What is Polyacrylamide Gel Electrophoresis (PAGE)?
- PAGE is a technique that is widely utilized in many scientific domains, including biochemistry, forensic chemistry, genetics, molecular biology, and biotechnology. Its major function is to separate and study biological macromolecules depending on their electrophoretic mobility, such as proteins or nucleic acids.
- Polyacrylamide gels are created by chemically crosslinking acrylamide monomers with a cross-linking agent, most commonly N,N’-methylenebisacrylamide. Ammonium persulfate initiates and catalyzes the polymerization process, which is catalyzed by N,N,N’,N’-tetramethylethylendiamine (TEMED). The resulting gel matrix is porous, allowing molecules to migrate during electrophoresis.
- Sodium dodecyl sulfate polyacrylamide gel electrophoresis (SDS-PAGE) is the most often utilized form of polyacrylamide gel electrophoresis for protein separation. SDS-PAGE uses an anionic detergent, sodium dodecyl sulfate (SDS), to denature the proteins and impart a uniform negative charge-to-mass ratio to each protein molecule. Because of the consistent charge distribution, the proteins may be sorted mostly based on their molecular weight.
- SDS-PAGE begins with the preparation of the polyacrylamide gel, where the concentration of acrylamide can be changed to regulate the gel’s pore size. Smaller pore diameters arise from higher acrylamide concentrations, allowing for better separation of smaller molecules. Following the preparation of the gel, the protein samples are combined with SDS and additional chemicals such as 2-mercaptoethanol to disrupt disulfide links, further denatureing the proteins. After that, the mixture is put into wells on the gel and an electric field is applied. The negatively charged proteins migrate through the gel at varying rates depending on their molecular weight, with smaller proteins migrating quicker and longer distances.
- The relative molecular weight of the proteins can be calculated by comparing the relative migration distance of each protein to the length of the gel, known as the relative front (Rf) value. This data is useful for protein analysis, identification, and purity determination.
- Polyacrylamide gel electrophoresis can also be used to analyze nucleic acids. A denaturant, such as urea, is typically employed in this scenario to disrupt the structure of nucleic acids, allowing them to be sorted based on size and charge.
- To summarize, polyacrylamide gel electrophoresis (PAGE) is a flexible technique for separating and analyzing biological macromolecules, most notably proteins and nucleic acids. It is based on the electrophoretic mobility concept, with SDS-PAGE being a popular version for protein separation based on molecular weight. PAGE is a helpful tool in many scientific areas for determining the content, structure, and purity of biological materials.
Definition of Polyacrylamide Gel Electrophoresis (PAGE)
Polyacrylamide Gel Electrophoresis (PAGE) is a technique used to separate and analyze proteins or nucleic acids based on their mobility in a porous polyacrylamide gel matrix under the influence of an electric field.
Types of Polyacrylamide Gel Electrophoresis (PAGE)
There are several types of Polyacrylamide Gel Electrophoresis (PAGE) that are commonly used in different applications. Here are some of the main types:
- Native PAGE: In native PAGE, the biomolecules are separated based on their charge, size, and shape while preserving their native conformation. This method is often used to analyze protein-protein interactions, protein complexes, and native protein structures.
- Denaturing PAGE: Denaturing PAGE is used to separate biomolecules based on their size by disrupting their native structure. Denaturing agents such as SDS (Sodium Dodecyl Sulfate) are added to denature proteins, while denaturing conditions such as high temperatures or low pH can be applied to nucleic acids. Denaturing PAGE is commonly used for protein and nucleic acid sequencing, protein purification, and protein quantification.
- SDS-PAGE: Sodium Dodecyl Sulfate Polyacrylamide Gel Electrophoresis (SDS-PAGE) is a widely used denaturing PAGE method for protein separation. SDS is an anionic detergent that denatures proteins and provides a negative charge to each protein in proportion to its mass. SDS-PAGE is commonly used for protein size determination, protein purity analysis, and protein quantification.
- Two-Dimensional Gel Electrophoresis (2D PAGE): Two-Dimensional Gel Electrophoresis combines two separation techniques to achieve higher resolution and separation power. In the first dimension, proteins are separated based on their isoelectric point using isoelectric focusing (IEF). Then, in the second dimension, the proteins are separated based on their molecular weight using SDS-PAGE. 2D PAGE is particularly useful for complex protein mixtures, protein profiling, and comparative proteomics.
- Preparative PAGE: Preparative PAGE is used for large-scale separation and purification of biomolecules. Larger sample volumes are loaded onto a larger gel, allowing for the collection and isolation of specific protein or nucleic acid bands for further downstream applications.
These are some of the commonly used types of Polyacrylamide Gel Electrophoresis (PAGE). Each type has its advantages and is chosen based on the specific experimental requirements and goals.
Principle of Polyacrylamide Gel Electrophoresis (PAGE)
Polyacrylamide Gel Electrophoresis (PAGE) operates on the principle that charged molecules migrate in an electric field towards an electrode with the opposite charge. In the case of SDS-PAGE, proteins are treated with sodium dodecyl sulfate (SDS), an anionic detergent that binds to the proteins and imparts a negative charge to them. The negatively charged proteins are then loaded onto a polyacrylamide gel and subjected to an electric field, causing them to migrate towards the positively charged electrode (anode). The proteins are separated based on their size as they move through the gel matrix, which acts as a molecular sieve. After the electrophoresis process, the separated proteins can be visualized using protein-specific staining techniques. By comparing the migration distance of unknown proteins to that of a known molecular weight marker, the size of the proteins can be determined. The concentration of acrylamide and the degree of cross-linking in the gel can be adjusted to control the resolution and separation of different-sized molecules.
Requirements for Polyacrylamide Gel Electrophoresis (PAGE)
To perform Polyacrylamide Gel Electrophoresis (PAGE), several requirements and equipment are necessary. These include:
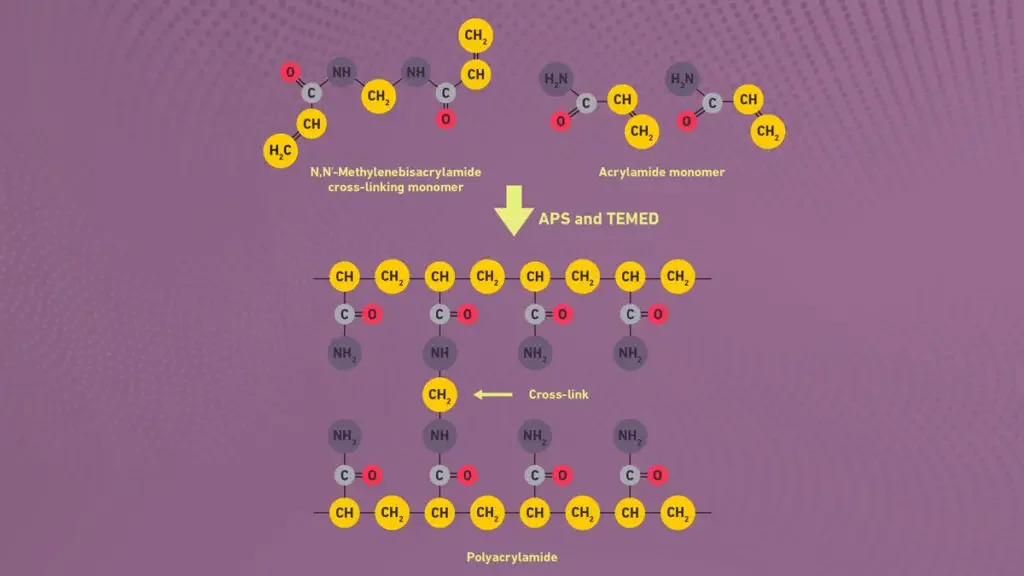
- Acrylamide solutions: Acrylamide solutions are required to prepare both the resolving gel and the stacking gel. These solutions are prepared by dissolving acrylamide and the cross-linking agent N,N’-methylenebisacrylamide in appropriate concentrations.
- Isopropanol/distilled water: Isopropanol or distilled water is used for various steps, such as sample preparation and dilution of solutions.
- Gel loading buffer: Gel loading buffer is added to the protein samples to provide density, color, and tracking dye, facilitating the loading of samples into the gel wells.
- Running buffer: Running buffer is the electrolyte solution that surrounds the gel during electrophoresis. It provides the ions necessary for the electric current to pass through the gel and helps maintain a stable pH.
- Staining and destaining solutions: Staining solutions are used to visualize the separated proteins or nucleic acids within the gel. Common staining methods include Coomassie Brilliant Blue or Silver staining. Destaining solutions are used to remove excess dye from the gel after staining.
- Protein samples: The samples to be analyzed, such as protein extracts or purified proteins, are loaded onto the gel for separation.
- Molecular weight markers: Molecular weight markers, also known as protein ladders, are a set of known proteins with different molecular weights. These markers are loaded onto the gel alongside the samples to estimate the size of the separated proteins.
In addition to the specific requirements, the equipment necessary for PAGE includes:
- Electrophoresis chamber and power supply: The electrophoresis chamber holds the gel and buffer during the separation process. The power supply provides the electric field required for the migration of charged molecules through the gel.
- Glass plates: Two glass plates, a short plate, and a top plate, are used to create the gel matrix. The gel is sandwiched between these plates during the casting process.
- Casting frame: A casting frame, also known as a gel cassette, is used to hold the glass plates together and create a defined space for the gel formation.
- Casting stand: The casting stand holds the casting frame in an upright position during gel preparation and polymerization.
- Combs: Combs are placed in the casting frame to create wells in the gel, where the protein samples and molecular weight markers are loaded.
These requirements and equipment are essential for conducting successful Polyacrylamide Gel Electrophoresis (PAGE) experiments and separating and analyzing biological molecules.
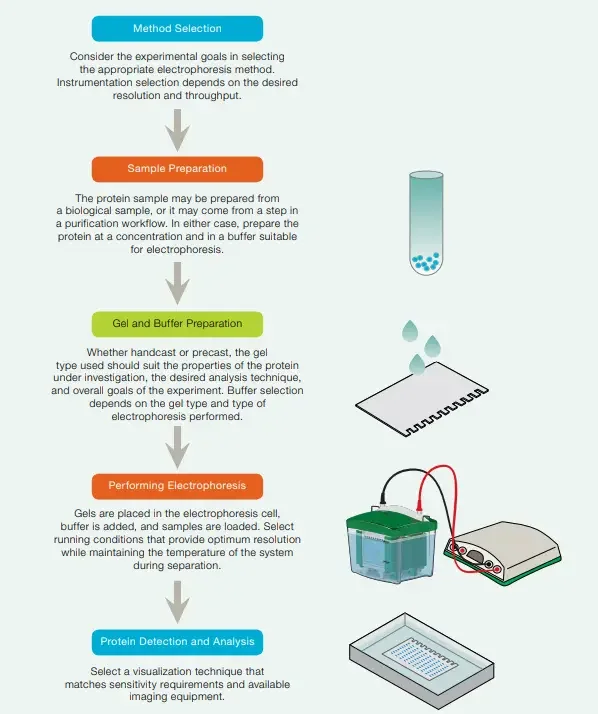
Steps Involved in Polyacrylamide Gel Electrophoresis (PAGE)
1. Sample preparation
Sample preparation is a crucial step in Polyacrylamide Gel Electrophoresis (PAGE) that involves the preparation of the material containing proteins or nucleic acids for analysis. Here are the key points regarding sample preparation:
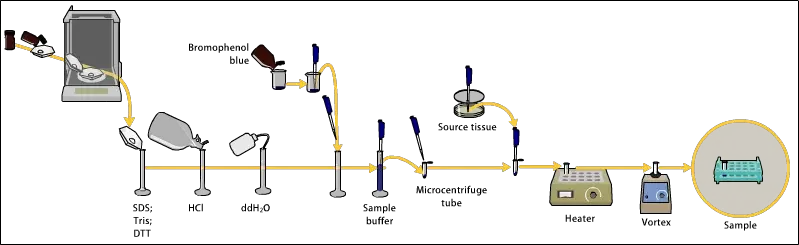
- Sample sources: Samples can be derived from various biological sources such as prokaryotic or eukaryotic cells, tissues, viruses, environmental samples, or purified proteins. Synthetic biomolecules like oligonucleotides can also be used as analytes.
- Mechanical disruption: Solid tissues or cells are often mechanically disrupted to release the proteins or nucleic acids they contain. This can be done using methods like blending (for larger sample volumes), homogenization (for smaller volumes), sonication, or cycling of high pressure. These techniques break down the samples into smaller particles and facilitate the extraction of biomolecules.
- Separation of cell compartments: In some cases, biochemical and mechanical techniques, including filtration and centrifugation, are employed to separate different cell compartments and organelles before performing electrophoresis. This helps in isolating specific cellular components for analysis.
- Denaturation: To ensure accurate separation based on size, proteins or nucleic acids are often denatured using chemical denaturants. For proteins, sodium dodecyl sulfate (SDS), an anionic detergent, is commonly used to denature secondary and non-disulfide-linked tertiary structures. SDS also imparts a negative charge to each protein in proportion to its mass. For nucleic acids, urea is used to disrupt hydrogen bonds between base pairs, causing strand separation. Heating the samples to at least 60 °C further promotes denaturation.
- Reduction of disulfide bonds: In protein analysis, disulfide bonds can be reduced to further denature the proteins. This is achieved by briefly heating the samples in the presence of a reducing agent like dithiothreitol (DTT) or 2-mercaptoethanol (beta-mercaptoethanol/BME). Reduction of disulfide linkages helps overcome tertiary protein folding and breaks up quaternary protein structures.
- Tracking dye: A tracking dye is often added to the sample solution. This dye has a higher electrophoretic mobility than the analytes, allowing the experimenter to monitor the progress of the solution through the gel during the electrophoretic run.
By following appropriate sample preparation techniques, the proteins or nucleic acids of interest are effectively denatured, ensuring accurate separation and analysis during PAGE.
2. Preparing acrylamide gels
Preparing acrylamide gels for Polyacrylamide Gel Electrophoresis (PAGE) involves several steps and specific components. Here is the process of preparing acrylamide gels:
- Gel composition: Acrylamide gels typically consist of acrylamide, bisacrylamide, an optional denaturant (such as SDS or urea), and a buffer with adjusted pH. The ratio of bisacrylamide to acrylamide can be varied based on specific requirements, but it is generally about 1 part in 35. The concentration of acrylamide in the gel can also be adjusted and typically ranges from 5% to 25%. Lower percentage gels are suitable for resolving high molecular weight molecules, while higher percentages are needed for smaller proteins.
- Degassing the solution: To prevent the formation of air bubbles during polymerization, the acrylamide gel solution may be degassed under a vacuum. This step helps ensure a smooth gel surface.
- Addition of butanol (optional): After pouring the resolving gel (for proteins), butanol may be added to the gel solution. Butanol helps remove bubbles and create a smooth gel surface.
- Initiating polymerization: Free radicals are necessary to initiate the polymerization process. Ammonium persulfate and TEMED (Tetramethylethylenediamine) are commonly used as a source of free radicals and a stabilizer, respectively. These components are added to the gel solution to trigger polymerization.
- Formation of the gel: Polymerization occurs when bisacrylamide cross-links with acrylamide molecules, resulting in the formation of a gel matrix. The bisacrylamide concentration and the total concentration of acrylamides (% T) determine the average pore diameter of the polyacrylamide gel. The % C (concentration of bisacrylamide) also influences pore size, with a concentration of 5% producing the smallest pores.
- Gel casting: The gel is usually polymerized between two glass plates using a gel caster. A comb is inserted at the top of the gel to create sample wells, where the protein or nucleic acid samples will be loaded.
- Comb removal: Once the gel is polymerized, the comb can be carefully removed, leaving behind the sample wells.
After preparing the acrylamide gel, it is ready for electrophoresis, where proteins or nucleic acids will be separated based on their size and charge when an electric field is applied.
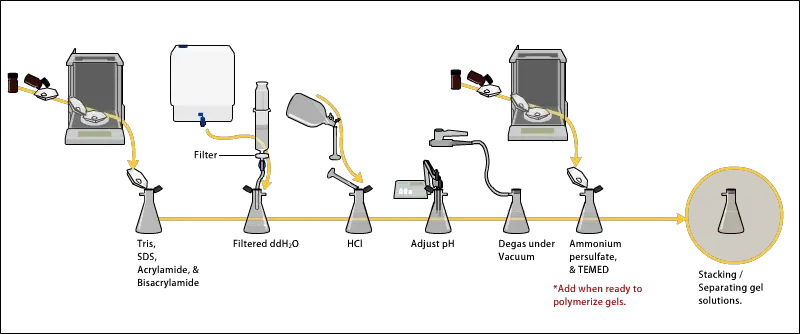
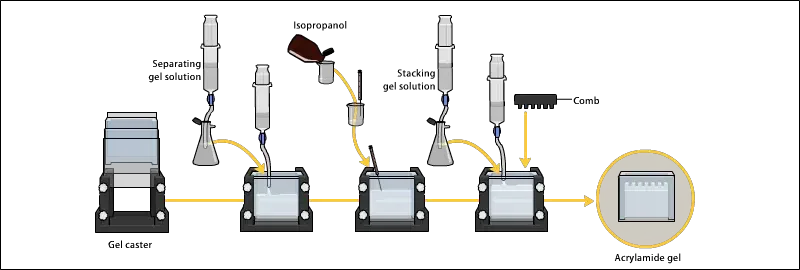
3. Electrophoresis
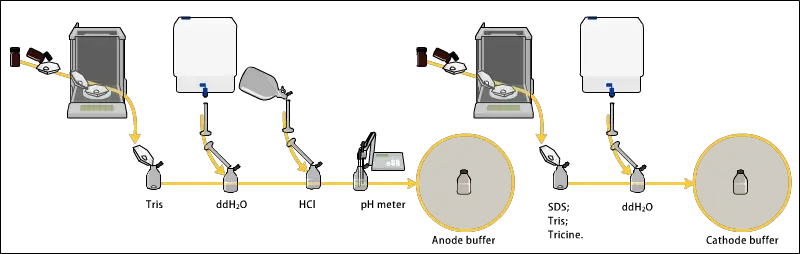
The process of electrophoresis in polyacrylamide gel involves several steps. Here is an overview of the electrophoresis process:
- Buffer selection: The choice of buffer system used in polyacrylamide gel electrophoresis (PAGE) depends on the nature of the sample and the experimental objective. Buffers may vary at the anode (positive electrode) and cathode (negative electrode) depending on the specific requirements of the experiment.
- Applying an electric field: An electric field is applied across the gel by connecting the gel apparatus to a power supply. The negative electrode (cathode) is positioned at the end of the gel where the samples are loaded, while the positive electrode (anode) is placed at the opposite end. The electric field causes the negatively charged proteins or nucleic acids in the samples to migrate through the gel.
- Migration through the gel: Biomolecules, such as proteins or nucleic acids, move through the gel matrix based on their size. Smaller molecules can pass through the pores in the gel more easily and therefore migrate farther from the point of origin. Larger molecules have more difficulty moving through the gel and remain closer to the point of origin.
- Running the gel: The gel is typically run for a specified period of time, which can range from a few hours to overnight, depending on the experimental conditions and objectives. The migration of biomolecules is influenced by the voltage applied across the gel. Higher voltages result in faster migration but may compromise accuracy compared to lower voltages.
- Separation based on size: As the biomolecules migrate through the gel, they separate based on their size. Smaller biomolecules travel a greater distance from the point of origin, while larger ones remain closer. The separation is primarily determined by molecular weight under denaturing conditions and higher-order conformation under native conditions.
- Gel mobility and analysis: Gel mobility is defined as the rate of migration traveled with a voltage gradient of 1V/cm and is measured in units of cm2/sec/V. For analytical purposes, the relative mobility (Rf) of biomolecules is determined by calculating the ratio of the distance traveled by the molecule on the gel to the total distance traveled by a tracking dye. This information can be used to plot calibration curves or standard markers, enabling the estimation of biomolecular sizes.
It’s important to note that certain glycoproteins and larger proteins may exhibit anomalous behavior or challenges in their analysis using SDS-PAGE or conventional gel systems, respectively. Additional techniques or modifications may be required to address these specific cases.
4. Detection
Detection is an essential step in the polyacrylamide gel electrophoresis (PAGE) process. Here are some key points about the detection of separated biomolecules:
- Staining: After the electrophoresis run, the gel can be stained to visualize the separated biomolecules. Different staining methods are used depending on the type of biomolecule. Coomassie Brilliant Blue staining is commonly used for proteins, while ethidium bromide staining is used for nucleic acids. Silver staining is another technique that can be used for both proteins and nucleic acids.
- Visualization of bands: Staining the gel allows the separated biomolecules to appear as distinct bands within the gel. Each band represents a different species or size of biomolecule. The intensity of the band can also provide information about the relative abundance of the biomolecule.
- Molecular weight markers: To determine the approximate molecular mass of unknown biomolecules, it is common practice to run molecular weight size markers in a separate lane of the gel. These markers consist of biomolecules of known molecular weights. By comparing the distance traveled by the unknown biomolecule bands relative to the marker bands, an estimation of their molecular mass can be obtained.
- Further processing: Depending on the specific experimental objectives, the gel may undergo further processing after staining. For example, in Western blotting, the separated biomolecules are transferred onto a membrane and probed with specific antibodies to detect and identify target proteins.
Overall, detection methods in PAGE allow researchers to visualize and analyze the separated biomolecules, determine their approximate sizes, and gain insights into their presence and abundance in the sample.
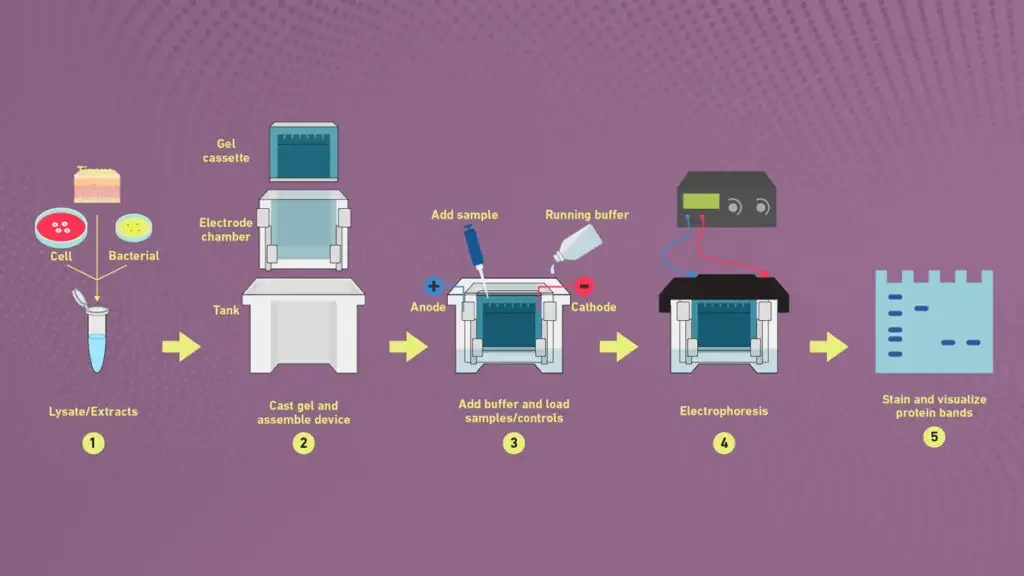
SDS PAGE vs native PAGE
- SDS-PAGE (Sodium Dodecyl Sulfate Polyacrylamide Gel Electrophoresis) and native PAGE (Polyacrylamide Gel Electrophoresis) are two variations of the PAGE technique that differ in their sample preparation and the information they provide.
- SDS-PAGE involves denaturing the proteins prior to electrophoretic separation. Proteins are treated with sodium dodecyl sulfate (SDS), an anionic detergent, along with heat and reducing agents like β-mercaptoethanol. This process disrupts the protein’s secondary and tertiary structures, breaks disulfide bridges, and coats the proteins with SDS. The denatured proteins have a uniform negative charge-to-mass ratio and are separated based solely on their molecular weight. SDS-PAGE is widely used for protein separation and is effective in analyzing a wide range of protein sizes, typically from 5 to 250 kDa.
- On the other hand, native PAGE preserves the proteins’ higher-order structure by leaving the bonds intact. It allows the analysis of proteins in their native or natural state. The distribution of proteins in the gel during native PAGE is primarily influenced by the protein’s charge, determined by its amino acid sequence and post-translational modifications, as well as the pH of the separation. Native PAGE is often preferred when studying protein complexes or bound proteins, as it maintains their biological activity. Sample preparation for native PAGE does not involve the use of SDS, reducing agents, or heat, and lower voltages may be used during electrophoresis.
- In summary, SDS-PAGE denatures proteins and separates them solely based on molecular weight, while native PAGE preserves the proteins’ structure and separates them primarily based on charge. The choice between SDS-PAGE and native PAGE depends on the specific research goals and whether the analysis requires information about protein size or the preservation of protein complexes and their natural activity.
What is 2D gel electrophoresis?
- 2D gel electrophoresis, also known as two-dimensional polyacrylamide gel electrophoresis or 2D PAGE, is a powerful technique used to separate and analyze complex protein mixtures. It was developed in 1975 by Joachim Klose and Patrick H. O’Farrell as a way to enhance the resolving power of protein separation.
- The technique combines two dimensions of separation, allowing for higher resolution and improved separation of proteins with similar properties. In the first dimension, proteins are separated based on their isoelectric point (pI), which is a measure of their charge at a specific pH. Isoelectric focusing (IEF) is commonly used in this step, where proteins migrate through a pH gradient generated in a gel, and eventually come to rest when their pI matches the local pH in the gel.
- After the first dimension separation, the proteins are then subjected to a second dimension separation, which is typically performed using SDS-PAGE (polyacrylamide gel electrophoresis). In this dimension, the proteins are separated based on their molecular mass. SDS-PAGE involves the use of sodium dodecyl sulfate (SDS) to denature the proteins and coat them with a negative charge proportional to their mass. When an electric field is applied, the proteins migrate through the gel based on their size, with smaller proteins migrating faster than larger ones.
- By combining the isoelectric point separation in the first dimension with the molecular mass separation in the second dimension, 2D gel electrophoresis generates a two-dimensional map of protein spots called an electropherogram. Each spot on the map represents a different protein, and the position of the spot provides information about the protein’s isoelectric point and molecular mass. This allows for the identification and comparison of proteins within complex mixtures.
- 2D gel electrophoresis is widely used in proteomics research and has various applications. It can be used for protein profiling, identification of protein isoforms or post-translational modifications, comparative analysis of protein expression between samples, and biomarker discovery. The technique provides a powerful tool for studying protein complexity and heterogeneity in biological samples.
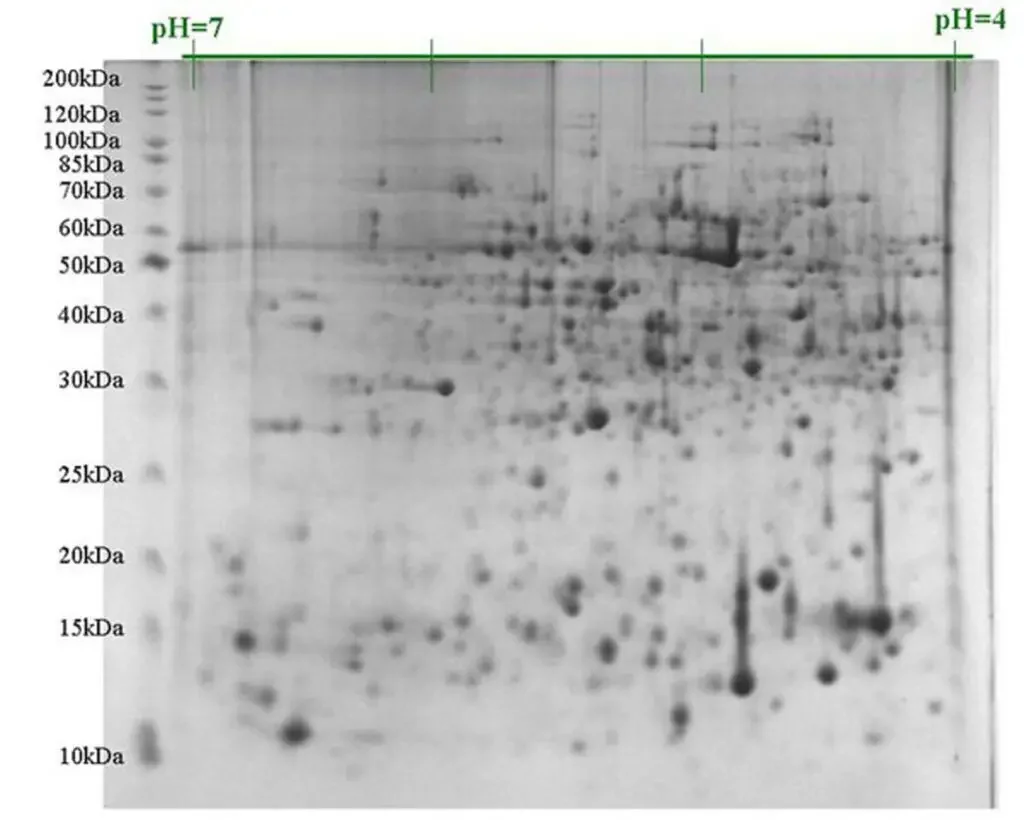
The Ratio of Acrylamide and Its Crosslinker Determines the Pore Size of Acrylamide Gels
- The pore size of polyacrylamide gels, which determines their sieving effect on the components being separated, is determined by the ratio of acrylamide to its cross-linking agent, bis-acrylamide. This ratio can be adjusted to achieve the desired separation characteristics of the gel.
- The relationship between the pore size, total acrylamide concentration (T), and the degree of cross-linking (C) can be expressed as percentages. The total acrylamide concentration is calculated by adding the mass of acrylamide (a) and bis-acrylamide (b) and dividing it by the total volume of the polyacrylamide solution. The degree of cross-linking is calculated by dividing the mass of bis-acrylamide by the sum of the masses of acrylamide and bis-acrylamide.
- In general, as the total acrylamide concentration (T) increases while the degree of cross-linking (C) remains constant, the pore size of the gel increases. However, when the total acrylamide concentration is constant, the pore size is largest at high and low values of the degree of cross-linking.
- For the electrophoretic separation of proteins, polyacrylamide gels typically have a degree of cross-linking (C) of 3%, corresponding to an acrylamide/bis-acrylamide ratio of 29:1. For nucleic acid separation, the degree of cross-linking is usually 5%, corresponding to a ratio of 19:1.
- Gels with higher degrees of cross-linking tend to be brittle, opaque, and relatively hydrophobic, making them challenging to handle and less suitable for electrophoretic separation.
- In practice, polyacrylamide gel electrophoresis (PAGE) is performed using gels with varying acrylamide concentrations ranging from 3% to 30%. Lower acrylamide content (3-7.5%) is commonly used for nucleic acid separation, while higher acrylamide content (8-30%) is used for protein electrophoresis. The specific acrylamide concentration is chosen based on the size and properties of the molecules being separated.
The relationship between the pore size of acrylamide gels, the concentration of total acrylamide and bis-acrylamide can be expressed as a percentage of total acrylamide concentration (T), and the degree of cross-linking (C) as follows:
T = (a + b)/V x 100[%],
C = b/(a + b) x 100[%]
- where T is the total acrylamide concentration,
- C is the degree of cross-linking
- a is the mass of acrylamide in g,
- b is the mass of bis-acrylamide in g, and
- V is the total volume of polyacrylamide solution in mL.
Applications of Polyacrylamide Gel Electrophoresis (PAGE)
Polyacrylamide Gel Electrophoresis (PAGE) has a wide range of applications in the field of biochemistry and molecular biology. Here are some common applications of PAGE:
- Measuring molecular weight: PAGE is commonly used to determine the molecular weight of proteins and nucleic acids by comparing their migration distances with known molecular weight markers.
- Peptide mapping: PAGE can be used to analyze and map the peptide fragments generated from the enzymatic digestion of proteins, providing information about the protein’s primary structure.
- Estimation of protein size: PAGE allows the estimation of protein size by separating proteins based on their molecular weight. This information is crucial for characterizing protein samples.
- Determination of protein subunits or aggregation structures: PAGE can reveal the presence and composition of protein subunits and complexes. It helps in studying protein-protein interactions and the formation of multimeric protein structures.
- Estimation of protein purity: PAGE can assess the purity of protein samples by detecting the presence of contaminants or impurities through the separation of different components.
- Protein quantitation: PAGE can be used for semi-quantitative or relative quantification of proteins by comparing the intensity of stained bands or using densitometry analysis.
- Monitoring protein integrity: PAGE is employed to assess the integrity and structural changes of proteins under various conditions, such as denaturation, aggregation, or degradation.
- Comparison of polypeptide composition: PAGE allows the comparison of the polypeptide composition between different samples, helping to identify similarities or differences in protein profiles.
- Post-electrophoresis applications: PAGE is often used as a preliminary step for downstream applications like Western blotting, where the separated proteins are transferred to a membrane for specific protein detection using antibodies.
- Staining and labeling: PAGE can be combined with staining techniques to visualize proteins in the gel. Coomassie Brilliant Blue staining is a commonly used method for protein visualization.
- Detection of post-translational modifications: PAGE can aid in the detection of protein post-translational modifications, such as protein ubiquitination, by analyzing the mobility shifts of modified protein forms.
Overall, PAGE is a versatile technique with numerous applications in protein and nucleic acid analysis, providing valuable insights into their size, structure, purity, and interactions.
Advantages of Polyacrylamide Gel Electrophoresis (PAGE)
Polyacrylamide Gel Electrophoresis (PAGE) offers several advantages that make it a valuable technique for molecular biology and biochemistry research:
- Stable chemically cross-linked gel: Polyacrylamide gels are chemically cross-linked, providing stability and durability during electrophoresis. The gel matrix remains intact and allows for consistent and reliable separation of biomolecules.
- Greater resolving power: PAGE offers high resolving power, leading to sharp and well-defined bands. It allows for the separation of closely sized molecules, providing detailed information about molecular weight differences or fragment sizes.
- Accommodates larger quantities of DNA: Polyacrylamide gels can handle larger quantities of DNA samples without significant loss in resolution. This feature is particularly useful when working with samples containing a higher amount of DNA or when analyzing complex mixtures.
- Extremely pure DNA recovery: The DNA recovered from polyacrylamide gels is highly pure. The gel matrix effectively separates DNA fragments, minimizing contamination and yielding purified samples suitable for downstream applications.
- Controllable pore size: The pore size of polyacrylamide gels can be easily adjusted by altering the concentrations of acrylamide and bisacrylamide monomers. This allows researchers to optimize the gel for specific applications, such as separating low molecular weight fragments or analyzing larger biomolecules.
- Separation of low molecular weight fragments: PAGE is particularly well-suited for the separation of low molecular weight fragments, such as small proteins or short DNA/RNA fragments. The gel matrix with fine pores enables efficient separation and resolution of these small biomolecules.
Overall, the stable and chemically cross-linked nature of polyacrylamide gels, combined with their high resolving power, controllable pore size, and ability to accommodate larger sample quantities, make PAGE advantageous for various molecular biology applications. It provides researchers with reliable and precise separation of biomolecules, contributing to the understanding of their structure, size, and composition.
Disadvantages of Polyacrylamide Gel Electrophoresis (PAGE)
Polyacrylamide Gel Electrophoresis (PAGE) has several disadvantages that researchers should consider when choosing this technique:
- Time-consuming preparation: Compared to agarose gels, polyacrylamide gels require more time and effort to prepare. The process involves handling toxic monomers, ensuring proper gel polymerization, and assembling the gel apparatus. This longer preparation time can be a drawback, especially when quick results are desired.
- Toxic monomers: Acrylamide, one of the monomers used in polyacrylamide gel preparation, is a neurotoxin and a potential carcinogen. Researchers must exercise caution when handling acrylamide and take appropriate safety measures to protect themselves and the environment.
- Gel leakage: Polyacrylamide gels can be prone to leakage during casting and running. Ensuring proper sealing and avoiding leaks can be challenging, requiring careful attention and expertise. Gel leakage can lead to distorted sample bands and compromised results.
- One-time use: Polyacrylamide gels are typically used for a single experiment and cannot be reused. Once the electrophoresis run is complete, the gel is usually discarded, requiring the preparation of a new gel for each subsequent experiment. This can increase the cost and time associated with the technique.
- Stable chemically cross-linked gel: While the stability of the chemically cross-linked gel is an advantage in terms of maintaining gel integrity during electrophoresis, it can also be a disadvantage. Once the gel is polymerized, it cannot be altered or adjusted. Researchers cannot make changes to the pore size or composition of the gel matrix once it has solidified, limiting the flexibility of the technique.
Despite these disadvantages, polyacrylamide gel electrophoresis remains a widely used and valuable technique for many applications. Researchers should carefully consider the specific requirements of their experiments and weigh the advantages and disadvantages of PAGE before choosing it as their preferred method of analysis.
FAQ
What is Polyacrylamide Gel Electrophoresis (PAGE)?
Polyacrylamide Gel Electrophoresis (PAGE) is a technique used to separate and analyze proteins or nucleic acids based on their size, charge, and shape using a polyacrylamide gel matrix.
What is the purpose of PAGE?
The main purpose of PAGE is to separate biomolecules, such as proteins or nucleic acids, into individual components based on their mobility in an electric field, allowing for further analysis and characterization.
How does PAGE work?
In PAGE, an electric field is applied to the gel matrix containing the biomolecules. The charged biomolecules migrate through the gel matrix at different rates based on their size and charge. Smaller molecules move faster, while larger ones move more slowly.
What are the different types of PAGE?
There are two main types of PAGE: native PAGE and denaturing PAGE. Native PAGE preserves the natural conformation of biomolecules, while denaturing PAGE disrupts the structure, typically using detergents or denaturing agents.
What is the role of acrylamide in PAGE?
Acrylamide is a key component in the gel matrix of PAGE. It polymerizes to form a stable gel matrix that provides a sieving effect, allowing for the separation of biomolecules based on size.
What is the difference between resolving gel and stacking gel in PAGE?
In PAGE, the gel matrix is divided into two regions: the resolving gel and the stacking gel. The resolving gel has a higher concentration of acrylamide and is responsible for separating biomolecules based on their size. The stacking gel has a lower concentration of acrylamide and helps to concentrate and focus the biomolecules before they enter the resolving gel.
How is protein size determined in PAGE?
Protein size is determined in PAGE by comparing the migration distance of protein bands on the gel to the migration of known molecular weight markers. A calibration curve can be constructed to estimate the size of unknown proteins.
Can PAGE be used for nucleic acid separation?
Yes, PAGE can be used for nucleic acid separation as well. It is commonly used for analyzing DNA fragments, RNA molecules, and other nucleic acid species.
What are the limitations of PAGE?
PAGE can be time-consuming and requires careful preparation. It may also have limitations in resolving very large or small biomolecules, and specialized techniques may be needed for complex samples.
What are the applications of PAGE?
PAGE has various applications in molecular biology, biochemistry, and biotechnology. It is used for protein purification, protein characterization, DNA sequencing, analysis of gene expression, and the study of protein-protein interactions, among others.
References
- https://vlab.amrita.edu/?sub=3&brch=186&sim=319&cnt=2
- https://conductscience.com/polyacrylamide-gel-electrophoresis/
- https://www.technologynetworks.com/analysis/articles/polyacrylamide-gel-electrophoresis-how-it-works-technique-variants-and-its-applications-359100
- https://www.news-medical.net/life-sciences/What-is-Polyacrylamide-Gel-Electrophoresis-(PAGE).aspx
- https://www.kharagpurcollege.ac.in/studyMaterial/115412Polyacrylamide%20and%20its%20electrophoresis,%20by%20Dr.Indranil%20Chakraborty.pdf
- https://www.bio-rad.com/webroot/web/pdf/lsr/literature/Bulletin_6040.pdf
- https://clinicalproteomicsjournal.biomedcentral.com/articles/10.1186/1559-0275-11-16
- https://openwetware.org/wiki/Polyacrylamide_gel_electrophoresis