What is Osmotic Pressure?
- Osmotic pressure is a fundamental concept in the realm of science, particularly in the study of solutions and their interactions with semi-permeable membranes. It is defined as the pressure that must be exerted on a solution to prevent the flow of solvent into it through a semi-permeable membrane. This pressure arises due to the inherent tendency of solvents, especially water, to move from a region of lower solute concentration to a region of higher solute concentration.
- The driving force behind osmotic pressure is the natural propensity of molecules to distribute randomly, aiming for a uniform concentration throughout a given space. This phenomenon is governed by the principles of diffusion.
- When two solutions of varying solute concentrations are separated by a semi-permeable membrane, which allows only the solvent to pass through, the solvent will naturally move from the dilute solution to the concentrated one. This movement continues until an equilibrium is achieved, where the concentrations on both sides of the membrane are equal.
- In biological contexts, osmotic pressure plays a pivotal role. The cellular environment is a delicate balance of intracellular and extracellular solute concentrations. Any disparity between these environments can instigate the movement of water either into or out of the cell. Such movement can have profound implications for cellular health, leading to potential cell rupture due to excessive water influx or cell dehydration due to water efflux.
- Furthermore, osmotic pressure is not merely a passive phenomenon. Certain organisms, notably plants, harness osmotic pressure for specific physiological functions, such as water transport. However, an imbalance in osmotic pressure can also pose challenges. For instance, a sudden increase in the extracellular solute concentration can draw water out of cells, leading to dehydration.
- From a technical standpoint, osmotic pressure can be categorized into two types: potential osmotic pressure and actual osmotic pressure. The former represents the maximum possible osmotic pressure under hypothetical conditions where a solution is separated from its pure solvent by a semi-permeable membrane. In contrast, the latter is the pressure exerted in real scenarios.
- In practical applications, the principles of osmosis and osmotic pressure find utility in various industries, including food preservation. The addition of sugar or salt to foods can draw out water from microbial cells, rendering them inactive and preserving the food.
- In conclusion, osmotic pressure is a crucial scientific concept with significant implications in biology and industry. It underscores the natural tendency of molecules to achieve equilibrium and highlights the delicate balance that exists in cellular environments.
Definition of Osmotic Pressure
Osmotic pressure is the force required to prevent the flow of solvent molecules through a semi-permeable membrane due to differences in solute concentrations between two solutions.
Osmotic Pressure Equation
Osmotic pressure, a fundamental concept in the study of solutions, can be quantitatively described using a specific equation. This equation provides a mathematical relationship between osmotic pressure and other variables, allowing scientists to predict and understand osmotic behavior in various systems.
The equation for osmotic pressure is given by:
Π=iCRT
Where:
- Π: Represents osmotic pressure. Contrary to the common mathematical constant, in this context, the symbol ΠΠ is universally accepted by chemists to denote osmotic pressure.
- i: Denotes the van’t Hoff factor, which accounts for the number of particles a solute dissociates into when dissolved in a solvent.
- C: Stands for the molar concentration of the solute. It is crucial to note that it’s the number of solute particles that influence osmotic interactions, not their volume or weight.
- R: Is the universal gas constant. While originally defined for gases, this constant is applicable to liquids as well, as both are considered “fluids” in chemistry. Fluids are characterized by their ability to allow particles to diffuse freely.
- T: Represents the temperature, measured in Kelvin (K). Kelvin is a preferred scale in scientific equations due to its absolute nature. Unlike Celsius, where zero is the freezing point of water, zero Kelvin signifies absolute zero, a state where molecular motion ceases. This absolute measure of heat provides a consistent reference point, making Kelvin a more precise scale for scientific calculations.
The relationship expressed by this equation was introduced by the renowned Dutch chemist, Jacobus van’t Hoff. His insights laid the foundation for understanding the osmotic behavior of ideal solutions. However, it’s essential to recognize that this equation is most accurate for solutions that exhibit ideal behavior.
In summary, the osmotic pressure equation offers a comprehensive framework to understand and predict the osmotic phenomena in solutions. By incorporating variables like the van’t Hoff factor, molar concentration, universal gas constant, and temperature, it provides a holistic view of the factors influencing osmotic pressure.
Understanding Osmotic Pressure – What is Osmosis?
Osmosis is a fundamental biological and chemical process characterized by the selective movement of solvent molecules across a semipermeable membrane. This movement is driven by a difference in solute concentration on either side of the membrane. To elucidate:
- Mechanism of Osmosis:
- Solvent molecules traverse from an area of lower solute concentration to an area of higher solute concentration.
- The semipermeable membrane, pivotal to this process, is selectively permeable. It permits the passage of solvent molecules while restricting the movement of solute particles.
- Equilibrium in Osmosis:
- The movement of solvent molecules continues until an equilibrium is achieved, where the solute concentrations on both sides of the membrane become equal.
- Osmotic Pressure Defined:
- When osmosis is in progress, a certain amount of pressure can be applied to the side with the higher solute concentration to counteract and halt the osmotic movement. This specific pressure, which effectively stops osmosis, is termed the osmotic pressure.
- Reverse Osmosis – A Special Case:
- If a pressure greater than the osmotic pressure is exerted on the solution side, an intriguing phenomenon occurs. Solvent molecules begin to move in the opposite direction, from the region of higher solute concentration to the region of lower concentration. This counterintuitive process is known as reverse osmosis.
In essence, osmotic pressure and the process of osmosis are central to understanding various biological and chemical systems. They play a crucial role in maintaining cellular homeostasis and are foundational to many technological applications, including water purification.
Osmotic Pressure and Hydrostatic Pressure
Osmotic pressure and hydrostatic pressure are two fundamental concepts in fluid dynamics and physiology, often discussed in the context of biological systems. While they may appear similar or even interchangeable in some references, a closer examination reveals distinct differences in their origins, mechanisms, and implications.
Hydrostatic Pressure: Derived from the Greek words “hydro” (meaning water) and “static” (meaning at rest), hydrostatic pressure refers to the pressure exerted by a fluid, such as water, when it is stationary within a confined space. This pressure arises primarily due to gravitational forces. Factors influencing hydrostatic pressure include the fluid’s density, the depth of the fluid column, and gravitational acceleration. For instance, deeper regions of a water column will experience higher hydrostatic pressures due to the weight of the water above.
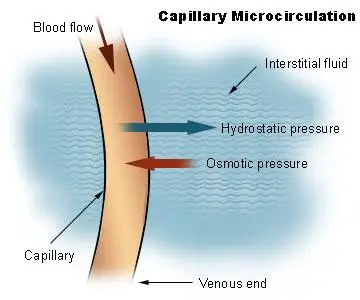
In the context of the human circulatory system:
- Blood Hydrostatic Pressure (BHP): Refers to the force exerted by blood against the walls of larger blood vessels.
- Capillary Hydrostatic Pressure (CHP): Denotes the pressure exerted by blood against capillary walls.
- Interstitial Fluid Hydrostatic Pressure (IFHP): Represents the pressure within the interstitial fluid, the fluid that surrounds cells.
Osmotic Pressure: Osmotic pressure, on the other hand, is the force required to prevent solvent molecules from passing through a semi-permeable membrane due to solute concentration differences. Unlike hydrostatic pressure, which is influenced by gravitational forces, osmotic pressure arises from solute concentration gradients. In the circulatory system, osmotic pressure, sometimes termed “oncotic pressure,” counteracts hydrostatic pressure, drawing fluid back into capillaries.
Key contributors to osmotic gradients in blood include plasma proteins. The osmotic pressure due to these proteins in the blood is termed Blood Colloidal Osmotic Pressure (BCOP), while that in the interstitial fluid is called Interstitial Fluid Colloidal Osmotic Pressure (IFCOP). Given that BCOP is typically greater than IFCOP, water tends to move from tissues back into capillaries, a process termed “reabsorption.”
Distinguishing the Two: While both osmotic and hydrostatic pressures play roles in fluid movement, their origins and mechanisms differ:
- Hydrostatic pressure is primarily a result of gravitational forces and fluid depth.
- Osmotic pressure arises from solute concentration differences and is not influenced by gravity.
In biological systems, particularly within capillaries, these pressures work in tandem, governing fluid exchange between blood and tissues. Hydrostatic pressure pushes fluid out of capillaries, while osmotic pressure pulls it back in, ensuring a delicate balance vital for physiological homeostasis.
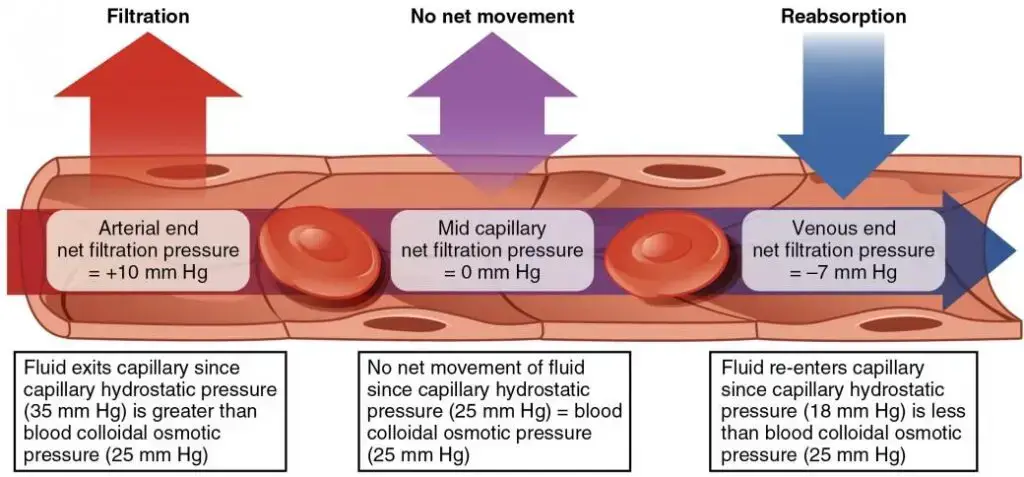
In conclusion, while osmotic and hydrostatic pressures are both pivotal in fluid dynamics, they are distinct forces with unique origins and implications, especially in the context of biological systems.
Turgor Pressure vs. Osmotic Pressure
In the realm of plant physiology, the concepts of turgor pressure and osmotic pressure play pivotal roles in understanding water dynamics and cellular health. While both pressures are influenced by water and solute concentrations, their origins, implications, and roles within plant cells differ significantly.
Turgor Pressure: Turgor pressure refers to the hydrostatic force exerted by the cell’s fluid (cytoplasm) against its cell wall. This pressure is vital for maintaining the structural integrity and rigidity of plant cells. When plant cells are filled with water, they exhibit a state of turgidity, making them appear swollen and rigid. This rigidity is crucial for the plant’s posture and overall health.
A high turgor pressure ensures that the plant cell membrane, or the plasma membrane, is pressed firmly against the cell wall, giving the cell its characteristic turgid appearance. Conversely, when turgor pressure decreases, cells lose their rigidity, leading to a flaccid state. Visually, this manifests as a wilted appearance in plants.
Osmotic Pressure: Osmotic pressure, on the other hand, is a measure of the force required to prevent water molecules from moving across a semi-permeable membrane due to solute concentration differences. In the context of plant cells, osmotic pressure can be thought of as the “pulling force” exerted by solute particles either within or outside the cell, influencing the movement of water molecules.
While turgor pressure is a manifestation of the internal pressure within a cell, osmotic pressure represents the potential force driving water movement due to solute concentration gradients. Essentially, osmotic pressure dictates the direction and rate of osmosis, the passive movement of water through biological membranes.
Interplay of Turgor and Osmotic Pressures: The relationship between turgor and osmotic pressures is dynamic. Osmotic pressure, influenced by solute concentrations, determines the direction of water flow across the cell membrane. As water enters the cell, the internal volume increases, leading to a rise in turgor pressure. Conversely, when water exits the cell, turgor pressure decreases, leading to a flaccid state.
In conclusion, while both turgor and osmotic pressures are integral to plant cell water dynamics, they serve distinct roles. Turgor pressure reflects the internal hydrostatic force within plant cells, ensuring structural rigidity, while osmotic pressure represents the potential force driving water movement based on solute gradients. Understanding the interplay between these pressures is crucial for a comprehensive grasp of plant physiology and cellular health.
Osmosis and Osmotic Potential
Osmosis, a fundamental concept in the realm of biology and chemistry, describes the spontaneous movement of water molecules across a semi-permeable membrane. This movement is driven by differences in water potential on either side of the membrane, with water molecules tending to flow from regions of higher water potential to those of lower potential. This process is analogous to simple diffusion, but it specifically pertains to the movement of the solvent (in most cases, water) rather than the solute.
The term “osmotic potential” is used to quantify the propensity of water molecules to migrate from one solution to another due to differences in solute concentration. Essentially, it provides a measure of the solution’s ability to draw water molecules across a membrane. A solution’s composition is defined by two primary components: the solute, which is the substance dissolved in the solvent, and the solvent itself, which is the medium in which the solute is dissolved.
Based on solute concentration, solutions can be categorized into three primary types:
- Isotonic Solution: Here, two solutions exhibit identical solute concentrations. As a result, there is no net movement of water molecules between them.
- Hypotonic Solution: This solution has a lower solute concentration and a higher concentration of water molecules compared to another solution.
- Hypertonic Solution: In contrast to a hypotonic solution, a hypertonic solution possesses a higher solute concentration and a lower concentration of water molecules.
The concept of osmolarity provides a metric to determine the solute concentration of a solution. It measures the number of osmoles of solute per liter of solution, aiding in the classification of the solution as isotonic, hypotonic, or hypertonic.
When considering pure water, devoid of any solutes, it represents the highest possible water potential. In comparison, any solution, due to the presence of solutes, will inherently have a water potential lower than that of pure water.
In scenarios where hypertonic and hypotonic solutions are juxtaposed, water molecules will naturally migrate from the hypotonic solution (higher osmotic potential) to the hypertonic solution (lower osmotic potential). This movement exemplifies the essence of osmosis, driven by the inherent desire to equilibrate osmotic potentials across the membrane.
In conclusion, osmosis and osmotic potential are integral to understanding the dynamics of water movement across membranes, influenced by solute concentrations. Their interplay is crucial in various biological processes, ensuring cellular health and function.
Types of Osmotic Pressure
Osmotic pressure, a fundamental concept in the realm of biology and chemistry, pertains to the pressure exerted by a solution to prevent the osmotic flow of water across a semi-permeable membrane. This pressure is intrinsically linked to the solute concentration within a solution. Based on the comparative solute concentrations of two solutions, osmotic pressure can be categorized into three distinct types:
- Isoosmotic Pressure: In situations where two solutions possess identical solute concentrations, they exhibit isoosmotic pressure. This means that both solutions exert the same osmotic pressure, leading to no net movement of water across a membrane separating them.
- Hypoosmotic Pressure: A solution displaying hypoosmotic pressure has a lower osmotic pressure in comparison to another reference solution. Consequently, water tends to move from the hypoosmotic solution to the reference solution, aiming to equilibrate the solute concentrations.
- Hyperosmotic Pressure: Contrary to hypoosmotic pressure, a solution with hyperosmotic pressure has a higher osmotic pressure than the surrounding or reference solution. This results in water molecules migrating from the reference solution into the hyperosmotic solution, striving to balance the solute concentrations.
In summary, these classifications of osmotic pressure are pivotal in understanding various biological phenomena, especially those related to cellular fluid dynamics and the maintenance of cellular homeostasis.
Importance of Osmotic Pressure
Osmotic pressure plays a pivotal role in various biological and chemical processes. Understanding its importance is crucial in both biological and industrial contexts. Here are some of the key reasons why osmotic pressure is significant:
- Cellular Health and Function:
- Osmotic pressure is vital for maintaining the proper balance of water and solutes within cells. This balance ensures that cells neither swell and burst (in a hypotonic environment) nor shrink and become dehydrated (in a hypertonic environment).
- Plant Turgidity:
- In plants, osmotic pressure is responsible for turgor pressure, which keeps plant cells rigid and firm. This turgidity is essential for maintaining the structural integrity of plants, especially in non-woody plants.
- Kidney Function:
- The kidneys regulate the balance of salts and water in the blood. Osmotic pressure plays a crucial role in the filtration and reabsorption processes in the nephrons of the kidneys.
- Blood Plasma Regulation:
- The osmotic pressure of blood plasma affects the movement of water between blood vessels and surrounding tissues. It’s essential for maintaining blood volume and blood pressure.
- Nerve Function:
- Proper osmotic balance is crucial for the function of nerve cells. Any imbalance can disrupt the transmission of nerve impulses, leading to potential complications.
- Digestion and Nutrient Absorption:
- The process of digestion involves breaking down food into smaller molecules that can be absorbed by the intestines. Osmotic pressure aids in the absorption of these nutrients into the bloodstream.
- Industrial Applications:
- Osmotic pressure is used in various industries, especially in the production of certain foods and beverages. For instance, osmotic pressure is utilized in the process of reverse osmosis to purify water.
- Pharmaceuticals:
- In medicine, understanding osmotic pressure is crucial for the formulation of intravenous solutions. These solutions must have an osmotic pressure similar to that of body fluids to prevent potential complications when administered.
- Research and Development:
- Osmotic pressure is a fundamental concept studied in various research areas, including biology, chemistry, and environmental science. It aids in the development of new technologies and solutions for various challenges.
- Environmental Implications:
- Osmotic pressure plays a role in soil moisture content and its availability to plants. It also affects the movement of water in aquatic ecosystems.
In summary, osmotic pressure is a fundamental force that governs the movement of water across membranes. Its importance spans across various biological processes and industrial applications, emphasizing its significance in both living organisms and technological advancements.
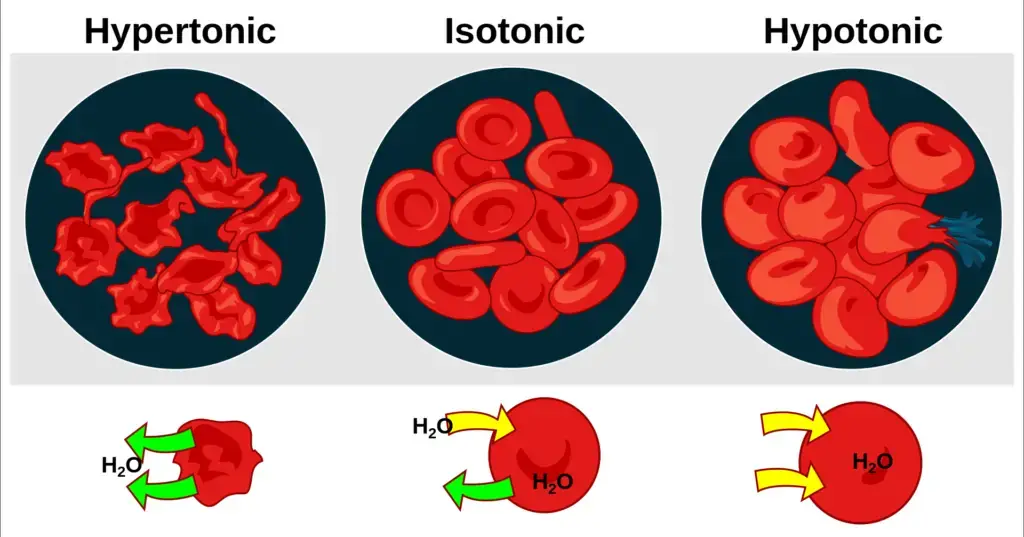
Examples of Osmotic Pressure
Osmotic pressure plays a pivotal role in various biological processes, ensuring the proper functioning and survival of organisms. Here are some illustrative examples derived from the intricate workings of the kidneys and cellular responses to osmotic environments:
- Osmotic Pressure in the Kidneys:
- Loop of Henle Dynamics: The kidneys, particularly the nephron’s loop of Henle, showcase varying osmotic pressures at different segments.
- Beginning of the Loop: Here, the tubular fluid exhibits an isoosmotic pressure relative to the plasma, meaning both have equivalent solute concentrations.
- Tip of the Loop: As the fluid descends deeper into the loop, it becomes hyperosmotic compared to the plasma, indicating a higher solute concentration.
- Exiting the Loop: Upon ascending and leaving the loop, the tubular fluid transitions to a hypoosmotic state relative to the plasma, signifying a reduced solute concentration.
- Loop of Henle Dynamics: The kidneys, particularly the nephron’s loop of Henle, showcase varying osmotic pressures at different segments.
- Cellular Response to Osmotic Environments:
- Freshwater Fish Cells in Varied Environments: The behavior of cells in different osmotic conditions can be exemplified by observing freshwater fish cells when subjected to diverse osmotic pressures.
- Hypoosmotic Environment: In this scenario, the external environment has a higher water potential and lower osmotic pressure than the fish cells. Consequently, water tends to move into the cells, potentially causing them to swell.
- Isoosmotic Environment: When the external environment mirrors the osmotic pressure of the fish cells, there is no net movement of water, maintaining cellular equilibrium.
- Hyperosmotic Environment: In an environment with a higher osmotic pressure than the fish cells, water tends to exit the cells, leading to potential cellular shrinkage.
- Freshwater Fish Cells in Varied Environments: The behavior of cells in different osmotic conditions can be exemplified by observing freshwater fish cells when subjected to diverse osmotic pressures.
In essence, these examples underscore the significance of osmotic pressure in regulating fluid dynamics within biological systems, ensuring cellular integrity and overall organismal health.
Solved Exercises
Exercise 1 – One mole of table salt is dissolved in one litre of water. At a temperature of 27oC, what would be the osmotic temperature of this solution?
To determine the osmotic pressure of a solution, we can use the formula:
Π=MRT
Where:
- Π is the osmotic pressure.
- M is the molar concentration of the solute.
- R is the ideal gas constant, which is approximately 0.0821 L.atm/mol.K.
- T is the temperature in Kelvin.
Given:
- M = 1 mol/L (since one mole of table salt is dissolved in one litre of water).
- T = 27°C = 27 + 273.15 = 300.15 K (converted to Kelvin).
However, there’s a catch. Table salt (NaCl) dissociates into two ions in water: one sodium ion (Na⁺) and one chloride ion (Cl⁻). Therefore, the effective number of particles (i) is 2.
So, the osmotic pressure is given by:
Π=iMRT
Where i is the van’t Hoff factor, which accounts for the dissociation of the solute in the solution. For NaCl, i = 2.
Plugging in the values:
Π=2×1mol/L×0.0821L.atm/mol.K×300.15K
Π=49.29atm
Thus, the osmotic pressure of the solution would be approximately 49.29 atm at 27°C.
Exercise 2 – The osmotic pressure of a potassium chloride solution (at 300K) is 50 atmospheres. What is the molar concentration of potassium chloride in this solution?
To determine the molar concentration of potassium chloride (KCl) in the solution, we can use the osmotic pressure formula:
Π=iMRT
Where:
- ΠΠ is the osmotic pressure.
- i is the van’t Hoff factor, which accounts for the dissociation of the solute in the solution.
- M is the molar concentration of the solute.
- R is the ideal gas constant, which is approximately 0.0821 L.atm/mol.K.
- T is the temperature in Kelvin.
Given:
- Π = 50 atm
- R = 0.0821 L.atm/mol.K
- �T = 300 K
Potassium chloride (KCl) dissociates into two ions in water: one potassium ion (K⁺) and one chloride ion (Cl⁻). Therefore, the effective number of particles (i) is 2.
Rearranging the formula to solve for M:
M = Π⁄iRT
Plugging in the values:
M = 50 atm⁄2 × 0.0821 L.atm/mol.K × 300 K
M = 50⁄49.26
M ≈ 1.015 mol/L
Thus, the molar concentration of potassium chloride in the solution is approximately 1.015 mol/L.
Quiz
What is osmotic pressure?
a) The pressure exerted by solvents
b) The pressure exerted by solutes
c) The pressure required to prevent the flow of solvent into a solution through a semipermeable membrane
d) The pressure exerted by gases
Which of the following is NOT affected by osmotic pressure?
a) Plant cell turgidity
b) Kidney function
c) Blood pressure
d) Boiling point of water
In a hypotonic solution, the osmotic pressure is:
a) Higher outside the cell than inside
b) Higher inside the cell than outside
c) Equal both inside and outside the cell
d) Not applicable
Osmotic pressure increases with:
a) Decrease in solute concentration
b) Increase in solute concentration
c) Decrease in solvent concentration
d) None of the above
Which of the following is true about osmotic pressure?
a) It is only applicable to gases
b) It is independent of temperature
c) It is directly proportional to temperature
d) It is inversely proportional to temperature
Reverse osmosis is a process where:
a) Solvent moves from a region of higher concentration to lower concentration
b) Solvent moves from a region of lower concentration to higher concentration against osmotic pressure
c) Solute moves from a region of higher concentration to lower concentration
d) None of the above
Osmotic pressure is a __ property.
a) Intensive
b) Extensive
c) Physical
d) Chemical
Which of the following factors does NOT influence osmotic pressure?
a) Nature of the solute
b) Temperature
c) Pressure
d) Color of the solution
In a hypertonic solution, a cell will:
a) Swell
b) Shrink
c) Remain unchanged
d) Burst
Osmotic pressure can be measured using:
a) Barometer
b) Thermometer
c) Osmometer
d) Manometer
FAQ
What is osmotic pressure?
Osmotic pressure is the minimum pressure required to prevent the flow of solvent into a solution through a semipermeable membrane.
How is osmotic pressure related to solute concentration?
Osmotic pressure is directly proportional to the solute concentration. The higher the solute concentration, the higher the osmotic pressure.
What is the difference between osmotic pressure and hydrostatic pressure?
While osmotic pressure is due to differences in solute concentration across a semipermeable membrane, hydrostatic pressure is the pressure exerted by a fluid at equilibrium due to the force of gravity.
How does temperature affect osmotic pressure?
Osmotic pressure increases with an increase in temperature because the kinetic energy of the molecules increases, leading to a higher rate of solvent movement.
What is reverse osmosis?
Reverse osmosis is a process where solvent molecules move from a region of lower solute concentration to a region of higher solute concentration, against the osmotic pressure, typically using an external pressure.
Why is osmotic pressure important in plants?
Osmotic pressure plays a crucial role in maintaining turgidity in plant cells, which helps in supporting the plant structure and its physiological processes.
How is osmotic pressure measured?
Osmotic pressure can be measured using an instrument called an osmometer.
What happens to a cell in a hypertonic solution?
In a hypertonic solution, where the surrounding solution has a higher solute concentration than the cell, the cell will lose water and shrink due to osmosis.
What is the significance of osmotic pressure in the human body?
Osmotic pressure helps in maintaining fluid balance across cell membranes, aids in nutrient absorption, and plays a vital role in kidney function and blood filtration.
Can osmotic pressure be negative?
No, osmotic pressure is always a positive value. However, the term “negative osmotic pressure” or “osmotic potential” is sometimes used in plant physiology to describe the tendency of water to move into a system.
References
- Atkins, Peter W.; de Paula, Julio (2010). “Section 5.5 (e)”. Physical Chemistry (9th ed.). Oxford University Press. ISBN 978-0-19-954337-3.
- Lewis, Gilbert Newton (1908). “The Osmotic Pressure of Concentrated Solutions and the Laws of the Perfect Solution”. Journal of the American Chemical Society. 30 (5): 668–683. doi:10.1021/ja01947a002
- Voet, Donald; Judith Aadil; Charlotte W. Pratt (2001). Fundamentals of Biochemistry (Rev. ed.). New York: Wiley. ISBN 978-0-471-41759-0.
- Osmosis, Tonicity, and Hydrostatic Pressure. (2023). Colostate.edu. http://www.vivo.colostate.edu/hbooks/pathphys/topics/osmosis.html#:~:text=Osmotic%20pressure%20is%20defined%20as,or%20a%20layer%20of%20cells.
- Capillary Exchange | Anatomy and Physiology II. (2023). Lumenlearning.com. https://courses.lumenlearning.com/suny-ap2/chapter/capillary-exchange/
- Colligative Properties. (2023). Purdue.edu. https://chemed.chem.purdue.edu/genchem/topicreview/bp/ch15/colligative.php
- Feher, J. (2017). Quantitative Human Physiology: An Introduction. Elsevier Inc. https://www.sciencedirect.com/book/9780128008836/quantitative-human-physiology