What is SDS-PAGE?
- SDS-PAGE (sodium dodecyl sulfate-polyacrylamide gel electrophoresis) is a powerful and frequently used technique for sorting proteins by molecular weight. Ulrich K. Laemmli created it, and it has become an indispensable instrument in forensics, genetics, biotechnology, and molecular biology.
- The idea of SDS-PAGE is to remove the influence of protein structure and charge, focusing simply on protein molecule size. The process employs two basic ingredients: sodium dodecyl sulfate (SDS) and polyacrylamide gel.
- SDS is an anionic detergent that denatures proteins by attaching to them and destroying their natural structure. Proteins are uniformly coated with negative charges proportionate to their molecular weight after being treated with SDS. This coating neutralizes the proteins’ intrinsic charge, allowing them to be sorted simply by size during electrophoresis.
- Polyacrylamide gel, on the other hand, serves as a medium for proteins to move across when subjected to an electric field. Polymerization of acrylamide and bisacrylamide monomers results in the gel matrix. The concentration of these monomers can be changed to produce gels with varying porosities, allowing protein separation within a specified molecular weight range.
- The SDS-PAGE procedure consists of multiple phases. First, the protein samples are treated with SDS and a reducing agent, such as -mercaptoethanol or dithiothreitol (DTT), which breaks disulfide bonds and denatures the proteins further. The protein mixture is then put into polyacrylamide gel wells.
- When an electric current is introduced to the gel matrix, the negatively charged SDS-coated proteins move towards the positive electrode. Smaller proteins migrate quicker and move farther across the gel than larger proteins because the migration rate is inversely proportional to the logarithm of the molecular weight.
- The divided proteins can be observed after electrophoresis by staining the gel with dyes such as Coomassie Brilliant Blue or silver stain. The protein bands that result can be measured and analyzed to identify the molecular weight and relative abundance of different proteins in a sample.
- SDS-PAGE has many applications in research and other disciplines. It’s frequently used to evaluate protein samples, purify specific proteins, determine protein purity, and investigate protein-protein interactions. Scientists can acquire significant insights into the composition and properties of complicated protein mixtures by isolating proteins depending on their molecular weight.
- To summarize, SDS-PAGE is a powerful technology that has transformed the field of protein analysis. This approach, which combines sodium dodecyl sulfate with polyacrylamide gel, allows for the separation of proteins simply based on their size, giving researchers a valuable tool for studying protein structure, function, and relationships.
Definition of SDS-PAGE
SDS-PAGE is a technique used to separate proteins based on their molecular weight by using a gel matrix and the denaturing agent sodium dodecyl sulfate (SDS).
Overview of SDS PAGE
SDS-PAGE (sodium dodecyl sulfate-polyacrylamide gel electrophoresis) is a popular method for studying protein mixtures. By sorting proteins depending on their size, it is possible to determine protein purity and estimate protein molecular weight.
To begin the SDS-PAGE process, denature the proteins in the mixture to remove any secondary or quaternary structures. The anionic detergent sodium dodecyl sulfate (SDS) is used to achieve this denaturation. SDS attaches to the proteins’ polypeptide backbones, causing them to unfold and become negatively charged. Because the amount of SDS bound is proportional to the length of the polypeptide chain, proteins with a significant negative charge result.
The preferred media for SDS-PAGE is polyacrylamide gel. This artificial gel is clear, chemically inert, and thermally stable. It can be made with varying hole diameters to accommodate proteins of various sizes. The gel is made up of acrylamide monomers that combine to form a polymer matrix. The proteins are pushed across the gel using electrophoresis, which is known as polyacrylamide gel electrophoresis (PAGE).
SDS-PAGE gel is made up of two layers: the stacking or spacer gel and the separating or resolving gel.
The stacking gel comprises big pores manufactured of polyacrylamide at a concentration of around 5%. It is generated on top of the separating gel and is made with a Tris buffer with a pH of about 6.8, which is higher than the pH of the electrophoresis buffer. The stacking gel’s objective is to concentrate the proteins into a tight zone before they enter the separating gel.
The separating gel, on the other hand, has smaller holes made of polyacrylamide in concentrations ranging from 5% to 30%. It’s made with a Tris buffer with a pH of 8.8. The proteins are segregated in this gel based on their molecular size. Smaller proteins can move more easily across the gel matrix and so migrate faster than larger proteins.
The negatively charged proteins migrate through the gel when an electric current is applied, with smaller proteins going farther than bigger ones. After the electrophoresis, the proteins can be seen by staining the gel with dyes like Coomassie Brilliant Blue or with other detection methods.
To summarize, SDS-PAGE is a strong technique for analyzing protein mixtures that uses denaturation with SDS and separation with polyacrylamide gel. Based on size separation, it is possible to determine protein purity and estimate molecular weight. Stacking and separating gels of varying hole sizes are used to promote protein concentration and separation, respectively.
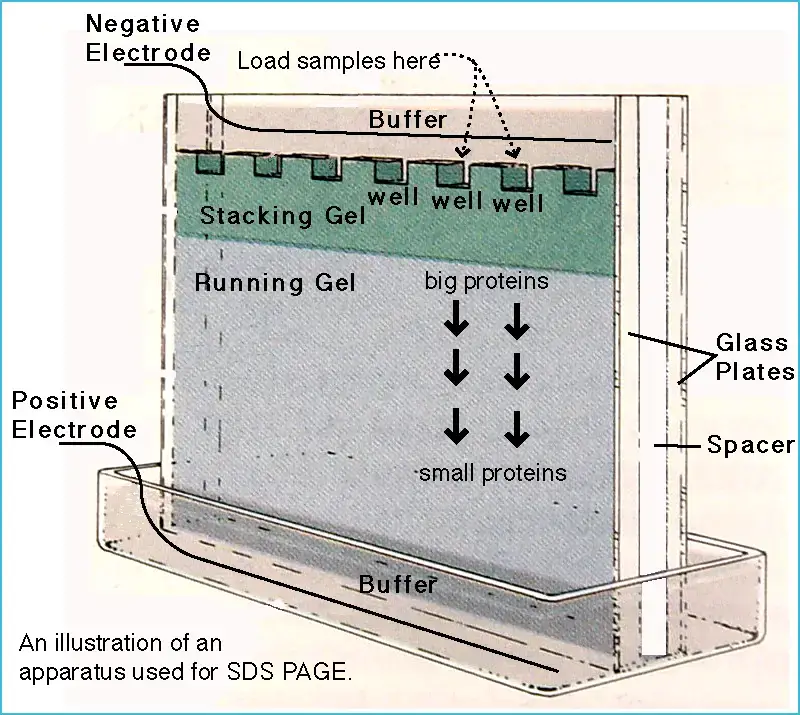
Properties of SDS-PAGE
SDS-PAGE (sodium dodecyl sulfate-polyacrylamide gel electrophoresis) has numerous different features that make it an excellent protein separation method:
- Polyacrylamide-Based Gel: Polyacrylamide gel is used as the medium in SDS-PAGE. Typically, this gel is manufactured as a slab gel sandwiched between two glass plates. The polyacrylamide gel provides a robust and inert matrix for protein separation based on mass.
- SDS (Sodium Dodecyl Sulfate): SDS is an essential component of SDS-PAGE. It functions as a surfactant and binds to proteins at a rate of around 1.4 grams of SDS per gram of protein. SDS conceals the proteins’ intrinsic charge and imparts a consistent charge-to-mass ratio, allowing for exact separation based on mass. Because of the presence of SDS, the proteins are mainly negatively charged, which overrides their intrinsic charge characteristics.
- Micelle Formation: In aqueous solutions, SDS forms spherical micelles above a particular concentration known as the critical micellar concentration (CMC). SDS has a CMC ranging from 7 to 10 millimolar. Because of their anionic outer surface, the micelles do not adsorb proteins and are composed of around 62 SDS molecules. Hydrophobic interactions are used by SDS monomers to interact with proteins.
- Protein Denaturation: SDS causes protein denaturation. Proteins unfold at concentrations greater than 0.1 millimolar, while most proteins denature at concentrations greater than 1 mM. Because secondary, tertiary, and quaternary structures are damaged by this denaturing process, proteins can be separated based on their primary structure.
- Protein Migration: Proteins migrate across the polyacrylamide gel towards the anode under the influence of an electric field. Based on its mass, each protein migrates at a particular rate. Smaller proteins move faster and farther through the gel, whereas larger proteins move more slowly and stay closer to the starting site.
- Quaternary Structure Determination: SDS-PAGE is typically used to determine the mass of individual protein subunits or monomers because it breaks the quaternary structure of proteins. Certain SDS-resistant protein complexes, on the other hand, can tolerate the denaturing effect of SDS, allowing their investigation. Due to the large activation energy required, heat treatment is frequently required to denature such compounds.
- Alternative Surfactants: While SDS is the most widely used surfactant in SDS-PAGE, other surfactants such as CTAB (cetyltrimethylammonium bromide) or 16-BAC (16-benzyldimethylhexadecylammonium chloride) can be employed in specialized applications such as CTAB-PAGE or BAC-PAGE.
To summarize, SDS-PAGE uses polyacrylamide gel, SDS surfactant, and the denaturing effect of SDS to separate proteins based on their mass. This technology has evolved into a critical tool in protein analysis and is widely employed in a variety of scientific domains.
Objective
SDS-PAGE (sodium dodecyl sulfate-polyacrylamide gel electrophoresis) goals can be summarized as follows:
- SDS-PAGE preparation: Understanding and learning how to prepare the gel is one of the key goals of SDS-PAGE. This includes properly preparing the stacking and separating gels, correctly preparing the polyacrylamide gel, and properly preparing the electrophoresis buffer with the appropriate pH and ionic strength.
- Protein Purity Determination: SDS-PAGE is a popular method for determining protein purity. SDS-PAGE analysis of a protein sample can indicate the presence of impurities or contaminants in the protein preparation. A pure protein will appear on the gel as a single band, while contaminants or other proteins will appear as numerous bands.
- Molecular Mass Determination: Determining the molecular mass of a pure protein is another important goal of SDS-PAGE. It is feasible to assess the approximate size of a protein by comparing its migration with known molecular weight markers. Protein migration in the gel is inversely related to molecular weight, which means that smaller proteins travel quicker and further than bigger ones.
- Quality Control: SDS-PAGE serves as an important quality control step in protein purification operations. It aids in determining the success of protein purification procedures and ensuring that contamination or degradation is not present. Researchers can have trust in the integrity and reliability of their experimental results by checking the purity and molecular weight of the protein of interest.
- Comparative Analysis: SDS-PAGE enables for the comparison of protein samples under different experimental circumstances. Researchers can examine changes in protein expression, post-translational modifications, and protein-protein interactions by running different samples on the gel at the same time. This comparative approach contributes to a better understanding of the functional and structural changes that proteins go through in response to various circumstances.
In summary, the goals of SDS-PAGE are to prepare the gel, determine protein purity, and estimate protein molecular mass. SDS-PAGE also functions as a quality control tool and allows for comparative study of protein samples. By fulfilling these goals, researchers will acquire significant insights into the properties and qualities of proteins, which will benefit fields such as molecular biology, biochemistry, and biotechnology.
Principle of SDS-PAGE
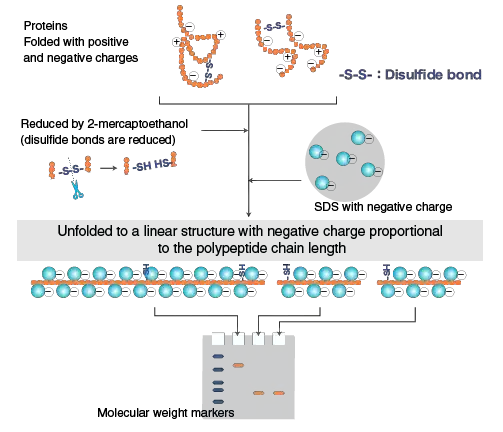
The principle of SDS-PAGE (sodium dodecyl sulfate-polyacrylamide gel electrophoresis) is based on the migration of charged molecules in an electric field and the separation of proteins based on their length.
- Charged Molecule Migration: According to the principle, charged molecules will migrate towards the electrode with the opposite charge when placed in an electric field. This migration occurs due to the attraction or repulsion between the charged molecule and the electrode. In the case of proteins, the migration depends on the relative mobility of the charged species.
- Influence of Size: Smaller molecules experience less resistance during electrophoresis and therefore migrate faster through the gel matrix. In SDS-PAGE, this principle applies to the separation of proteins based on their size. Smaller proteins will migrate more quickly through the gel compared to larger proteins.
- Influence of Structure and Charge: The structure and charge of proteins also play a role in their migration rate. However, in SDS-PAGE, the use of sodium dodecyl sulfate (SDS) and polyacrylamide gel largely eliminates the influence of protein structure and charge. SDS is a detergent that binds to the protein backbone at a constant molar ratio. It denatures the proteins and imparts a negative charge to the polypeptide chain, which is proportional to its length.
- Polyacrylamide Gel Matrix: The polyacrylamide gel serves as a mesh-like matrix for the separation of proteins based on their length. Polymerized acrylamide forms a stable gel that can withstand the electrophoretic process. The strength of the gel allows for easy handling and manipulation during the experiment. Polyacrylamide gel electrophoresis provides an effective medium for separating proteins of typical sizes.
By combining the denaturing effect of SDS and the use of polyacrylamide gel, SDS-PAGE enables the separation of proteins solely based on their polypeptide chain length. This principle allows researchers to analyze protein samples, determine their molecular weight, and assess their purity. SDS-PAGE is a widely used technique in various scientific disciplines, including molecular biology, biochemistry, and biotechnology, for the qualitative and quantitative analysis of proteins.
What is the Role of SDS in SDS-PAGE?
The role of SDS (sodium dodecyl sulfate) in SDS-PAGE (sodium dodecyl sulfate-polyacrylamide gel electrophoresis) is crucial for the denaturation and uniform charge distribution of proteins. SDS is a detergent that plays multiple roles in the process:
- Denaturation of Proteins: SDS has a strong denaturing effect on proteins. It disrupts the native conformation of proteins by breaking the non-covalent bonds, particularly the disulfide bonds that contribute to the protein’s tertiary structure. This denaturation process unfolds the proteins into linear chains.
- Binding to Proteins: SDS binds to the protein backbone in a ratio of approximately 1.4 grams of SDS per gram of protein. It interacts with hydrophobic regions of the protein, thereby coating the polypeptide chain. This binding ensures that the proteins are fully denatured and uniformly coated with SDS molecules.
- Imparting Negative Charge: SDS is an anionic detergent, meaning it carries a negative charge. As it binds to the proteins, it imparts a negative charge to the polypeptide chain in proportion to its length. The negatively charged SDS molecules effectively mask the intrinsic charge of the proteins, making the migration of proteins in the gel solely dependent on their size.
- Formation of SDS-Protein Complexes: The binding of SDS to proteins results in the formation of SDS-protein complexes. These complexes have a uniform charge-to-mass ratio, allowing proteins of different sizes to be separated based on their molecular weight during electrophoresis.
- Formation of SDS Micelles: In aqueous solutions above a certain concentration called the critical micellar concentration (CMC), SDS molecules form spherical micelles. These micelles aid in the solubilization of hydrophobic regions of proteins and ensure their uniform dispersion in the gel.
By combining the denaturing and charge-imparting properties of SDS, SDS-PAGE eliminates the influence of protein structure and charge, enabling the separation of proteins based solely on their polypeptide chain length. This technique has become a cornerstone of protein analysis, allowing researchers to assess protein purity and determine molecular weight accurately.
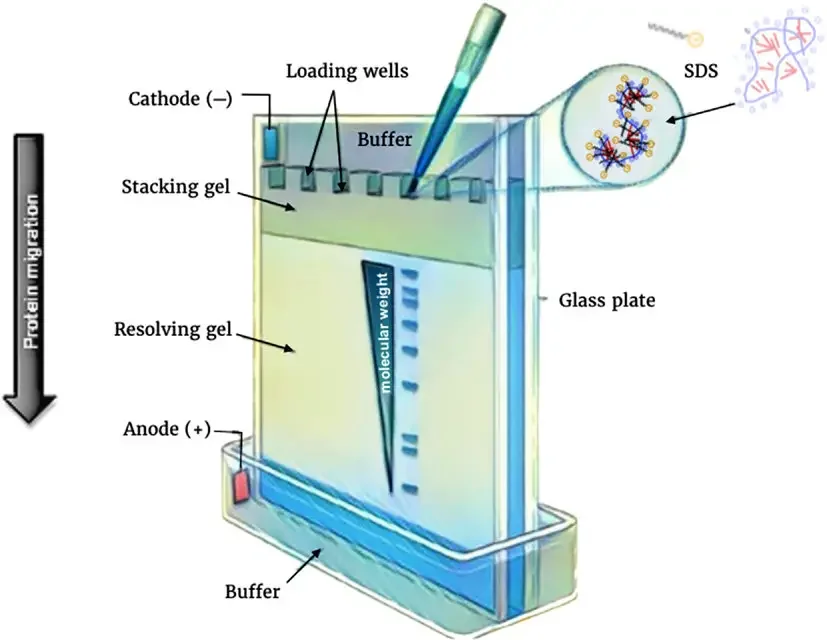
Requirement for SDS-PAGE and their roles
In SDS-PAGE (sodium dodecyl sulfate-polyacrylamide gel electrophoresis), several materials are used to carry out the procedure effectively. These materials and their roles in SDS-PAGE are as follows:
- Power Supplies: Power supplies are used to convert the alternating current (AC) to direct current (DC). They provide the necessary electrical energy to drive the electrophoresis process.
- Gels: The gel matrix used in SDS-PAGE can be prepared in the laboratory or purchased as precast gels. Polyacrylamide is the main component of the gel matrix, and it forms a mesh-like structure that allows for the separation of proteins based on their molecular weight.
- Electrophoresis Chambers: Electrophoresis chambers are designed to hold the SDS-PAGE gels securely during the electrophoresis process. The chambers provide a stable environment for the separation of proteins.
- Protein Samples: Protein samples to be analyzed are prepared by diluting them with SDS-PAGE sample buffer. The sample buffer contains sodium dodecyl sulfate (SDS), which denatures the proteins and imparts a negative charge to them. Additionally, a reducing agent such as dithiothreitol or 2-mercaptoethanol is added to break the disulfide bonds and prevent tertiary protein folding.
- Running Buffer: The protein samples loaded onto the gel are run in SDS-PAGE running buffer. The running buffer provides the appropriate pH and ionic strength for the separation of proteins during electrophoresis.
- Staining and Destaining Buffer: After the electrophoresis is complete, the gel is typically stained with a dye, such as Coomassie Stain Solution, to visualize the protein bands. The stained gel is then destained using a destaining solution to remove excess dye and enhance the contrast of the protein bands.
- Protein Ladder: A protein ladder, also known as a molecular weight marker, is a mixture of proteins with known molecular weights. It is loaded onto a separate lane of the gel and serves as a reference to determine the molecular weight of the proteins of interest.
- Tris: Tris is used as a buffer in SDS-PAGE because it maintains a stable pH within the desired range (typically pH 7.0 to 9.0). It helps provide the appropriate environment for protein separation.
- Acrylamide: Acrylamide is a key component in the preparation of the polyacrylamide gel. It undergoes polymerization to form a cross-linked network that creates the gel matrix.
- Bisacrylamide (N,N’-Methylenebisacrylamide): Bisacrylamide is a cross-linking agent used in the polymerization of acrylamide gels. It forms covalent bonds between the acrylamide molecules, contributing to the stability and structure of the gel.
- Sodium Dodecyl Sulfate (SDS): SDS is an anionic detergent that denatures proteins by binding to them and disrupting their native structure. It also imparts a negative charge to the proteins in proportion to their polypeptide chain length, allowing for size-based separation during electrophoresis.
- Ammonium Persulfate (APS): APS is a chemical catalyst used to initiate the polymerization of acrylamide and bisacrylamide, leading to the formation of the gel matrix.
- N,N,N’,N’-Tetramethylethylenediamine (TEMED): TEMED is often used in conjunction with ammonium persulfate as a catalyst for the polymerization of acrylamide gels. It helps initiate the reaction and promotes gel formation.
These materials collectively play essential roles in SDS-PAGE, enabling the denaturation, separation, and visualization of proteins based on their molecular weight.
Chemical Requirement for SDS PAGE
- Acrylamide/Bisacrylamide Solution 30% (29:1)
- 2.5X Tris-SDS Buffer (pH 8.8) 65 ml 125 ml 2-8o
- 5X Tris-SDS Buffer (pH 6.8)
- Prestained Protein Ladder
- 5X Tris-Glycine-SDS Gel Running Buffer
- 5X Sample Loading Buffer
- Protein Sample 1
- Protein Sample 2
- Staining solution
- Destaining solution
- Ammonium persulphate (APS)
- Tetramethylethylenediamine (TEMED)
- Agarose
Other Material Requireds;
- Glass wares: Conical flask, Measuring cylinder, Beaker
- Reagents: Distilled water
- Other requirements: Protein Electrophoresis apparatus, Micropipettes, Tips, Microwave/Burner/Hotplate
SDS or sodium dodecyl sulphate
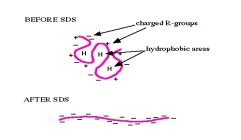
- SDS, or sodium dodecyl sulfate, is an anionic detergent that is commonly employed in biological and biochemical methods such as SDS-PAGE (polyacrylamide gel electrophoresis). Its role in SDS-PAGE is critical for protein denaturation and separation depending on molecular weight.
- SDS binds firmly to proteins via hydrophobic interactions when given to a protein sample. SDS binding to proteins disturbs their original structure and causes them to unfold into linear chains. SDS binds to proteins in a steady ratio of around 1.4 grams of SDS per gram of protein. Because the negative charge of SDS dominates any intrinsic charge of the protein, this interaction assures that the proteins acquire a homogeneous negative charge proportional to their mass.
- The SDS-protein complexes move through the polyacrylamide gel under the influence of an electric field during SDS-PAGE. The gel functions as a molecular filter, allowing proteins to be separated based on their sizes or molecular weights. Smaller proteins can travel more readily and quickly through the gel, whereas larger proteins encounter greater resistance and migrate more slowly. As a result, the proteins are divided into molecular units based on their weights.
- Standard proteins with known molecular weights are run on the same gel as reference markers to determine the molecular weight of an unknown protein. The relative mobility (Rf) of these markers is measured, which represents the distance traveled by the protein band relative to the distance traversed by a tracking dye such as bromophenol blue. An atypical curve is created by graphing the Rf values of known protein markers against their respective molecular weights on a semi-log graph. Based on the Rf value, this curve can be used to extrapolate the molecular weight of an unknown protein.
- In summary, SDS is essential in SDS-PAGE because it denaturates proteins, imparts a consistent negative charge, and allows for size-based protein separation in the gel. It is a crucial component in this extensively used approach for determining protein molecular weight.

How does SDS PAGE work?
- SDS-PAGE, or sodium dodecyl sulfate-polyacrylamide gel electrophoresis, is a popular method for sorting proteins by molecular weight. The procedure includes numerous important phases and mechanisms that allow proteins to be separated in a gel matrix.
- The SDS-PAGE technique begins with the preparation of the gel, which is divided into two parts: stacking gel and running gel. The pores in the stacking gel are bigger than those in the running gel. A voltage is delivered across the gel as it is placed in an electrophoresis chamber.
- The sample buffer containing the protein combination is injected into wells at one end of the gel when the voltage is applied. SDS is present in the sample buffer, which denatures the proteins and gives them a negative charge. It also contains glycine, which is positively charged in the stacking gel but negatively charged as it migrates into the flowing gel.
- As the voltage is applied, chloride ions in the sample buffer and stacking gel migrate rapidly towards the positive pole, forming an ion front. The differential in mobility of glycine and chloride ions causes a large voltage gradient inside the gel, causing protein-SDS complexes to move to the negative pole.
- The wide pores in the stacking gel provide little resistance, allowing the protein-SDS complexes to stack up and concentrate near the junction of the stacking and running gels. This stacking action results in a dense band of protein-SDS complexes.
- As the glycine ions move into the flowing gel, the pH of the gel changes, bringing it closer to the pKa of the glycine amino groups. As a result, a considerable part of glycine molecules become negatively charged. These negatively charged glycine molecules begin to migrate at the same pace as the chloride ions, closing the voltage gap that previously regulated protein mobility across the gel.
- The running gel, with its narrower pores, provides resistance to protein mobility. Because of the gel’s sieving capabilities, proteins migrate at varying rates depending on their size. Smaller protein-SDS complexes can move more easily and quickly across the gel matrix, whereas bigger complexes encounter greater resistance and migrate more slowly.
- Protein migration in the running gel is proportional to the logarithm of their molecular weight within a specified range given by the gel’s porosity. As a result, proteins of various sizes separate into distinct bands that run the length of the gel, forming a molecular weight ladder.
- The molecular weight of unknown proteins can be calculated by comparing the migration positions of known protein standards of various molecular weights with the migration of unknown proteins.
- In summary, SDS-PAGE separates proteins depending on their molecular weight using an electric field. The use of SDS and varied pore sizes in the stacking and running gels allows for the concentration and separation of protein-SDS complexes, resulting in discrete bands that can be used to calculate molecular weight.
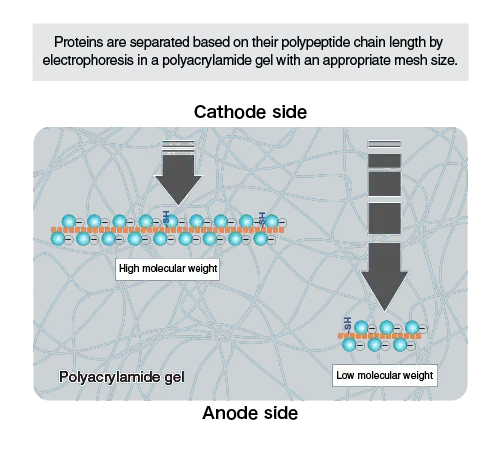
Protocol of SDS-PAGE
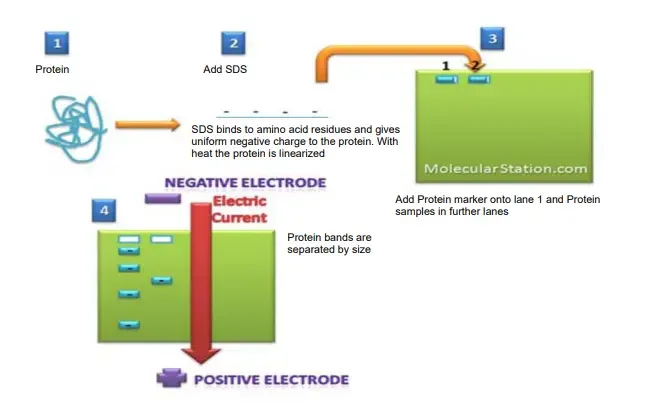
- Make sure that glass panels are secured to the unit, along with the spacers positioned on two edges.
- Make 1 % agarose (0.05g within 5ml distillated water). Boil until the agarose dissolves and then pour an even layer of horizontal agarose at the bottom of the plates to secure the plate. Allow it to solidify it to cool for 5-10 mins.
Preparation of 12% Separating Gel
For the preparation of separating gel include the ingredients as follows:
Ingredients | Amount |
30% Acrylamide-bisacrylamide Solution | 6 ml |
Distilled water | 3 ml |
2.5X Tris-SDS Buffer (pH 6.8) | 6 ml |
10% APS Solution | 125 ul |
TEMED | 18 ul |
Place the gel between the plates and let it set for about one hour. After the gel has cooled, immediately pour it onto the plates.
Poured, add distilled water until it is level the gel. After about an hour, remove all the liquid by flipping the casting.
Preparation of 5% Stacking Gel
To prepare the stacking gel mix the ingredients in the following order:
Ingredients | Amount |
30% Acrylamide-bisacrylamide Solution | 1.3 ml |
Distilled water | 5.1 ml |
5X Tris-SDS Buffer (pH 6.8) | 1.6 ml |
10% APS Solution | 75 ul |
TEMED | 10 ul |
After the addition of TEMED thoroughly mix all ingredients by swirling the beaker. Place the stacking gel on the top of the separating gel. Then, immediately, place the comb, making sure to avoid air bubbles. It should be allowed to set for about 30 minutes.
Important: Acrylamide is a potential neurotoxin, and must be handled with extreme attention. Always wear a face-mask and wear gloves.
- Place 1X Tris-Glycine-SDS Gel Running Buffer into the unit in a way that it connects the two electrodes and consequently completes that flow. Take the comb out of the stacking Gel cautiously.
- Sample Preparation: Take 2 tubes for protein samples. Label them accordingly. You should take 20 ml of each sample from the tube and add 5ml of the 5X sample loading buffer it. The tubes that contain Protein Samples at 100oC in the bath of boiling water. Don’t boil the tube that has PrestainedProtein Ladder.
- Take 5ml of the Prestained Protein Ladder and 20ml of the samples right after the heat treatment of the wells made by the comb within the Stacking Gel.
- The power cable should be connected to an electrophoretic power source according to the standard protocols: Red-Anode as well as Black-Cathode. The electrophoresis process is 100 V at 10 mA until the dye front is 0.5 cm higher than that of the gel sealing.
- Take the gel out between the plates by putting it into the plastic tray that contains distillate water. The gel should be cleaned for 1 minute. Then, rinse the gel with water and go to destain the stain procedure.
Staining and Destaining of Gel
- After removing the gel from the electrophoresis unit, remove any excess water from the gel’s surface.
- Place the gel in a tray or container and add 50ml of Staining Solution to cover the gel completely. Allow the gel to soak in the staining solution until the protein bands become apparent. Sometimes, it may be necessary to incubate the gel in the staining solution overnight to ensure optimal band visualization.
- Once the desired staining intensity is achieved, carefully remove the gel from the staining solution. The staining solution can be reused for up to three times, as long as it remains effective.
- Rinse the stained gel with distilled water to remove the excess stain. Gently shake the gel in a tray filled with distilled water, and then replace the water with fresh distilled water. Repeat this rinsing process three to four times, changing the water each time, to ensure thorough removal of the stain.
- Prepare 50ml of Destaining Solution and add it to the tray containing the gel. The destaining solution is typically a solvent that helps remove the background stain from the gel. Shake the tray containing the gel at a constant rate to facilitate the destaining process.
- Continue destaining until the protein bands on the gel become clear and distinct, while the background stain is removed. This may take some time and may require periodic evaluation of the gel’s appearance.
- Once the desired destaining is achieved, carefully remove the gel from the destaining solution. Similar to the staining solution, the destaining solution can also be reused for up to three times, provided it still effectively removes the background stain.
After completing the staining and destaining steps, the gel can be visually examined to observe the protein bands. Alternatively, the gel can be further processed for documentation, such as by scanning or photographing, to preserve and analyze the results.
Observation and Result of SDS-PAGE
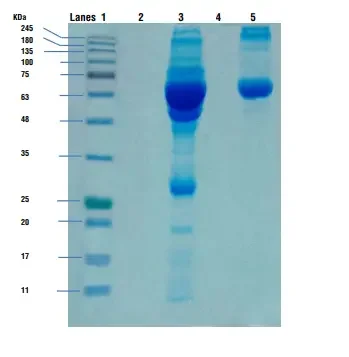
- Lane 1: Prestained Protein Ladder
- Lane 3: Protein Sample 1
- Lane 5: Protein Sample 2
Interpretation
Following staining, and then destaining, gel, you can compare the molecular mass of the samples to the weight of the protein marker. Protein Sample 1 is a serum sample so multiple bands are observed. Protein Sample 2 is a purified protein and therefore 1 major band is visible.
Sample Preparation for SDS-PAGE
- Take 1ml of culture solution of E.coli strain DH5α, in 1.5 ml of eppendorf’s tube.
- Then centrifuge at 12000 rpm for 5 min.
- Then discard the supernatant.
- Then add 500 μl of TE in the tube and dissolve the tube by gentle shaking.
- Then add same volume of sample buffer.
- Finally heat the sample on boiling bath for 5 minutes and then immediately keep on
ice.
The sample to analyze is optionally mixed with a chemical denaturant if so desired, usually SDS for
proteins or urea for nucleic acids. SDS is an anionic detergent that denatures secondary and non–
disulphide linked tertiary structures, and additionally applies a negative charge to each protein in
proportion to its mass. Urea breaks the hydrogen bonds between the base pairs of the nucleic acid,
causing the constituent strands to separate. Heating the samples to at least 60 °C further promotes denaturation.
Protocol of SDS-PAGE
The SDS-PAGE method is composed of gel preparation, sample preparation, electrophoresis, protein staining or western blotting and analysis of the generated banding pattern.
1. Gel Preparation
Gel preparation is a crucial step in gel electrophoresis for separating proteins based on their size. Here are the steps involved in gel preparation:
- Gathering Materials: Gather all the necessary materials, including combs, glass plates, spacer (silicone tubing), and binder clips. Ensure that the glass plates are thoroughly cleaned with ethanol before use.
- Assembling the Gel Casting Mold: Assemble the gel casting mold using the cleaned glass plates. The mold consists of two glass plates held together with binder clips, leaving a space between them to pour the gel.
- Preparing the Acrylamide Solution: Combine all the reagents required for gel preparation, except for TEMED (Tetramethylethylenediamine). This solution usually includes acrylamide, bisacrylamide, buffer, and water.
- Adding TEMED: When the gel is ready to be poured, add TEMED to the acrylamide solution. TEMED acts as a catalyst for polymerization.
- Pouring the Separating Gel: Pour the acrylamide solution into the space between the glass plates, forming the separating gel. To prevent contact with air (oxygen), which inhibits polymerization, overlay the solution with water. Allow the acrylamide to polymerize for 20-30 minutes to form a gel.
- Removing Air Bubbles: Before the gel polymerizes, add butanol to the top of the gel. The addition of butanol helps to remove any unwanted air bubbles that may be present in the gel.
- Inserting the Comb: Insert a comb into the spaces between the glass plates. The comb is used to create wells or lanes in the gel, where the protein samples will be loaded. Choose an appropriate comb with the desired number of lanes based on the number of samples and molecular weight markers to be used.
- Gel Polymerization: Allow the acrylamide to polymerize completely, forming a solid gel. Once polymerized, the gel becomes a “gel cassette” held between the glass plates.
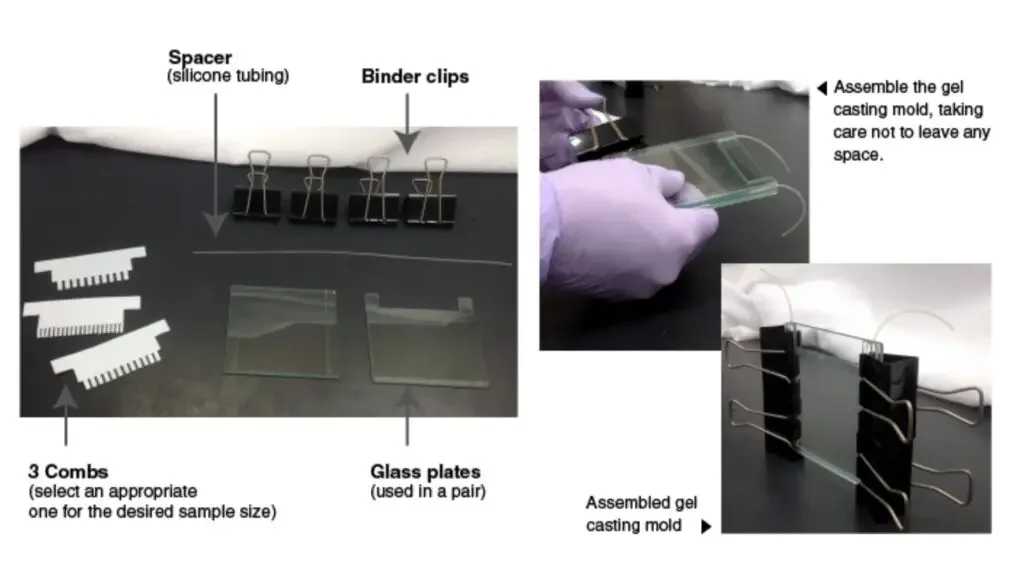
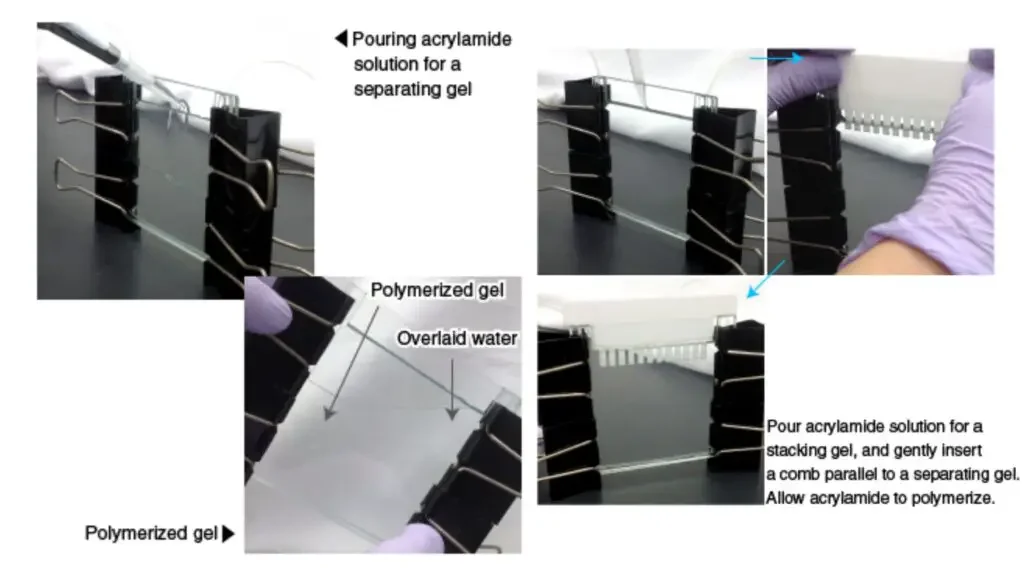
Gel preparation is tailored to the specific requirements of the experiment. The concentration of acrylamide determines the mesh size of the gel, affecting the separation of proteins. Higher acrylamide concentrations (6-15%) are suitable for separating small proteins, while gradient gels with varying acrylamide concentrations may be used for specialized applications.
In addition, a stacking gel can be used before the separating gel to concentrate proteins before they enter the separation process. The stacking gel takes advantage of the differential migration rates of glycine ions, chloride ions, and proteins to create a concentrated protein zone.
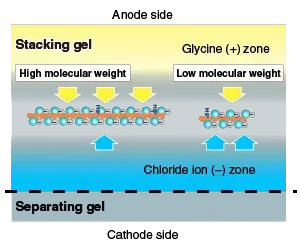
Proper gel preparation ensures the optimal conditions for separating proteins based on their size during gel electrophoresis.
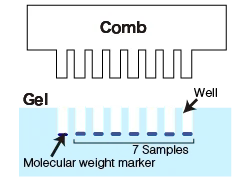
Once the gel is prepared, it can be used for gel electrophoresis experiments to separate and analyze proteins based on their molecular weight or other properties.
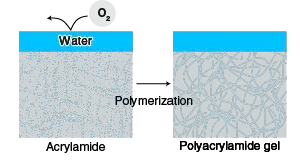
2. Sample preparation
Sample preparation is a crucial step in protein analysis and gel electrophoresis. Here are the steps involved in sample preparation:
- Boiling Water: Start by boiling water in a beaker. This water will be used for various steps in the sample preparation process.
- Preparation of Sample Buffer: Add 2-mercaptoethanol to the sample buffer. 2-mercaptoethanol helps to denature the proteins and reduce any disulfide bonds that may be present.
- Sample and Marker Preparation: Place the buffer solution in microcentrifuge tubes and add the protein sample to it. Similarly, prepare separate tubes containing molecular weight markers. These markers help determine the molecular weights of the proteins during gel electrophoresis.
- Protein Denaturation: Boil the samples, including the protein sample and markers, for a short duration, typically less than 5 minutes. This step ensures complete denaturation of the proteins.
- Addition of Sample Buffer: After boiling, add an appropriate volume of sample buffer to each sample tube. The sample buffer helps to stabilize the proteins and provides them with the necessary charge for electrophoresis.
- Mixing and Heat Treatment: Mix the contents of the tubes by flicking them gently to ensure proper mixing of the sample and buffer. Heat the tubes at 100°C for 3 minutes using a heat block. This step further denatures the proteins and helps to break down any secondary or tertiary structures.
- Centrifugation: After heat treatment, centrifuge the tubes at high speed (around 15,000 rpm) for 1 minute at 4°C. This step helps to separate any precipitated or insoluble material, and the supernatant is used for SDS-PAGE (sodium dodecyl sulfate-polyacrylamide gel electrophoresis).
- Loading Buffer Addition: To the protein sample (cell or tissue lysate), add an equal volume of loading buffer. The loading buffer contains additional components such as tracking dyes and stabilizers, which aid in sample loading and visualization during electrophoresis.
- Additional Boiling and Centrifugation: Boil the mixture of protein sample and loading buffer at 95°C for 5 minutes. Following this, centrifuge the mixture at a high speed (around 16,000 xg) for 5 minutes. These steps ensure complete denaturation and proper mixing of the sample before gel electrophoresis.
- Storage or Electrophoresis: The prepared samples can be stored at -20°C if not immediately used for gel electrophoresis. Alternatively, proceed with gel electrophoresis by loading the samples onto the gel.
Proper sample preparation is essential for accurate and reliable results in gel electrophoresis. It involves denaturation, reduction of disulfide bonds, and appropriate mixing of samples with buffer and loading dye to ensure optimal separation and analysis of proteins.
3. Electrophoresis
The steps of electrophoresis involve loading the denatured samples onto a polyacrylamide gel, applying a voltage to induce migration of proteins, and observing the separated bands. Here are the detailed steps:
- Gel Preparation: Prepare the polyacrylamide gel by casting it in a gel cassette or gel apparatus. The gel should be immersed in an electrophoresis buffer containing suitable electrolytes.
- Sample Loading: Using a pipette, carefully load the denatured protein samples into the wells of the gel. Each sample is placed in its designated well.
- Power Supply Connection: Place the gel cassette with the loaded samples into the electrode assembly of the electrophoresis apparatus. Ensure that it is securely fixed in the clamp stand. Connect the electrophoresis unit to a power supply.
- Electrophoresis Buffer Addition: Pour 1x electrophoresis buffer into the opening of the casting frame, allowing it to fill the wells of the gel. The buffer acts as a medium for the migration of proteins during electrophoresis.
- Application of Voltage: Once the gel is ready and the buffer is added, cover the tank with a lid to create a closed system. Turn on the power supply and apply a suitable voltage, typically around 100 V or 10-20 V per cm of gel length. The voltage causes negatively charged molecules, including proteins, to migrate through the gel towards the positively charged anode.
- Electrophoresis Duration: Allow the electrophoresis process to run for the desired duration, typically ranging from half an hour to several hours depending on factors such as the voltage applied and the length of the gel. The separation occurs as proteins of different sizes migrate through the gel at different rates, based on their molecular weights.
- Visualization of Bands: After electrophoresis, the gel is removed from the apparatus and bands are visualized. One common method is to use UV light to observe the protein bands. UV illumination allows for the visualization of bands that may be stained or labeled with fluorescent dyes.
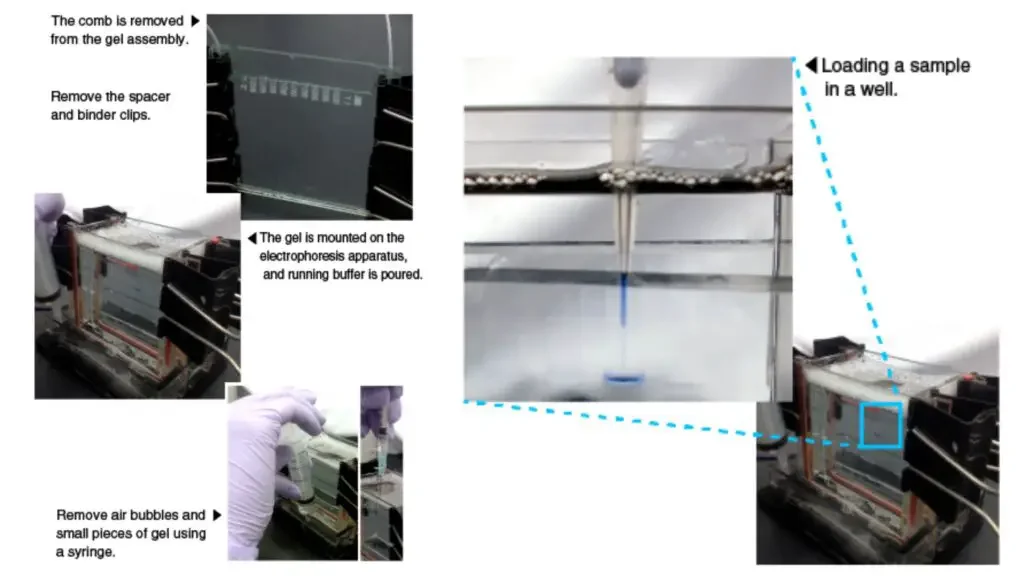
These steps provide a general overview of the process of electrophoresis, allowing proteins to be separated based on their molecular sizes. It is important to note that specific protocols and variations may exist depending on the type of electrophoresis technique or analysis being performed.
4. Gel staining
Gel staining is a crucial step in protein analysis that allows for the visualization and detection of separated proteins within the gel. Here is an overview of the gel staining procedure:
- Fixation: After the completion of electrophoretic separation, the gel is typically fixed to immobilize the proteins and prepare them for staining. A common fixation solution consists of 50% ethanol and 10% glacial acetic acid. The gel is immersed in this solution for approximately 1 hour.
- Staining solution preparation: While the gel is being fixed, the staining solution is prepared. For Coomassie staining, a widely used and straightforward method, the staining solution is composed of 50% methanol, 10% glacial acetic acid, and 0.1% Coomassie Brilliant Blue dye. The staining solution should be mixed thoroughly.
- Staining: After the fixation period, the gel is transferred to a fresh staining solution, ensuring complete immersion of the gel. The staining step allows the dye to bind to the proteins, resulting in their visualization as blue bands. The gel is typically stained for a period ranging from 1 to 12 hours, although the specific staining time can vary depending on the desired intensity of staining and the sensitivity of the proteins being analyzed.
- Destaining: Once the desired level of staining has been achieved, the gel is destained to remove excess dye and enhance the contrast between the stained protein bands and the gel background. The destaining solution consists of 40% methanol and 10% glacial acetic acid. The gel is immersed in the destaining solution, and the solution is changed several times to facilitate efficient destaining. The destaining process continues until the background is clear, and the protein bands are distinctly visible.
Different staining methods and dyes may have variations in the staining and destaining procedures. Some staining techniques, such as silver staining, amido black staining, and fast green FCF staining, may require different staining solutions and destaining protocols. Additionally, fluorescent stains and immunological detection methods like Western Blotting offer alternative approaches for protein visualization and analysis.
Gel staining is a critical step in protein analysis as it allows researchers to observe and analyze the separated proteins within the gel, providing valuable insights into protein size, abundance, and potential modifications.
5. Analysis
Analysis of protein samples following gel electrophoresis involves the examination and interpretation of the banding pattern obtained through protein staining. Here are some important considerations during the analysis:
- Banding Pattern: Protein staining in the gel results in the formation of distinct bands representing different proteins based on their molecular weight. The banding pattern provides a visual representation of the proteins present in the sample and their relative abundance.
- Glycoproteins: Glycoproteins, which have varying levels of glycosylation, can exhibit broader and blurred bands on the gel. This is due to the uneven adsorption of SDS at the glycosylation sites, affecting the migration and resulting in less well-defined bands.
- Membrane Proteins: Membrane proteins, characterized by their hydrophobic nature and tendency to bind lipids, may have lower solubility in aqueous solutions. This can lead to precipitation and incomplete migration in SDS-PAGE, causing a “tailing” effect above the band of the transmembrane protein. Adjusting the SDS concentration and protein loading can help mitigate this issue.
- Overloading: Overloading the gel with a high concentration of a particular soluble protein can result in a semicircular band, which may obscure or overlap with neighboring bands. This can make it challenging to distinguish proteins with similar molecular weights.
- Contrast and Purity: The contrast between bands within a lane can provide insights into the purity of the protein sample. Low contrast may indicate the presence of multiple proteins (low purity) or, in the case of purified proteins, proteolytic degradation. Degradation can be observed as degradation bands below the intact protein band, eventually resulting in a homogeneous “smear” below the degraded band.
- Documentation: The banding pattern is typically documented by photographing or scanning the gel. This allows for a permanent record of the protein separation and facilitates further analysis and comparison. Documentation is important for subsequent recovery or identification of specific protein bands.
- Gel Extraction: In some cases, it may be necessary to recover the proteins present in individual bands for further analysis or downstream applications. Gel extraction techniques can be employed to isolate and collect proteins from specific gel regions, enabling subsequent characterization or identification.
The analysis of protein banding patterns obtained from gel electrophoresis provides valuable information about the protein samples, including their molecular weight, relative abundance, and potential modifications. By carefully examining and interpreting these patterns, researchers can gain insights into the composition and behavior of the proteins under investigation.
Molecular mass determination
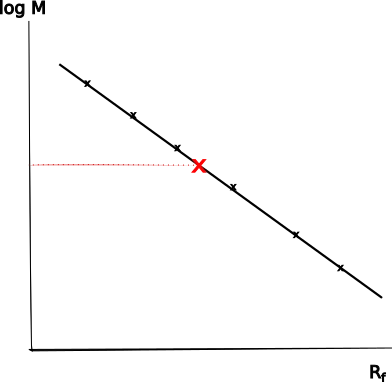
Molecular mass determination is an important aspect of protein analysis following gel electrophoresis. The following steps are involved in the process:
- Measurement of Relative Migration Distances: To determine the molecular weight of proteins accurately, the relative migration distances of individual protein bands are measured within the separating gel. These measurements are typically conducted in triplicate to improve precision.
- Calculation of Relative Mobility (Rf value): The relative mobility, also known as the Rf value or Rm value, is calculated by dividing the distance traveled by the protein band by the distance traveled by the buffer front. Both distances are measured from the beginning of the separation gel. The buffer front distance corresponds approximately to the migration distance of a dye, such as bromophenol blue, present in the sample buffer.
- Construction of a Semi-logarithmic Plot: The relative distances of proteins from the size marker are plotted semi-logarithmically against their known molecular weights. This plot allows for a linear relationship to be observed between the logarithm of the molecular weight and the relative mobility.
- Determination of Molecular Weight: By comparing the relative mobility of an unknown protein with the linear portion of the plot or performing regression analysis, the molecular weight of the protein can be estimated. This estimation is based on its relative mobility value.
Factors Affecting Molecular Weight Determination:
- Glycosylated Proteins: Protein bands with glycosylations may appear blurred, making the determination of their molecular weight less precise.
- Basic or Acidic Proteins: Proteins containing a high number of basic amino acids, such as histones, may exhibit slower migration due to their positive charges. This can result in an overestimation of their molecular weight or even prevent their migration into the gel. Conversely, proteins rich in acidic amino acids may migrate more quickly, leading to an underestimation of their molecular mass.
Molecular mass determination is a valuable tool for characterizing proteins and assessing their sizes. By accurately measuring relative migration distances and utilizing size markers, researchers can estimate the molecular weight of unknown proteins, providing insights into their structural properties and facilitating further analysis and comparison.
Different Gel Staining Methods
1. Coomassie Blue staining – Requirement and Procedure
Coomassie Blue staining is a widely used method for visualizing proteins separated by SDS-PAGE (Sodium Dodecyl Sulfate-Polyacrylamide Gel Electrophoresis). It provides high sensitivity and allows for long-term storage of the gels. Here is the procedure for Coomassie Blue staining:
Reagents Required:
- Gel Fix Solution (500 mL):
- Methanol: 250 mL
- Glacial acetic acid: 50 mL
- Water: 200 mL
- Coomassie Solution:
- Coomassie Brilliant Blue R-250 (CBB R-250): 0.1%
- Methanol: 40%
- Glacial acetic acid: 10%
- Filter the stain solution using Whatman No. 1 filter paper.
- Destain Solution:
- Methanol: 50 mL
- Glacial acetic acid: 35 mL
- Gel Storage Solution:
- Glacial acetic acid: 25 mL
- Water: 475 mL
Procedure:
- After completing the SDS-PAGE electrophoresis, carefully remove the gel from the gel apparatus and place it in a plastic tray.
- Add the Gel Fix Solution to the tray, ensuring that the gel is completely immersed. Place the tray on a rocking table or gently agitate the solution to fix the proteins. Allow the gel to fix for approximately 2 hours.
- Remove the Gel Fix Solution from the tray and discard it. Add the Coomassie Solution to the tray, covering the gel completely. Place the tray on a rocking table or gently agitate the solution to ensure uniform staining. Allow the gel to stain for 2-4 hours. The staining time may vary depending on the desired intensity of staining.
- After the staining step, carefully pour off the Coomassie Solution and wash the gel several times with distilled water. Rinse the gel gently to remove excess stain, and continue washing until the background becomes clear.
- Prepare the Destain Solution by combining methanol and glacial acetic acid. Add the Destain Solution to the tray, covering the gel completely. Place the tray on a rocking table or gently agitate the solution to destain the gel. Destain for approximately 4 hours or until clear blue bands against a clear background are visible. The duration of destaining can vary depending on the staining intensity and gel thickness.
- Once destaining is complete, carefully pour off the Destain Solution and discard it. The gel can now be stored or photographed as required.
To store the gel, prepare the Gel Storage Solution by combining glacial acetic acid and water. Transfer the gel into the Gel Storage Solution, ensuring it is fully immersed. The gel can be stored in this solution for long-term storage and subsequent analysis.
Coomassie Blue staining provides a visible representation of protein bands on the gel, allowing for analysis and documentation of protein separation.
2. Silver Staining – Requirement and Procedure
Silver staining is a highly sensitive protein detection method commonly used for visualizing proteins separated by SDS-PAGE (Sodium Dodecyl Sulfate-Polyacrylamide Gel Electrophoresis). It offers excellent sensitivity and is suitable for detecting low-abundance proteins. Here is the procedure for silver staining:
Reagents Required:
- Fixing Solution:
- Ethanol: 50 mL
- Acetic acid: 10 mL
- Water: 40 mL
- 30% Ethanol Solution
Procedure:
- After completing the SDS-PAGE electrophoresis, carefully remove the gel from the gel apparatus and place it in a suitable container.
- Prepare the Fixing Solution by combining ethanol, acetic acid, and water. Immerse the gel in the Fixing Solution and allow it to fix for 40 minutes. For a clearer background, an overnight immersion in the fixer can be done.
- Remove the Fixing Solution and wash the gel with 30% Ethanol Solution for 10 minutes. Ensure that the gel is fully immersed during the wash.
- After the ethanol wash, rinse the gel with ultrapure water for 10 minutes to remove any residual ethanol.
- Decant the water and prepare the Sensitizer Solution. Immerse the gel in the Sensitizer Solution and incubate for 10 minutes. The Sensitizer Solution enhances the sensitivity of protein detection during silver staining.
- Remove the Sensitizer Solution and wash the gel twice with water, with each wash lasting for 10 minutes. This step helps remove excess Sensitizer Solution.
- Decant the water and immerse the gel in the Silver Solution for 10 minutes. The Silver Solution allows the detection of proteins as silver-stained bands.
- Decant the Silver Solution and wash the gel with water for 1.5 minutes to remove unreacted silver ions.
- Prepare the Developer Solution according to the manufacturer’s instructions. Immerse the gel in the Developer Solution and incubate for 3 to 7 minutes. The Developer Solution triggers the development of silver-stained bands corresponding to the proteins of interest.
- To stop the development process, add 5 mL of Stop Solution to the Developer Solution and incubate for 5 minutes. The Stop Solution halts the reaction and fixes the developed bands.
- Remove the Developer/Stop Solution and wash the gel with ultrapure water for 15 minutes to remove any remaining chemicals.
- The silver-stained gel can be photographed or stored in fresh ultrapure water. Storing the gel in water helps preserve the silver-stained bands.
For double staining, the gel can be stained first with Coomassie Brilliant Blue R-250 (CBB R-250) following the manufacturer’s instructions, and then silver stained using the above procedure. This allows for visualizing proteins with high sensitivity and compatibility with downstream analysis techniques.
Fluorescent stains such as SYPRO and Lucy stains can also be used for protein electrophoresis. These stains offer the advantage of fluorescence detection and can be applied either by immersing the gel in the fluorescent stain in the dark or by mixing the stain with the cathode buffer during electrophoresis.
3. Reversible Gel Staining – Requirement and Procedure
Reversible gel staining is a useful technique that allows for the detection of proteins on polyacrylamide gels (PAGE gels) and subsequent Western blotting. It offers the advantage of reversibility, meaning that after staining, the gel can be destained and further processed for downstream applications. One example of a reversible gel stain is R-PROB, which specifically detects proteins. Here is the procedure for reversible gel staining:
Reagents Required:
- Reversible Protein Detection Kit for Membranes and Polyacrylamide Gels (RPROB)
- Fixing solution (F7264)
- 10% acetic acid
- EDTA 50 mM
Procedure:
- After completing the SDS-PAGE electrophoresis, carefully remove the gel from the gel apparatus and place it in a suitable container.
- Prepare the fixing solution according to the manufacturer’s instructions. Immerse the gel in the fixing solution and incubate for 20 minutes. Repeat this step twice to ensure proper fixation of the proteins on the gel.
- Rinse the fixed gel with water for two washes, with each wash lasting 30 minutes. This step helps remove residual fixing solution from the gel.
- Prepare the staining solution by following the instructions provided with the R-PROB stain. Incubate the gel in the staining solution for 20 to 40 minutes with gentle agitation. The R-PROB stain will bind to the proteins, allowing their visualization.
- After the staining step, carefully wash off the excess stain by rinsing the gel with 10% acetic acid. This helps remove any non-specifically bound stain from the gel.
- For destaining, prepare an EDTA solution with a concentration of 50 mM. Wash the gel with the EDTA solution to remove the stain. This step helps reverse the staining process by dissociating the stain from the proteins.
- After destaining with EDTA, wash the gel with water or fixing solution for two washes, with each wash lasting 15 minutes. These additional washes help remove any residual stain and ensure that the gel is ready for further processing.
Following reversible gel staining, the gel can be further processed for Western blotting. The destained gel can be transferred onto a membrane, and the proteins of interest can be detected using appropriate antibodies and detection methods.
4. Copper staining – Requirement and Procedure
Copper staining is a technique used to visualize the migration and separation of proteins on a gel. It involves the use of a copper chloride (CuCl2) solution as a reversible stain. Here is the procedure for copper staining:
Reagents Required:
- 0.3 M CuCl2 solution
- 0.25 M Tris and 0.25 M EDTA solution
Procedure:
- After completing the SDS-PAGE electrophoresis, remove the gel from the gel apparatus and rinse it briefly with distilled water. The rinse should not exceed 30 minutes.
- Prepare a 0.3 M CuCl2 solution. Immerse the gel in the CuCl2 solution and incubate for 10 minutes. The CuCl2 solution will bind to the proteins on the gel, allowing their visualization.
- After the staining step, rinse the gel with deionized water to remove excess stain. This step helps create a clear background for protein visualization.
- The proteins will appear as clear zones against a blue background. The intensity of staining may vary depending on the protein concentration and other factors.
- If you need to completely destain the gel for subsequent Western blotting or other applications, wash the stained gel in a 0.25 M Tris and 0.25 M EDTA solution with a pH of 9. Repeat this wash step multiple times until the gel is destained to the desired level.
- After destaining, the gel can be transferred to a transfer buffer for further processing, such as protein transfer to a membrane for Western blotting.
It is important to note that copper staining is a reversible stain, meaning the stain can be removed from the gel by appropriate washing steps. This allows for downstream applications without interference from the staining reagent.
Electrophoresis Buffers
Electrophoresis Buffers
Electrophoretic Method | |||
---|---|---|---|
SDS-PAGE (InvitrogenTM) | BN-PAGE (InvitrogenTM) | NSDS-PAGE | |
Sample Buffer | 106 mM Tris HCl 141 mM Tris Base 0.51 mM EDTA 0.22 mM SERVA Blue G-250 0.175 mM Phenol Red 2% LDS 10% Glycerol pH 8.5 | 50 mM BisTris 50 mM NaCl 16mMHCl 10% Glycerol 0.001% Ponceau S pH 7.2 | 100 mM Tris HCl 150 mM Tris Base 0.01875% Coomassie G-250 0.00625% Phenol Red 10% Glycerol pH 8.5 |
Run Buffer | 50 mM MOPS 50 mM Tris Base 1 mM EDTA 0.1% SDS pH 7.7 | Cathode 50 mM BisTris 50 mM Tricine 0.02% Coomassie G-250 pH 6.8 Anode 50 mM BisTris 50 mM Tricine pH 6.8 | 50 mM MOPS 50 mM Tris Base 0.0375% SDS pH 7.7 |
Applications of SDS-PAGE
SDS-PAGE, or Sodium Dodecyl Sulfate-Polyacrylamide Gel Electrophoresis, has a wide range of applications in various fields. Here are some key applications of SDS-PAGE:
- Molecular Weight Determination: SDS-PAGE is commonly used to measure the molecular weight of proteins and other molecules. By comparing the migration of protein samples with known molecular weight markers, the size of the protein can be estimated.
- Protein Size Estimation: SDS-PAGE allows for the estimation of the size of proteins based on their migration distance within the gel. This information is useful for studying protein structure and function.
- Peptide Mapping: SDS-PAGE can be used for peptide mapping, which involves the identification and characterization of peptides within a protein sample. This technique helps in studying protein structure, post-translational modifications, and sequence analysis.
- Comparative Analysis: SDS-PAGE enables the comparison of the polypeptide composition of different protein structures or samples. It can reveal differences in protein expression, identify isoforms, and assess protein purity.
- Protein Purity Assessment: SDS-PAGE is often used to evaluate the purity of protein samples. By visualizing the presence of additional bands or contaminants, the purity of a protein preparation can be determined.
- Western Blotting: SDS-PAGE is an integral part of Western blotting, a widely used technique for detecting and identifying specific proteins within a complex mixture. SDS-PAGE separates proteins based on size, and subsequent transfer to a membrane allows for the detection of target proteins using specific antibodies.
- Protein Ubiquitination Studies: SDS-PAGE is employed in the study of protein ubiquitination, a post-translational modification that regulates protein degradation and signaling. By separating and visualizing ubiquitinated proteins, researchers can investigate their role in cellular processes.
- HIV Test: In medical diagnostics, SDS-PAGE is utilized in the HIV test. It separates HIV proteins by their molecular weight, and subsequent Western blot analysis with patient-specific antibodies helps determine the presence of HIV-specific proteins in the blood serum.
- Proteinuria Evaluation: SDS-PAGE is used to assess proteinuria, a condition characterized by abnormal levels of proteins in the urine. By analyzing the levels of various serum proteins in urine samples using SDS-PAGE, such as Albumin, Alpha-2-macroglobulin, and IgG, proteinuria can be evaluated.
Overall, SDS-PAGE is a versatile technique that plays a vital role in protein analysis, protein characterization, and diagnostic applications. Its ease of use, cost-effectiveness, and compatibility with other analytical methods make it a valuable tool in various research and diagnostic laboratories.
Important Instructions for SDS PAGE
When performing SDS-PAGE, it is important to follow certain instructions to ensure accurate and reliable results. Here are some important instructions to keep in mind:
- Read the Procedure: Before starting the experiment, carefully read through the entire procedure to understand the steps involved and any specific requirements.
- Prepare Reagents in Advance: Prepare the required reagents, such as the 10 percent APS solution and 1X Tris-Glycine SDS Gel running buffer, prior to starting the experiment. Follow the instructions provided to accurately prepare these solutions. Store them at the recommended temperature and utilize them within the specified timeframe.
- Thaw Samples: If you have refrigerated samples, ensure that you thaw them completely before use. This will ensure that the samples are in the appropriate state for analysis.
- Cleanse the Equipment: Before starting the experiment, clean the entire machine or equipment you will be using. Use an appropriate detergent and distilled water to cleanse the equipment thoroughly. Ensure that the plates or gels are free from any dirt or contaminants that could affect the results.
- Mixing Reagents: Prior to use, mix the prepared solutions thoroughly. For example, mix the 1X Tris-Glycine SDS Gel running buffer well before adding it to the gel apparatus. Proper mixing ensures that the reagents are evenly distributed and ready for use.
- Reuse of Running Buffer: If specified, the 1X Tris-Glycine SDS Gel running buffer can be reused multiple times, typically up to 4-5 times. Keep track of the number of uses to avoid using it beyond the recommended limit. Check for any signs of contamination or degradation before reusing the buffer.
By following these important instructions, you can ensure the accuracy, reliability, and reproducibility of your SDS-PAGE experiments.
Advantages of SDS PAGE
SDS-PAGE (Sodium Dodecyl Sulfate Polyacrylamide Gel Electrophoresis) offers several advantages in protein analysis and characterization. Here are some key advantages of SDS-PAGE:
- Molecular Weight Determination: SDS-PAGE allows for the accurate determination of the molecular weight of proteins in a sample. By comparing the migration of protein bands on the gel with standard protein markers of known molecular weights, researchers can estimate the size of the protein under investigation.
- Protein Detection: SDS-PAGE enables the detection of specific proteins based on their unique characteristics and mass. By visualizing protein bands on the gel, researchers can identify the presence or absence of specific proteins in a sample. This is particularly useful in protein analysis and diagnostic applications.
- Species Identification: The differentiation of species based on protein mass is made possible by SDS-PAGE. By comparing the protein profiles of different species, researchers can identify and distinguish them based on their distinct protein patterns. This can be particularly valuable in fields such as evolutionary biology, forensics, and environmental monitoring.
- High Resolution: SDS-PAGE is a high-resolution gel electrophoresis system. It offers excellent separation and resolution of protein molecules based on their mass. This allows for precise analysis and characterization of proteins in complex mixtures, leading to a better understanding of protein composition and interactions.
- Wide Applicability: SDS-PAGE is widely applicable across various scientific disciplines. It is used in biochemistry, molecular biology, proteomics, clinical diagnostics, and pharmaceutical research. Its versatility and effectiveness make it a valuable tool in protein analysis and characterization.
- Comparability and Standardization: SDS-PAGE provides a standardized method for protein separation and analysis. The use of protein markers with known molecular weights allows for comparability between different experiments and laboratories. This ensures consistent and reliable results across different studies.
- Cost-Effective: SDS-PAGE is a relatively cost-effective method compared to other protein analysis techniques, such as mass spectrometry. It has lower instrument and reagent costs, making it accessible to a wide range of researchers and institutions.
In summary, SDS-PAGE offers numerous advantages in protein analysis, including accurate molecular weight determination, specific protein detection, species identification, high resolution, wide applicability, comparability, and cost-effectiveness. Its simplicity and effectiveness have made it a fundamental technique in the field of protein science.
Limitations of SDS PAGE
SDS-PAGE (Sodium Dodecyl Sulfate Polyacrylamide Gel Electrophoresis) has certain limitations that should be taken into consideration. Here are some limitations associated with SDS-PAGE:
- Limited Information about Structure: SDS-PAGE primarily provides information about the molecular weight and size of proteins. While it can help identify different protein bands, it does not provide detailed information about the three-dimensional structure or functional properties of proteins. Other techniques, such as X-ray crystallography or nuclear magnetic resonance (NMR), are required for more in-depth structural analysis.
- Nucleic Acid Detection: SDS-PAGE is not suitable for the visualization or analysis of nucleic acids, such as DNA or RNA. Since SDS-PAGE is designed to denature and separate protein molecules based on their mass, nucleic acids will not be visible on the gel. Specific techniques like agarose gel electrophoresis or polymerase chain reaction (PCR) are used for nucleic acid analysis.
- Limited Purity Assessment: While SDS-PAGE can indicate the presence of multiple protein bands and their relative intensities, it does not provide direct information about the purity of a protein sample. Quantification methods such as UV-Vis spectroscopy or other biochemical assays are needed to determine the purity of a protein sample accurately.
- Size-Based Resolution: SDS-PAGE separation is primarily based on the molecular weight of proteins. It may not effectively separate proteins with similar molecular weights but different structural or conformational characteristics. Other techniques, such as two-dimensional gel electrophoresis or liquid chromatography, may be required for higher resolution and better separation of complex protein mixtures.
- Sample Preparation Artifacts: SDS-PAGE requires proper sample preparation, including denaturation and reduction of disulfide bonds, to ensure accurate results. Improper sample handling or incomplete denaturation can lead to artifacts or distorted protein bands on the gel, affecting the interpretation of results.
- Inherent Variability: SDS-PAGE, like any experimental technique, has inherent variability and limitations related to reproducibility. Factors such as gel preparation, running conditions, and staining protocols can introduce variability between experiments, affecting the consistency of results.
- Limited Dynamic Range: SDS-PAGE has a limited dynamic range for protein separation. It may not be suitable for analyzing proteins with very high or very low molecular weights, as they may be difficult to visualize or may migrate outside the gel matrix.
Despite these limitations, SDS-PAGE remains a valuable and widely used technique in protein analysis due to its simplicity, cost-effectiveness, and ability to provide information on protein size and separation. It is often used in combination with other techniques to overcome these limitations and obtain a more comprehensive understanding of proteins.
Problems with solution
When working with solutions in various laboratory techniques, certain problems may arise that can affect the quality and reliability of the results. Here are some common problems that can occur with solutions and their potential solutions:
- Low Current and Long Run Time: If the run takes an unusually long time due to low current, increase the voltage by 25-50%. This will help maintain an appropriate current flow and expedite the electrophoresis process.
- Poor Resolution of Gel: If the gel exhibits poor resolution, it may be due to overloading the wells with too much sample. Avoid overfilling the wells as it can lead to artifacts and affect the separation. Follow the recommended volume of gel loading for the specific gel size. Adjust the sample loading accordingly if using a smaller gel.
- Stacking Gel Length: When pouring the stacking gel, ensure that its length is approximately 1 cm from the bottom of the well to the top of the separating gel. This will allow for proper stacking of the protein samples and enhance the resolution.
- Fast Run Time: If the electrophoresis run occurs too quickly, it may be due to the applied current being too high. Decrease the voltage by 25-50% to reduce the speed of the run and achieve better separation.
- Band Overload for Purified Protein Sample: If multiple bands are seen for a purified protein sample, it may indicate an issue with the sample preparation. Ensure that the sample is prepared correctly and without contamination. Carry out the electrophoresis as soon as the sample is prepared to minimize any potential degradation or additional bands.
- Polymerization of Stacking Gel: Allow the stacking gel to polymerize for at least 30 minutes before removing the combs. This ensures that the gel has properly solidified, allowing the samples to sink to the bottom of the well during loading.
- Bubble-Free Gel: When pouring the gel, make sure there are no bubbles present. Bubbles can cause bands on certain parts of the gel to remain in place and not move down during electrophoresis. Take care to eliminate any bubbles before the gel solidifies.
- Proper Screw Tightening: Avoid overtightening the screws on the clamp assembly. Tightening them excessively can distort the gel and affect the separation. Use an appropriate level of tightening to secure the gel without causing deformation.
- Homogeneity of Gel Mixture: Before pouring the gel, ensure that the gel mixture is well mixed. Thoroughly mix the components to achieve a homogeneous solution, as an uneven mixture can lead to inconsistent gel formation and affect the quality of the results.
By addressing these common problems with solutions, you can improve the reliability and accuracy of your experimental outcomes in various laboratory techniques.
FAQ
What is SDS-PAGE?
SDS-PAGE (Sodium Dodecyl Sulfate Polyacrylamide Gel Electrophoresis) is a technique used to separate proteins based on their molecular weight. It involves denaturing proteins with SDS and subjecting them to electrophoresis through a polyacrylamide gel matrix.
Why is SDS used in SDS-PAGE?
SDS is an anionic detergent that denatures proteins, coats them with a negative charge relative to their mass, and unfolds them into linear structures. This allows the proteins to be separated based on size during electrophoresis.
What is the purpose of a stacking gel in SDS-PAGE?
The stacking gel serves as a sieving matrix that helps concentrate the protein samples into thin, focused bands before they enter the separating gel. It improves the resolution and separation of proteins during electrophoresis.
How does SDS-PAGE separate proteins?
During SDS-PAGE, an electric field is applied to the gel, causing proteins to migrate through the gel matrix based on their size. The smaller proteins move faster and migrate further, while larger proteins migrate more slowly and remain closer to the sample loading wells.
What is the purpose of a molecular weight marker in SDS-PAGE?
A molecular weight marker, also known as a protein ladder, is a mixture of proteins with known molecular weights. It is loaded onto the gel alongside the samples and serves as a reference to estimate the size of the unknown proteins in the samples.
How is protein visualization achieved in SDS-PAGE?
After electrophoresis, proteins are often visualized by staining the gel with dyes such as Coomassie Brilliant Blue or silver stain. These dyes bind to proteins and allow their detection as bands or spots on the gel.
Can SDS-PAGE be used to determine protein concentration?
While SDS-PAGE provides information about protein size and separation, it is not a reliable method for quantifying protein concentration. Spectrophotometric methods, such as Bradford assay or BCA assay, are commonly used for protein quantification.
Can SDS-PAGE identify post-translational modifications?
SDS-PAGE alone cannot identify specific post-translational modifications (PTMs). Additional techniques, such as Western blotting or mass spectrometry, are typically employed to detect and characterize PTMs.
What are the limitations of SDS-PAGE?
Some limitations of SDS-PAGE include its inability to provide detailed structural information, difficulty in visualizing nucleic acids, limited assessment of purity, variability in results, and restricted dynamic range for very large or small proteins.
What are the applications of SDS-PAGE?
SDS-PAGE is widely used in various fields of research and industry. It is utilized for protein analysis, purification, characterization, and protein-protein interaction studies. It is also an important technique in molecular biology, biochemistry, biotechnology, and clinical diagnostics.
References
- Nowakowski AB, Wobig WJ, Petering DH. Native SDS-PAGE: high resolution electrophoretic separation of proteins with retention of native properties including bound metal ions. Metallomics. 2014 May;6(5):1068-78. doi: 10.1039/c4mt00033a. PMID: 24686569; PMCID: PMC4517606.
- https://ruo.mbl.co.jp/bio/e/support/method/sds-page.html
- https://www.aatbio.com/resources/buffer-preparations-and-recipes/sds-page-10x-sds-running-buffer
- https://www.bio-rad.com/en-in/applications-technologies/sds-page-analysis?ID=LW7FGX4VY
- http://microbiology.ucdavis.edu/heyer/wordpress/wp-content/uploads/2013/11/dna_page.pdf
- https://www.cbrnetechindex.com/Biological-Detection/Technology-BD/Electrophoresis-BD-T/Polyacrylamide-Gel-Electrophoresis-BD-E
- https://www.rockland.com/resources/sds-page-protocol/
- https://www.bio.davidson.edu/Courses/genomics/method/SDSPAGE/SDSPAGE.html
- https://www.ruf.rice.edu/~bioslabs/studies/sds-page/gellab2.html
- https://www.sigmaaldrich.com/IN/en/technical-documents/protocol/protein-biology/gel-electrophoresis/sds-page
- https://thesciencenotes.com/sds-page/