What is cryo-EM?
- Cryo-electron microscopy (cryo-EM) is a sophisticated imaging technique designed to visualize the three-dimensional (3D) structures of biological molecules and complexes at nearly atomic resolution. This method involves rapidly freezing samples to temperatures below -150 °C, which immobilizes them in vitreous ice. The frozen specimens are then examined using an electron microscope. In this process, an electron beam traverses the sample, producing a series of two-dimensional (2D) projections from multiple angles. Computational algorithms subsequently reconstruct a 3D model from these 2D images.
- Cryo-EM differentiates itself from conventional electron microscopy (EM) primarily through its use of cryogenic temperatures. This allows for the observation of radiation-sensitive specimens without causing significant damage, a notable advantage over traditional methods. Cryo-EM encompasses several sub-disciplines, including cryo-electron tomography, single-particle cryo-EM, and electron crystallography. Each of these methods is employed to investigate different biological contexts, ranging from whole cells and viruses to individual protein molecules.
- Cryo-EM’s applications are diverse and extend across various biological research areas. For instance, cryo-electron tomography provides 3D reconstructions of entire cells and viral particles, while single-particle cryo-EM focuses on analyzing isolated macromolecules by averaging numerous 2D projections to build detailed 3D structures. Furthermore, cryo-EM is often combined with other techniques, such as X-ray crystallography and nuclear magnetic resonance (NMR) spectroscopy, to gain comprehensive insights into molecular structures.
- The ongoing advancements in cryo-EM are regularly reviewed in scientific literature, highlighting improvements in methodological approaches and the expanding range of biological systems that can be studied. For those new to this field, understanding cryo-EM’s principles and its applications provides a valuable perspective on its role in structural biology. Key resources, such as the Electron Microscopy Data Bank (EMDB) and the Protein Data Bank (PDB), offer extensive repositories of cryo-EM data, supporting further research and discovery in this rapidly evolving discipline.
Principle of cryo EM
- The working principle of cryo-electron microscopy (cryo-EM) revolves around the advanced imaging of biological specimens using electron beams in a cryogenic state. This technique builds on the foundational principles of electron microscopy but addresses key limitations associated with radiation damage to biological materials.
- In electron microscopy, imaging is achieved by directing an electron beam through a specimen. Unlike light microscopy, which uses visible light to form images through optical lenses, electron microscopy employs electrons accelerated under high vacuum at voltages typically ranging from 80 to 300 kV. The electrons interact with the specimen, and their scattering is captured and focused by electromagnetic lenses to create an image. The key advantage of electron microscopy over light microscopy is its higher resolving power, due to the shorter wavelength of electrons compared to visible light, allowing for the visualization of structures at much finer resolutions.
- However, biological specimens are particularly susceptible to damage from electron irradiation. The interaction of electrons with organic material can lead to bond breakage and the generation of free radicals, causing significant structural alterations. To mitigate this issue, cryo-EM employs a cryogenic approach where specimens are rapidly frozen, typically in liquid ethane or nitrogen, to preserve them in a glass-like state. This rapid vitrification of the sample prevents the formation of ice crystals that could otherwise damage the specimen.
- At cryogenic temperatures, the extent of radiation damage is significantly reduced, allowing for higher electron doses without compromising the structural integrity of the specimen. This is because the lower temperatures slow down the molecular movements and reduce the formation of damaging free radicals. Imaging at temperatures around liquid nitrogen or liquid helium further minimizes radiation effects, enabling the acquisition of high-resolution images.
- In addition to cryogenic preservation, cryo-EM also uses computational techniques to improve image quality. One such method involves averaging multiple images of identical particles or structures. This approach, known as single-particle reconstruction, helps enhance the signal-to-noise ratio by combining data from numerous low-dose images. By averaging these images, noise is reduced, and clearer, more accurate 3D reconstructions can be achieved.
- Cryo-EM thus combines the benefits of low-temperature preservation and image averaging to provide detailed structural insights into biological macromolecules, ranging from individual proteins to complex cellular structures. This method represents a significant advancement in structural biology, allowing researchers to observe and analyze biological entities at unprecedented levels of detail.
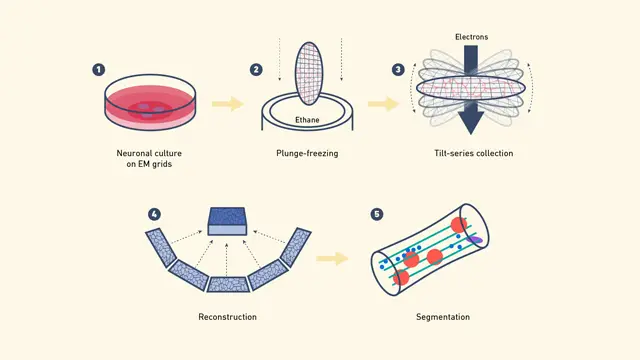
Types of cryo EM
Cryo-electron microscopy (cryo-EM) encompasses several specialized techniques, primarily divided into two main types: cryo-transmission electron microscopy (cryo-TEM) and cryo-scanning electron microscopy (cryo-SEM). Each technique offers distinct advantages for studying biological specimens in their native, vitrified state.
- Cryo-Transmission Electron Microscopy (Cryo-TEM):
- Principle: In cryo-TEM, the sample is vitrified and loaded into a transmission electron microscope (TEM). An electron beam is directed through the sample, and the electrons interact with the specimen, resulting in scattering and absorption.
- Imaging: This interaction produces a series of two-dimensional (2D) projection images of the sample. To construct a three-dimensional (3D) representation, the sample is tilted at various angles, and multiple 2D images are collected.
- Processing: Advanced computational algorithms are applied to these projection images to reconstruct a 3D density map. This map illustrates the electron density distribution, revealing detailed insights into the atomic arrangement of the sample.
- Applications: Cryo-TEM is ideal for investigating the internal structure of biological macromolecules, viruses, and cellular components, providing high-resolution 3D models.
- Cryo-Scanning Electron Microscopy (Cryo-SEM):
- Principle: Cryo-SEM focuses on examining the surface topography of vitrified samples. The specimen is typically fractured or cleaved to expose its internal surfaces before being placed in a scanning electron microscope (SEM).
- Imaging: In SEM, a focused electron beam scans the surface of the sample. The interaction between the electron beam and the specimen generates secondary and backscattered electrons, which are detected to form a surface image.
- Applications: Cryo-SEM is particularly useful for studying surface details and morphology of biological specimens. It allows researchers to observe the external features and surface structures of samples, which is beneficial for understanding surface interactions and topography.
How does cryo EM work? – Protocol of cryo EM
The operation of cryo-electron microscopy (cryo-EM) involves a series of precise steps designed to capture high-resolution images of biological specimens in their native, vitrified state. The workflow for cryo-EM can be detailed as follows:
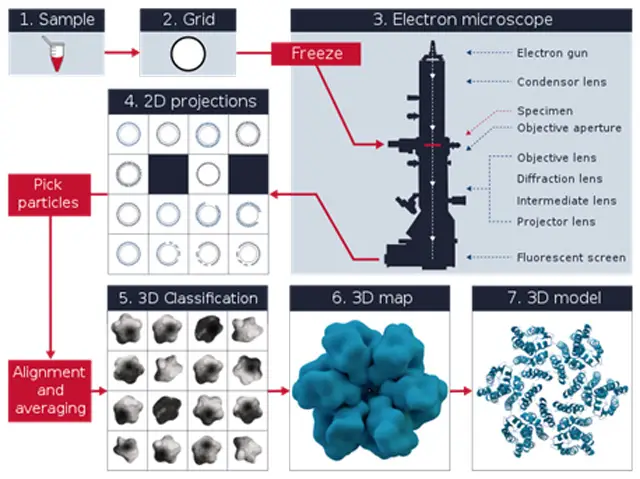
- Sample Preparation:
- Sample Solution: The process begins with a purified biological sample, typically a protein or molecular complex dissolved in solution.
- Vitrification: A small drop of the sample solution is placed on a specialized grid. This grid is then rapidly plunged into a cryogenic fluid, such as liquid ethane, to freeze the sample quickly. This rapid freezing converts the water in the sample into amorphous ice, preserving the native structure of the biological molecules.
- Cryo-EM Microscopy:
- Sample Handling: The vitrified sample is loaded onto the microscope stage and maintained at extremely low temperatures to prevent any damage or alteration.
- Microscope Calibration: The cryo-EM microscope is calibrated and aligned to ensure accurate imaging.
- Data Acquisition: An electron beam is directed at the sample, generating a series of two-dimensional (2D) projection images from various angles. These images capture different aspects of the sample’s structure.
- Image Processing:
- Correction and Enhancement: The collected 2D images are processed to correct for any artifacts and improve the signal-to-noise ratio.
- Particle Identification: Individual particles are identified within the images through a process called particle picking, where specific features of interest are isolated.
- 3D Reconstruction:
- Data Compilation: A large dataset of particle images is compiled and analyzed.
- Computational Reconstruction: Advanced computational algorithms are used to reconstruct a three-dimensional (3D) density map from the 2D images. This map represents the electron density distribution within the sample.
- Model Fitting and Analysis:
- Atomic Modeling: Atomic models are fitted into the 3D density map. These models are refined iteratively to best match the experimental data.
- Validation and Interpretation: The refined models are validated and analyzed to understand the structural features of the molecules under study.
Cryo-EM can be applied to different types of electron microscopy, including:
- Cryo-Transmission Electron Microscopy (Cryo-TEM):
- Imaging Technique: In cryo-TEM, the vitrified sample is examined by transmitting an electron beam through it. Multiple 2D projection images are collected as the sample is tilted at different angles.
- 3D Reconstruction: These images are used to create a 3D density map, providing insights into the internal structure of the sample.
- Cryo-Scanning Electron Microscopy (Cryo-SEM):
- Surface Imaging: Cryo-SEM is used to study the surface structure of vitrified samples. The sample is often fractured to expose its internal surfaces.
- Surface Analysis: A focused electron beam scans the sample’s surface, and secondary and backscattered electrons are detected to form a surface image.
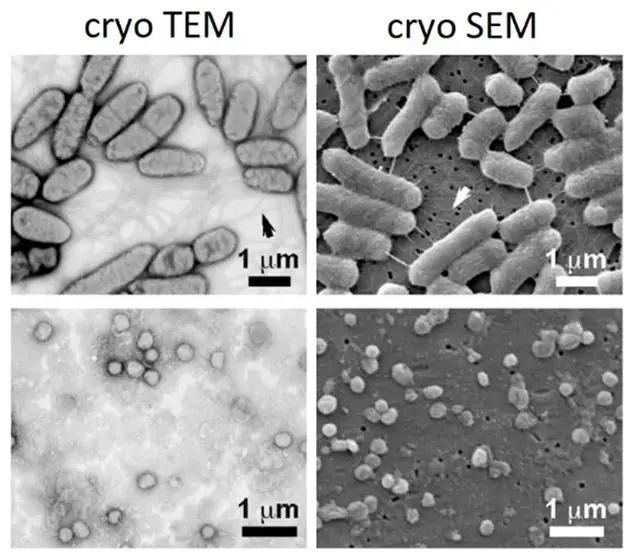
Advantages of Cryo-Electron Microscopy (cryo-EM)
- High, Near-Atomic Resolution Imaging
- Cryo-EM provides exceptional resolution, approaching near-atomic levels. This high-resolution capability allows for detailed visualization of biological macromolecules at an unprecedented level of detail, revealing structural features that are critical for understanding molecular function and interactions.
- Sample Preservation in a Near-Native State
- The technique employs vitrification to rapidly freeze samples, preserving them in a state that closely resembles their natural environment. This preservation is crucial for studying biological specimens as it prevents structural alterations that can occur during sample preparation with other methods.
- Wide Range of Biological Samples
- Cryo-EM is versatile and applicable to a broad spectrum of biological samples. This includes proteins, viruses, cells, and tissues. Its adaptability makes it a valuable tool for various biological and biomedical research applications.
- Study of Large, Flexible Complexes
- Cryo-EM is particularly effective for analyzing large and flexible molecular complexes that are challenging to study using other techniques. Its ability to accommodate large structures and flexible assemblies makes it indispensable for investigating complex biological systems.
- Single Particle Analysis Without Size Limit
- Unlike techniques that require crystallization, cryo-EM enables single particle analysis of samples without size restrictions. This feature allows for the study of macromolecules and assemblies in their native, non-crystalline forms, broadening the scope of structural research.
Limitations of Cryo-Electron Microscopy (cryo-EM)
- Homogeneity Requirement
- Cryo-EM relies on the uniformity of the particle population in the sample to achieve high-resolution results. For effective imaging and accurate structural determination, the sample must exhibit a certain degree of homogeneity. Variability within the sample can compromise the quality of the reconstructed images.
- Computational Demands
- The technique generates extensive datasets during imaging, which are computationally intensive to process. Advanced computational algorithms are required to analyze these large volumes of data, demanding significant computational resources and time.
- Resolution Limitations
- Despite its high-resolution capabilities, cryo-EM may encounter limitations in resolving finer structural details. Certain features of the sample may remain indistinct or poorly defined, especially when dealing with complex or heterogeneous structures.
- Challenges with Larger Samples
- Analyzing larger samples can be problematic. The technique is better suited for smaller or more manageable specimens, as larger structures may present difficulties in maintaining sample integrity and achieving uniform imaging conditions.
- Cost and Expertise
- Cryo-EM equipment is expensive and requires specialized training to operate effectively. The high cost of the technology and the need for expert users can be barriers to accessibility and widespread adoption.
Applications of Cryo-Electron Microscopy (cryo-EM)
- Structural Biology
- Function: Cryo-EM has significantly advanced structural biology by providing high-resolution structures of challenging biological targets.
- Applications: This technique is particularly useful for studying membrane proteins, large macromolecular assemblies, and flexible or heterogeneous complexes that are difficult to resolve with traditional methods.
- Outcome: It aids in elucidating protein structures, functions, mechanisms, and interactions, which are crucial for understanding various biological processes.
- Virology
- Function: Cryo-EM enables the detailed visualization of viral structures, including capsids, envelopes, and surface proteins.
- Applications: This capability is instrumental in vaccine development, antiviral drug design, and exploring viral assembly, replication, and infection mechanisms.
- Outcome: Recent advancements include detailed structural insights into viruses such as the SARS-CoV2 omicron variant, which helps in devising targeted therapeutic strategies.
- Cell Biology
- Function: Cryo-EM provides high-resolution images of cellular architecture and organization.
- Applications: It is used to visualize cellular organelles, cytoskeletal networks, and molecular complexes, facilitating the study of fundamental cellular processes like mitosis, endocytosis, intracellular transport, and organelle biogenesis.
- Outcome: This approach enhances the understanding of cellular mechanisms and the spatial organization of cellular components.
- Drug Discovery
- Function: Cryo-EM plays a critical role in drug discovery by elucidating the structures of drug targets.
- Applications: The technique is applied to study targets such as G protein-coupled receptors (GPCRs) and ion channels, which are crucial for rational drug design and optimization.
- Outcome: It supports the understanding of ligand binding mechanisms and the development of drugs with improved efficacy and specificity.
- Neurobiology
- Function: Cryo-EM is employed to investigate neuronal structures and macromolecular complexes related to neurodegenerative diseases.
- Applications: It helps in studying synaptic vesicles, neurotransmitter receptors, and the molecular mechanisms underlying synaptic transmission and neuronal signaling.
- Outcome: This research contributes to a better understanding of neurological disorders and the development of potential treatments.
- Biochemistry and Molecular Biology
- Function: Cryo-EM aids in studying macromolecular assemblies involved in vital cellular processes.
- Applications: The technique is used to investigate processes such as DNA replication, transcription, translation, and protein folding.
- Outcome: It provides valuable structural insights into the molecular mechanisms of these essential biological processes.
Differences Between cryo TEM and cryo SEM
Cryo-electron microscopy (cryo-EM) encompasses various techniques, with cryo-transmission electron microscopy (cryo-TEM) and cryo-scanning electron microscopy (cryo-SEM) being two primary methods. Each of these techniques has distinct features and applications. The main features of cryo-TEM and cryo-SEM are compared as follows:
Cryo-TEM
- Equipment:
- Transmission Electron Microscope: Utilizes a high-vacuum transmission electron microscope to pass electrons through the sample.
- Electron Beam Interaction:
- Transmission Through the Sample: Electrons are transmitted through the sample, interacting with it to produce images.
- Sample Thickness:
- Thin Samples Required: The sample must be sufficiently thin to allow electrons to pass through, typically in the range of 100 nm or less.
- Information Obtained:
- Internal Structure: Provides detailed insights into the internal structure of the sample, including molecular arrangements and interactions.
- Resolution:
- High Resolution: Achieves near-atomic resolution, allowing for detailed visualization of molecular structures.
- Applications:
- Molecular Structures and Interactions: Primarily used for analyzing the structures of individual macromolecules and complexes, suitable for single-particle analysis.
Cryo-SEM
- Equipment:
- Scanning Electron Microscope: Utilizes a scanning electron microscope to scan the surface of the sample with a focused electron beam.
- Electron Beam Interaction:
- Focused on the Surface: Electrons interact with the surface of the sample, emitting secondary and backscattered electrons to create images.
- Sample Thickness:
- Any Thickness: Can be applied to samples of varying thicknesses, including those that are much thicker than those used in cryo-TEM.
- Information Obtained:
- Surface Topography and Morphology: Focuses on the surface structure and topography of the sample, providing detailed images of surface features.
- Resolution:
- Lower Resolution: Generally provides lower resolution compared to cryo-TEM, though still effective for surface analysis.
- Applications:
- Surface Characterization: Suitable for examining large-scale structures or materials, and for studying surface details of samples.
Comparison
- Technique: Cryo-TEM uses a transmission electron microscope to visualize internal structures, while cryo-SEM uses a scanning electron microscope to image surfaces.
- Sample Preparation: Cryo-TEM requires thin samples, whereas cryo-SEM can handle samples of varying thicknesses.
- Resolution: Cryo-TEM offers high-resolution imaging, ideal for molecular-level details, while cryo-SEM provides surface-level imaging with generally lower resolution.
- Applications: Cryo-TEM is well-suited for molecular structures and interactions, whereas cryo-SEM excels in surface characterization and large-scale structural analysis.
Feature | Cryo-TEM | Cryo-SEM |
---|---|---|
Equipment | Transmission Electron Microscope | Scanning Electron Microscope |
Electron Beam | Transmitted through the sample | Focused on the surface |
Sample Thickness | Thin samples (typically ≤100 nm) | Any thickness |
Information Obtained | Internal structure and molecular arrangements | Surface topography and morphology |
Resolution | High resolution (near-atomic) | Lower resolution |
Applications | Molecular structures, single-particle analysis | Surface characterization, large-scale structures |
Comparison of the Main Features of Conventional EM and Cryo-EM
- Sample Preparation
- Conventional EM:
- Method: Involves multiple steps including fixation, staining, dehydration, and the use of chemical compounds or polymers.
- Impact: These procedures can alter native structures and introduce artifacts, potentially affecting the accuracy of structural interpretations.
- Cryo-EM:
- Method: Employs flash-freezing techniques, known as vitrification, to rapidly freeze the sample in its native state.
- Impact: This approach preserves native structures with minimal distortion, reducing the introduction of artifacts.
- Conventional EM:
- Sample Integrity
- Conventional EM:
- Condition: The native structures of samples can be altered due to the fixation and staining processes.
- Result: The preservation of sample integrity is compromised, which may affect the accuracy of the structural data obtained.
- Cryo-EM:
- Condition: Samples are well-preserved as they are rapidly frozen, maintaining their native state.
- Result: This method ensures that the sample’s native structures are retained, providing more accurate structural information.
- Conventional EM:
- Resolution
- Conventional EM:
- Resolution: Achieves high resolution in the nanometer range (nm).
- Detail: This level of resolution is suitable for observing fine cellular and subcellular structures but may not capture atomic-level details.
- Cryo-EM:
- Resolution: Provides higher resolution, reaching near-atomic levels (Å).
- Detail: This enhanced resolution allows for detailed visualization of biomolecules and complex assemblies at the atomic level.
- Conventional EM:
- Specimen Types
- Conventional EM:
- Suitability: Ideal for examining cellular and subcellular structures.
- Scope: This method is effective for imaging larger biological structures but may be less suitable for complex biomolecular assemblies.
- Cryo-EM:
- Suitability: Best suited for studying biomolecules and complex macromolecular assemblies.
- Scope: Cryo-EM is particularly valuable for analyzing smaller or more delicate biological samples that require preservation of their native state.
- Conventional EM:
- Amount of Sample
- Conventional EM:
- Requirement: Requires larger amounts of sample material.
- Implication: This can be a limiting factor when working with limited or precious samples.
- Cryo-EM:
- Requirement: Minimal amounts of biomolecules or complexes can be analyzed.
- Implication: This allows for the examination of scarce or precious samples with high precision.
- Conventional EM:
Feature | Conventional EM | Cryo-EM |
---|---|---|
Sample Preparation | Multiple steps: fixation, staining, dehydration; potential artifacts | Flash-freezing (vitrification) to preserve native state |
Impact on Sample | Can alter native structures, introducing artifacts | Preserves native structures with minimal distortion |
Sample Integrity | Compromised due to fixation and staining | Well-preserved, maintaining native state |
Resolution | High resolution in the nanometer range (nm) | Higher resolution, near-atomic levels (Å) |
Detail | Suitable for fine cellular and subcellular structures | Detailed visualization of biomolecules and complex assemblies |
Specimen Types | Ideal for cellular and subcellular structures | Best for biomolecules and complex macromolecular assemblies |
Amount of Sample | Requires larger amounts of material | Can analyze minimal amounts of biomolecules or complexes |
Images of Cryo-Electron Microscopy (cryo-EM)
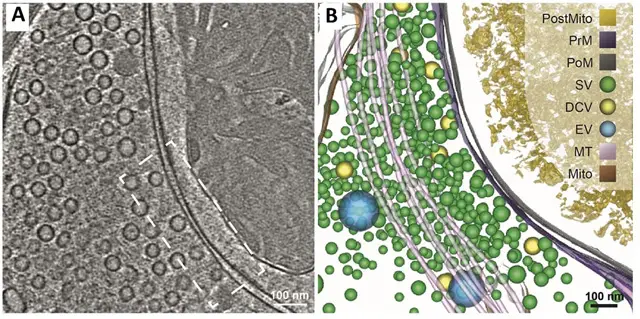
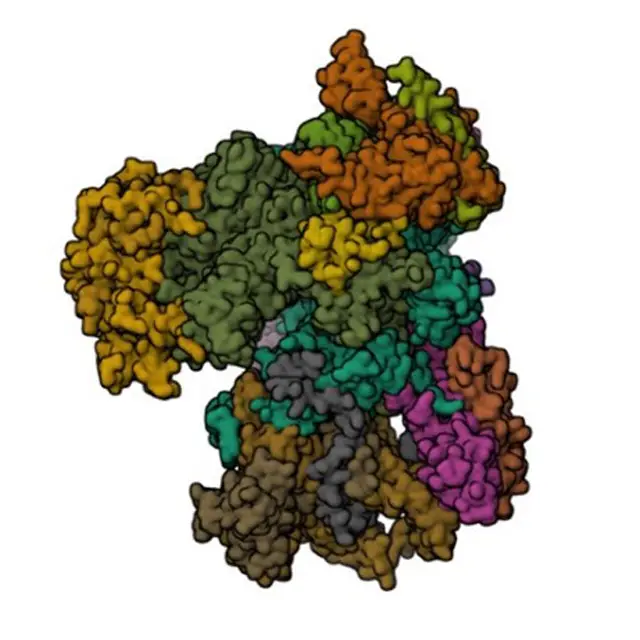
- Milne JL, Borgnia MJ, Bartesaghi A, Tran EE, Earl LA, Schauder DM, Lengyel J, Pierson J, Patwardhan A, Subramaniam S. Cryo-electron microscopy–a primer for the non-microscopist. FEBS J. 2013 Jan;280(1):28-45. doi: 10.1111/febs.12078. Epub 2012 Dec 17. PMID: 23181775; PMCID: PMC3537914.
- https://www.technologynetworks.com/analysis/articles/cryo-electron-microscopy-principle-strengths-limitations-and-applications-377080
- https://en.wikipedia.org/wiki/Cryogenic_electron_microscopy
- https://www.chemistryworld.com/news/explainer-what-is-cryo-electron-microscopy/3008091.article
- https://www.lsi.umich.edu/science/centers-technologies/cryo-electron-microscopy
- https://www.thermofisher.com/in/en/home/electron-microscopy/life-sciences/cryo-em.html