetone phosphate → Glyceraldehyde 3-phosphate )
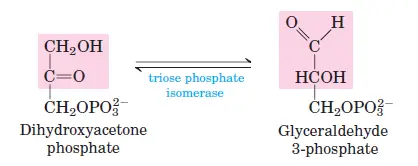
In the fifth stage of glycolysis, the molecule dihydroxyacetone phosphate undergoes a transformation to become more compatible with the subsequent reactions of the glycolytic pathway. While glyceraldehyde 3-phosphate can progress through the subsequent stages of glycolysis, dihydroxyacetone phosphate cannot. To address this, the enzyme triose phosphate isomerase facilitates the isomerization of dihydroxyacetone phosphate, converting it into glyceraldehyde 3-phosphate. This ensures that both molecules produced from the earlier cleavage of fructose 1,6-bisphosphate can continue through the glycolytic pathway. With this isomerization, the preparatory phase of glycolysis is brought to completion, setting the stage for the energy-yielding reactions that follow.
B. Phase 2: Payoff Phase
The “Payoff Phase,” commonly referred to as the energy extraction phase, is a pivotal stage in glycolysis. During this phase, glyceraldehyde 3-phosphate undergoes a series of transformations, culminating in the formation of pyruvate. Notably, this phase is characterized by the production of 4 ATPs for every glucose molecule processed. However, considering the energy investment in the initial phase, the net gain stands at 2 ATPs for each glucose molecule.
6. Step 6- Oxidative Phosphorylation of Glyceraldehyde 3-phosphate (glyceraldehyde 3-phosphate → 1,3-bisphosphoglycerate)
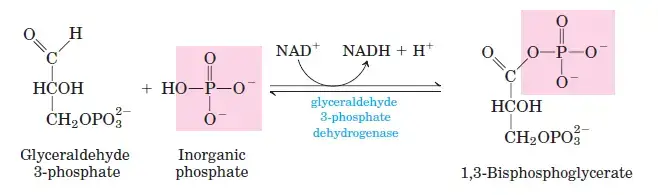
In the sixth stage of glycolysis, the molecule glyceraldehyde 3-phosphate undergoes a significant transformation, marking one of the pivotal energy-generating steps of the pathway. This reaction is facilitated by the enzyme glyceraldehyde 3-phosphate dehydrogenase, which aids in the conversion of glyceraldehyde 3-phosphate to 1,3-bisphosphoglycerate. A noteworthy aspect of this step is the involvement of the coenzyme NAD+. During the reaction, NAD+ is reduced, accepting a hydrogen atom from glyceraldehyde 3-phosphate, resulting in the formation of NADH. Given that a single glucose molecule yields two molecules of glyceraldehyde 3-phosphate, this step produces two NADH molecules for every glucose molecule that enters glycolysis. This step is crucial as it sets the stage for subsequent energy-yielding reactions in the glycolytic pathway.
7. Step 7- Transfer of phosphate from 1, 3-diphosphoglycerate to ADP (1,3-bisphosphoglycerate → 3-phosphoglycerate, ATP)
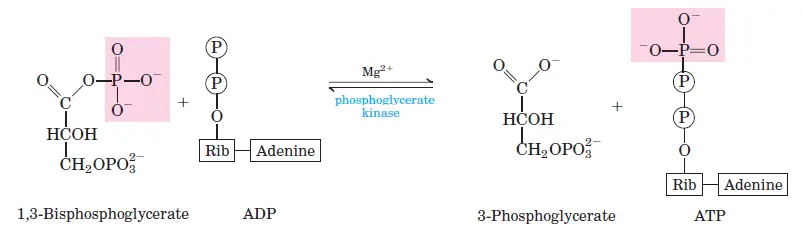
In the seventh stage of glycolysis, a pivotal ATP-producing reaction takes place. The molecule 1,3-bisphosphoglycerate undergoes a phosphate transfer to ADP, facilitated by the enzyme phosphoglycerate kinase. This enzymatic action results in the formation of ATP and the molecule 3-phosphoglycerate. Given the stoichiometry of the glycolytic pathway, where one glucose molecule gives rise to two molecules of 1,3-bisphosphoglycerate, this step culminates in the generation of two ATP molecules. This phase underscores the energy-conservation aspect of glycolysis, highlighting its significance in cellular metabolism.
8. Step 8 – Isomerization of 3-phosphoglycerate (3-phosphoglycerate → 2-phosphoglycerate)
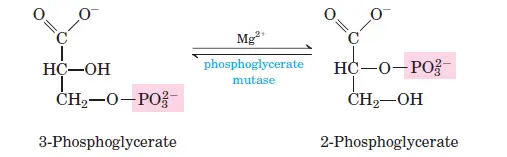
In the eighth stage of glycolysis, the molecule 3-phosphoglycerate undergoes a structural rearrangement. This isomerization process involves the migration of the phosphoryl group from the third carbon (C3) to the second carbon (C2) position. The enzyme responsible for catalyzing this transformation is phosphoglycerate mutase. As a result of this enzymatic action, 2-phosphoglycerate is produced. Notably, this reaction is reversible in nature, allowing for flexibility in metabolic pathways depending on cellular needs. This step exemplifies the intricate regulatory mechanisms inherent in biochemical pathways, ensuring optimal energy production and utilization.
9. Step 9- Dehydration 2-phosphoglycerate (2-phosphoglycerate → phosphopyruvate )
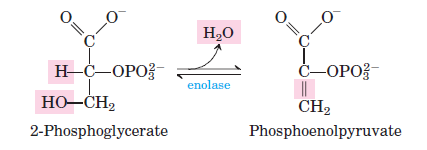
During the ninth phase of glycolysis, the molecule 2-phosphoglycerate undergoes a dehydration process, leading to the removal of a water molecule. This transformation is facilitated by the enzyme enolase, also known as phosphopyruvate hydratase. As a result of this enzymatic activity, phosphoenolpyruvate is formed. It’s noteworthy that this reaction is irreversible, signifying its critical role in directing the metabolic pathway forward. For every mole of 2-phosphoglycerate processed, two moles of water are eliminated, underscoring the dehydration nature of this step. This stage exemplifies the precision and specificity of enzymatic reactions in metabolic pathways, ensuring the efficient progression of glycolysis.
10. Step 10 – Transfer of phosphate from phosphoenolpyruvate (Phosphoenolpyruvate → Pyruvate)
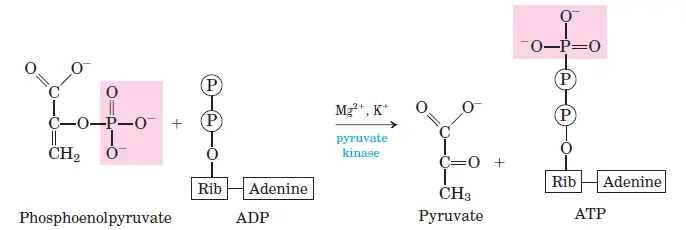
In the tenth and final step of glycolysis, phosphoenolpyruvate undergoes a transformation mediated by the enzyme pyruvate kinase. During this process, phosphoenolpyruvate is transmuted into its enol form of pyruvate. Intriguingly, this enol pyruvate does not remain stable for long; it spontaneously and swiftly rearranges to produce the keto form of pyruvate, commonly referred to as ketopyruvate. At a neutral pH of 7.0, this keto form is the predominant species. A significant aspect of this reaction is the energy generation: the enzyme pyruvate kinase facilitates the transfer of a phosphoryl group from phosphoenolpyruvate directly to ADP, culminating in the production of ATP. This step underscores the intricate balance of energy conservation and expenditure in metabolic pathways, ensuring cellular energy homeostasis.
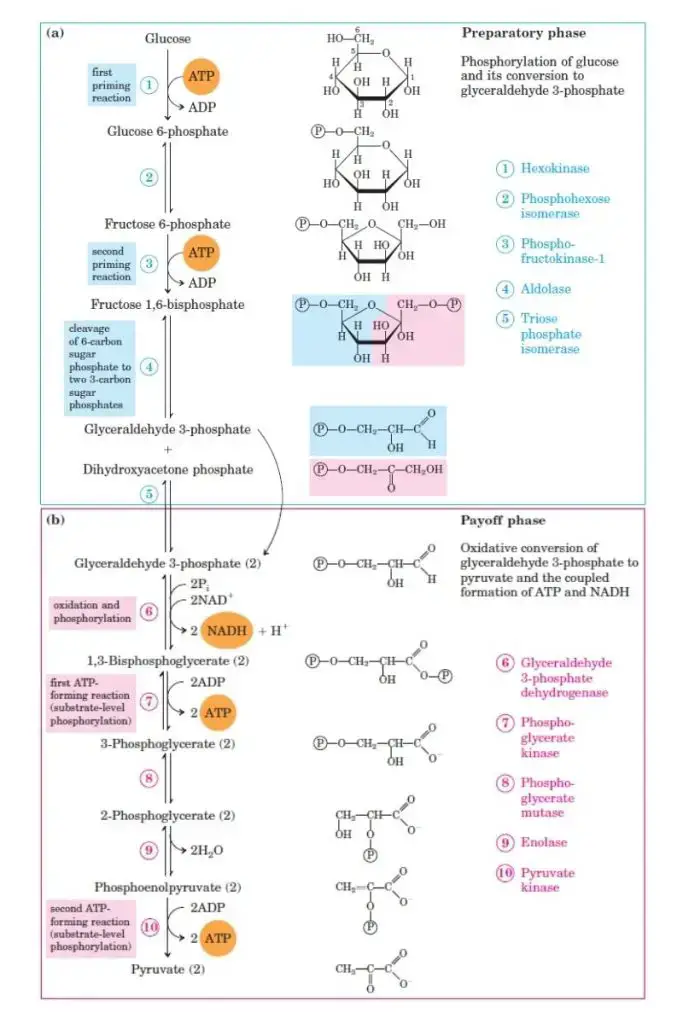
Additional Step in Anaerobic Condition
- In anaerobic conditions, certain tissues in animals face a challenge due to the limited supply of oxygen, which is essential for the aerobic oxidation of pyruvate and NADH generated during glycolysis. To circumvent this limitation and ensure the continuity of the glycolytic pathway, a unique mechanism is employed to regenerate NAD+ from NADH.
- This mechanism involves the enzymatic reduction of pyruvate to lactate, a reaction catalyzed by the enzyme Lactate Dehydrogenase (LDH). As a result of this reduction, NADH donates its electrons to pyruvate, forming lactate and regenerating NAD+ in the process. This regenerated NAD+ can then participate in further rounds of glycolysis, ensuring a continuous supply of ATP in the absence of oxygen.
- Certain tissues, such as skeletal and smooth muscles, often operate under hypoxic or low-oxygen conditions and, as a result, predominantly produce lactate. A notable example is erythrocytes (red blood cells), which, despite the presence of oxygen, produce lactate as the end product of glycolysis. This is attributed to the absence of mitochondria in erythrocytes, which prevents them from undergoing aerobic respiration.
- In essence, the conversion of pyruvate to lactate in anaerobic conditions serves as a crucial adaptive mechanism, allowing cells to maintain energy production and metabolic activities even when oxygen is scarce.
Glycolysis 10 Steps Summery
Glycolysis is a central metabolic pathway that involves the breakdown of glucose into pyruvate. This ten-step process is intricately orchestrated by specific enzymes and is vital for cellular energy production. Here’s a detailed breakdown of the glycolysis pathway:
- Step 1: Phosphorylation of Glucose: In the initial phase, glucose undergoes phosphorylation to form glucose-6-phosphate. This reaction, catalyzed by either glucokinase (in liver cells) or hexokinase (in other tissues), consumes one ATP molecule. This step is a pivotal, irreversible reaction that sets the pace for the entire glycolytic pathway.
- Step 2: Isomerization to Fructose-6-Phosphate: The glucose-6-phosphate is then transformed into fructose-6-phosphate by the enzyme phosphohexose isomerase. This reaction showcases aldose-ketose isomerism and is reversible.
- Step 3: Formation of Fructose 1,6-Bisphosphate: Fructose-6-phosphate is further phosphorylated to yield fructose 1,6-bisphosphate. This step, facilitated by phosphofructokinase-1, is another irreversible stage and is considered the rate-limiting step of glycolysis.
- Step 4: Cleavage into Triose Phosphates: The enzyme aldolase cleaves fructose 1,6-bisphosphate into two three-carbon compounds: glyceraldehyde-3-phosphate and dihydroxyacetone phosphate.
- Step 5: Isomerization of Dihydroxyacetone Phosphate: Dihydroxyacetone phosphate is isomerized to form another molecule of glyceraldehyde-3-phosphate, ensuring that two molecules of this triose phosphate are available for the subsequent steps.
- Step 6: Oxidation to 1,3-Bisphosphoglycerate: Glyceraldehyde-3-phosphate is oxidized, forming 1,3-bisphosphoglycerate. This step, mediated by glyceraldehyde-3-phosphate dehydrogenase, also produces NADH.
- Step 7: ATP Generation: The high-energy phosphate of 1,3-bisphosphoglycerate is transferred to ADP, producing ATP and 3-phosphoglycerate. This substrate-level phosphorylation is catalyzed by phosphoglycerate kinase.
- Step 8: Phosphate Shift: The phosphate group of 3-phosphoglycerate is relocated to the second carbon, yielding 2-phosphoglycerate. This rearrangement is facilitated by phosphoglycerate mutase.
- Step 9: Dehydration to Phosphoenolpyruvate: The enzyme enolase catalyzes the dehydration of 2-phosphoglycerate, resulting in the formation of phosphoenolpyruvate.
- Step 10: Final ATP Production: In the concluding step, phosphoenolpyruvate is converted to pyruvate by pyruvate kinase, generating another ATP molecule.
In summary, glycolysis is a meticulously regulated process that ensures efficient glucose breakdown and energy production. Each step is facilitated by specific enzymes, ensuring the precise conversion of glucose to pyruvate.
Result of Glycolysis/Products of Glycolysis
Glycolysis is a central metabolic pathway that breaks down glucose to produce energy and precursor molecules. The culmination of this intricate process yields several key products, each playing a pivotal role in cellular metabolism:
- Pyruvate: One of the primary end products of glycolysis is pyruvate. From a single glucose molecule, glycolysis generates two molecules of pyruvate. This compound can either enter the citric acid cycle for further oxidation under aerobic conditions or be reduced to lactate in anaerobic conditions.
- ATP (Adenosine Triphosphate): ATP is the primary energy currency of the cell. During glycolysis, a total of four ATP molecules are synthesized. However, it’s essential to note that the glycolytic pathway also consumes two ATP molecules in its initial steps. Therefore, the net gain of ATP from glycolysis is two molecules.
- NADH+H+: Nicotinamide adenine dinucleotide (NAD+) is a coenzyme that plays a crucial role in oxidation-reduction reactions. In glycolysis, NAD+ is reduced to form two molecules of NADH along with protons (H+). These NADH molecules can subsequently donate electrons to the electron transport chain, leading to further ATP production in aerobic conditions.
In summary, glycolysis is a process that:
- Oxidizes glucose to form pyruvate.
- Reduces NAD+ to generate NADH.
- Produces a net gain of two ATP molecules through substrate-level phosphorylation.
These products are vital for the cell’s energy requirements and serve as intermediates for other metabolic pathways.
Production of ATP in glycolysis/Energetics of glycolysis
The energetics of glycolysis provides insight into the ATP yield during the breakdown of glucose. The amount of ATP generated varies depending on the cellular environment, specifically whether it is aerobic or anaerobic.
Aerobic Conditions: Under aerobic conditions, oxygen is present, allowing for the complete oxidation of glucose. In this scenario, additional ATP is produced due to the oxidation of NADH molecules in the mitochondria. Each NADH molecule, when oxidized, contributes to the generation of 2.5 ATP molecules.
Anaerobic Conditions: In the absence of oxygen, glycolysis proceeds anaerobically. Here, there is no net production of NADH, as it is used up in the conversion of pyruvate to lactate. Consequently, the ATP yield is lower compared to aerobic conditions.
The ATP production in various steps of glycolysis can be tabulated as follows:
Table 1: Energetics of Glycolysis under Different Conditions
Steps | Aerobic Conditions | Anaerobic Conditions |
---|---|---|
Step-1 | -1 ATP | -1 ATP |
Step-3 | -1 ATP | -1 ATP |
Step-5 | 2 x NADH = 5 ATP | 2 x NADH = 5 ATP |
Step-6 | 2 x ATP | 2 x ATP |
Step-9 | 2 x ATP | 2 x ATP |
Regeneration of NAD+ | – | -2 x NADH = -5 ATP |
NET ATP | 9 – 2 = 7 ATP | 4 – 2 = 2 ATP |
In summary, while glycolysis under aerobic conditions yields a net of 7 ATP molecules, under anaerobic conditions, the net yield is reduced to 2 ATP molecules. This distinction underscores the significance of oxygen in maximizing energy extraction from glucose.
Glycolysis and shuttle pathways
The NADH that is produced during glycolysis can participate, in the presence of oxygen and mitochondria, in the shuttle pathways to the synthesis ATP. Three ATP molecules can be generated from each NADH molecule if the cytosolic NADH uses the malate-aspartate shuttle. This contrasts with the glycerolphosphate-sea shuttle, which produces only 2 ATP.
Irreversible steps in glycolysis
The majority of reactions that occur during glycolysis can be reversed. The three steps that are catalysed in glycolysis by the enzymes, hexokinase or glucokinase as well as phosphofructokinase, pyruvate and glucokinase are irreversible. These three stages are responsible for controlling glycolysis. With alternate arrangements at the three irreversible steps, the reversal in glycolysis leads to the synthesis from pyruvate of glucose (gluconeogenesis).
Fates of Pyruvate
Pyruvate, the pivotal product of glycolysis, assumes different fates depending on the organism and prevailing metabolic conditions. These pathways are crucial for maintaining energy balance and ensuring the proper functioning of cells. Here are the three essential routes that pyruvate can take:
1. Oxidation of Pyruvate:
- In aerobic organisms, pyruvate undergoes oxidative metabolism.
- Pyruvate is transported into the mitochondria, where it is further processed.
- During this process, pyruvate is converted into acetyl-coenzyme A (acetyl-CoA).
- One molecule of carbon dioxide (CO2) is released during this conversion.
- Acetyl-CoA then enters the citric acid cycle (also known as the Krebs cycle) within the mitochondria.
- In the citric acid cycle, acetyl-CoA is completely oxidized to produce carbon dioxide (CO2) and water (H2O), releasing energy in the form of ATP.
- This pathway is a hallmark of aerobic respiration and follows glycolysis in aerobic organisms and plants.
2. Lactic Acid Fermentation:
- In conditions where oxygen is scarce or insufficient, such as during intense muscle activity in skeletal muscle cells, pyruvate cannot be oxidized due to the lack of oxygen.
- Under such anaerobic conditions, pyruvate is enzymatically reduced to form lactate through a process known as anaerobic glycolysis.
- Lactate production from glucose also occurs in other anaerobic organisms through a similar process, known as lactic acid fermentation.
- This pathway helps regenerate nicotinamide adenine dinucleotide (NAD+) to keep glycolysis running, even in the absence of oxygen.
3. Alcoholic Fermentation:
- Some microbes, such as brewer’s yeast, employ a different anaerobic pathway.
- In these organisms, pyruvate derived from glucose is converted into ethanol (alcohol) and carbon dioxide (CO2).
- This process is referred to as alcoholic fermentation.
- Alcoholic fermentation is often observed in conditions where oxygen concentration is low, making it a vital metabolic pathway in certain microorganisms.
- It is also considered one of the most ancient forms of glucose metabolism.
In summary, the fate of pyruvate is determined by the availability of oxygen and the specific metabolic requirements of the organism or cell. These pathways, whether oxidative or fermentative, play a fundamental role in energy production and maintaining metabolic homeostasis.
Regulation of Glycolysis
Glycolysis, a central metabolic pathway responsible for glucose catabolism, is finely regulated to ensure that the cell meets its energy demands while responding to metabolic conditions. Various mechanisms govern the regulation of glycolysis, and these mechanisms involve enzyme modifications, gene expression, protein-protein interactions, and post-translational modifications. Here are the key regulatory aspects of glycolysis:
1. Allosteric Modification:
- Allosteric regulation plays a pivotal role in controlling glycolysis. Enzymes involved in glycolysis can be modulated by the binding of specific molecules at regulatory sites.
- Allosteric regulation generally follows the principle that substrates stimulate enzyme activity, whereas products inhibit it. When substrate concentrations are high, enzyme activity is stimulated, favoring product formation.
2. Gene Expression:
- The expression of genes coding for glycolytic enzymes can be regulated to control the overall rate of glycolysis. Changes in gene expression can lead to alterations in enzyme levels within the cell, impacting glycolytic flux.
3. Protein-Protein Interaction:
- Protein-protein interactions can influence the activity of glycolytic enzymes. Complexes formed between different enzymes or regulatory proteins can either enhance or inhibit glycolytic reactions.
4. Post-Translational Modification:
- Enzyme activity can be altered through post-translational modifications, such as phosphorylation and dephosphorylation.
- Phosphorylation, in particular, is a common regulatory mechanism. Phosphorylated enzymes are often inactive, while dephosphorylation activates them.
5. Cellular Localization:
- The subcellular localization of glycolytic enzymes can impact their activity. Enzymes may be sequestered in specific cellular compartments or brought into proximity with their substrates.
6. Hormonal Regulation (Insulin and Glucagon in Animals):
- Blood glucose levels are tightly controlled through hormonal regulation, primarily involving insulin and glucagon.
- Low blood glucose levels stimulate the release of glucagon and epinephrine. These hormones activate protein kinase A, which phosphorylates glycolytic enzymes, rendering them inactive. This occurs during fasting states, where glycolysis is inhibited.
- High blood glucose levels trigger the release of insulin, which activates phosphatases. These enzymes dephosphorylate glycolytic enzymes, restoring their activity. Under the influence of insulin, glycolysis is promoted.
Regulation of Rate-Limiting Enzymes:
- Four key enzymes in glycolysis are particularly critical for regulation:
- Glucokinase and Hexokinase: These enzymes catalyze the first step of glycolysis. Glucokinase is inducible and found in specific cells like liver and pancreas, while hexokinase is ubiquitous. Their properties and regulation differ, with glucokinase being induced by insulin and having low affinity for glucose.
- Phosphofructokinase-1 (PFK-1): PFK-1 catalyzes an irreversible step in glycolysis. It is regulated by allosteric effectors, including AMP and fructose-2,6-bisphosphate (F2,6BP). A low insulin/glucagon ratio leads to phosphorylation/inactivation of PFK-1, while high insulin/glucagon ratio results in its activation.
- Pyruvate Kinase: Pyruvate kinase in the liver is phosphorylated and deactivated by epinephrine/glucagon-induced protein kinase A during fasting. In contrast, muscle pyruvate kinase remains unaffected, allowing glycolysis to continue in muscle tissues.
These regulatory mechanisms ensure that glycolysis responds to cellular energy needs and maintains glucose homeostasis. They enable the cell to adapt to various metabolic conditions, optimizing energy production and substrate utilization. Glycolysis regulation is a highly coordinated process essential for overall cellular metabolism.
Substrates Entry Points
Three ways substrates can enter glycolysis pathways are known as “entry points”. These are:
- Dietary glucose – glucose is directly absorbed from the gastrointestinal tract into the bloodstream and enters the pathway.
- Glycogenolysis – Glycogenolysis is when glucose is released from the liver and enters the pathway.
- Other monosaccharides – Galactose, fructose, and other monosaccharides enter the glycolysis pathway at different levels through common intermediates.
Glycogen stored in skeletal muscles cannot be completely broken down into glucose. It cannot be completely broken down into glucose and can therefore only be used to fuel glycolysis in the skeletal muscle cells where it is stored.
Transport into Cells
To allow cells to use circulating glucose, it must move from the bloodstream into the intracellular space. Various transporters (GLUT 1-4) transport glucose into cells. They all have different kinetics, and regulation methods depending on the function of glycolysis.
N.B. For an explanation of the term Km, please see our article on enzyme kinetics.
GLUT transporter | Key feature | Location | Reason |
GLUT-1 | Low Km, so highly active | All cells | Regulates basal uptake even at low glucose levels, ensures a constant supply of energy for survival |
GLUT-2 | Concentration-dependent, due to high Km | Liver, pancreatic islets | Acts as a glucose sensor – increases uptake in high glucose levels for storage. Also regulates insulin release from the pancreas |
GLUT-4 | Insulin-dependent | Muscle, adipose, heart | Increases uptake in presence of insulin (i.e. after meals) for storage |
Phases of Glycolysis
Glycolysis is a two-part process. First, energy is used to produce high-energy intermediates. These intermediates then release their energy in the second phase.
- Energy investment phase – two ATP molecules are required to make high-energy intermediates.
- Energy pay out phase – The intermediate is metabolized, producing four ATP molecules as well as two NADH molecules.
Post-glycolysis Processes
After glycolysis, several crucial post-glycolysis processes take place to regenerate NAD+ for the continuation of glycolysis and to channel metabolites into various metabolic pathways. These processes depend on the availability of oxygen and the metabolic needs of the cell. Here are the key post-glycolysis processes:
1. Anoxic Regeneration of NAD+:
- Lactic Acid Fermentation: In the absence of oxygen, as in anaerobic conditions, NAD+ regeneration occurs through lactic acid fermentation. This process involves the reduction of pyruvate to lactate while converting NADH back to NAD+. Lactic acid fermentation is utilized by organisms like lactic acid bacteria, which play a role in the conversion of milk into yogurt. During intense exercise, human muscles also employ lactic acid fermentation to regenerate NAD+, leading to the buildup of lactic acid and muscle soreness.
- Ethanol Fermentation: Certain yeast organisms use ethanol fermentation under anaerobic conditions. Pyruvate is first converted into acetaldehyde and then into ethanol, effectively regenerating NAD+.
2. Aerobic Regeneration of NAD+ and Disposal of Pyruvate:
- Under aerobic conditions, NAD+ is regenerated more efficiently. NADH generated during glycolysis is transported into the mitochondria through shuttle systems like the malate-aspartate shuttle and the glycerol phosphate shuttle.
- In the mitochondria, NADH donates electrons to the electron transport chain (ETC), a series of electron acceptors located in the inner mitochondrial membrane. Ultimately, these electrons are transferred to oxygen, forming water, and regenerating NAD+. This process also generates a proton gradient, driving ATP synthesis through oxidative phosphorylation.
- Pyruvate, under aerobic conditions, undergoes a series of enzymatic reactions catalyzed by the pyruvate dehydrogenase complex (PDC). This complex converts pyruvate into acetyl CoA, carbon dioxide (CO2), and NADH. Acetyl CoA enters the citric acid cycle (TCA cycle) to generate more ATP.
3. Conversion of Carbohydrates into Fatty Acids and Cholesterol:
- Acetyl CoA produced in the mitochondria from pyruvate serves as a vital link between carbohydrate metabolism, fatty acid synthesis, and cholesterol synthesis.
- Acetyl CoA needs to be transported to the cytosol for fatty acid and cholesterol synthesis. This transfer is achieved through citrate formation within the mitochondria. Citrate is then transported to the cytosol and cleaved into acetyl CoA and oxaloacetate by ATP-citrate lyase. These acetyl CoA molecules are used for fatty acid synthesis or cholesterol synthesis.
4. Conversion of Pyruvate into Oxaloacetate for the Citric Acid Cycle (TCA Cycle):
- Pyruvate generated from glycolysis can be actively converted into oxaloacetate through the action of the pyruvate carboxylase enzyme.
- Oxaloacetate is a critical component of the TCA cycle. It combines with acetyl CoA to initiate the cycle, leading to the generation of ATP and reducing equivalents like NADH and FADH2.
These post-glycolysis processes illustrate the adaptability of cellular metabolism to varying oxygen levels and metabolic demands. The regulation of these processes ensures that the cell can efficiently generate energy and produce essential molecules under different conditions.
Importance of Glycolysis
Glycolysis is a fundamental and essential metabolic pathway that holds immense importance for various reasons. Here are some of the key reasons why glycolysis is vital for cells and organisms:
- Energy Production: Glycolysis is a primary source of energy production, especially when oxygen availability is limited or absent (anaerobic conditions). It converts glucose into smaller molecules of pyruvate, producing a small amount of ATP along the way. This ATP is crucial for powering various cellular processes.
- Universal Process: Glycolysis is present in almost all living organisms, from bacteria to humans. It’s a fundamental process that has been conserved throughout evolution, highlighting its importance for life.
- Energy Generation in Anaerobic Conditions: In situations where oxygen is scarce, such as during intense exercise or in certain microenvironments within tissues, glycolysis becomes the main pathway for energy production. It enables cells to keep functioning even when oxygen supply is insufficient for aerobic respiration.
- Carbon Skeleton Precursors: Glycolysis also produces intermediate molecules that can be used for other metabolic pathways. For instance, some of the carbon skeletons generated during glycolysis can be used for the synthesis of amino acids, nucleotides, and fatty acids.
- NADH Production: Glycolysis generates NADH molecules, which serve as carriers of high-energy electrons. NADH can be further used in the electron transport chain during aerobic respiration to produce additional ATP.
- Regulation of Blood Glucose: Glycolysis plays a role in regulating blood glucose levels. When blood glucose levels are high, excess glucose can enter cells and undergo glycolysis, helping to maintain blood glucose homeostasis.
- Emergency Energy Source: In times of stress or during situations where other energy sources are limited, glycolysis provides a quick and immediate source of energy.
- Cancer Metabolism: Cancer cells often rely heavily on glycolysis, even in the presence of oxygen (aerobic glycolysis or the Warburg effect). This altered metabolism helps cancer cells meet their high energy demands for rapid growth and proliferation.
- Drug Targets: Several enzymes in the glycolytic pathway are potential targets for drug development. Targeting these enzymes can impact the energy metabolism of cancer cells, offering potential avenues for cancer therapy.
- Cell Signaling: Some glycolytic intermediates also act as signaling molecules that influence various cellular processes, such as gene expression and cell growth.
In summary, glycolysis is a central metabolic pathway that serves as a primary energy source, especially in situations where oxygen is limited. Its significance spans across various organisms and cellular functions, from providing energy to influencing cellular processes and even playing a role in disease processes.
Structure of glycolysis components in Fischer projections and polygonal model
Fischer projections depict the chemical changes that occur in glycolysis intermediates. This image can be used to compare with the polygonal model representation. A video shows another comparison of Fischer projections with Poligonal Models in glycolysis. You can also see video animations on YouTube for another metabolic pathway (Krebs Cycle), and for the representation and application of Polygonal Models in Organic Chemistry.
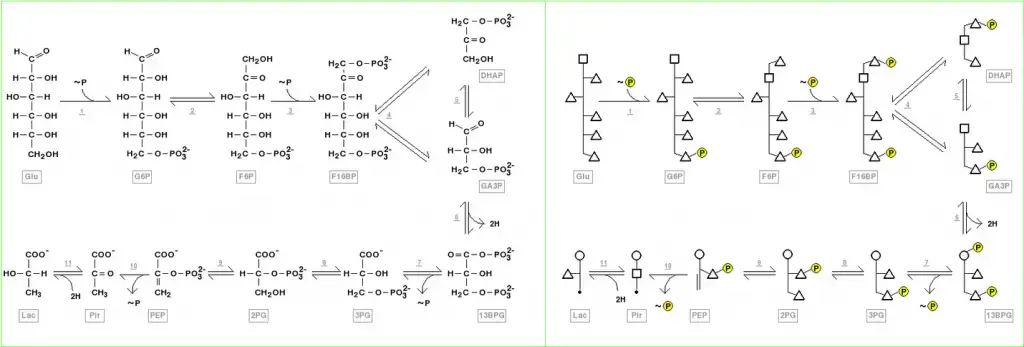
Interactive pathway map
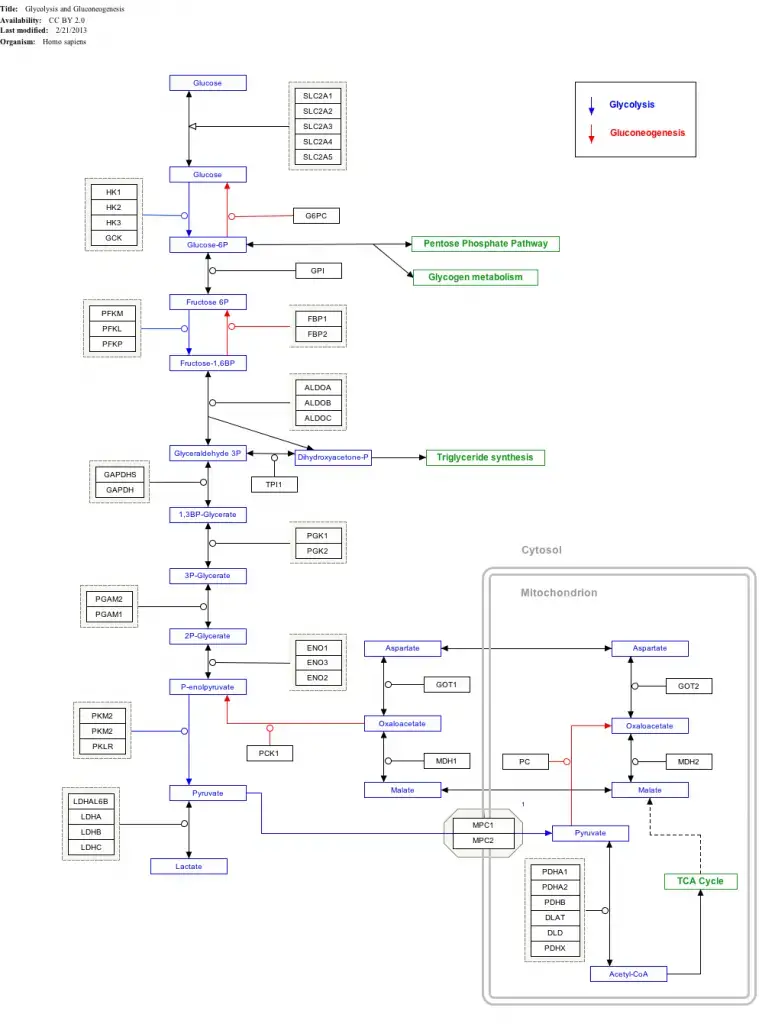
Glycolysis in disease
Defects in the glycolytic pathway have been associated with various diseases, including diabetes, genetic disorders, and cancer, underscoring the pivotal role of glycolysis in cellular metabolism and health.
1. Diabetes: Diabetes is a complex metabolic disorder characterized by the body’s inability to produce or properly respond to insulin, a hormone crucial for glucose regulation. In individuals with diabetes, defects in glycolysis may contribute to the disease’s pathogenesis. Specifically:
- Insulin Insufficiency: In type 1 diabetes, autoimmune destruction of pancreatic beta cells can lead to a deficiency in insulin production. This deficiency can disrupt glucose uptake by cells, resulting in elevated blood glucose levels (hyperglycemia).
- Insulin Resistance: In type 2 diabetes, cells become resistant to the effects of insulin, impairing glucose uptake. Dysregulation in glycolytic pathways may play a role in insulin resistance, contributing to hyperglycemia.
2. Genetic Diseases: Genetic disorders associated with defects in genes encoding glycolytic enzymes can manifest as metabolic diseases. Examples include:
- Pyruvate Kinase Deficiency: This metabolic disorder results from mutations in the PKLR gene, leading to reduced pyruvate kinase activity. This deficiency affects glycolysis by impairing the conversion of phosphoenolpyruvate (PEP) to pyruvate.
- Hyperinsulinemic Hypoglycemia: This disorder arises from mutations in the GCK gene, affecting glucokinase production in pancreatic beta cells. Glucokinase is essential for glucose sensing and insulin release.
- Glycogen Storage Diseases (GSDs): Certain GSDs, like GSD type XII, involve defects in enzymes related to glycolysis. For example, GSD type XII results from mutations in the ALDOA gene, affecting fructose-bisphosphate aldolase, a glycolytic enzyme.
- Other Enzyme Deficiencies: Genetic mutations can affect enzymes such as phosphofructokinase (PFK), glucose-6-phosphate isomerase (GPI), triosephosphate isomerase (TPI), and phosphoglycerate mutase (PGAM), leading to various metabolic disorders.
3. Cancer: Emerging research has highlighted the altered glycolytic metabolism in cancer cells, a phenomenon known as the Warburg effect. Cancer cells often exhibit enhanced glucose consumption and reliance on glycolysis for energy production, even in the presence of oxygen (aerobic glycolysis). The implications of glycolysis in cancer include:
- Increased Glucose Utilization: Cancer cells have a significantly higher glucose uptake compared to normal cells, potentially supporting their rapid growth and proliferation.
- Warburg Effect: The preference for glycolysis in cancer cells may be due to the need for rapid ATP generation and metabolic intermediates for biosynthesis.
- Targeting Cancer Metabolism: Research is ongoing to explore the therapeutic potential of targeting glycolysis as a way to disrupt the energy supply to cancer cells and inhibit their growth. However, this area of study continues to evolve, and more research is needed for a comprehensive understanding of the role of glycolysis in cancer.
In summary, glycolysis plays a fundamental role in both health and disease. Defects in this metabolic pathway can contribute to the pathogenesis of diseases such as diabetes and genetic disorders. Additionally, the altered glycolytic metabolism observed in cancer cells has sparked interest in potential therapeutic strategies targeting cancer metabolism. Further research is essential to deepen our understanding of these complex relationships and develop effective treatments.
Intermediates for other pathways
The glycolytic pathway, which converts glucose into pyruvate, generates various glycolytic intermediates that play critical roles in a wide range of metabolic pathways. These intermediates serve as versatile building blocks for other metabolic processes, highlighting the interconnectedness of cellular metabolism. Here are some essential glycolytic intermediates and their roles in various metabolic pathways:
- Glucose 6-phosphate (G6P):
- G6P is the first intermediate in glycolysis, and it can also serve as a precursor for other metabolic pathways.
- In the Pentose Phosphate Pathway (PPP), G6P is a starting point for the production of NADPH, which is crucial for antioxidant defense and biosynthetic processes.
- G6P can be converted into glycogen for storage in the liver and muscles or used in the synthesis of nucleic acids.
- Fructose 6-phosphate (F6P):
- F6P, the second glycolytic intermediate, can also participate in the Pentose Phosphate Pathway (PPP) to produce NADPH and ribose-5-phosphate for nucleotide synthesis.
- In photosynthetic organisms, it is involved in starch synthesis within chloroplasts.
- Glyceraldehyde 3-phosphate (G3P):
- G3P, a key intermediate in glycolysis, is a versatile molecule with multiple metabolic roles.
- In gluconeogenesis, G3P is used to synthesize glucose when blood sugar levels are low.
- In photosynthesis, two G3P molecules can combine to form glucose, facilitating carbon fixation and energy storage in plants.
- G3P is also involved in the biosynthesis of thiamine (vitamin B1).
- Dihydroxyacetone phosphate (DHAP):
- DHAP, formed alongside G3P, can be converted to G3P through the action of triosephosphate isomerase, allowing it to reenter the glycolytic pathway.
- Alternatively, DHAP participates in other metabolic pathways, such as glycogen metabolism and lipid biosynthesis.
- 1,3-bisphosphoglycerate (1,3BPG):
- 1,3BPG is generated during glycolysis and can serve different functions in various organisms.
- In plants, it plays a role in the Calvin cycle, contributing to carbon fixation and the production of glucose.
- In humans, a portion of 1,3BPG follows an alternate route known as the Luebering-Rapoport pathway, ultimately influencing oxygen release from red blood cells.
- 3-phosphoglycerate (3PG):
- 3PG is an intermediate formed by dephosphorylating 1,3BPG in glycolysis.
- In the Calvin cycle of photosynthesis, 3PG is phosphorylated to regenerate 1,3BPG, facilitating the fixation of carbon dioxide.
- Phosphoenolpyruvate (PEP):
- PEP is a high-energy intermediate produced by converting 2-phosphoglycerate in glycolysis.
- In glycolysis, PEP plays a crucial role in the final substrate-level phosphorylation step, producing pyruvate.
- PEP is also involved in the shikimate pathway, contributing to the synthesis of amino acids, vitamins, and plant hormones in various organisms.
- In C4 plants, PEP is essential for carbon dioxide fixation in the C4 carbon fixation pathway, which reduces photorespiration and enhances carbon capture efficiency.
In summary, glycolytic intermediates are pivotal molecules in cellular metabolism, serving as substrates, precursors, and contributors to numerous metabolic pathways. Their diverse roles highlight the complexity and adaptability of metabolic networks, allowing organisms to meet their energy and biosynthetic demands under varying conditions.
Change in free energy for each step of glycolysis
Step | Reaction | ΔG°’ / (kJ/mol) | ΔG / (kJ/mol) |
---|---|---|---|
1 | Glucose + ATP4− → Glucose-6-phosphate2− + ADP3− + H+ | −16.7 | −34 |
2 | Glucose-6-phosphate2− → Fructose-6-phosphate2− | 1.67 | −2.9 |
3 | Fructose-6-phosphate2− + ATP4− → Fructose-1,6-bisphosphate4− + ADP3− + H+ | −14.2 | −19 |
4 | Fructose-1,6-bisphosphate4− → Dihydroxyacetone phosphate2− + Glyceraldehyde-3-phosphate2− | 23.9 | −0.23 |
5 | Dihydroxyacetone phosphate2− → Glyceraldehyde-3-phosphate2− | 7.56 | 2.4 |
6 | Glyceraldehyde-3-phosphate2− + Pi2− + NAD+ → 1,3-Bisphosphoglycerate4− + NADH + H+ | 6.30 | −1.29 |
7 | 1,3-Bisphosphoglycerate4− + ADP3− → 3-Phosphoglycerate3− + ATP4− | −18.9 | 0.09 |
8 | 3-Phosphoglycerate3− → 2-Phosphoglycerate3− | 4.4 | 0.83 |
9 | 2-Phosphoglycerate3− → Phosphoenolpyruvate3− + H2O | 1.8 | 1.1 |
10 | Phosphoenolpyruvate3− + ADP3− + H+ → Pyruvate− + ATP4− | −31.7 | −23.0 |
Concentrations of metabolites in erythrocytes
Compound | Concentration / mM |
---|---|
Glucose | 5.0 |
Glucose-6-phosphate | 0.083 |
Fructose-6-phosphate | 0.014 |
Fructose-1,6-bisphosphate | 0.031 |
Dihydroxyacetone phosphate | 0.14 |
Glyceraldehyde-3-phosphate | 0.019 |
1,3-Bisphosphoglycerate | 0.001 |
2,3-Bisphosphoglycerate | 4.0 |
3-Phosphoglycerate | 0.12 |
2-Phosphoglycerate | 0.03 |
Phosphoenolpyruvate | 0.023 |
Pyruvate | 0.051 |
ATP | 1.85 |
ADP | 0.14 |
Pi | 1.0 |
How to Remember the Enzyme Names?
Remembering enzyme names can be challenging, but using mnemonic devices or associations can make it easier. Here’s a simple mnemonic that you can use to remember the enzymes involved in glycolysis:
“Hannah’s Pretty Pink Apron Turns Gracefully, Making Pies Perfectly.”
Each word in this mnemonic corresponds to the first letter of an enzyme’s name:
- Hannah’s (Hexokinase)
- Pretty (Phosphofructokinase)
- Pink (Phosphoglycerate Kinase)
- Apron (Aldolase)
- Turns (Triose Phosphate Isomerase)
- Gracefully (Glyceraldehyde-3-Phosphate Dehydrogenase)
- Making (Enolase)
- Pies (Pyruvate Kinase)
- Perfectly (Phosphoglycerate Mutase)
You can use this mnemonic to help you recall the enzyme names in the correct order of their appearance in the glycolytic pathway.
Additionally, creating associations or mental images related to the enzyme names or their functions can help reinforce your memory. For instance, you might visualize “Hannah” using a hexagon-shaped cookie cutter (like glucose is a hexagon), “Pretty Pink” could remind you of the pink color of ATP molecules (from ATP production), and so on.
Feel free to create your own associations or mnemonics that make sense to you. Associating enzyme names with memorable images or stories can make learning and recalling them much more enjoyable and effective.
How to Remember Each Step of Glycolysis Serially? Here is 5 Shortcut
here are five mnemonic shortcuts to help you remember the steps of glycolysis in order:
1. “Good People Always Drink Green Tea After Cold Play.”
- Each word corresponds to the first letter of a step in glycolysis:
- Good (Glucose Entry)
- People (Preparation Phase)
- Always (ATP and Pyruvate Formation)
- Drink (Dihydroxyacetone Phosphate Conversion)
- Green (Glyceraldehyde-3-Phosphate Dehydrogenase)
- Tea (Triose Phosphate Isomerase)
- After (ATP Generation – Substrate-Level Phosphorylation)
- Cold (Cleavage Phase)
- Play (Pyruvate Formation – Final Energy-Payoff Phase)
2. “Glorious People Are Also Daring, Diverse, and Generous To People.”
- This mnemonic uses the first letter of each word to represent a step:
- Glorious (Glucose Entry)
- People (Preparation Phase)
- Are (ATP and Pyruvate Formation)
- Also (Dihydroxyacetone Phosphate Conversion)
- Daring (Glyceraldehyde-3-Phosphate Dehydrogenase)
- Diverse (Triose Phosphate Isomerase)
- Generous (ATP Generation – Substrate-Level Phosphorylation)
- To (Cleavage Phase)
- People (Pyruvate Formation – Final Energy-Payoff Phase)
3. “Great Pyramids Allow Tigers Down Ancient Canyons, Proclaiming Perfectly.”
- This mnemonic maps each word to a glycolysis step:
- Great (Glucose Entry)
- Pyramids (Preparation Phase)
- Allow (ATP and Pyruvate Formation)
- Tigers (Dihydroxyacetone Phosphate Conversion)
- Down (Glyceraldehyde-3-Phosphate Dehydrogenase)
- Ancient (ATP Generation – Substrate-Level Phosphorylation)
- Canyons (Cleavage Phase)
- Proclaiming (Phosphoglycerate Mutase)
- Perfectly (Pyruvate Formation – Final Energy-Payoff Phase)
4. “Girls Play All Day, Dance, Dance, Till Friday’s Party.”
- Each word corresponds to a glycolysis step:
- Girls (Glucose Entry)
- Play (Preparation Phase)
- All (ATP and Pyruvate Formation)
- Day (Dihydroxyacetone Phosphate Conversion)
- Dance (Dihydroxyacetone Phosphate Isomerization)
- Dance (ATP Generation – Substrate-Level Phosphorylation)
- Till (Triose Phosphate Isomerase)
- Friday’s (Phosphoglycerate Kinase)
- Party (Pyruvate Formation – Final Energy-Payoff Phase)
5. “Going Places Always Demands Drama, Disguises, and Pretty Performances.”
- Use the first letter of each word to remember the steps:
- Going (Glucose Entry)
- Places (Preparation Phase)
- Always (ATP and Pyruvate Formation)
- Demands (Dihydroxyacetone Phosphate Conversion)
- Drama (Dihydroxyacetone Phosphate Isomerization)
- Disguises (ATP Generation – Substrate-Level Phosphorylation)
- and (Aldolase Cleavage)
- Pretty (Phosphoglycerate Kinase)
- Performances (Pyruvate Formation – Final Energy-Payoff Phase)
Choose the mnemonic that works best for you or create your own using associations that resonate with you. Associating each step with a memorable image or story can help reinforce your memory of the glycolytic pathway.
Quiz Practice
- Which molecule serves as the starting substrate for glycolysis?
a. Glucose
b. Pyruvate
c. Acetyl CoA
d. Lactate
- How many molecules of ATP are consumed during the initial energy-investment phase of glycolysis?
a. 1
b. 2
c. 3
d. 4
- In glycolysis, glucose is converted into two molecules of what three-carbon compound?
a. G3P
b. 1,3-BPG
c. Pyruvate
d. Acetyl CoA
- Which of the following molecules is NOT produced during glycolysis?
a. NADH
b. ATP
c. FADH2
d. Pyruvate
- Under anaerobic conditions, pyruvate is converted into which compound to regenerate NAD+ and allow glycolysis to continue?
a. Lactate
b. Acetyl CoA
c. Ethanol
d. Citrate
- What is the net gain of ATP molecules produced directly from glycolysis (excluding the initial ATP consumption)?
a. 1 ATP
b. 2 ATP
c. 3 ATP
d. 4 ATP
- In glycolysis, which enzyme catalyzes the conversion of fructose-6-phosphate to fructose-1,6-bisphosphate?
a. Phosphofructokinase-1 (PFK-1)
b. Hexokinase
c. Pyruvate kinase
d. Aldolase
- What is the end product of glycolysis in the absence of oxygen when pyruvate cannot enter the citric acid cycle?
a. Acetyl CoA
b. Lactate
c. Ethanol
d. Citrate
- Which of the following is NOT a regulatory enzyme in glycolysis?
a. Glucokinase
b. Phosphofructokinase-1 (PFK-1)
c. Pyruvate kinase
d. Aldolase
- In glycolysis, what is the overall outcome in terms of ATP and NADH production from one molecule of glucose?
a. 2 ATP and 2 NADH
b. 4 ATP and 2 NADH
c. 2 ATP and 4 NADH
d. 4 ATP and 4 NADH
FAQ
Where does glycolysis take place?
Glycolysis takes place in the cytoplasm of cells.
What is aerobic glycolysis?
Aerobic glycolysis is the process of oxidation of glucose into pyruvate followed by the oxidation of pyruvate into CO2 and H2O in the presence of a sufficient amount of oxygen.
What is anaerobic glycolysis?
Anaerobic glycolysis is the process that takes place in the absence of enough oxygen resulting in the reduction of pyruvate into lactate and reoxidation of NADH into NAD+.
does glycolysis require oxygen?
Glycolysis requires no oxygen. It is an anaerobic type of respiration performed by all cells, including anaerobic cells that are killed by oxygen.
is glycolysis aerobic or anaerobic?
Glycolysis is an anaerobic process. None of its nine steps involve the use of oxygen.
how many nadh are produced by glycolysis?
Two NADH molecules are produced by glycolysis.
what does glycolysis produce?
Glucose is the source of almost all energy used by cells. Overall, glycolysis produces two pyruvate molecules, a net gain of two ATP molecules, and two NADH molecules.
in glycolysis, atp molecules are produced by _.a. photophosphorylationb. oxidative phosphorylationc. photosynthesisd. cellular respiratione. substrate-level phosphorylation
Ans: e. substrate-level phosphorylation.
For each glucose that enters glycolysis, _____ acetyl coa enter the citric acid cycle.
For each glucose that enters glycolysis, 2 acetyl CoA enter the citric acid cycle.
why is atp required for glycolysis?
Energy is needed at the start of glycolysis to split the glucose molecule into two pyruvate molecules. These two molecules go on to stage II of cellular respiration. The energy to split glucose is provided by two molecules of ATP.
in addition to atp, what are the end products of glycolysis?
The end products of glycolysis are: pyruvic acid (pyruvate), adenosine triphosphate (ATP), reduced nicotinamide adenine dinucleotide (NADH), protons (hydrogen ions (H2+)), and water (H2O). Glycolysis is the first step of cellular respiration, the process by which a cell converts nutrients into energy.
how many atp are produced in glycolysis?
Glycolysis produces 2 ATP
which kind of metabolic poison would most directly interfere with glycolysis?
A poison that closely mimics the structure of glucose but is not metabolized. If the poison cannot be metabolized, then no NADH, pyruvate or ATP will be produced in glycolysis.
where does glycolysis take place in eukaryotic cells?
Glycolysis takes place in the cytoplasm. Within the mitochondrion, the citric acid cycle occurs in the mitochondrial matrix, and oxidative metabolism occurs at the internal folded mitochondrial membranes (cristae).
why is glycolysis considered to be one of the first metabolic pathways to have evolved?
Photosynthesis is one of the earliest reactions where carbon dioxide and water come together to form glucose. In glucose the energy of the sun is trapped. Glycolysis breaks down glucose molecules in carbon dioxide and water. Breaking down glucose releases energy.
Most of the cells respire anaerobically. All these cells have glycolysis in their metabolic pathway. Therefore it is one of the earliest metabolic pathways
References
- A. Hassan, Baydaa. (2019). glycolysis Embden–Meyerhof–Parnas (EMP) pathway. 10.13140/RG.2.2.36127.82089.
- Protasoni, Margherita & Zeviani, Massimo. (2021). Mitochondrial Structure and Bioenergetics in Normal and Disease Conditions. International Journal of Molecular Sciences. 22. 586. 10.3390/ijms22020586.
- Fernie, A. R., Carrari, F., & Sweetlove, L. J. (2004). Respiratory metabolism: glycolysis, the TCA cycle and mitochondrial electron transport. Current opinion in plant biology, 7(3), 254–261. https://doi.org/10.1016/j.pbi.2004.03.007
- Granner, D. K., & Rodwell, V. W. (2006). Harper’s illustrated biochemistry (27th ed.). New York: Lange Medical Books/McGraw-Hill.
- Rath, L. (2019, February 20). Cancer and Sugar: Is There a Link? WebMD; WebMD. https://www.webmd.com/cancer/features/cancer-sugar-link
- Bailey, R. (2022). Learn About The 10 Steps of Glycolysis. ThoughtCo. Retrieved 2 May 2022, from https://www.thoughtco.com/steps-of-glycolysis-373394.
- Nelson, D., Lehninger, A., Cox, M., & Nelson, D. (2005). Lecture notebook for Lehninger principles of biochemistry, fourth edition (pp. 522-535). W.H. Freeman.
- (2022). Retrieved 3 May 2022, from https://www.cliffsnotes.com/study-guides/biology/biochemistry-i/glycolysis/glycolysis-regulation.