What is Phytoplankton?
- Phytoplankton, derived from the Greek words φυτόν (phyton) meaning ‘plant’ and πλαγκτός (planktos) signifying ‘wanderer’ or ‘drifter,’ represent the autotrophic constituents of the planktonic assemblage, constituting a pivotal element within both marine and freshwater ecosystems.
- These diminutive organisms are pivotal in understanding the complex dynamics of aquatic environments. Phytoplankton are indispensable contributors to the global carbon cycle and serve as the primary foundation of marine and freshwater food chains, where they engage in the vital process of photosynthesis akin to their terrestrial counterparts.
- The life of phytoplankton hinges upon photosynthesis, the same physiological mechanism that sustains terrestrial plants. To facilitate this process, phytoplankton necessitate an abundant supply of solar illumination.
- Accordingly, they thrive within the well-illuminated surface layers, known as the euphotic zone, prevalent in oceans and lakes. Unlike their terrestrial counterparts, phytoplankton display a dispersed distribution over a significantly larger surface area. This distinctive trait renders them less susceptible to seasonal fluctuations and imparts an ability to respond with alacrity to global climatic fluctuations.
- Despite their diminutive size, phytoplankton exert a disproportionate influence on the Earth’s ecosystems. These microorganisms collectively account for approximately half of the planet’s photosynthetic activity and contribute to at least half of global oxygen production.
- Remarkably, their biomass constitutes a mere 1% of the total global plant biomass. Phytoplankton’s significance transcends their size; they are taxonomically diverse, encompassing a spectrum ranging from photosynthetic bacteria to plant-like algae and armored coccolithophores.
- Prominent among these are the diatoms, cyanobacteria, and dinoflagellates, with numerous other taxa populating the phytoplanktonic realm.
- Typically, phytoplankton organisms are imperceptible to the unaided eye due to their minuscule dimensions. However, when they aggregate in substantial quantities, they manifest as discernible patches of color on the water’s surface. These vibrant hues are attributed to the presence of chlorophyll within their cellular structures, complemented by accessory pigments such as phycobiliproteins or xanthophylls in specific species.
- In summary, phytoplankton constitute an imperative element of aquatic ecosystems, with their photosynthetic prowess shaping the dynamics of oceans, seas, and freshwater bodies. Their ability to harness sunlight for energy production, coupled with rapid responses to climatic variations, underscores their pivotal role in global biogeochemical cycles. The taxonomic diversity among phytoplankton further enriches the intricate tapestry of life within Earth’s aquatic realms, exemplifying nature’s ability to thrive in even the most minuscule and enigmatic forms.
Definition of Phytoplankton
Phytoplankton are microscopic, photosynthetic organisms found in aquatic environments that serve as the foundation of marine and freshwater food chains and contribute significantly to global oxygen production and carbon cycling.
Characteristics Features of Phytoplankton
Phytoplankton are a diverse group of microscopic photosynthetic organisms that inhabit aquatic ecosystems, and they exhibit several characteristic features that define their biology and ecological roles. These features include:
- Microscopic Size: Phytoplankton are typically very small, with individual cells measuring from a few micrometers to a few hundred micrometers in size. Their diminutive dimensions make them virtually invisible to the naked eye, and they require specialized microscopes for observation.
- Photosynthetic Autotrophy: Phytoplankton are autotrophic, meaning they produce their own food through photosynthesis. They use sunlight, carbon dioxide (CO2), and nutrients to synthesize organic compounds, primarily chlorophyll, which gives them their green color. This ability is fundamental to their role as primary producers in aquatic food webs.
- Buoyancy: Many phytoplankton possess adaptations for buoyancy control, enabling them to move vertically within the water column to access optimal light and nutrient conditions. Some species have gas vesicles or oil droplets that aid in buoyancy regulation.
- Diversity: Phytoplankton encompass a wide diversity of taxonomic groups, including cyanobacteria (blue-green algae), diatoms, dinoflagellates, coccolithophores, and green algae, among others. This taxonomic diversity translates into a variety of ecological functions and responses to environmental conditions.
- Silica and Calcium Carbonate Shells: Diatoms and some coccolithophores are characterized by unique shells made of silica and calcium carbonate, respectively. These protective structures, known as frustules (for diatoms) and coccoliths (for coccolithophores), contribute to their distinctive appearances and play essential roles in their survival and ecology.
- Rapid Reproduction: Phytoplankton can reproduce rapidly under favorable conditions, leading to the formation of dense populations or “blooms.” This capacity for rapid growth makes them highly responsive to changes in nutrient availability and light levels.
- Role in Food Webs: Phytoplankton form the base of aquatic food webs, serving as a primary food source for zooplankton, small fish, and other aquatic organisms. This fundamental role in trophic dynamics connects them to higher trophic levels and influences ecosystem structure and productivity.
- Seasonal and Spatial Variability: Phytoplankton populations exhibit seasonal and spatial variability in response to changing environmental conditions. Factors such as temperature, nutrient availability, and light penetration influence their distribution and abundance.
- Global Carbon Cycling: Phytoplankton are crucial contributors to the global carbon cycle. They capture and store atmospheric CO2 through photosynthesis, and when they die and sink to deeper ocean layers, they transport carbon to the ocean’s interior, contributing to long-term carbon sequestration.
- Environmental Indicators: Changes in phytoplankton abundance and composition can serve as indicators of environmental health and water quality. Monitoring phytoplankton communities helps assess the impacts of factors like nutrient pollution, climate change, and ocean acidification on aquatic ecosystems.
In summary, phytoplankton are vital components of aquatic ecosystems, displaying distinctive characteristics that underpin their ecological roles, adaptability, and significance in global biogeochemical processes. Their microscopic stature belies their immense ecological influence and underscores the importance of understanding and conserving these microscopic wonders.
What Do Phytoplankton Eat?
Phytoplankton, the microscopic photosynthetic organisms inhabiting aquatic environments, exhibit a distinct nutritional requirement driven by their autotrophic nature. These primary producers, crucial to aquatic food webs and global biogeochemical cycles, rely on specific minerals and vitamins for their sustenance.
1. Macronutrients: Phytoplankton primarily depend on essential macronutrients abundant in aquatic environments. These macronutrients include:
- Iron: Iron is a critical micronutrient for phytoplankton, as it plays a fundamental role in various cellular processes, particularly photosynthesis. Iron limitation can curtail phytoplankton growth, impacting overall productivity.
- Phosphate: Phosphate serves as a vital source of phosphorus, a key component of nucleic acids and energy-rich molecules like adenosine triphosphate (ATP). Adequate phosphate availability is essential for phytoplankton metabolic functions.
- Silicic Acid: Silicic acid is especially important for diatoms, a prominent phytoplankton group characterized by their silica-based cell walls. Diatoms require silicic acid for the construction of their frustules or shells.
- Nitrate: Nitrate serves as a primary nitrogen source for many phytoplankton species. Nitrogen is an essential component of amino acids, proteins, and chlorophyll, making nitrate a critical nutrient for phytoplankton growth.
2. Vitamin B: Phytoplankton also require certain vitamins to support various metabolic processes. Notably, Vitamin B is indispensable for phytoplankton survival. This group of water-soluble vitamins, including various B vitamins such as B1 (thiamine), B2 (riboflavin), B7 (biotin), and others, participates in enzymatic reactions essential for cellular metabolism. A deficiency in these vitamins can impede phytoplankton growth and overall ecosystem productivity.
In aquatic ecosystems, the availability of these macronutrients and vitamins is a pivotal factor in regulating phytoplankton populations. Nutrient limitation, wherein one or more of these essential components is in short supply, can lead to reduced phytoplankton abundance and altered community compositions. Such shifts in phytoplankton populations can reverberate throughout the food web, affecting higher trophic levels and biogeochemical cycling.
Understanding the nutritional requirements of phytoplankton is integral to comprehending the intricate dynamics of aquatic ecosystems and their responses to environmental changes. Moreover, phytoplankton’s reliance on specific nutrients underscores their role as sentinel organisms in assessing water quality and ecological health in aquatic environments.
Types of Phytoplankton
Phytoplankton, the microscopic photosynthetic organisms that populate aquatic ecosystems, exhibit remarkable diversity in their structural and compositional attributes. Their classification is contingent upon distinctive features, which encompass cell wall arrangement, cell structure, size, and habitat preferences. These nuanced classifications offer valuable insights into the multifaceted world of phytoplankton. In accordance with cell wall characteristics and cell structure, phytoplankton are broadly categorized into five types:
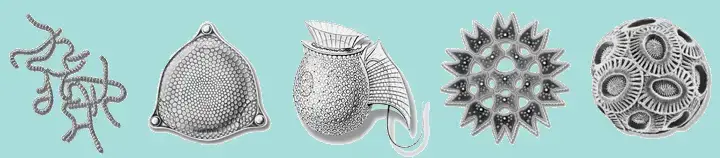
- Diatoms: Diatoms are microalgae characterized by their intricate shell-like structures composed of translucent silica. Their classification is based on the nature of their valves and the ornamentation found within them:
- Centric Diatoms: These diatoms exhibit a discoid, cylindrical, or solenoid cell shape, with markings radiating from the center.
- Pennate Diatoms: Pennate diatoms assume ovate, elongated, fusiform, sigmoid, or roughly circular shapes, with markings situated on either side of the apical axis.
- Dinoflagellates: Dinoflagellates are unicellular organisms propelled by two flagella, enabling locomotion. They may be either naked or armored with cellulose plates, and many possess the intriguing property of bioluminescence.
- Blue-Green Algae: These organisms, often found in colonies, derive their blue coloration from the pigment phycocyanin. While typically unicellular, they can exhibit colonial growth.
- Green Algae: Green algae are characterized by their green hue, a consequence of the presence of chloroplasts. They predominantly inhabit coastal waters in tropical and subtropical regions.
- Coccolithophores: Coccolithophores represent the smallest phytoplankton species frequently observed in open sea environments. They possess soft bodies enveloped by diminutive, calcified circular shields. Some coccolithophores possess flagella, while others lack this locomotive appendage.
Additionally, phytoplankton can be categorized based on their size, with size classes ranging from the minuscule to the substantial:
- Ultraplankton: These are the tiniest of phytoplankton, measuring less than 2 µm in size.
- Nanoplankton: Falling within the range of 2 to 20 µm, nanoplankton encompass a broader size spectrum.
- Microplankton: Ranging from 20 to 200 µm, microplankton form a significant component of phytoplankton communities.
- Macroplankton: Sized between 200 and 2000 µm, macroplankton represent larger and more conspicuous phytoplankton.
- Megaplankton: This category comprises phytoplankton exceeding 2000 µm in size, constituting the largest members of the phytoplankton community.
Furthermore, phytoplankton exhibit habitat preferences, leading to their classification based on their habitat:
- Neritic Phytoplankton: Inhabit the waters overlying continental shelves.
- Oceanic Phytoplankton: Thrive in the vast expanses of ocean waters beyond continental shelves.
- Brackish Water Phytoplankton: Populate brackish water environments, including mangroves, estuaries, and lagoons, where freshwater and saltwater mix, creating unique ecological niches.
These taxonomic classifications serve as a foundation for understanding the intricate diversity and ecological roles of phytoplankton in aquatic ecosystems, underscoring the significance of these microscopic organisms in global biogeochemical cycles and food webs.
Economic Importance of Phytoplankton
Phytoplankton, the microscopic photosynthetic organisms inhabiting aquatic environments, hold significant economic importance owing to their diverse applications in various industries. These autotrophic microorganisms, although diminutive in size, offer multifaceted contributions to the global economy. The following table elucidates some notable phytoplankton species and their diverse applications:
Phytoplankton Species | Applications |
---|---|
Chlorella vulgaris | – Food supplement for humans and animals |
– Valuable source of animal feed | |
Spirulina | – Nutritional food supplement for humans |
– Utilized in cosmetic products | |
Odontella aurita | – Cosmetic industry, as a natural ingredient |
– Pharmaceuticals, including drug synthesis | |
– Nutrition, suitable for both adults and infants | |
Phaeodactylum tricornutum | – Fuel production, particularly biodiesel |
– Nutritional applications | |
Porphyridium cruentum | – Cosmetic industry, utilized in cosmetic formulations |
– Pharmaceuticals, contributing to drug production | |
– Nutritional value, enhancing diets for various purposes |
These applications exemplify the versatility of phytoplankton and their significance across various sectors of the economy. Phytoplankton are not only valuable as nutritional supplements for both human and animal consumption but also serve as essential components in industries such as cosmetics and pharmaceuticals. Additionally, certain phytoplankton species, like Phaeodactylum tricornutum, hold promise in biofuel production, offering a sustainable source of energy.
Furthermore, the cultivation and commercialization of phytoplankton have emerged as viable economic ventures. Mass cultivation of specific phytoplankton species has the potential to generate substantial income, particularly in the context of the growing demand for natural and sustainable products in the food, cosmetic, and pharmaceutical industries.
In summary, the economic importance of phytoplankton is underscored by their pivotal roles in nutrition, pharmaceuticals, cosmetics, and even as potential biofuel sources. As industries continue to explore sustainable and environmentally friendly alternatives, phytoplankton are poised to play an increasingly prominent role in meeting these demands while contributing to economic growth.
Factors affecting productivity of Phytoplankton
The productivity of phytoplankton, the microscopic photosynthetic organisms in aquatic ecosystems, is influenced by a complex interplay of environmental factors. Understanding these factors is crucial for predicting the response of phytoplankton to changing conditions and their impact on global carbon cycling. Here, we delve into the scientific aspects of the factors affecting phytoplankton productivity:
- Temperature: Temperature plays a fundamental role in phytoplankton physiology and productivity. It influences metabolic rates, growth rates, and the timing of bloom events. Global warming projections indicate rising ocean temperatures, which can have both positive and negative effects on phytoplankton productivity, depending on the species and region.
- Irradiance (Light Intensity): Light is the primary energy source for phytoplankton through photosynthesis. Light intensity and quality (wavelength) affect the rate of photosynthesis. Changes in cloud cover, sea ice extent, and ocean circulation can alter the amount and quality of light reaching the surface, impacting phytoplankton growth.
- Nutrient Concentrations: Nutrient availability, including macronutrients like nitrogen and phosphorus, is a critical factor influencing phytoplankton productivity. Adequate nutrient supply supports robust growth, while nutrient limitation can restrict productivity. Changes in nutrient cycling due to factors like stratification and ocean acidification can affect nutrient availability.
- Carbon Dioxide (CO2): CO2 is both a carbon source for photosynthesis and a factor influencing ocean acidification. Elevated CO2 levels can potentially enhance photosynthesis in some phytoplankton species, but this effect may be offset by other environmental changes, such as nutrient limitation.
- Stoichiometry: The elemental composition (stoichiometry) of phytoplankton, including the ratios of carbon, nitrogen, and phosphorus, has significant implications for their nutritional quality. Stoichiometry influences the transfer of energy through marine food webs, impacting higher trophic levels.
- Climate Change: Climate change, driven by factors like global warming and altered ocean circulation, can lead to shifts in phytoplankton communities. These changes may have cascading effects on marine food webs and carbon transport to the ocean interior.
- Stratification: Ocean stratification refers to the layering of water based on temperature and salinity. Increased stratification can reduce the vertical mixing of nutrients from deeper waters to the surface, potentially limiting nutrient availability for phytoplankton in surface waters.
- Ocean Acidification: Rising atmospheric CO2 levels lead to ocean acidification, which can affect phytoplankton physiology. Some species may benefit from increased CO2, while others may face challenges in building their calcium carbonate shells or tests.
- Nutrient Supply: Changes in oceanic circulation patterns can impact the supply of nutrients to surface waters. Reduced nutrient supply, often associated with increased stratification, can lead to nutrient limitation for phytoplankton.
- Future Projections: Global change, including warming, altered stratification, and changes in nutrient supply, is expected to influence phytoplankton productivity. Climate models project a range of scenarios, and understanding how these changes interact is critical for predicting future phytoplankton dynamics.
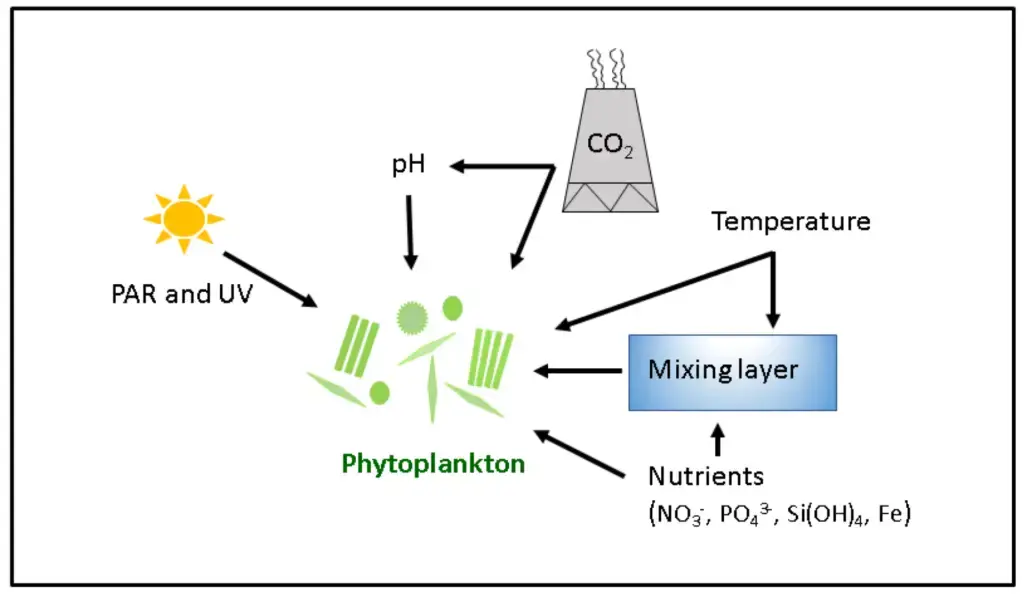
In summary, the productivity of phytoplankton is intricately linked to a suite of environmental factors, and their responses to changing conditions have far-reaching implications for marine ecosystems and the global carbon cycle. Scientific research plays a vital role in unraveling these complex interactions and forecasting the consequences of environmental changes on phytoplankton communities.
Factors affecting abundance of Phytoplankton
The abundance of phytoplankton, the microscopic photosynthetic organisms in aquatic ecosystems, is influenced by a complex interplay of factors, ranging from environmental conditions to ecological interactions. Here, we explore the key factors that affect the abundance of phytoplankton in a scientific context:
- Nutrient Availability: Nutrients, particularly macronutrients like nitrogen, phosphorus, and silicic acid, play a pivotal role in regulating phytoplankton abundance. The availability of these nutrients in aquatic environments directly influences the growth and proliferation of phytoplankton. Nutrient-rich areas often exhibit higher phytoplankton biomass.
- Light Intensity: Phytoplankton are photosynthetic organisms dependent on sunlight for energy through photosynthesis. Light intensity and its penetration depth into the water column dictate the depth at which phytoplankton can thrive. Inadequate light limits their growth, while sufficient light promotes photosynthesis and higher abundance.
- Water Temperature: Temperature affects phytoplankton metabolism and growth rates. Warmer waters generally support increased metabolic activity and, in some cases, lead to higher phytoplankton abundance. However, temperature preferences vary among different phytoplankton species.
- Stratification: Water column stratification, caused by differences in temperature and salinity, can affect the vertical distribution of phytoplankton. Stable stratification can trap phytoplankton in the surface layer, enhancing their abundance, while mixing events may redistribute them.
- Predation and Grazing: Phytoplankton are grazed upon by various zooplankton and other herbivores. The abundance of herbivores, their feeding rates, and the presence of top predators in the food web influence phytoplankton populations. Grazing pressure can regulate phytoplankton abundance.
- Competition: Phytoplankton species often compete for limited resources, including nutrients and light. Competitive interactions among different species can determine which species dominate and influence overall abundance patterns.
- Climate Variability: Climatic factors, such as El Niño-Southern Oscillation (ENSO) events and oceanic circulation patterns, can impact phytoplankton dynamics. ENSO, for instance, can disrupt typical nutrient supply mechanisms, affecting phytoplankton abundance.
- Biological Interactions: Phytoplankton can engage in mutualistic relationships with other organisms, such as symbiotic interactions with bacteria. These relationships can influence their growth and overall abundance.
- Human Activities: Anthropogenic factors, such as nutrient pollution from agricultural runoff or industrial discharges, can lead to nutrient enrichment in aquatic ecosystems, potentially causing harmful algal blooms. Human-induced changes in water temperature and stratification also affect phytoplankton.
- Research Efforts: Scientific studies, like the North Atlantic Aerosols and Marine Ecosystems Study (NAAMES), aim to better understand phytoplankton dynamics and their impacts on atmospheric aerosols, clouds, and climate. Such research contributes to our knowledge of the factors influencing phytoplankton abundance.
In conclusion, the abundance of phytoplankton is governed by a complex interplay of environmental factors, ecological interactions, and human influences. These factors collectively shape phytoplankton populations in aquatic ecosystems, with implications for nutrient cycling, food webs, and the broader functioning of marine and freshwater environments. Scientific investigations, such as the NAAMES study, provide valuable insights into these intricate relationships.
Diversity of Phytoplankton
The diversity of phytoplankton, the microscopic photosynthetic organisms inhabiting aquatic ecosystems, is a fascinating aspect of marine and freshwater biology. Phytoplankton represent a diverse assemblage of photoautotrophic microorganisms in aquatic food webs, contributing significantly to ecosystem structure and functioning. Here, we explore the remarkable diversity of phytoplankton in a scientific context:
- Taxonomic Diversity: Phytoplankton encompass a vast array of taxonomic groups, including eukaryotic protists, prokaryotic cyanobacteria, and archaea. This taxonomic diversity distinguishes them from terrestrial autotrophs, which are predominantly plants. It is estimated that there are approximately 5,000 known species of marine phytoplankton, with countless more yet to be discovered and described.
- Protistan Eukaryotes: A significant portion of phytoplankton diversity consists of protistan eukaryotes, which include various groups of algae. These algae exhibit a wide range of morphological and physiological adaptations, allowing them to thrive in diverse aquatic environments.
- Prokaryotic Phytoplankton: In addition to eukaryotic algae, prokaryotic phytoplankton are represented by cyanobacteria, which are among the most ancient photosynthetic microorganisms on Earth. Cyanobacteria play a critical role in primary production and nutrient cycling in aquatic ecosystems.
- Dominant Groups: While phytoplankton diversity is extensive, several groups stand out as ecologically significant. These include:
- Diatoms: Diatoms are renowned for their intricate silica cell walls and are among the most abundant and diverse phytoplankton in both marine and freshwater environments.
- Cyanobacteria: Cyanobacteria, including Prochlorococcus and Synechococcus, are the dominant photosynthetic prokaryotes in the world’s oceans. They thrive in oligotrophic regions and are vital contributors to primary production.
- Dinoflagellates: Dinoflagellates are another prominent group of phytoplankton known for their diversity and ecological significance. They often dominate in more productive ecosystems, influenced by upwelling or terrestrial nutrient inputs.
- Ecological Niches: Phytoplankton have evolved diverse ecological strategies to occupy specific niches within aquatic ecosystems. Their adaptations include differences in size, shape, photosynthetic pigments, and nutrient utilization. These adaptations enable various phytoplankton species to thrive in distinct environmental conditions.
- Dimethyl Sulfide Production: Some phytoplankton, like coccolithophorids, are involved in the production of dimethyl sulfide (DMS), a sulfur-containing compound. DMS can influence cloud formation and albedo, impacting climate dynamics through the CLAW hypothesis.
- Trophic Interactions: Phytoplankton diversity influences trophic interactions within aquatic food webs. Different phytoplankton species support various trophic levels, from herbivorous zooplankton to higher predators. This diversity is a critical driver of ecosystem productivity and stability.
- Biogeographic Variations: The composition of phytoplankton communities varies geographically and in response to environmental conditions. Oligotrophic regions often feature small-sized picoplankton and nanoplankton, while more productive areas favor larger dinoflagellates.
In conclusion, the diversity of phytoplankton is a testament to the adaptability and resilience of these microscopic organisms. Their taxonomic variety and ecological strategies contribute to the intricate web of life in aquatic ecosystems, shaping nutrient cycling, carbon sequestration, and the functioning of the world’s oceans and freshwater bodies. Understanding phytoplankton diversity is crucial for unraveling the complexity of aquatic ecosystems and their responses to environmental changes.
Aquaculture, the controlled cultivation of aquatic organisms, relies significantly on phytoplankton as a key food source for the animals being farmed. This practice is common in both aquaculture and mariculture, where phytoplankton serves as a vital component of the diet for various aquatic species. Here, we delve into the role of phytoplankton in aquaculture:
1. Mariculture: In mariculture, phytoplankton naturally occurs in the aquatic environment and is introduced into enclosures where farmed species are cultivated. The circulation of seawater within these enclosures helps distribute and maintain the phytoplankton populations, which serve as a primary food source for the cultured organisms.
2. Aquaculture: In contrast, aquaculture involves the intentional cultivation of phytoplankton, which is then used as food for the cultured organisms. Phytoplankton can be obtained through two primary methods:
- Collection from Natural Sources: Phytoplankton can be collected directly from natural bodies of water, although this method is less common due to the challenges associated with collecting and maintaining diverse phytoplankton populations.
- Cultivation: The more prevalent approach is to culture phytoplankton under controlled conditions. Phytoplankton production in artificial settings is itself a form of aquaculture. Culture sizes can vary widely, from small-scale laboratory cultures to large-scale commercial operations.
3. Purpose of Phytoplankton Cultivation: Phytoplankton cultivation serves multiple purposes in aquaculture:
- Foodstock Production: Phytoplankton is often cultivated to produce a food source for other aquacultured organisms. For example, phytoplankton is used to culture rotifers, which are then used to feed various aquatic species.
- Nutritional Supplement: Phytoplankton can also serve as a nutritional supplement for captive invertebrates kept in aquaria, providing essential nutrients and supporting the health of these organisms.
4. Culture Conditions: To ensure efficient growth of plankton, specific conditions must be provided in the cultivation process:
- Culture Medium: Marine phytoplankton cultures typically use seawater with a specific gravity ranging from 1.010 to 1.026 as the culture medium.
- Sterilization: The culture medium must be sterilized to prevent biological contamination, often achieved through high-temperature autoclaving or ultraviolet radiation exposure.
- Nutrient Addition: Fertilizers are added to the culture medium to facilitate plankton growth by providing essential nutrients.
- Aeration and Agitation: Continuous aeration or agitation is essential to keep phytoplankton suspended in the culture and to supply dissolved carbon dioxide for photosynthesis.
- Light: Phytoplankton require light for photosynthesis. The illumination provided typically has a color temperature of around 6,500 K, with variations in temperature successfully used in different systems. A daily light exposure of approximately 16 hours is optimal for growth.
In summary, phytoplankton play a critical role in aquaculture by serving as a primary food source and nutritional supplement for cultured aquatic organisms. The cultivation of phytoplankton under controlled conditions ensures a reliable and sustainable food supply for a wide range of aquaculture practices, contributing to the production of seafood and aquatic products worldwide.
Ecology of Phytoplankton
The ecology of phytoplankton, the microscopic photosynthetic organisms inhabiting aquatic ecosystems, is a complex and integral component of the Earth’s biosphere. The ecological dynamics of phytoplankton encompass a wide range of processes and interactions, influencing both marine and freshwater ecosystems. Here, we delve into the key ecological aspects of phytoplankton:
- Photosynthetic Autotrophy: Phytoplankton are primary producers that harness solar energy through photosynthesis to convert carbon dioxide (CO2) and nutrients into organic matter. This fundamental autotrophic capability underpins their role as the primary energy source for aquatic food webs.
- Euphotic Zone Habitat: Phytoplankton inhabit the euphotic zone, the well-lit surface layer of oceans, seas, and lakes. This zone allows for photosynthesis to occur, ensuring access to sunlight—a crucial ecological requirement.
- Biodiversity: Phytoplankton exhibit remarkable taxonomic diversity, comprising numerous species and groups such as diatoms, dinoflagellates, cyanobacteria, and coccolithophores. This biodiversity contributes to a complex web of ecological interactions and functions.
- Resource Competition: Phytoplankton species compete for resources, primarily nutrients (e.g., nitrogen, phosphorus, and silicic acid) and light. Nutrient availability and light penetration govern their distribution and growth rates, influencing community structure.
- Seasonal and Spatial Variability: Phytoplankton populations exhibit seasonal and spatial fluctuations in response to changing environmental conditions. Factors like temperature, nutrient availability, and light levels influence the timing and magnitude of phytoplankton blooms.
- Photic Adaptations: Different phytoplankton species possess distinct photosynthetic pigments that enable them to efficiently absorb various wavelengths of underwater light. This diversity allows them to exploit different spectral compositions of light efficiently.
- Nutrient Dependence: Phytoplankton are crucially dependent on nutrients, including macronutrients (e.g., nitrogen and phosphorus) and micronutrients (e.g., iron). Nutrient availability regulates their growth, and imbalances can lead to nutrient limitation.
- Biological Pump: Phytoplankton contribute to the “biological pump” by transporting carbon from the surface ocean layers to deeper waters as they die and sink. This process facilitates carbon sequestration and influences the global carbon cycle.
- Environmental Responses: Changes in environmental conditions, such as warming due to climate change and ocean acidification, can impact phytoplankton distribution, community composition, and productivity. These responses have far-reaching consequences for marine ecosystems.
- Role in Food Webs: Phytoplankton serve as the base of aquatic food webs, providing a critical food source for zooplankton, small fish, and marine organisms. Their availability and abundance influence higher trophic levels, including commercially valuable fish species.
- El Niño-Southern Oscillation (ENSO): ENSO cycles in the Equatorial Pacific can influence phytoplankton dynamics, leading to changes in community structure, biomass, and density. These variations impact marine ecosystems and fisheries.
- Monitoring and Indicators: Phytoplankton serve as indicators of ecological condition and health in estuarine and coastal environments. Satellite ocean color observations are used to monitor changes in their distribution and abundance, aiding in ecosystem assessment.
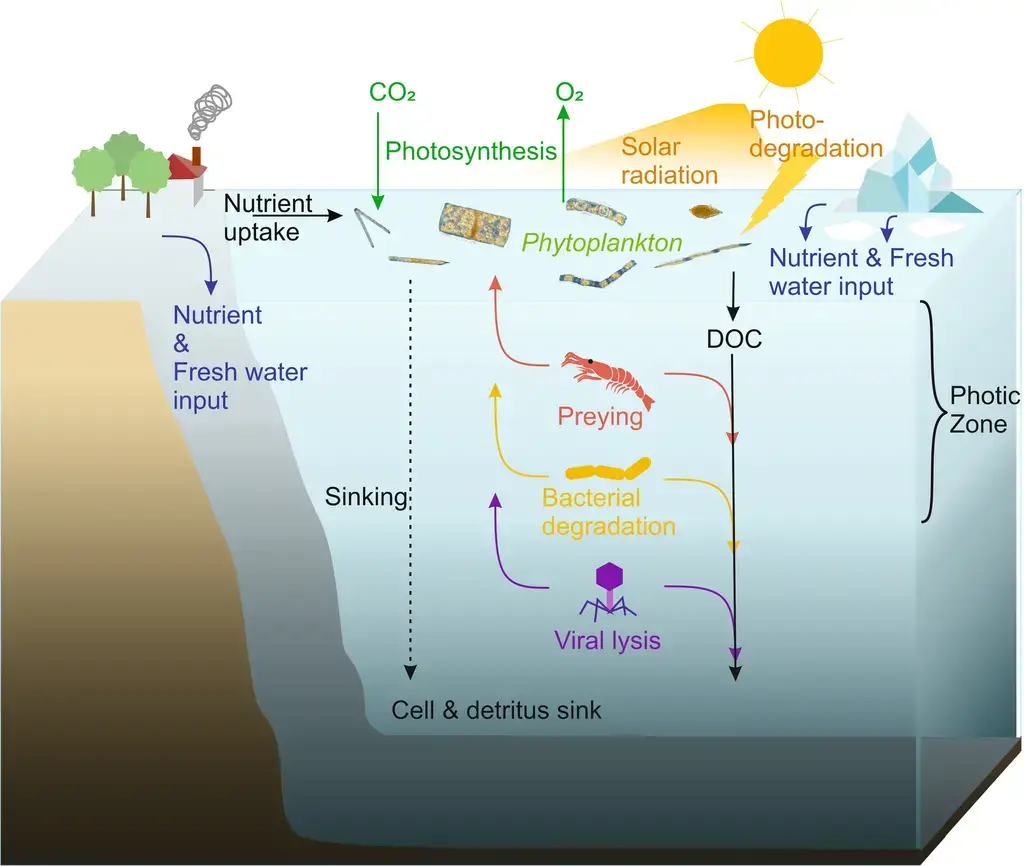
In summary, the ecology of phytoplankton is a complex interplay of ecological, physiological, and environmental factors. Their role as primary producers, their interactions with nutrient cycles, and their responses to environmental changes make them vital components of aquatic ecosystems and essential subjects of scientific study and monitoring.
Role of Planktons in Carbon Sequestration
- Phytoplankton, comprising a diverse array of microscopic photosynthetic organisms, play a pivotal role in carbon sequestration, offering a potent biological mechanism to mitigate the impacts of excess atmospheric carbon dioxide (CO2) on global climate change. Their exceptional ability to capture and store carbon underscores their significance in the carbon cycle.
- Certain species of phytoplankton possess a remarkable tolerance to elevated CO2 levels, positioning them as efficient carbon sequestration agents. Notably, diatoms, a prominent phytoplankton group, demonstrate an impressive capacity to absorb substantial quantities of CO2 from the atmosphere. Studies have revealed that diatoms alone can sequester an estimated 10 to 20 billion metric tonnes of CO2 annually, making them a critical component of the global carbon sequestration process.
- The carbon sequestration process initiated by phytoplankton is intrinsically linked to their photosynthetic activity. During photosynthesis, phytoplankton utilize atmospheric CO2 and convert it into organic carbon compounds, primarily through the fixation of carbon into biomass. In this process, carbon is effectively removed from the atmosphere and stored within the phytoplankton cells.
- Additionally, phytoplankton contribute to carbon sequestration through the so-called “biological pump.” As phytoplankton bloom and subsequently die, their sinking biomass transports carbon from the surface ocean layers to the deeper ocean, effectively sequestering it for extended periods. This process, known as the biological pump, contributes significantly to the long-term storage of carbon in the oceanic realms.
- Moreover, beyond their role in carbon sequestration, some phytoplankton species exhibit the capability to sequester pollutants, a phenomenon termed phycoremediation. Phytoplankton can absorb and metabolize various pollutants, including heavy metals and organic contaminants, facilitating their removal from aquatic ecosystems. This phycoremediation process not only enhances water quality but also underscores the multifaceted ecological services rendered by phytoplankton.
- In conclusion, phytoplankton, with their proficiency in carbon sequestration and pollution remediation, emerge as key players in mitigating global environmental challenges. Their contribution to carbon capture and storage, particularly by diatoms, offers a promising avenue for addressing the concerns associated with rising atmospheric CO2 levels and climate change. Furthermore, their role in phycoremediation underscores their importance in maintaining the health and sustainability of aquatic ecosystems.
Importance of Phytoplankton
Phytoplankton, the microscopic photosynthetic organisms inhabiting aquatic ecosystems, play a pivotal role in the natural world and are of immense importance due to their multifaceted contributions to the environment and global ecosystems. Their significance can be summarized as follows:
- Primary Producers: Phytoplankton serve as the foundation of aquatic food webs, converting sunlight, carbon dioxide, and nutrients into organic matter through photosynthesis. They provide the primary source of energy and essential nutrients for higher trophic levels, including zooplankton, fish, and marine mammals.
- Carbon Sequestration: Phytoplankton capture and store atmospheric carbon dioxide (CO2) during photosynthesis. When they die and sink to deeper ocean layers, they transport carbon to the ocean’s interior, contributing to long-term carbon sequestration. This process helps regulate global climate by reducing CO2 levels in the atmosphere.
- Oxygen Production: Phytoplankton are responsible for a significant portion of Earth’s oxygen production through photosynthesis. They generate oxygen as a byproduct of converting CO2 into organic matter, contributing substantially to atmospheric oxygen levels.
- Biogeochemical Cycling: Phytoplankton play a crucial role in nutrient cycling, particularly for elements like carbon, nitrogen, phosphorus, and silica. They recycle nutrients within aquatic ecosystems, influencing nutrient availability and supporting the overall health and productivity of these ecosystems.
- Indicator of Water Quality: Changes in phytoplankton abundance and composition can serve as indicators of water quality and ecosystem health. Monitoring phytoplankton communities helps assess the impacts of factors such as nutrient pollution, eutrophication, and climate change on aquatic environments.
- Economic Importance: Phytoplankton contribute directly to fisheries by providing food for fish and other commercially valuable species. Additionally, some species of phytoplankton are cultured for use in aquaculture as a source of nutrition for farmed aquatic organisms.
- Biochemical Resources: Phytoplankton are a source of bioactive compounds with pharmaceutical, nutritional, and industrial applications. Some species are used in the production of dietary supplements, cosmetics, and pharmaceutical drugs.
- Biodiversity: Phytoplankton encompass a wide diversity of taxonomic groups, contributing to overall marine and freshwater biodiversity. Their presence and interactions with other organisms support a rich and complex web of life in aquatic ecosystems.
- Global Climate Regulation: By influencing the Earth’s carbon and oxygen cycles, phytoplankton indirectly contribute to global climate regulation. Their role in oceanic carbon uptake and production of climate-regulating aerosols is particularly noteworthy.
- Scientific Research: Phytoplankton serve as valuable model organisms for studying fundamental ecological and physiological processes. Research on phytoplankton helps scientists better understand critical aspects of aquatic ecosystems and informs broader ecological and environmental studies.
In summary, phytoplankton are integral to the functioning of aquatic ecosystems and have far-reaching effects on the Earth’s climate, biodiversity, and ecological health. Their importance extends beyond their microscopic size, highlighting their essential role in sustaining life and maintaining the balance of our planet’s ecosystems.
Examples of Phytoplankton
Phytoplankton, a diverse assemblage of photosynthetic microorganisms comprising over 5000 recorded species, populate various aquatic environments. This diversity manifests as distinct species adapted to specific ecological niches. Presented below are examples of some extensively studied phytoplankton species:
- Coccolithophorids: Coccolithophorids are noteworthy members of the phytoplankton community, characterized by their distinctive calcium carbonate plates known as coccoliths. These microorganisms are not only vital microfossils but also play a role in the production of dimethyl sulfide (DMS). DMS is considered a potential mechanism for climate regulation, as elevated levels of coccolithophorids can lead to increased DMS production. When oxidized, DMS generates sulfur dioxide and sulfate aerosols, which act as cloud seed nuclei. This phenomenon enhances cloud coverage and sunlight reflection, affecting climate dynamics.
- Cyanobacteria: Cyanobacteria, while minuscule, are highly adaptable phytoplankton that thrive in less turbulent waters with limited nutrient availability. They exhibit remarkable diversity and resilience to changing aquatic conditions. Cyanobacterial species often outcompete other phytoplankton when water temperatures fluctuate or nutrient levels diminish.
- Diatoms: Diatoms are exceptionally significant phytoplankton due to their rapid reproductive rates, despite their microscopic size. They serve as indicators of water quality, undergoing a “bloom-and-bust” life cycle. Diatoms proliferate swiftly when nutrients reach well-illuminated ocean surfaces. However, when essential nutrients, such as silicon, become depleted, their growth ceases. Diatoms also contribute substantially to the organic matter present in the sediment of large bodies of water.
- Dinoflagellates: Dinoflagellates play a crucial role in supporting coral reef ecosystems by serving as a primary food source for many species. These phytoplankton can form harmful algal blooms characterized by a distinctive red color, commonly referred to as “red tide.”
It is essential to recognize that these examples represent a fraction of the vast diversity within the phytoplankton realm. Each species exhibits unique ecological adaptations and responses to environmental conditions, contributing to the intricate dynamics of aquatic ecosystems. Additionally, phytoplankton’s impact extends beyond their microscopic dimensions, influencing marine food webs, biogeochemical cycles, and even global climate processes.
Role of phytoplankton
The role of phytoplankton in marine ecosystems is of paramount importance, as these microscopic photosynthetic organisms serve as the foundation of aquatic food webs and play a crucial role in biogeochemical cycles. In this scientific discussion, we explore the multifaceted roles of phytoplankton:
- Primary Producers: Phytoplankton, through the process of photosynthesis, convert solar energy into organic carbon compounds. They are autonomous primary producers, forming the base of the marine food web. Their ability to synthesize organic matter from inorganic carbon dioxide is fundamental to the transfer of energy and nutrients in aquatic ecosystems.
- Carbon Cycling: Phytoplankton play a central role in the global carbon cycle. They fix atmospheric carbon dioxide into organic carbon compounds, effectively removing CO2 from the atmosphere. This photosynthetically fixed carbon is rapidly recycled within the surface ocean, contributing to the organic matter pool and influencing atmospheric gas composition.
- Nutrient Recycling: Phytoplankton contribute to the recycling of essential nutrients, including nitrogen, phosphorus, and trace elements. They take up these nutrients from the surrounding water and incorporate them into their biomass. When phytoplankton die or are grazed upon, these nutrients are released back into the ecosystem, sustaining the nutrient balance.
- Marine Food Web: Phytoplankton are the primary energy source for higher trophic levels in marine ecosystems. Zooplankton, small fish, and other organisms feed on phytoplankton, initiating energy transfer through the food web. This trophic cascade continues up to apex predators, maintaining ecosystem structure and function.
- Microbial Loop: Phytoplankton contribute to the microbial loop, a critical component of nutrient cycling in marine environments. When phytoplankton release organic matter, it becomes available to heterotrophic bacteria. These bacteria play a pivotal role in remineralizing organic compounds back into inorganic nutrients, ensuring their availability for phytoplankton and other organisms.
- Export of Organic Matter: While much of the organic matter produced by phytoplankton is recycled within the surface ocean, a fraction of it sinks to the deep ocean as particulate organic carbon. This export of organic matter contributes to carbon sequestration in the deep ocean, affecting the long-term carbon balance of the planet.
- Harmful Algal Blooms (HABs): Under specific conditions, phytoplankton can undergo rapid population growth, leading to the formation of harmful algal blooms (HABs). These blooms can produce toxins harmful to marine life and humans, disrupt ecosystems, and impact fisheries and aquaculture.
In summary, phytoplankton serve as the primary drivers of marine ecosystems, influencing nutrient cycling, carbon sequestration, and the structure of aquatic food webs. Their pivotal role in biogeochemical processes underscores their significance in regulating Earth’s climate and supporting life in the oceans. However, their capacity to form harmful algal blooms also highlights the need for monitoring and understanding the complex dynamics of phytoplankton communities in a changing environment.
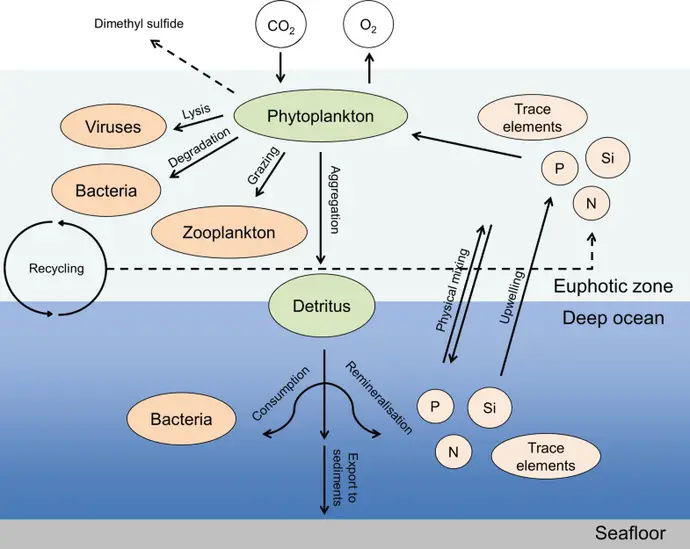
Quiz
What is the primary source of energy for phytoplankton?
A. Geothermal heat
B. Chemical reactions
C. Photosynthesis
D. Hydrothermal vents
Which of the following is a characteristic feature of diatoms?
A. Calcium carbonate shell
B. Two flagella for locomotion
C. Ovate or elongated shape
D. Phycocyanin pigment
What is the main pigment responsible for the blue color of blue-green algae (cyanobacteria)?
A. Chlorophyll
B. Xanthophyll
C. Phycocyanin
D. Carotenoid
Where are phytoplankton typically found within aquatic environments?
A. In deep, dark waters
B. In the hypolimnion of lakes
C. In the well-lit surface layers
D. Near hydrothermal vents
What is the size range for nanoplankton?
A. Less than 2 µm
B. 2 to 20 µm
C. 2 to 200 µm
D. More than 2000 µm
Phytoplankton that inhabit waters overlying continental shelves are referred to as:
A. Oceanic phytoplankton
B. Neritic phytoplankton
C. Brackish water phytoplankton
D. Estuarine phytoplankton
How do phytoplankton obtain energy for survival?
A. By consuming other microorganisms
B. By absorbing dissolved organic matter
C. By feeding on detritus
D. Through photosynthesis
What is the primary role of phytoplankton in marine ecosystems?
A. Top predator
B. Decomposers of organic matter
C. Filter feeders
D. Base of the food chain
Which environmental factor is essential for phytoplankton growth and influences their stoichiometry?
A. Wind speed
B. Atmospheric pressure
C. Nutrient concentrations
D. Geomagnetic field
Phytoplankton play a vital role in carbon sequestration. Which type of phytoplankton is known for its high tolerance to CO2 absorption?
A. Dinoflagellates
B. Diatoms
C. Coccolithophores
D. Blue-green algae
FAQ
What is phytoplankton?
Phytoplankton are microscopic, photosynthetic organisms that float in aquatic environments. They are a crucial component of aquatic food webs and play a significant role in Earth’s ecosystems.
Where are phytoplankton found?
Phytoplankton can be found in various aquatic environments, including oceans, seas, lakes, rivers, and even some brackish water areas like estuaries.
How do phytoplankton obtain energy?
Phytoplankton obtain energy through photosynthesis, using sunlight to convert carbon dioxide and nutrients into organic compounds.
What are the main types of phytoplankton?
The main types of phytoplankton include diatoms, dinoflagellates, blue-green algae (cyanobacteria), green algae, and coccolithophores.
What is the ecological importance of phytoplankton?
Phytoplankton serve as the foundation of aquatic food webs, providing energy and nutrients to various marine and freshwater organisms. They also play a role in carbon sequestration and oxygen production.
How do phytoplankton affect climate?
Some phytoplankton release dimethyl sulfide (DMS), which can influence cloud formation and, in turn, affect Earth’s climate.
What factors influence phytoplankton abundance?
Factors affecting phytoplankton abundance include temperature, light availability, nutrient concentrations, and water stratification, among others.
Are phytoplankton affected by climate change?
Yes, climate change can impact phytoplankton communities by altering ocean temperatures, circulation patterns, and nutrient availability.
How are phytoplankton used in aquaculture?
Phytoplankton are used as food for cultured aquatic organisms in aquaculture and mariculture, helping to support the growth of various species.
What is the role of phytoplankton in carbon sequestration?
Phytoplankton can absorb carbon dioxide (CO2) from the atmosphere during photosynthesis, contributing to carbon sequestration in the oceans.
References
- Tandon et al. (2017). A promising approach to enhance microalgae productivity by exogenous supply of vitamins. Microb Cell Fact. 16(1):219.
- Benoiston et al. (2017). The evolution of diatoms and their biogeochemical functions. Philos Trans R Soc Lond B Biol Sci.372(1728):pii: 20160397.
- Helliwell, KE. (2017). The roles of B vitamins in phytoplankton nutrition: new perspectives and prospects. New Phytol. 216(1): 62-68.
- Lee et al. (2017). The role of algae and cyanobacteria in the production and release of odorants in water. Environ Pollut. 227: 252-262.