What is Channel Protein?
- A channel protein is a unique arrangement of amino acids that is imbedded in the cell membrane and provides a hydrophilic pathway for water and tiny polar ions.
- As with all transport proteins, the size and form of each channel protein excludes all save the most particular molecules.
- Embedded beneath the membrane is a channel protein of generic type. Ions, represented by the little green hexagons, traverse the channel protein.
- They relocate from a high concentration area to a low concentration area.
- A channel protein’s primary function is to rapidly transfer ions and water molecules through the membrane.
- Channel proteins are transmembrane proteins that are involved in the entry and departure of substances from the cell. Open channel proteins and gated channel proteins are the two types of channel proteins.
- Ion channels for chloride, potassium, calcium, and sodium are examples of channel proteins. Aquaporins are an unique sort of channel proteins that can rapidly transport water across a membrane.
Types of Channel Protein
There are present 2 types of channel protein such as;
1. Non-gated Channel
- A Channel protein may exist in a state that is always open. This protein is known as a non-gated channel.
- These proteins allow ions and water to pass through the usually hydrophobic cell membrane, which would normally fight the passage of these molecules.
- When the constant movement of water and ions into or out of the cell is required to maintain the equilibrium of water and ions, a non-gated channel protein is required.
- However, it can be detrimental to leave a channel open at all times.
- The second type of protein channel addresses this issue.
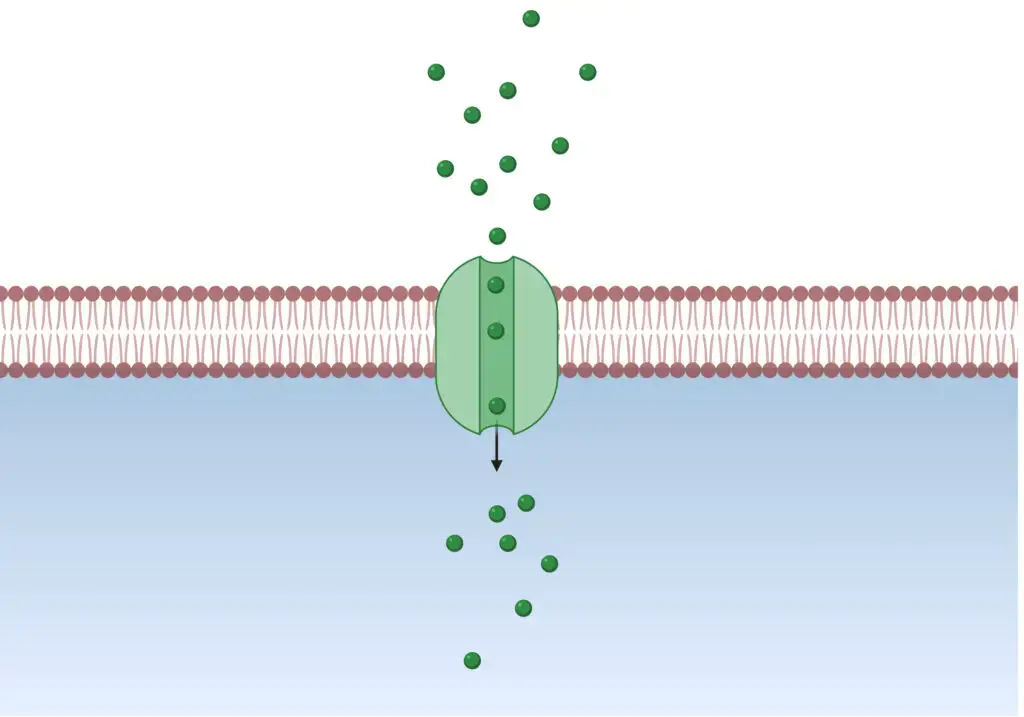
2. Gated Channel
- A gated channel protein remains closed until a certain chemical or electrical signal is received.
- These channel proteins are crucial to numerous biological activities.
- The capacity to gate an ion channel permits the accumulation of electrical energy within the cell. This is the sole determinant of nerve function.
- On the surface of nerve cells, channel proteins respond to electrical impulses generated by the flooding of ions through the membrane next to them.
- As they open, ions escape and the electrical disruption continues. This swiftly transmits a signal throughout the body.
- Below is an image of a gated channel protein reacting to a signal molecule.
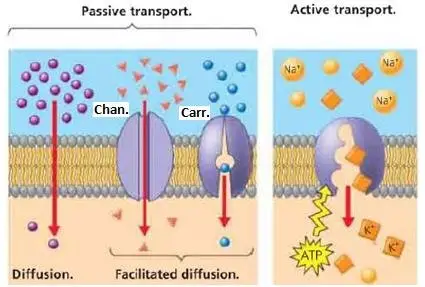
Structure of Channel Protein
- Most channel proteins are composed of many identical protein subunits that create a hydrophilic core.
- When a signal is received, the conformation of a gated channel changes, enabling access to the hydrophilic pathway.
- Typically, non-gated channels are composed of identical subunits connected in a circular.
- Although the interior of the circle is hydrophilic, the exposed amino acids within the hydrophobic cell membrane are likewise non-polar. This aids in the protein’s anchoring within the membrane.
- If the protein attempted to escape the membrane, polar forces would drive it back into place.
Function of Channel Protein
- Permitting the straightforward passage of water and ions
- Allowing ions or water to flow freely from one membrane to another in order to determine the location of ions or water.
- Hold back the chemicals until any of the signals appear to generate active charges for the transport of ions and water.
- To transfer them solely via the plasma membrane or not at all To maintain balance when necessary
Channel Proteins and Carrier Proteins
- There are four distinct forms of intracellular transport. Small gas molecules, such as oxygen and carbon dioxide, and many non-polar compounds, such as steroid hormones and pharmaceutical medicines, undergo simple diffusion.
- These compounds are chemically and physically suited to pass through the cell membrane.
- Hydrophilic, positively charged molecules have difficulty flowing through the membrane. Ions, water, and sugars such as glucose are examples. Channel proteins are primarily responsible for facilitated diffusion.
- While the chemicals are still travelling in the direction of their concentration (from high to low), the cell membrane allows them to pass through. This permits them to move at speeds close to diffusion.
- Not all assisted diffusion, however, is mediated by channel proteins. Facilitated diffusion also involves carrier proteins, which bind and transport molecules across the membrane. Large molecules such as glucose cannot flow through the channel proteins’ small pathway. Uniporters are carrier proteins that attach to glucose molecules one at a time. The binding activity alters the protein’s structure, causing it to deposit the molecule on the other side of the cell. These carrier proteins transport molecules down their concentration gradient without requiring energy.
- When compounds must be transported against their concentration gradient, carriers of more complexity are required. Active transport is the process of using a carrier protein and ATP to move a molecule against its concentration gradient. The energy is required since molecules tend to disperse and spread out on their own. It takes a great deal of energy to move certain ions and molecules, but this is vital for the evolution of life. Additional transporter proteins have developed for cotransport. A molecule can be transported against its concentration gradient by transferring it down its gradient. This type of carrier protein enables cells to transport substances by utilising the ion gradient created by other ATP carrier proteins.
- Stereospecificity is the primary distinction between a channel protein and a carrier protein. While channel proteins restrict passage to molecules of a specific size, they do not bind the molecules. At the active site of carrier proteins, the chemical to be delivered must bind. This site binds selectively to a single molecule and attempts to transport it alone. The binding activity permits the big molecule to pass through the cell membrane.
Example of Channel Protein
Chloride channel
- Chloride channels are a superfamily of chloride-specific ion channels that are poorly understood.
- These channels are named for chloride because its concentration in vivo is significantly higher than that of other anions.
- In humans, several families of voltage-gated and ligand-gated channels (e.g., the CaCC families) have been identified.
- Voltage-gated chloride channels play numerous essential physiological and cellular activities, including pH regulation, volume homeostasis, organic solute transport, cell migration, cell proliferation, and cell differentiation.
- The chloride channels can be classified into a variety of classes based on sequence homology.
- Chloride channels are essential for establishing the resting membrane potential of a cell and maintaining its volume.
- These channels transport Cl and other anions, including HCO3, I, SCN, and NO3.
- These channels have a structure unlike any other known channels. The subunits of chloride channels comprise between one and twelve transmembrane segments. Others are activated by Ca2+, extracellular ligands, or pH.
Potassium channel
- Potassium channels are the most prevalent form of ion channel, present in nearly all organisms.
- They generate holes across cell membranes that are selective for potassium. Potassium channels are present in the majority of cell types and regulate a vast array of cellular processes.
- Potassium channels transmit potassium ions swiftly (up to the diffusion rate of K+ ions in bulk water) and selectively down their electrochemical gradient (excluding, most notably, sodium despite the sub-angstrom difference in ionic radius).
- Biologically speaking, these channels set or reset the resting potential of many cells. In excitable cells, such as neurons, the action potential is shaped by the delayed counterflow of potassium ions.
- By regulating the duration of the cardiac action potential in cardiac muscle, potassium channels can contribute to life-threatening arrhythmias. Potassium channels may possibly play a role in vascular tone maintenance.
- In addition to regulating physiological activities like as hormone secretion (e.g., insulin release from beta-cells in the pancreas), their dysfunction can result in disease (such as diabetes).
- Some toxins, including dendrotoxin, are strong due to the fact that they inhibit potassium channels.
- Four major kinds of potassium channels exist:
- Calcium-activated potassium channels – open when calcium ions or other signalling molecules are present.
- Inwardly rectifying potassium channel – facilitates the inward flow of current (positive charge) (into the cell).
- Tandem pore domain potassium channels – such as “resting potassium channels” or “leak channels” that determine the negative membrane potential of neurons – are constitutively open or have significant basal activation.
- Voltage-gated potassium channels – are ion channels that open or close in response to transmembrane voltage changes.
Calcium channel
- A calcium channel is an ion channel with selective calcium ion permeability. Although there are other ligand-gated calcium channels, it is commonly synonymous with voltage-gated calcium channel.
Sodium channel
- Sodium channels are proteins that are part of the cell membrane and make up ion channels that let sodium ions (Na+) pass through the cell membrane.
- They are part of the superfamily of cation channels and can be put into groups based on what opens the channel for ions, such as a change in voltage (“voltage-gated,” “voltage-sensitive,” or “voltage-dependent” sodium channel; also called “VGSCs” or “Nav channel”) or a substance binding to the channel (“ligand-gated,” “ligand-sensitive,” or “ligand-dependent” sodium channel; also called “Nav channel”) (ligand-gated sodium channels).
- The rising phase of action potentials is caused by sodium channels in cells that can get excited, like neurons, myocytes, and some types of glia.
- There are three different states for these channels: resting, active, and inactive.
- Even though the ions couldn’t move through the channels in the resting and inactive states, there is a difference in how their structures are set up.
- When it comes to moving ions across cell membranes, sodium channels are very picky. Many different things can be done to get the high selectivity for the sodium ion. All of them involve putting the sodium ion inside a larger molecule in a space of a certain size.
References
- Hanna, Hanna. (2016). Electropermeabilization of inner and outer cell membranes with microsecond pulsed electric fields : effective new tool to control mesenchymal stem cells spontaneous Ca2+ oscillations.
- https://www.slideshare.net/rakhiadarsh/carrier-and-channel-proteins
- https://en.wikipedia.org/wiki/Membrane_transport_protein
- https://www.biologyonline.com/dictionary/carrier-protein
- https://teaching.ncl.ac.uk/bms/wiki/index.php/Channel_proteins
- https://askabiologist.asu.edu/venom/protein-channels
- https://pediaa.com/difference-between-channel-and-carrier-proteins/
- https://kaiserscience.wordpress.com/biology-the-living-environment/cells/ion-channels-and-carrier-proteins/
- https://biologydictionary.net/channel-protein/
- https://lambdageeks.com/channel-protein-function/