Passive Transport Definition
- Passive transport, also known as passive diffusion, is the passage of an ion or molecule through a cell wall along a concentration gradient, or from a region of high concentration to a region of low concentration.
- Comparable to transferring from the metro train to the platform or leaving a packed area. Passive transport essentially allows an ion or molecule “space to breathe.”
- This concept is most easily recalled when contrasted with its opponent, active transportation. Active transport, like physical activity, requires energy. In contrast, passive transport requires no energy at all.
- Passive transport, also known as passive diffusion, is the passage of an ion or molecule through a cell wall along a concentration gradient, or from a region of high concentration to a region of low concentration.
- Comparable to transferring from the metro train to the platform or leaving a packed area. Passive transport essentially allows an ion or molecule “space to breathe.”
- This concept is most easily recalled when contrasted with its opponent, active transportation. Active transport, like physical activity, requires energy. In contrast, passive transport requires no energy at all.
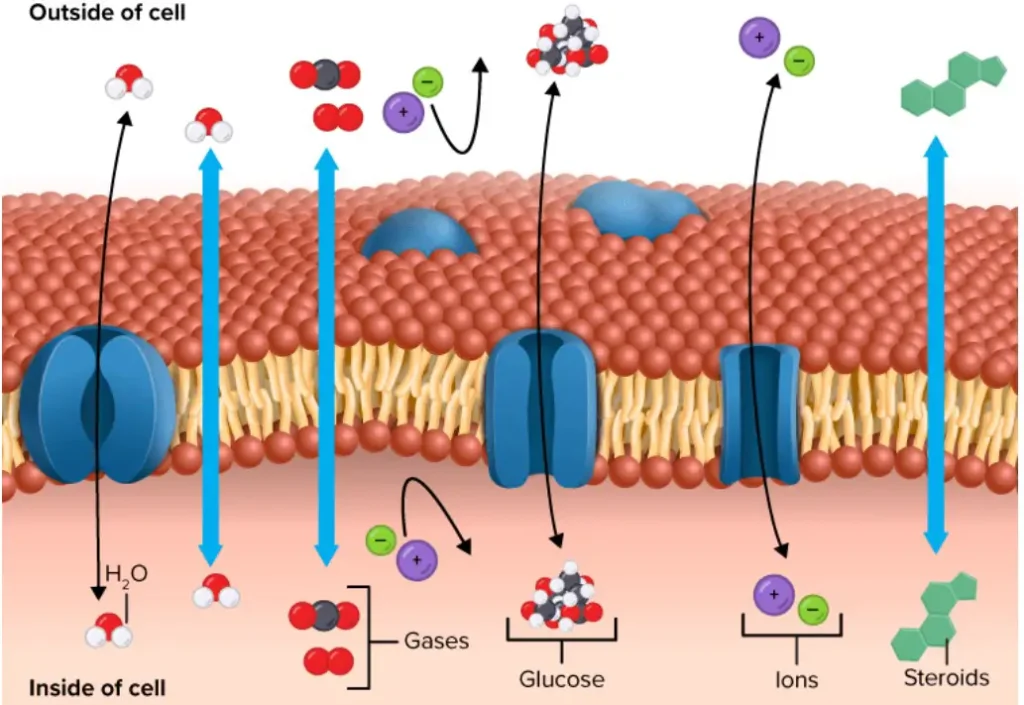
What is Diffusion?
- Diffusion is a passive transport method. The concentration of a single material tends to migrate from areas of high concentration to areas of low concentration until the concentration is uniform throughout the space.
- You are familiar with substances diffusing through the air. Consider, for instance, a person opening a bottle of perfume in a crowded room.
- The concentration of the fragrance is strongest in the bottle and lowest at the room’s perimeter. The perfume vapour will diffuse, or travel out from the bottle, and as it spreads, an increasing number of individuals will smell it.
- Through diffusion, substances travel within the cytoplasm of the cell, and by diffusion, certain substances migrate past the plasma membrane.
- The process of diffusion consumes no energy. Rather, the varying concentrations of materials in various regions represent a sort of potential energy, and diffusion is the dissipation of this potential energy as materials travel down their concentration gradients, from high to low.
- Each substance in a medium, such as extracellular fluid, has its own concentration gradient that is independent of the gradients of other substances. Moreover, each component will disperse in accordance with the gradient.
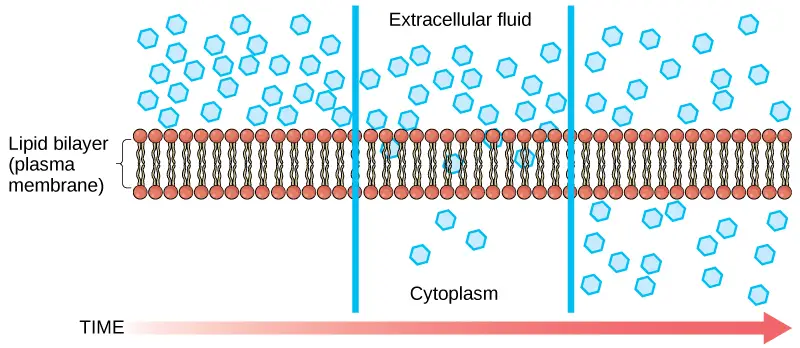
Factors affects the rate of Diffusion
- Extent of the concentration gradient: The larger the concentration gradient, the faster the diffusion. The closer a material’s distribution approaches equilibrium, the slower its rate of diffusion.
- Mass of the diffusing molecules: Molecules that are more massive diffuse more slowly because it is more difficult for them to migrate between the molecules of the substance they are moving through.
- Temperature: Greater temperatures enhance the energy and, consequently, the movement of molecules, so accelerating the rate of diffusion.
- Density of the solvent: When the density of the solvent increases, the diffusion rate drops. The molecules move more slowly because it is more difficult for them to pass through the denser material.
Characteristics of Passive Transport
- No energy expenditure: Passive transport does not require the cell to expend energy in order to move molecules or ions across the cell membrane.
- Movement down the concentration gradient: Passive transport moves molecules or ions from areas of high concentration to areas of low concentration, which is also known as moving down the concentration gradient.
- No transport proteins required for simple diffusion: In simple diffusion, molecules move directly across the cell membrane, without the need for transport proteins.
- Transport proteins required for facilitated diffusion: Facilitated diffusion requires transport proteins to move molecules or ions across the cell membrane.
- Saturation: Facilitated diffusion can become saturated when all the transport proteins are occupied by molecules or ions.
- No specificity: Passive transport does not exhibit specificity, meaning that any molecule or ion that can pass through the cell membrane can be transported.
- Rate dependent on temperature and concentration gradient: The rate of passive transport is dependent on temperature and the concentration gradient of the molecules or ions being transported.
Overall, passive transport allows for the movement of molecules or ions across the cell membrane without requiring the cell to expend energy.
Types Of Passive Transport
There are four types of passive transport:
- Simple Diffusion: Diffusion is the movement of chemicals from a higher concentration region to a lower concentration region. The difference in concentration between two locations is referred to as a concentration gradient, and diffusion continues until this gradient is neutralised. Liquids and gases undergo diffusion because their particles travel randomly from one location to another. It is a necessary process for several life processes in living organisms. Substances enter and exit cells through simple diffusion.
- Facilitated Diffusion: Facilitated diffusion is the passive movement of ions or molecules across the cell membrane by means of certain transmembrane integral proteins. Large and insoluble molecules necessitate a carrier substance for their transport across the plasma membrane. This procedure requires no external or cellular energy. Glucose transporter, ion channels, and aquaporins are assisted diffusion examples. The cell membrane is only permeable to a restricted number of tiny, non-polar molecules. Hence, transmembrane protein-mediated diffusion facilitation is essential.
- Filtration: The process of separating particles from liquids and gases called filtration. Filtration is illustrated by the selective absorption of nutrients by the body. This mechanism occurs along the concentration gradient without requiring any energy. An example of a biological filter is the kidney. The glomerulus filters the blood and reabsorbs the essential chemicals. In the process of filtration, the cell membrane allows only soluble and easily permeable substances to pass through its pores.
- Osmosis: In order to equalise the concentration of other substances, water and other molecules flow across a selectively permeable membrane during osmosis. Temperature and concentration gradient influence osmosis. The higher the concentration gradient, the larger the osmosis rate. In addition, the rate of osmosis increases as the temperature rises. There is a conflict theory regarding the osmosis process. Few biologists argue that osmosis is an active transport rather than a passive one.
1. Simple Diffusion
- Simple diffusion is the spontaneous movement of materials from an area of high concentration to an area of low concentration until equilibrium is reached. This process does not require any energy expenditure and relies on the concentration gradient as a source of potential energy for the transport of molecules.
- During simple diffusion, molecules or solute particles move in a random manner known as Brownian motion. The flux, which measures the number of moles of solute crossing one square centimeter of membrane per second, can be calculated using Torrell’s equation proposed in 1953. The equation takes into account the mobility of the solute, the concentration of the material available for diffusion, and the driving force of the concentration gradient.
- The concentration gradient acts as the driving force for molecular transport across the membrane. As equilibrium is achieved and the concentrations on both sides become equal, the flux across the membrane reaches zero.
- Simple diffusion occurs in liquids and gases due to the random movement of their particles. Common examples of molecules transported through simple diffusion include oxygen, carbon dioxide, and ethanol.
- In prokaryotes such as bacteria, simple diffusion is also responsible for the transport of water, nutrients, gases, and the excretion of waste materials. These organisms rely on the passive movement of molecules through their cell membranes.
- In summary, simple diffusion is a spontaneous process that allows for the movement of molecules from areas of high concentration to low concentration without the need for energy. It plays a vital role in various biological processes, including gas exchange, nutrient uptake, and waste removal in organisms.
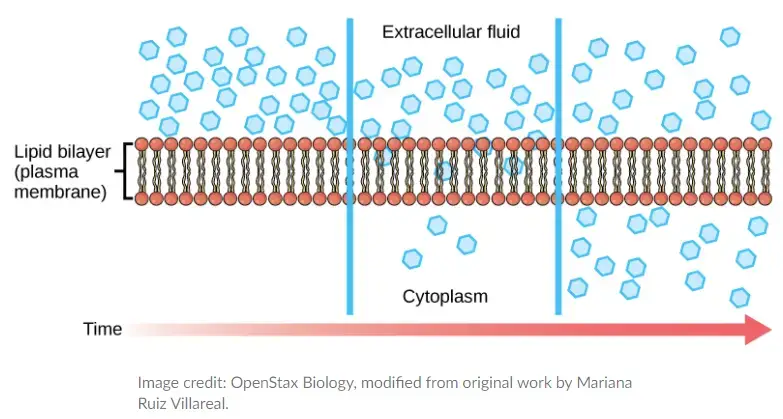
Factors affecting on Simple Diffusion
- Concentration Gradient: The extent of the concentration gradient plays a crucial role in simple diffusion. A greater difference in the concentration of a molecule on either side of the membrane leads to a higher diffusion rate. However, as the concentrations approach equilibrium, the rate of diffusion decreases.
- Molecular Weight: The mass of molecules also influences diffusion. Materials with higher molecular weight diffuse at a slower rate compared to those with lower molecular weight.
- Temperature: The temperature of the biological system directly impacts the rate of diffusion. Increasing the system’s temperature enhances the rate of diffusion, while decreasing the temperature reduces it. Thus, diffusion rate is directly proportional to temperature.
- Solvent Density: The density of the solvent inversely affects diffusion rate. Higher solvent density leads to a lower diffusion rate, as molecules encounter more resistance in moving across the system. Conversely, lower solvent density reduces the resistance, enabling molecules to cross the permeable barrier more readily.
- Solubility: Solubility plays a significant role in diffusion. Non-polar and lipid-soluble materials exhibit faster diffusion rates compared to polar and non-lipid materials, as they can easily pass through the plasma membrane.
- Surface Area and Membrane Thickness: The surface area of the plasma membrane directly influences the rate of diffusion. A larger surface area allows for a higher diffusion rate across the membrane. On the other hand, membrane thickness has an inverse relationship with diffusion rate. A thicker membrane impedes diffusion, leading to a lower rate.
- Distance Traveled: The distance that a substance must travel affects the diffusion rate. Longer distances result in slower diffusion rates. Smaller-sized or flattened cells facilitate faster transport of molecules through passive diffusion by reducing the distance that substances need to traverse.
In summary, the factors affecting simple diffusion include the concentration gradient, molecular weight, temperature, solvent density, solubility, surface area of the membrane, membrane thickness, and the distance that substances need to travel. Understanding these factors helps elucidate the mechanisms and dynamics of simple diffusion in biological systems.
2. Facilitated diffusion
- Facilitated diffusion (also known as facilitated transport or passive-mediated transport) is the spontaneous passive transfer of molecules or ions across a biological membrane by certain transmembrane integral proteins.
- Being passive, assisted transport does not directly require chemical energy from ATP hydrolysis in the transport phase itself; instead, molecules and ions travel along their concentration gradient, indicating the diffusive nature of the transport.
- In numerous ways, facilitated diffusion differs from simple diffusion.
- The transport is dependent on the molecular binding of the payload to the membrane-embedded channel or carrier protein.
- Unlike free diffusion, which is linear in the concentration difference between the two phases, the rate of assisted diffusion is saturable with regard to the concentration difference between the two phases.
- Due to the presence of an active binding event, the temperature dependency of assisted transport is significantly different from that of free diffusion, where the dependence on temperature is minimal.
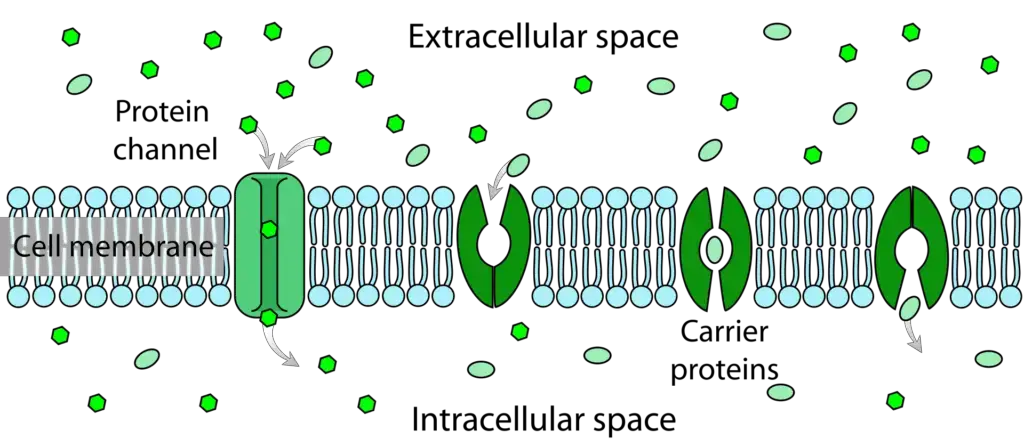
- Due to the hydrophobic nature of the fatty acid tails of the phospholipids that make up the lipid bilayer, polar molecules and large ions dissolved in water cannot pass freely across the plasma membrane.
- Small, non-polar molecules, such as oxygen and carbon dioxide, can easily diffuse through the membrane. Hence, tiny polar molecules are transported via transmembrane channels formed by proteins.
- These channels are gated, which means they can open and close, hence deregulating the passage of ions or tiny polar molecules across membranes, sometimes against the osmotic gradient. Larger molecules are transported by transmembrane carrier proteins, such as permeases, whose conformation changes as the molecules are moved across the membrane (e.g. glucose or amino acids).
- In water, nonpolar compounds, such as retinol or lipids, are insoluble. Water-soluble carriers move them through watery compartments of cells or extracellular space (e.g. retinol binding protein). The metabolites are not changed since enhanced diffusion requires no energy.
- Only permease is capable of changing its form to transport metabolites. The process of transporting a changed metabolite across a cell membrane is known as group translocation transport.
- Glucose, sodium ions, and chloride ions are examples of chemicals and ions that must easily pass the plasma membrane but are virtually impermeable to the lipid bilayer of the membrane.
- Thus, their transport must be “facilitated” by transmembrane proteins that provide an alternate route or bypass mechanism. Proteins such as glucose transporters, organic cation transport proteins, urea transporter, monocarboxylate transporter 8 and monocarboxylate transporter 10 mediate this process.
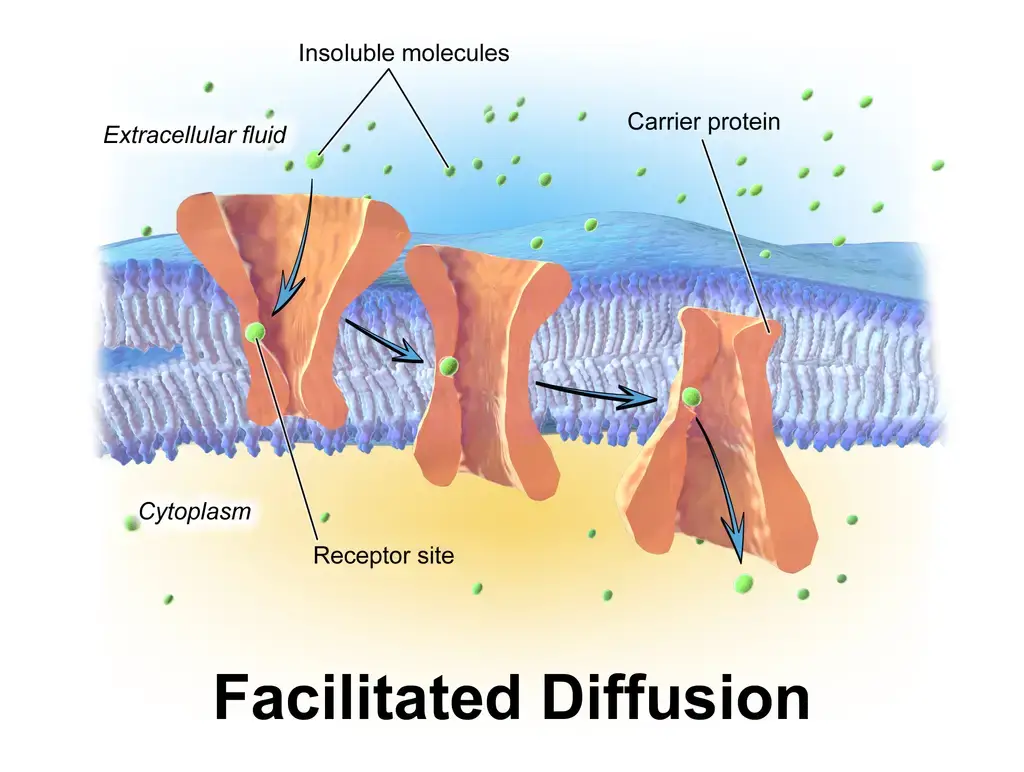
a. Channel-mediated transport
Channel-mediated transport is a process in which molecules or ions spontaneously pass through specific transmembrane integral proteins, known as transport proteins, across the biological membrane.
These transport proteins, called channel proteins, are selective in the materials they transport. They have hydrophilic domains that are exposed to the intracellular and extracellular fluids, and a hydrophobic channel in their core, which forms a hydrated opening through the membrane layers.
The structure of channel proteins allows them to create a passage that prevents polar molecules from coming into direct contact with the non-polar central layer of the membrane. An example of channel-mediated transport is the movement of water through specialized channels called aquaporins.
Channel proteins can be categorized into two types:
- Gated channels: These channels are regulated by channel gates, which control the transport of molecules. They require specific signals, such as voltage changes, mechanical stress, or the binding of a ligand, to open and allow the passage of molecules.
- Non-gated channels: Unlike gated channels, non-gated channels are not regulated by any specific signals. They are always open and available for the continuous transport of molecules.
In different parts of the renal tubules in the kidney, both gated and non-gated channels can be found. For instance, nerve cells and muscle cells involved in the transmission of electric impulses possess gated channels for sodium, potassium, and calcium in their membranes.
In summary, channel-mediated transport involves the spontaneous movement of molecules or ions across the biological membrane through specific transport proteins known as channel proteins. These proteins form hydrophilic domains that interact with the surrounding fluids and create a hydrophobic channel through the membrane, allowing for selective transport. Channel proteins can be either gated, requiring specific signals for opening, or non-gated, always open for continuous transport.
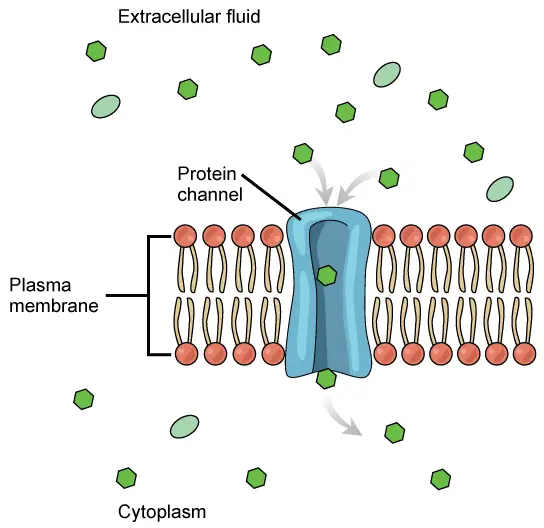
b. Carrier-mediated transport
- Carrier-mediated transport is a mechanism by which molecules are transported across the biological membrane with the help of carrier proteins. These carrier proteins bind to the specific molecules to be transported, which triggers a conformational change in the protein. This change in shape allows the molecule to be moved across the membrane.
- In carrier-mediated transport, the movement of molecules is driven by the concentration gradient. When there is a higher concentration of the molecule on one side of the membrane compared to the other side, the carrier protein binds to the molecule and facilitates its transport across the membrane to the side with lower concentration.
- A well-known example of carrier-mediated transport is the group of carrier proteins called glucose transport proteins, or GLUTs. These carrier proteins are responsible for transporting glucose and other hexose sugars across plasma membranes in the body. GLUTs play a crucial role in maintaining glucose homeostasis and providing cells with the necessary energy source.
- Carrier-mediated transport is a highly specific and regulated process, as carrier proteins have binding sites that are specific to certain molecules. This specificity allows for selective transport of molecules across the membrane, ensuring the efficient uptake and distribution of essential substances in biological systems.
- In summary, carrier-mediated transport involves the use of carrier proteins to facilitate the movement of molecules across the biological membrane. These proteins undergo conformational changes upon binding to specific molecules and transport them based on the concentration gradient. Examples of carrier-mediated transport include the transportation of glucose and other sugars through plasma membranes by glucose transport proteins (GLUTs).
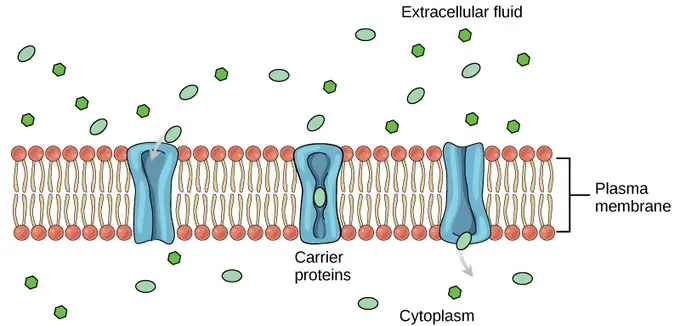
Factors Affecting Facilitated Diffusion
Facilitated diffusion, a type of passive transport that relies on specific carrier proteins, is influenced by several factors:
- Temperature: The rate of facilitated diffusion is directly influenced by temperature. As temperature increases, the energy of molecules also increases, resulting in faster transfer of molecules across the membrane.
- Concentration: The concentration gradient of molecules on both sides of the membrane plays a crucial role in facilitated diffusion. The direction of movement is determined by the concentration difference. Molecules move from an area of higher concentration to an area of lower concentration.
- Diffusion Distance: The rate of diffusion is inversely proportional to the distance over which diffusion occurs. Longer diffusion distances result in slower diffusion rates. A shorter distance between the molecule and the carrier protein facilitates faster and more efficient diffusion.
- Size of Molecules: The size of molecules also affects the rate of facilitated diffusion. Smaller molecules tend to move more rapidly than larger molecules. The diffusion rate is directly related to the size of the molecule, with smaller molecules diffusing more quickly than larger ones.
3. Filtration
- Filtering is a physical separation procedure that separates solid matter and fluid from a mixture by passing only the fluid through a filter media with a complicated structure.
- Solid particles that are too large to pass through the filter medium are referred to as oversize, while the fluid that does pass through is known as the filtrate.
- Oversize particles can create a filter cake on top of the filter and obstruct the filter lattice, preventing the fluid phase from passing through the filter. This phenomenon is known as blinding. The size of the largest particles that can pass through a filter is known as the filter’s effective pore size.
- The separation of solids and liquids is poor; some liquid will contaminate the solids, and the filtrate will contain minute particles (depending on the pore size, filter thickness and biological activity).
- There are biological, geological, and industrial forms of filtration. Filtration happens in both natural and manmade systems.
- In addition to separating particulates from a fluid stream, biological and physical filtration devices also remove chemical species and living organisms by entrainment, phagocytosis, adsorption, and absorption. Slow sand filters and trickling filters are examples.
- It is often used as a broad word for microphagy, in which organisms filter minute food particles from their surroundings through a variety of mechanisms. Filter-feeding organisms range in size from the microscopic Vorticella to the basking shark, one of the largest fishes, and the baleen whales.
- Filtration is used to separate particles and fluid in a suspension, with the fluid being a liquid, gas, or supercritical fluid. One or both of the components may be separated, depending on the application.
- As a physical process, filtration permits the separation of substances with distinct chemical compositions. A solvent is selected that dissolves one component, but not the other. By dissolving the mixture in the selected solvent, one component will enter the solution and pass through the filter, while the remaining component will be retained.
- In chemical engineering, filtration is commonly used. It can be integrated with other unit operations to process the feed stream, such as in the biofilter, a filter and biological digesting device.
- In sieving, separation happens at a single perforated layer, whereas in filtration, separation occurs at many perforated layers (a sieve). In sieving, particles too large to pass through the sieve’s holes are kept (see particle size distribution). In filtration, a multilayer lattice holds particles incapable of following the filter’s tortuous pathways. Oversize particles may form a cake layer on the filter surface and may also obstruct the filter lattice, preventing the fluid phase from passing through the filter (blinding). Commercially, the word filter is applied to membranes whose separation lattice is so thin that the surface becomes the primary zone of particle separation, despite the fact that these products might also be referred to as sieves.
- Filtration is distinct from adsorption, which relies on surface charge for separation. Commercially, some adsorption devices incorporating activated charcoal and ion-exchange resin are referred to as filters, despite the fact that filtration is not their primary mechanical function.
- Because there is no filter medium, filtration varies from magnetic removal of magnetic impurities from fluids (usually lubricating oil, coolants, and fuel oils). There are commercial devices referred to as “magnetic filters,” although their name does not reflect their operation.
- In biological filters, particles of excessive size are captured and ingested, and the resulting metabolites may be discharged. In animals (including humans), for instance, renal filtration removes waste from the blood, and in water treatment and sewage treatment, undesired elements are removed by adsorption into a biological film developed on or within the filter media, as in slow sand filtration.
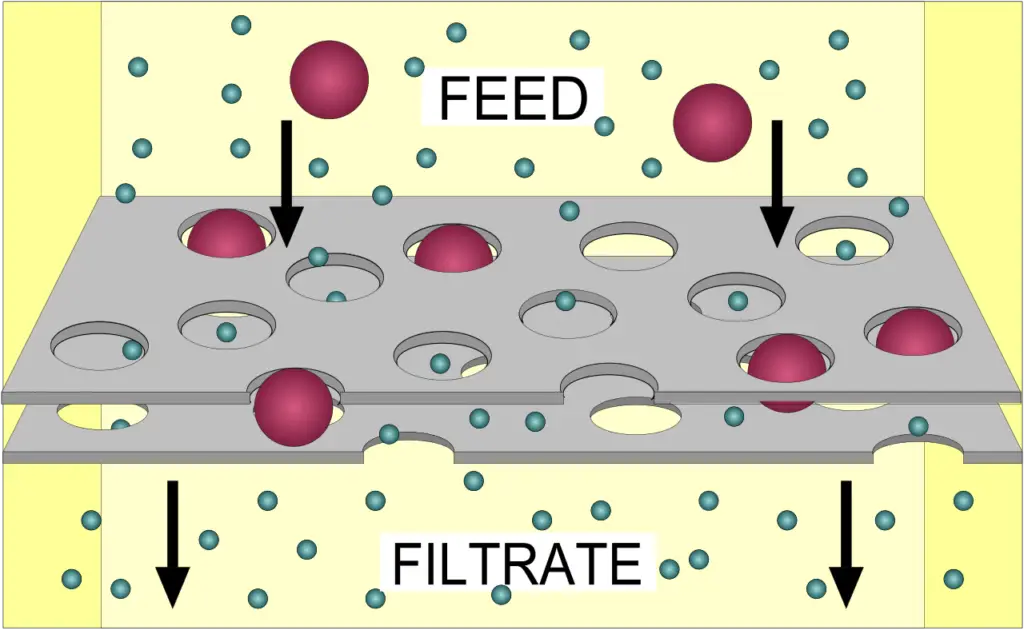
4. Osmosis
- Osmosis is the spontaneous net movement or diffusion of solvent molecules through a selectively permeable membrane from a region of high water potential (region of lower solute concentration) to a region of low water potential (region of higher solute concentration) in the direction that tends to equalise the solute concentrations on both sides.
- It can also be used to describe a physical process in which any solvent travels through a selectively permeable barrier (permeable to the solvent, but not to the solute) that separates two solutions of different concentrations. It is possible to make osmosis function.
- Osmotic pressure is defined as the external pressure necessary to prevent any net passage of solvent across a membrane. Osmotic pressure is a colligative property, which means that it depends on the solute’s molar concentration but not its identity.
- Biological systems require osmosis because biological membranes are semipermeable. In general, these membranes are impermeable to big and polar molecules, such as ions, proteins, and polysaccharides, but they are permeable to non-polar or hydrophobic molecules, such as lipids, and tiny molecules, such as oxygen, carbon dioxide, nitrogen, and nitric oxide.
- Solubility, charge, or chemistry, as well as solute size, determine permeability. Aquaporins enable the diffusion of water molecules through the phospholipid bilayer of the plasma membrane, tonoplast membrane (vacuole), and organelle membranes (small transmembrane proteins similar to those responsible for facilitated diffusion and ion channels).
- Osmosis is the fundamental mechanism for transporting water into and out of cells. The majority of a cell’s turgor pressure is maintained through osmosis across the cell membrane between the cell’s interior and its comparatively hypotonic surroundings.
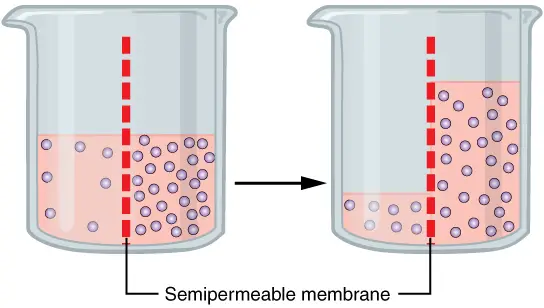
The diffusion of water molecules through a selectively permeable membrane is osmosis. The net migration of water molecules from a solution with a high water potential through a partially permeable barrier into a region with a low water potential. A cell with a reduced negative water potential will absorb water, but this also depends on other elements such as solute potential (the pressure of solute molecules within the cell) and pressure potential (external pressure e.g. cell wall). There are three different kinds of Osmosis solutions: isotonic, hypotonic, and hypertonic. Isotonic solution occurs when the extracellular solute concentration is equal to the intracellular solute concentration. In an isotonic solution, water molecules continue to travel between the solutions, but at the same rate in both directions. As a result, water movement is balanced between the inside and outside of the cell. A hypotonic solution is one in which the concentration of solutes outside the cell is lower than the concentration inside. In hypotonic solutions, water enters the cell via the concentration gradient (from higher to lower water concentrations). This can lead to cell swelling. In this fluid, cells without a cell wall, such as animal cells, could explode. A hypertonic solution is one in which the solute concentration is greater than the concentration within the cell. In hypertonic solution, water will migrate out of the cell, causing it to shrink.
Mechanism
- The mechanism responsible for driving osmosis is typically represented in biology and chemistry textbooks as either the dilution of water by solute (resulting in a lower concentration of water on the higher solute concentration side of the membrane and thus a diffusion of water along a concentration gradient) or as a solute’s attraction to water (resulting in less free water on the higher solute concentration side of the membrane and therefore net movement of water towards the solute). Both of these claims have been rejected with absolute certainty.
- The inapplicability of the diffusion model of osmosis is caused by the fact that osmosis can move water across a membrane towards a higher concentration of water.
- The “bound water” concept is challenged by the fact that osmosis is independent of the solute molecules’ colligative property or hydrophilicity.
- It is difficult to define osmosis without a mechanical or thermodynamic explanation, but fundamentally, there is an interaction between the solute and water that opposes the pressure that otherwise free solute molecules would exert. Notable is the ability to transfer ambient heat into mechanical energy (water rising).
- Many thermodynamic explanations are provided for the idea of chemical potential and how the function of water in a solution differs from that of pure water as a result of the increased pressure and the presence of a counteracting solute such that the chemical potential remains intact.
- The virial theorem demonstrates that the attraction between the molecules (water and solute) lowers the pressure; consequently, the pressure exerted by water molecules on each other in solution is less than in pure water, allowing pure water to “force” the solution until the pressure reaches equilibrium.
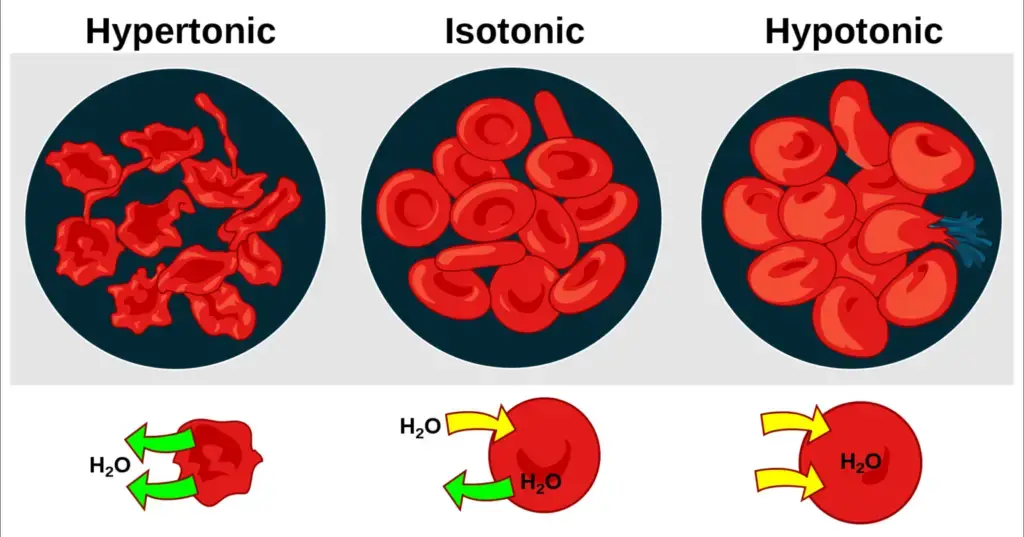
Passive Transport using Membrane Proteins
- Occasionally, molecules are unable to traverse the cell membrane on their own. These chemicals require transport proteins to promote their movement across the membrane, a process referred to as facilitated diffusion. Attached to the cell membrane, these specialised proteins are referred to as channel proteins or carrier proteins (Figure). In reality, they traverse the cell membrane from the inside to the exterior of the cell.
- Channel proteins create an open channel or passageway for molecules to cross the cell membrane. Many channel proteins permit ion diffusion. Ions are atomic charges. It is difficult to cross the cell membrane without aid due to the charge. Specificity of channel proteins for the molecules they transport. For instance, sodium ions cross the membrane via a sodium-specific channel protein.
- Carrier proteins bind the chemicals and transport them across the cell membrane. These proteins bind a molecule on one side of the membrane, transform as they transport it across the membrane, and deposit it on the opposite side. Although though proteins are involved in each of these transport mechanisms, neither requires energy. Hence, these remain forms of passive mobility.
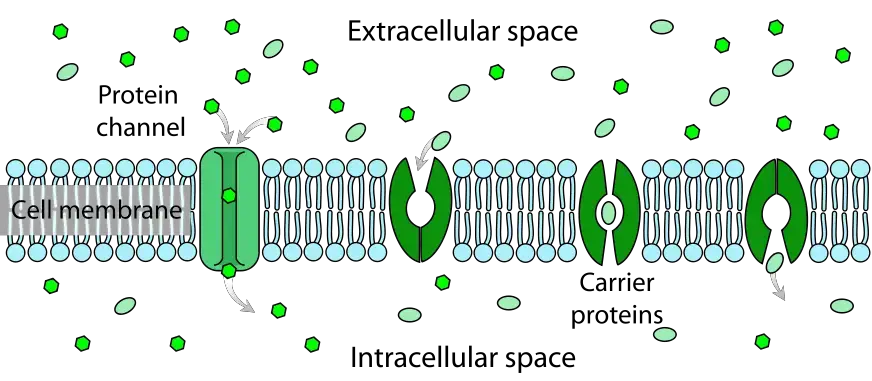
Significance of Passive Transport
The significance of passive transport is paramount for cellular life and maintaining normal function within organisms. Here are some key points highlighting its importance:
- Essential Exchange: Passive transport allows for the exchange of biological molecules necessary for cellular function. It facilitates the incorporation of vital substances and the removal of waste products, ensuring the proper functioning of cells.
- Blood-Brain Barrier: Passive diffusion is crucial in the blood-brain barrier, enabling specific molecules, such as sodium thiopental, to cross the membrane and reach the brain.
- Placental Exchange: Passive diffusion, particularly across the placenta, plays a vital role in the exchange of solute particles between the mother and fetus, allowing for essential nutrients and oxygen to reach the developing fetus.
- Small Molecular Weight Transport: Passive forms of transport, such as diffusion and osmosis, facilitate the movement of small molecular weight materials across membranes. This includes the transportation of digested food molecules (e.g., amino acids, glucose) from the intestine to the blood and the elimination of waste products (e.g., carbon dioxide, urea) from body cells into the bloodstream.
- Gas Exchange: Passive transport is involved in the exchange of gases like oxygen and carbon dioxide. Oxygen moves from areas of high concentration (in the air sac) to lower concentration (in the blood), while carbon dioxide moves from areas of high concentration (in the blood) to lower concentration (in the air sac).
- Osmosis and Nutrient Distribution: Osmosis, a form of passive transport, plays a critical role in the distribution of essential nutrients throughout the body. It allows for the passage of liquid solvents through semipermeable cell membranes, ensuring the proper delivery of nutrients to cells.
- Renal Filtration: In animals, including humans, passive transport is instrumental in renal filtration, which removes waste products from the blood, maintaining overall health and homeostasis.
In summary, passive transport is of significant biological importance as it facilitates essential exchanges, enables nutrient distribution, aids in waste removal, and supports the overall functioning of cells and organisms. Its role in various physiological processes highlights its indispensable nature in maintaining life.
Differences Between Simple Diffusion and Facilitated Diffusion
Simple diffusion and facilitated diffusion, while sharing similarities, differ in several key aspects:
- Requirement of Proteins: Facilitated diffusion relies on channel or carrier proteins to facilitate the transport of molecules across the membrane. In contrast, simple diffusion does not require the assistance of proteins and occurs directly through the lipid bilayer.
- Transport Rate: Facilitated diffusion exhibits a saturable transport rate because it is dependent on the availability of channel or carrier proteins. Only one molecule is transported at a time through facilitated diffusion. On the other hand, simple diffusion shows a linear relationship with the concentration difference. The diffusion rate increases with a higher concentration gradient, and there is no saturation stage due to its independent nature from carrier proteins.
- Diffusion Rate: The rate of diffusion in facilitated transport is generally slower than that of channel-mediated transport. Channel proteins enable diffusion at a rapid rate, transporting tens of millions of molecules per second. In contrast, carrier proteins facilitate diffusion at a comparatively slower rate, ranging from a thousand to a million molecules per second.
- Temperature Dependence: Facilitated diffusion is more temperature-dependent compared to simple diffusion. The binding events involved in facilitated diffusion are activated by temperature changes. Therefore, temperature variations have a more pronounced effect on facilitated diffusion. In contrast, simple diffusion is less affected by temperature changes and exhibits a milder temperature dependence.
Differences | Simple Diffusion | Facilitated Diffusion |
---|---|---|
Requirement of Proteins | No proteins involved | Requires channel or carrier proteins |
Transport Rate | Linear relationship with concentration difference | Saturable transport rate, one molecule at a time |
Diffusion Rate | Generally faster (channel-mediated transport) | Slower (carrier-mediated transport) |
Temperature Dependence | Mild temperature dependence | More temperature-dependent, activated binding events |
Example | Oxygen, carbon dioxide, ethanol | Glucose transport through GLUT proteins |
Channels and Carrier Proteins for Passive Transport (Facilitated Transport Protein)
Some molecules, such as carbon dioxide and oxygen, can diffuse directly across the plasma membrane, while others require assistance to traverse its hydrophobic interior. In facilitated diffusion, a type of passive transport, molecules diffuse across the plasma membrane with the aid of membrane proteins, such as channels and transporters. These molecules have the potential to diffuse into (or out of) the cell by traveling down their concentration gradient. However, because they are charged or polar, they cannot cross the phospholipid layer without assistance. Facilitated transport proteins shield these molecules from the hydrophobic membrane core and provide a pathway for their passage.
Channels
- Channels are integral membrane proteins that traverse the lipid bilayer, forming hydrophilic tunnels that span the membrane. These tunnels allow specific target molecules to pass through the membrane via diffusion.
- One of the defining features of channels is their selectivity. Each channel is designed to accept only one type of molecule or a few closely related molecules for transport. This selectivity ensures precise and controlled movement of specific substances across the membrane.
- Passage through a channel protein is advantageous for polar and charged compounds. These molecules can avoid the hydrophobic core of the plasma membrane, which would otherwise impede or slow down their entry into the cell. By utilizing channels, these compounds can traverse the membrane more efficiently.
- Channel proteins exhibit different modes of operation. Some channels remain open at all times, allowing continuous flow of molecules. On the other hand, other channels are “gated,” meaning they can open or close in response to specific signals. These signals may include electrical impulses or the binding of a particular molecule.
- An example of channel proteins is aquaporins. Aquaporins serve as channels that facilitate the rapid passage of water molecules across the membrane. They play crucial roles in various biological contexts, such as in plant cells, red blood cells, and specific regions of the kidney, where they minimize water loss through urine.
- In summary, channels are specialized membrane proteins that create hydrophilic tunnels across the membrane, enabling the diffusion of specific molecules. They exhibit selectivity, allowing only specific types of molecules to pass through. Channels provide a means for polar and charged compounds to bypass the hydrophobic core of the membrane. Whether open or gated, channels serve vital roles in facilitating the movement of substances across the membrane.
Carrier proteins
- Carrier proteins are a distinct class of transmembrane proteins that play a crucial role in facilitated transport across the cell membrane.
- Unlike channels that form hydrophilic tunnels, carrier proteins possess the ability to change their shape. This shape change allows them to transport a target molecule from one side of the membrane to the other.
- Similar to channel proteins, carrier proteins also exhibit selectivity, typically binding and transporting one or a few specific substances. This selectivity ensures that only the intended molecules are transported across the membrane.
- The binding of the target molecule to the carrier protein triggers a conformational change in the protein structure. This change in shape enables the carrier protein to move the molecule to the opposite side of the membrane. In this way, carrier proteins actively facilitate the transport of molecules by providing a pathway for their movement.
- It’s important to note that carrier proteins involved in facilitated diffusion do not actively pump molecules against a concentration gradient. Instead, they allow hydrophilic molecules to move down an existing concentration gradient, ensuring that the process remains passive.
- Carrier proteins are vital for the transport of various essential substances in biological systems. By facilitating the movement of specific molecules across the cell membrane, they contribute to maintaining homeostasis and supporting cellular functions.
- In summary, carrier proteins are transmembrane proteins that change shape to transport target molecules across the membrane. They exhibit selectivity, and their shape change is triggered by the binding of the target molecule. Unlike pumps, carrier proteins in facilitated diffusion enable the passive movement of hydrophilic molecules down a concentration gradient. Their involvement is crucial for efficient transportation and the proper functioning of biological systems.
Examples of Passive Transport
1. BAC Goin’ Up
- When it comes to alcohol consumption, understanding how the body processes ethanol, the primary ingredient in alcoholic beverages, is crucial. Once ethanol enters the body, it quickly enters the bloodstream, leading to an increase in Blood Alcohol Concentration (BAC).
- The rapid increase in BAC can be attributed to the remarkable ability of ethanol molecules to undergo simple diffusion. Simple diffusion is a form of passive transport that occurs without the need for energy expenditure. Due to their extremely small size, ethanol molecules can easily pass through cell and tissue membranes, allowing them to enter the bloodstream effortlessly.
- This efficient diffusion process explains why individuals can have a measurable BAC even without feeling noticeably intoxicated. It also clarifies why some people can become significantly intoxicated within a matter of minutes after consuming a shot or a drink.
- The ease with which ethanol molecules navigate through the body’s membranes plays a significant role in the effects alcohol has on the body. It underscores the importance of understanding the impact of alcohol consumption and the potential risks associated with high BAC levels.
- In summary, the swift rise in BAC after consuming alcohol is due to the ability of ethanol molecules to undergo simple diffusion. Their minuscule size enables them to pass through cell and tissue membranes effortlessly, affecting the body without requiring any additional energy.
2. Neurotransmission Impossible
- Although neurons play a complex role in our brain function, it’s fascinating to discover that their communication relies on a process that seems deceptively simple. Despite their intricacy, neurons actually rely on passive transport to transmit signals.
- Within the intricate network of synapses in our brain, sodium (Na+) and potassium (K+) ions play a critical role. These ions operate along a concentration gradient. In a resting neuron, the concentration of K+ ions is higher on the inside, while the concentration of Na+ ions is higher on the outside. However, during active potential (when the neuron fires), specialized protein “pumps” on the outer membrane allow Na+ ions to enter the neuron while K+ ions exit.
- Similar to the ethanol molecules mentioned in Example 1, Na+ and K+ ions move from areas of high concentration to areas of lower concentration. However, they require assistance to accomplish this task. Due to their need for help, these ions undergo facilitated diffusion rather than simple diffusion.
- Facilitated diffusion ensures that the Na+ and K+ ions can traverse the cell membrane efficiently and in the appropriate direction. This process involves the presence of specific channel proteins and carrier proteins that aid in the movement of these ions. These proteins create channels or binding sites that allow selective transport of the ions across the neuronal membrane.
- In summary, the communication between neurons relies on a process called facilitated diffusion, where sodium (Na+) and potassium (K+) ions move across the cell membrane with the assistance of specialized proteins. This process ensures the efficient transmission of signals within the intricate web of synapses in our brain.
3. (Not) a Pile of Waste
- While we may associate our intestines with the elimination of waste, they serve a far more essential purpose: extracting nutrients from the food we consume. Despite the relatively larger size of vitamins and minerals compared to ethanol and ions, our bodies employ a passive transport mechanism to extract them.
- This mechanism is known as filtration, which involves the separation of solids from liquids and liquids from gases using a membrane. Bringing it back to our example, we can observe that nutrients, in liquid form, undergo separation from waste, in solid form, by traversing the intestinal membrane and entering the bloodstream.
- The intestinal membrane acts as a selective barrier, allowing the passage of small molecules, such as nutrients, while impeding the movement of larger solid waste particles. Through this process, nutrients are efficiently absorbed from the digested food and transported into the bloodstream for distribution throughout the body.
- This filtration process is vital for our overall health and well-being. By extracting essential nutrients from our food, our intestines ensure that our bodies receive the nourishment they require to function optimally.
- In summary, our intestines play a crucial role in extracting nutrients from our food through a process called filtration. This passive transport mechanism allows the separation of nutrients from solid waste by selectively allowing small molecules to pass through the intestinal membrane and enter the bloodstream. By efficiently absorbing nutrients, our bodies obtain the necessary sustenance for proper functioning.
4. Fresh Veggies
- Have you ever noticed how soaking a raisin in water can transform it back into a plump grape? This intriguing phenomenon is not just about rehydrating the raisin; it exemplifies another type of passive transport called osmosis.
- Unlike other forms of passive transport, osmosis aims to establish equilibrium rather than simply move along a concentration gradient. When a raisin is soaked in water, water molecules pass through the raisin’s membrane not only to reach the less-concentrated interior but also to equalize the grape with its external environment. This process can occur with various fruits and vegetables that have undergone some degree of dehydration.
- Osmosis allows for the rehydration of dehydrated produce, revitalizing its texture, flavor, and appearance. By immersing dried fruits or vegetables in water, the water molecules move across the produce’s cellular membranes, reestablishing a balance of moisture and restoring the produce to a fresh and plump state.
- This process is particularly noticeable with dehydrated fruits like raisins or dried apricots, where the osmotic flow of water can reverse the shriveled appearance and restore their juiciness. It is a testament to the remarkable properties of osmosis and the ability of water to traverse cellular membranes to achieve equilibrium.
- In summary, the process of osmosis plays a significant role in rehydrating dehydrated produce such as raisins or dried fruits. By immersing these fruits in water, osmosis allows water molecules to move across the cellular membranes, equalizing the water concentration and restoring the produce to a fresh and revitalized state.
FAQ
What is passive transport?
Passive transport is the movement of molecules or ions across a cell membrane from an area of high concentration to an area of low concentration without the input of energy.
What are the two types of passive transport?
The two types of passive transport are diffusion and facilitated diffusion.
How does diffusion work in passive transport?
Diffusion is the movement of molecules or ions from an area of high concentration to an area of low concentration. It occurs until the concentration of the substance is the same on both sides of the membrane.
What is facilitated diffusion in passive transport?
Facilitated diffusion is the movement of molecules or ions across a membrane with the help of a transport protein. It is used for molecules that cannot diffuse through the membrane on their own.
Does passive transport require energy input?
No, passive transport does not require energy input. It occurs spontaneously, driven by the concentration gradient.
What are the factors that affect the rate of passive transport?
The factors that affect the rate of passive transport are the concentration gradient, temperature, surface area, and the size of the molecule.
What types of molecules can pass through the cell membrane by passive transport?
Small and nonpolar molecules, such as oxygen and carbon dioxide, can pass through the cell membrane by passive transport.
Can water molecules pass through the cell membrane by passive transport?
Yes, water molecules can pass through the cell membrane by passive transport through a process called osmosis.
What is the role of ion channels in passive transport?
Ion channels are protein channels that allow the passage of ions across the cell membrane by passive transport. They are specific to certain ions and are regulated by various mechanisms.
What is the difference between active and passive transport?
Active transport requires energy input to move molecules or ions against their concentration gradient, while passive transport occurs spontaneously without the input of energy, moving molecules or ions along their concentration gradient.
References
- Feher, J. (2017). Passive Transport and Facilitated Diffusion. Quantitative Human Physiology, 161–169. doi:10.1016/b978-0-12-800883-6.00015-x
- Chen I, Lui F. Physiology, Active Transport. [Updated 2022 Sep 12]. In: StatPearls [Internet]. Treasure Island (FL): StatPearls Publishing; 2022 Jan-. Available from: https://www.ncbi.nlm.nih.gov/books/NBK547718/
- https://flexbooks.ck12.org/cbook/ck-12-biology-flexbook-2.0/section/2.13/primary/lesson/passive-transport-bio/
- https://ib.bioninja.com.au/standard-level/topic-1-cell-biology/14-membrane-transport/types-of-transport.html
- https://www.aakash.ac.in/important-concepts/biology/passive-transport
- https://humanbiology.pressbooks.tru.ca/chapter/4-7-passive-transport/
- https://study.com/academy/lesson/active-and-passive-transport-across-the-cell-membrane.html
- https://conductscience.com/passive-transport/
- https://www.diffen.com/difference/Active_Transport_vs_Passive_Transport
- https://openoregon.pressbooks.pub/mhccmajorsbio/chapter/passive-transport-diffusion/
- https://www.futurelearn.com/info/courses/introduction-to-human-cells-the-basis-of-life/0/steps/330524
- https://www.biologyonline.com/dictionary/passive-transport
- https://www.khanacademy.org/test-prep/mcat/cells/transport-across-a-cell-membrane/a/passive-transport-and-active-transport-across-a-cell-membrane-article
- https://biologydictionary.net/passive-transport/
- https://opentextbc.ca/biology/chapter/3-5-passive-transport/
- https://www.sciencedirect.com/topics/biochemistry-genetics-and-molecular-biology/passive-transport