What is Freeze Fracture Technique?
- The freeze fracture technique, first developed by Hans Moor in 1961, is a pivotal method in cell biology used to study the ultrastructure of biological specimens, particularly cell membranes. This technique involves freezing a sample, then fracturing it to expose the inner structures, which are then replicated and examined using transmission electron microscopy (TEM).
- To begin the process, the biological sample is rapidly frozen to immobilize its cellular components. This freezing is done in the presence of cryoprotective agents such as glycerol, which help prevent the formation of ice crystals that could damage the structure of the specimen. Once frozen, the sample is fractured under high vacuum at liquid nitrogen temperatures. This fracture usually occurs along natural lines of weakness within the cell, such as the plasma membrane or or the surfaces of organelles, creating two distinct faces: the PF-face (plasma fracture-face) and the EF-face (extracellular fracture-face).
- Following the fracture, the exposed surfaces are shadowed with platinum vapor at an angle to add three-dimensional detail to the surface. Carbon is then evaporated onto the sample vertically, forming a carbon-metal replica of the fractured surface. After the organic material is digested away, the resulting carbon replica is mounted onto an electron microscope (EM) grid for detailed examination.
- The freeze-fracture method provides a unique representation of the structural and functional elements of cell membranes. The electron beam used in TEM passes through the carbon portions of the replica while being absorbed by the platinum areas, producing high-resolution, three-dimensional images. These images allow scientists to study the intricate details of cell membranes in ways that traditional sectioning methods cannot.
- One of the critical applications of the freeze-fracture technique is its ability to visualize the arrangement of proteins and other molecules within the membrane, contributing to our understanding of membrane function. Additionally, this method is used in various areas of cell biology and nanotechnology, offering insights into molecular structures and materials science.
- The freeze-fracture technique has therefore been instrumental in advancing our knowledge of cellular structure and function, especially in membrane biology, and continues to be a valuable tool in scientific research.
Principle of Freeze Fracture Technique
The principle of the freeze-fracture technique is based on the physical separation of biological membranes at their hydrophobic core when the sample is frozen and then fractured. Here are the key aspects of this principle:
- Fracture Plane: When a frozen biological sample is fractured, the fracture tends to occur along the plane that bisects the lipid bilayer of membranes. This is due to the inherent structural properties of the membranes, which have a hydrophobic core. The fracture plane typically follows the central hydrophobic region, splitting the membrane into two leaflets.
- Visualization of Membrane Structures: The resulting fracture exposes the internal surfaces of the membranes, allowing for the visualization of integral membrane proteins as intramembrane particles. This provides a unique perspective on the organization and distribution of these proteins within the membrane plane.
- Replica Formation: After fracturing, a thin layer of metal (usually platinum and carbon) is deposited onto the exposed surfaces to create a replica. This replica captures the fine details of the membrane structure, which can then be examined under a transmission electron microscope (TEM).
- Minimal Artifacts: Because the technique involves rapid freezing and minimal chemical treatment, it preserves the native state of the cellular structures better than many other methods. This allows for a more accurate representation of the biological sample’s ultrastructure.
Overall, the freeze-fracture technique provides valuable insights into the functional morphology of cells and has significantly advanced our understanding of membrane organization and dynamics in cell biology.
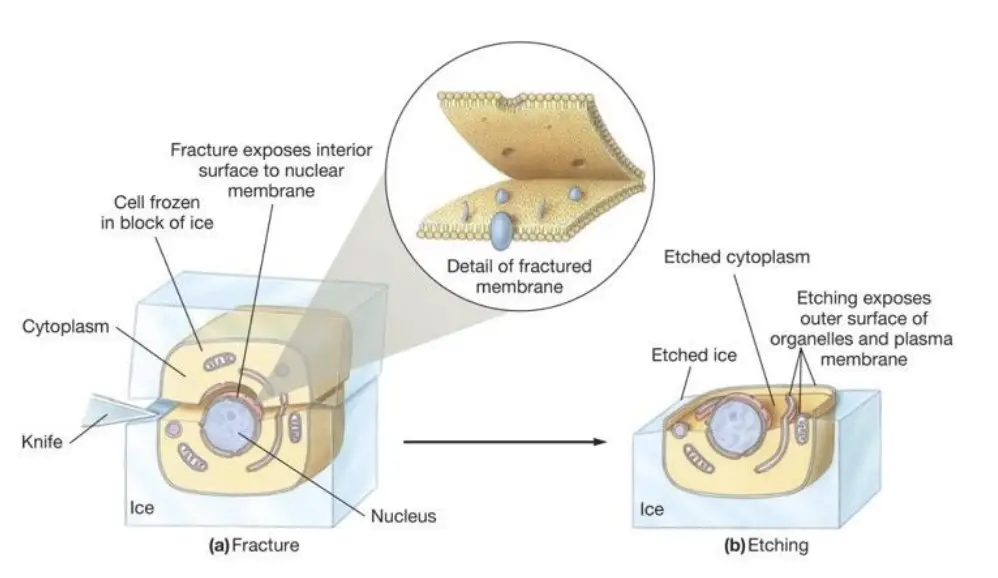
Requirement for Freeze Fracture Technique
REAGENTS
The reagents required for the freeze-fracture technique are crucial for preparing biological samples and ensuring the preservation of their ultrastructural integrity. Here are the key reagents typically used:
- Glutaraldehyde Fixative:
- Concentration: 0.5% to 2.0% (EM grade).
- Purpose: Used for chemical fixation of the biological specimen to preserve cellular structures before freezing. It helps to stabilize proteins and other macromolecules.
- Source: Commonly available from suppliers like Agar Scientific Ltd.
- Buffer Solutions:
- Phosphate Buffered Saline (PBS) or Sodium Cacodylate Buffer:
- Purpose: These buffers are used to dilute glutaraldehyde and maintain the pH during fixation. They help to maintain the physiological conditions of the sample.
- Cryoprotectants:
- Glycerol:
- Purpose: Used to protect the sample from ice crystal damage during the rapid freezing process. It helps to stabilize the structure of the biological material.
- Optional Reagents:
- Other Cryoprotectants: Depending on the specific requirements, other cryoprotectants may be used, but glycerol is the most common.
- Detergents: In some protocols, mild detergents may be used to help permeabilize membranes or remove specific components, although this is less common in standard freeze-fracture protocols.
- Cleaning Agents:
- After replication, cleaning agents may be required to remove any residual biological material from the metal replicas before examination under the electron microscope.
- Safety Precautions:
- Personal Protective Equipment (PPE): Gloves, goggles, and lab coats should be worn when handling glutaraldehyde and other reagents due to their toxic nature.
EQUIPMENT
Here are the key pieces of equipment typically used:
- Rapid Freezing Apparatus:
- Liquid Nitrogen or Propane Freezing System: This equipment is used for the rapid immersion freezing of biological samples. It allows for quick cooling to prevent ice crystal formation, which can damage cellular structures.
- Freeze-Fracture Machine:
- A specialized machine designed for the freeze-fracture process. This machine typically includes features for:
- Fracturing: Controlled fracturing of the frozen samples.
- Vacuum Chamber: To create a vacuum environment necessary for metal deposition.
- A specialized machine designed for the freeze-fracture process. This machine typically includes features for:
- Metal Deposition System:
- Sputter Coater or Vacuum Evaporator: This equipment is used to deposit a thin layer of metal (usually platinum and carbon) onto the fractured surfaces to create replicas for electron microscopy.
- Transmission Electron Microscope (TEM):
- A high-resolution microscope used to examine the metal replicas. The TEM allows researchers to visualize the fine details of the cellular structures captured in the replicas.
- Sample Mounting Devices:
- Various types of carriers or holders for mounting the biological samples during the freezing and fracturing processes. These devices ensure that the samples are securely positioned for optimal results.
- Cryogenic Storage:
- Cryogenic Freezer: For storing samples at low temperatures before and after the freezing process to maintain their integrity.
- Cleaning Equipment:
- Tools and solutions for cleaning the metal replicas after the replication process to remove any residual biological material.
- Personal Protective Equipment (PPE):
- Safety gear such as gloves, goggles, and lab coats to protect users from hazardous materials, especially when handling toxic reagents like glutaraldehyde and during the use of cryogenic materials.
Preparation of the freeze-fracture machine before a run
Preparing the freeze-fracture machine before a run is crucial to ensure optimal performance and successful results. Below are the steps typically involved in preparing the freeze-fracture machine:
Preparation of the Freeze-Fracture Machine Before a Run
- Open the Vacuum Chamber:
- Open the door to the vacuum chamber of the freeze-fracture machine to access the internal components.
- Swivel Gun Mounting Arc:
- Swivel the gun mounting arc outwards to allow for easy access to the evaporation guns.
- Disconnect Evaporation Leads:
- Unplug the evaporation leads from the guns to ensure safety during maintenance.
- Remove Electron Beam Evaporation Guns:
- Unscrew the electron beam evaporation guns from the gun mounting arc for inspection and cleaning.
- Check Connectors:
- Inspect the condition of the connectors to the leads. Ensure they are clean and free from any debris.
- Clean Connectors:
- Blow out any loose flakes of carbon from inside the plugs and sockets. Use applicator sticks with acetone-dampened tissue to remove dirt from the connectors.
- Remove Upper Cathode Cover:
- For each evaporation gun, pull off the detachable outer guard and remove the upper cathode cover by unscrewing the screws that hold it in place.
- Inspect and Clean the Guns:
- Check the condition of the evaporation guns for any signs of wear or contamination. Clean the internal components if necessary.
- Reassemble the Guns:
- Once cleaned and inspected, reassemble the evaporation guns and securely attach them back to the gun mounting arc.
- Close the Vacuum Chamber:
- After all components are checked and reassembled, close the vacuum chamber door securely.
- Start Pumping:
- Begin the vacuum pumping process to achieve a good vacuum. This may take at least 3 hours and is often left overnight for optimal conditions.
- Final Checks:
- Perform final checks to ensure that all systems are functioning correctly and that the machine is ready for the freeze-fracture run.
Protocol for Freeze Fracture Technique
- Sample Preparation:
- Fixation: Immerse the biological samples in a glutaraldehyde solution (0.5% to 2.0%) in PBS or sodium cacodylate buffer for a specified time (typically 1-2 hours) at room temperature. This step preserves the cellular structure.
- Washing: Rinse the samples thoroughly with buffer to remove excess fixative.
- Cryoprotection:
- Glycerol Treatment: Incubate the fixed samples in a solution of glycerol (typically 10-30% in buffer) for a period (e.g., 30 minutes to 1 hour) to protect against ice crystal formation during freezing.
- Rapid Freezing:
- Mounting: Place the samples in suitable carriers for freezing.
- Freezing: Quickly immerse the mounted samples in liquid nitrogen or propane to achieve rapid freezing. This step is crucial to prevent ice crystal damage.
- Fracturing:
- Fracturing in the Freeze-Fracture Machine: Transfer the frozen samples to the freeze-fracture machine. Under vacuum, the samples are fractured at low temperatures, exposing internal structures.
- Metal Replication:
- Metal Deposition: After fracturing, the exposed surfaces are coated with a thin layer of platinum and carbon using a vacuum deposition system. This creates a replica of the fractured surfaces.
- Cleaning the Replica:
- Cleaning: Remove any residual biological material from the metal replicas using appropriate cleaning agents. This step is essential for clear visualization under the electron microscope.
- Mounting for Electron Microscopy:
- Mounting: Carefully mount the cleaned replicas on suitable grids for examination.
- Examination:
- TEM Analysis: Use a transmission electron microscope to examine the metal replicas. Analyze the structural details of the biological samples.
Results and Interpretation for Freeze Fracture Technique
The results and interpretations derived from this technique are crucial for understanding cellular and molecular biology.
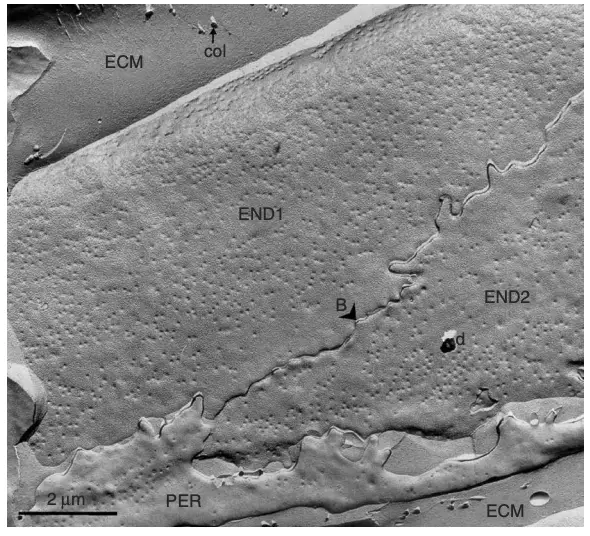
muscle. | Image Source: Severs, N. J. (2007). Freeze-fracture electron microscopy. Nature Protocols
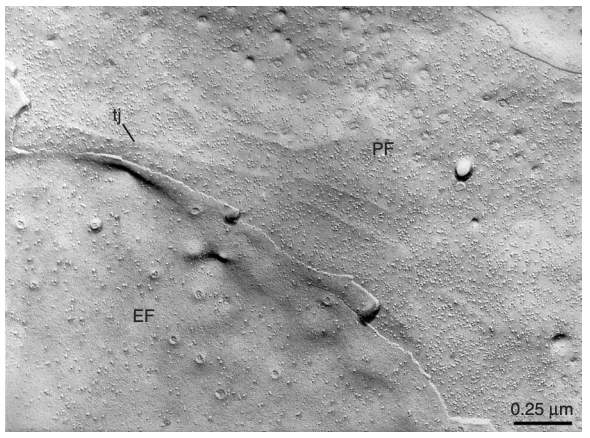
membrane structure of adjacent endothelial cells. | Image Source: Severs, N. J. (2007). Freeze-fracture electron microscopy. Nature Protocols
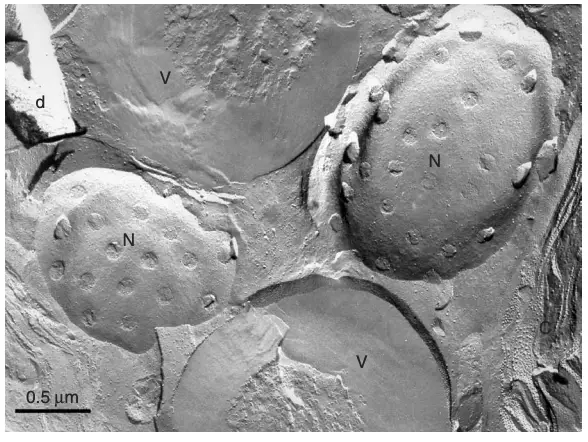
The results obtained from the freeze-fracture technique provide valuable insights into the structural organization of biological membranes and cellular components. Here’s an overview of the expected results and their interpretation:
Results and Interpretation for Freeze Fracture Technique
1. Visualizing Membrane Structures:
- Fracture Faces: The freeze-fracture technique reveals two distinct fracture faces: the E-face (extracellular face) and the P-face (protoplasmic face). The E-face typically shows the outer leaflet of the lipid bilayer, while the P-face reveals the inner leaflet.
- Interpretation: The presence of specific structures, such as proteins, can be inferred from the morphology observed on these faces. For example, integral membrane proteins may appear as particles or aggregates on the fracture faces.
2. Protein Distribution:
- Particle Density: The density and distribution of particles (proteins) on the fracture faces can indicate the organization and clustering of membrane proteins.
- Interpretation: High particle density may suggest areas of protein aggregation or clustering, which can be important for understanding membrane function, signaling, and interactions.
3. Membrane Domains:
- Rafts and Domains: The technique can reveal the presence of lipid rafts or membrane domains, which are specialized regions within the membrane.
- Interpretation: The identification of these domains can provide insights into membrane fluidity, protein interactions, and the functional compartmentalization of cellular processes.
4. Cytoskeletal Interactions:
- Cytoskeletal Elements: Freeze-fracture can also expose cytoskeletal elements that are associated with the membrane.
- Interpretation: Observing these interactions can help elucidate the role of the cytoskeleton in maintaining membrane integrity and facilitating cellular processes such as migration and division.
5. Comparative Analysis:
- Experimental Conditions: By comparing freeze-fracture replicas from different experimental conditions (e.g., treated vs. untreated cells), researchers can assess the impact of specific treatments on membrane structure.
- Interpretation: Changes in the appearance or distribution of membrane components can indicate alterations in cellular function, signaling pathways, or responses to external stimuli.
6. Quantitative Analysis:
- Image Analysis: Advanced imaging techniques and software can be used to quantify the density and distribution of particles on the fracture faces.
- Interpretation: Statistical analysis of these data can provide robust conclusions about the biological significance of observed changes in membrane structure.
Applications Freeze Fracture Technique
- Membrane Structure Analysis:
- Description: The technique is primarily used to study the structural organization of biological membranes, including the arrangement of lipids and proteins.
- Application: It helps in visualizing the distribution of integral membrane proteins and understanding their roles in membrane function.
- Cellular Organelles:
- Description: Freeze fracture can be used to investigate the internal structures of cellular organelles.
- Application: This includes studying the architecture of mitochondria, endoplasmic reticulum, and Golgi apparatus, providing insights into their functional organization.
- Epithelial Cell Membranes:
- Description: The technique is particularly useful for examining the specialized membranes of epithelial cells.
- Application: For example, it has been used to study the unique structures of the plasma membrane in the urinary bladder, revealing the organization of uroplakins 29.
- Cytoskeletal Interactions:
- Description: Freeze fracture can reveal interactions between membranes and the cytoskeleton.
- Application: This is important for understanding how the cytoskeleton influences membrane dynamics and cellular shape.
- Vesicle and Endosome Studies:
- Description: The technique can be applied to study vesicles and endosomes.
- Application: It helps in understanding the processes of endocytosis and exocytosis by visualizing the membrane dynamics involved.
- Comparative Studies:
- Description: Freeze fracture allows for comparative studies between different cell types or conditions.
- Application: Researchers can assess how various treatments (e.g., drugs, environmental changes) affect membrane structure and organization.
- Artificial Membranes and Liposomes:
- Description: The technique is also applicable to artificial membranes and liposomes.
- Application: This is useful in the development of drug delivery systems and studying membrane properties in synthetic biology.
- Pathological Studies:
- Description: Freeze fracture can be used to investigate pathological changes in cell membranes.
- Application: It aids in understanding diseases that affect membrane integrity, such as cancer and neurodegenerative disorders.
- Developmental Biology:
- Description: The technique can be applied in developmental biology to study changes in membrane structure during cell differentiation.
- Application: This helps in understanding how membrane dynamics contribute to developmental processes.
- Cryo-Electron Microscopy:
- Description: Freeze fracture is often used in conjunction with cryo-electron microscopy to provide complementary information.
- Application: This combination enhances the resolution and detail of membrane structures observed.
Advantages Freeze Fracture Technique
- High Resolution:
- Description: Freeze fracture provides high-resolution images of membrane structures, allowing for detailed visualization of the organization of lipids and proteins.
- Benefit: This level of detail is often superior to that obtained from conventional thin-section electron microscopy.
- Planar Views of Membranes:
- Description: The technique produces planar views of the internal organization of membranes by splitting them into half-membrane leaflets.
- Benefit: This unique perspective allows for the examination of the distribution and arrangement of integral membrane proteins as intramembrane particles.
- Preservation of Native Structure:
- Description: Rapid freezing preserves the native state of biological samples, minimizing artifacts that can occur during sample preparation.
- Benefit: This leads to more accurate representations of cellular structures and functions.
- Versatility:
- Description: Freeze fracture can be applied to a wide range of biological samples, including tissues, cells, and artificial membranes.
- Benefit: This versatility allows researchers to study various biological systems and conditions, including pathological states.
- Combination with Other Techniques:
- Description: Freeze fracture can be combined with other techniques, such as immunogold labeling and cryo-electron microscopy.
- Benefit: This integration enhances the ability to identify specific proteins and understand their functional roles within the membrane.
- Dynamic Studies:
- Description: The technique can capture dynamic cellular events by using ultrarapid freezing methods.
- Benefit: This capability allows researchers to study transient structures and processes, such as vesicle trafficking and membrane fusion.
- Quantitative Analysis:
- Description: Advanced imaging and analysis software can be used to quantify the density and distribution of particles on fracture faces.
- Benefit: This quantitative approach provides robust data for statistical analysis and interpretation of membrane organization.
- Minimal Sample Preparation:
- Description: Compared to other electron microscopy techniques, freeze fracture requires less extensive sample preparation.
- Benefit: This reduces the risk of introducing artifacts and preserves the integrity of the sample.
- Insight into Membrane Dynamics:
- Description: The technique allows for the observation of changes in membrane structure under different experimental conditions.
- Benefit: This provides insights into membrane dynamics, fluidity, and the effects of various treatments on cellular membranes.
- Historical Significance:
- Description: Freeze fracture has played a crucial role in advancing our understanding of cell biology over the past several decades.
- Benefit: Its historical significance underscores its reliability and importance in the field of ultrastructure research.
Limitations Freeze Fracture Technique
- Complex Interpretation:
- Description: The images produced by freeze fracture can be complex and may require a high level of expertise to interpret accurately.
- Limitation: Misinterpretation can lead to incorrect conclusions about membrane structure and function.
- Sample Preparation Sensitivity:
- Description: The success of the technique heavily relies on the quality of sample preparation, including freezing and fracturing conditions.
- Limitation: Inadequate preparation can introduce artifacts or lead to incomplete preservation of structures, affecting the reliability of the results.
- Limited to Membrane Studies:
- Description: Freeze fracture is primarily focused on studying membranes and their components.
- Limitation: It may not be suitable for investigating other cellular structures, such as cytoplasmic components or organelles, without additional techniques .
- Requires Specialized Equipment:
- Description: The technique necessitates specialized equipment for rapid freezing and fracturing.
- Limitation: This can be a barrier for some laboratories, particularly those with limited resources or access to advanced technology.
- Potential for Artifacts:
- Description: Although freeze fracture minimizes artifacts compared to other methods, some artifacts can still occur during the freezing and fracturing process.
- Limitation: These artifacts can complicate the interpretation of the resulting images and may misrepresent the true structure of the membranes.
- Not Suitable for All Samples:
- Description: Certain biological samples may not be amenable to freeze-fracture analysis due to their size, composition, or structural properties.
- Limitation: This limits the applicability of the technique to a subset of biological materials.
- Limited Information on Protein Function:
- Description: While freeze fracture can reveal the distribution of proteins within membranes, it does not provide direct information about their functional states or interactions.
- Limitation: Additional techniques, such as biochemical assays or live-cell imaging, may be needed to complement the findings.
- Time-Consuming Protocols:
- Description: The freeze-fracture process involves multiple steps, including freezing, fracturing, and replicating.
- Limitation: This can be time-consuming, especially for large-scale studies or when optimizing protocols for new samples.
- Limited Depth of Field:
- Description: The technique provides a two-dimensional view of three-dimensional structures.
- Limitation: This can result in a loss of depth information, making it challenging to fully understand the spatial relationships between different membrane components.
- Potential for Overlapping Structures:
- Description: In complex samples, overlapping structures can obscure the visualization of individual components.
- Limitation: This can complicate the analysis and interpretation of the resulting images, particularly in densely packed membranes.
- Severs, N. J. (2007). Freeze-fracture electron microscopy. Nature Protocols, 2(3), 547–576. doi:10.1038/nprot.2007.55
- Carson JL. Fundamental technical elements of freeze-fracture/freeze-etch in biological electron microscopy. J Vis Exp. 2014 Sep 11;(91):51694. doi: 10.3791/51694. PMID: 25285532; PMCID: PMC4828069.