What is Membrane Protein?
Membrane proteins are proteins that are embedded within or attached to the lipid bilayer of a cell membrane. These proteins play crucial roles in various cellular functions, including cell signaling, transport of molecules, and structural support.
- Membrane proteins are proteins that are part of biological membranes or interact with them. Depending on their location, several broad categories of membrane proteins exist.
- Integral membrane proteins are a permanent component of a cell membrane and can either traverse the membrane (transmembrane) or associate with one side or the other (integral monotopic). Occasionally, peripheral membrane proteins are associated with the cell membrane.
- About one-third of all human proteins are membrane proteins, and more than half of all pharmaceuticals target these proteins.
- Nevertheless, compared to other classes of proteins, determining membrane protein structures remains difficult due in large part to the difficulty of establishing experimental conditions that can preserve the correct conformation of the protein when it is isolated from its native environment.
Location of Membrane Proteins
Membrane proteins are connected with the cell membrane, the lipid bilayer that separates the cytoplasm from the rest of the cell and the outside world. Depending on their structure and function, membrane proteins can occupy a variety of different spots within the cell membrane.
Integral membrane proteins are categorized further according to their location within the membrane’s lipid bilayer.
- Transmembrane proteins: Transmembrane proteins are proteins that traverse the entire membrane, with domains reaching into both the cytoplasm and the extracellular space.
- Monolayer-associated proteins: Monolayer-associated proteins are proteins that are only partially incorporated into the membrane and interact with the lipid bilayer at a single site.
Proteins of the peripheral membrane are not directly coupled with the lipid bilayer but are instead linked to it via weak, non-covalent contacts. It’s possible to locate them on the cytoplasmic or extracellular side of the membrane.
It’s possible that certain membrane proteins are enriched in one area of the membrane over another. On the membrane, receptor proteins tend to congregate in structures called lipid rafts, which contain a higher concentration of lipids like cholesterol and sphingolipids.
The nuclear membrane, the mitochondrial membrane, and the endoplasmic reticulum membrane all contain membrane proteins. Although they perform comparable roles to membrane proteins, the proteins in these organelles may have different structures or changes to better perform their designated tasks.
In conclusion, membrane proteins are connected with the cell membrane and localize there depending on their structure and function. It is possible that some membrane proteins are located in additional cellular membranes or are localized to distinct areas of the membrane.
What Are Cellular Membranes Made Of?
- There are lipids and proteins in every cellular membrane. Phospholipids, the main type of lipid in cell membranes, have a hydrophobic (water-repelling) tail and a hydrophilic (water-attracting) head. The hydrophilic ends of these phospholipids face the aqueous environments on each side of the membrane, while the hydrophobic ends of the phospholipids face each other within the bilayer.
- Other forms of lipids, such as cholesterol, may also be present in cellular membranes and contribute to the regulation of membrane fluidity and stability. Glycolipids are lipids with carbohydrate chains attached; these lipids are involved in cell identification and signaling.
- Proteins also play a crucial role in the structure and function of cellular membranes. There are two types of membrane proteins: those that are either directly linked with the membrane surface or those that are embedded within the lipid bilayer (integral proteins) (peripheral proteins). Transport, enzymatic activity, signal transduction, and cell adhesion are just some of the many roles that these proteins can play.
- Carbohydrates and other tiny molecules may also be present in cellular membranes in addition to lipids and proteins. Examples include the glycocalyx, an outer layer of carbohydrate chains on certain membranes that aids in cell identification and signaling.
- Lipids, proteins, and other components all play important roles in maintaining the membrane’s shape and function, making the composition of cellular membranes dynamic and complex.
Types of Membrane Proteins
There are present different types of membrane proteins such as;
- Integral membrane proteins
- Peripheral membrane proteins
- Lipid-Bound Proteins
- Amphitropic Proteins
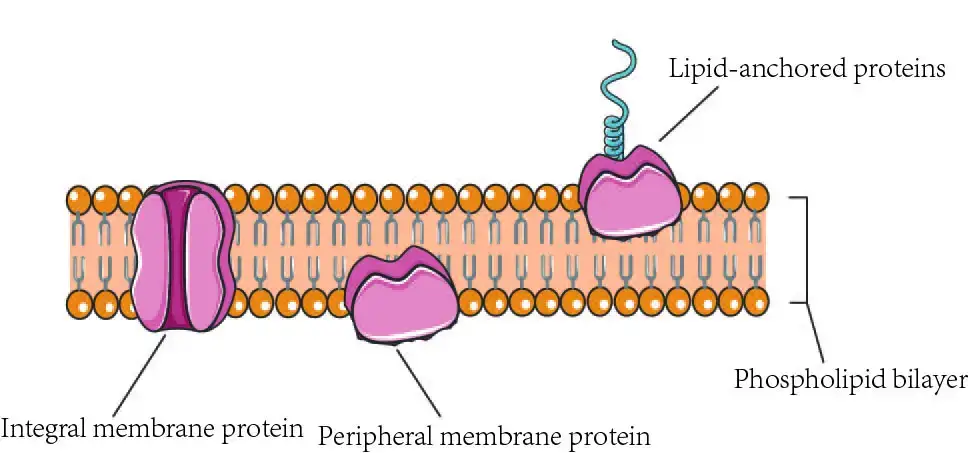
Peripheral membrane proteins
- Electrostatic and hydrogen bonding hold peripheral proteins to the membrane surface largely. They can be split into two fundamental types: those attached to the charged surface of integral proteins and those associated to the anionic head groups of phospholipids.
- By adjusting the pH or the salt concentration of the medium, it is simple and clean to remove peripheral proteins from the membrane surface. The release of peripheral proteins with alkaline carbonate is a common technique that is frequently employed.
- If the peripheral protein is linked to the membrane via Ca2+, a chelating agent will release it. A real peripheral protein interacts minimally or not at all with the hydrocarbon interior of the membrane and is released from the membrane without lipids.
- Some well-known examples of peripheral proteins can also be categorized as amphitropic proteins. Cytochrome c (which binds to an integral protein) and myelin basic protein (MBP) are classic examples of peripheral proteins (binds to membrane phospholipids).
- Cytochrome c is likely the most thoroughly researched peripheral protein. Decades of research have established its critical role in the mitochondrial electron transport system, where it connects Complex III to Complex IV.
- More recently, it was shown that this very little, primitive protein is an example of a “moonlighting” protein, performing a completely different function, that of triggering apoptosis.
- Cytochrome c is poorly linked to cytochrome c oxidase on the outer side of the inner mitochondrial membrane (intermembrane gap). With 0.15 M KCl, cytochrome c may be separated from the mitochondrial membrane.
- In fact, the protein is frequently lost during normal mitochondrial isolation and must be replenished. The simple loss of cytochrome c is what makes this peripheral protein such an effective apoptotic trigger.
- MBP is the greatest example of a peripheral protein that primarily binds to anionic phospholipids on the surface of a membrane via electrostatic forces. The myelin sheath is regarded as the membrane with the lowest degree of dynamism, and so contains very few proteins.
- The key myelin proteins, myelin proteolipid protein, MBPs, and myelin-associated glycoprotein, likely serve a structural function.
- MBP is involved in the myelination of central nervous system nerves, and abnormalities in this protein are thought to play a significant role in multiple sclerosis.
Amphitropic Proteins
- Amphitropic proteins are a relatively novel protein class whose significance is becoming increasingly more evident. The peripheral proteins that are coupled to membrane surface phospholipids, integral ecto- and endo-proteins, and amphitropic proteins have a great deal of homology.
- Combining electrostatic and hydrophobic forces, amphitropic proteins can alternate between conformations that are either water-soluble or weakly attached to the membrane surface. Due to the reversible nature of the membrane connection, an amphitropic protein coexists as both a free globular protein and a membrane-bound protein.
- Following a conformational shift triggered by phosphorylation, acylation, or ligand interaction that exposes a previously inaccessible membrane binding site on the protein, the water-soluble globular form is transformed into the membrane-bound form.
- Frequently, membrane interaction is based on the creation of a hydrophobic loop of amino acids, or sometimes an a-helix, which can permeate the lipid bilayer.
- Many essential amphitropic proteins are integrally involved in cell signaling processes. Src kinase, protein kinase C, and phospholipase C are other examples.
- Following contact with the right substrate, the shape of the globular form of the amphitropic exzyme is altered, exposing a hydrophobic section of the protein that enters into the membrane and associates closely with its membrane target.
- In Escherichia coli, for instance, globular pyruvate oxidase binds to its substrate pyruvate and cofactor thiamine pyrophosphate, therefore revealing a hydrophobic helix within the protein.
- After binding to the membrane, the modified enzyme transfers electrons from the cytoplasm to the membrane’s electron transport chain.
- In addition to water-soluble channel-forming polypeptide toxins, the category of amphitropic proteins contains water-soluble channel-forming polypeptides (eg, colicin A and a-hemolysin).
Integral Proteins
- 20–30% of all cellular proteins are integral, and these proteins are firmly and permanently linked to the membrane. Whereas peripheral and amphitropic proteins are weakly attached to the surface of membranes and can be easily dissociated to produce lipid-free globular proteins, integral proteins are incorporated into the hydrophobic center of the membrane and can only be removed by more extreme means.
- In the past, integral proteins were extracted from membranes using acetone and other nonpolar organic solvents, chaotropic agents, or detergents. In the early attempts to isolate integral proteins, membrane lipids were removed by dissolving them with ice-cold acetone.
- Proteins form a white powder known as “acetone powder” when they precipitate. Obviously, the proteins have been denatured to the point that they are almost useless for biochemical study.
- Using chaotropic chemicals to successfully isolate some still-functional integral proteins. Chaotropic agents act by altering the structure of water, hence nullifying the Hydrophobic Effect, the primary force supporting membranes.
- Although less dangerous than acetone, chaotropic substances can also denature integral membrane proteins. Chaotropic agents are highly water-soluble solutes that destabilize membranes by removing all of the membrane’s water. 6 – 8 M urea is the best example of a chaotropic agent.
- High quantities of guanidinium chloride, lithium perchlorate, and thiocyanate are more instances. Currently, the most effective method for separating integral proteins uses detergents. This extensive issue is explored in Chapter 13, which describes the restoration of proteins in membranes.
- Although many of globular proteins have been crystallized and their three-dimensional structures precisely determined by crystallography, only a few of integral membrane proteins have been resolved.
- The difficulty in getting 3-D structures for integral proteins resides in producing pure crystals. Integral proteins are extracted as aggregates with lipids (and/or detergents) remaining attached, whereas peripheral proteins are obtained as monomers free of membrane lipids.
- The aggregates are extremely difficult to crystallize. Hence, structural information on integral proteins is frequently obtained by indirect approaches such as enzyme degradation studies and hydropathy plots.
Types of Integral Proteins
They are categorized based to their link to the bilayer:
- Integral polytopic proteins: Integral polytopic proteins are transmembrane proteins with multiple membrane crossings. The transmembrane topology of these proteins may differ. These proteins have one of the following structural structures:
- Helix bundle proteins: Helix bundle proteins, which are present in all biological membranes;
- Beta barrel proteins: Beta barrel proteins, which are exclusive to the outer membranes of Gram-negative bacteria, mitochondria, and chloroplasts.
- Bitopic proteins: Bitopic proteins are transmembrane proteins with a single membrane crossing. These proteins have transmembrane helices with significantly altered amino acid distributions compared to polytopic proteins.
- Integral monotopic proteins: Integral monotopic proteins are integral membrane proteins that are connected to only one side of the membrane and do not extend across the entire membrane.
Lipid-Bound Proteins
- Proteins with lipid anchors constitute a second classification. Yet, many of these proteins are transmembrane proteins with extra lipid-anchors.
- They could either be classed as integral proteins or lipid-anchored proteins. Rhodopsin is the quintessential example of a seven a-helix membrane-spanning protein, but it is also covalently connected to the membrane via myristic acid.
- This protein’s classification is arbitrarily determined. Myristic acid, palmitic acid, prenylated hydrophobic acyl chains, and proteins connected through glycosylphosphatidylinositols (GPI) are the most important examples of lipid-anchors.
- Some proteins are lipid-anchored to the endocytoplasmic (inner leaflet) surface of cells. Due to the fact that lipid-anchors on their own are relatively fragile, these proteins are frequently further anchored by other common interactions (electrostatic and hydrophobic).
1. Myristoylated Lipid-Anchored Proteins
- Myristic acid (14:0), a saturated fatty acid with 14 carbons, is frequently used as a lipid anchor for proteins. Myristic acid is present in membranes at extremely low concentrations compared to palmitic acid (16:0), the second extensively used fatty acid lipid membrane anchor.
- Myristoylation of calcineurin B and the catalytic component of cyclic AMP-dependent protein kinase was originally observed in 1982. Myristic acid was discovered to be acylated to an N-terminal glycine via an amide bond to the anchoring protein.
- This connection is exclusive to eukaryotic and viral proteins and is formed early during translation. Often, it is an irreversible change of the protein. Electrostatic interactions between positively charged protein side chains and negatively charged membrane phospholipids further attach myristoylated proteins.
- Certain N-myristoylated proteins receive further fatty acyl modifications via attachment of palmitoyl groups to cysteines via reversible thioesters.
- Tyrosine kinases belonging to the Src family are an example of dually acylated proteins (eg, Fyn, Lck). N-Myristoylated proteins have multiple crucial functions, including participation in multiple signal transduction pathways.
- Several additional fatty acids, such as shorter-chain and unsaturated acids, can be linked to the N-terminal glycine under exceptional conditions where myristic acid is scarce.
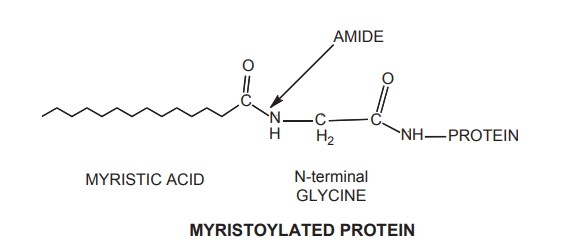
2. Palmitoylated Lipid-Anchored Proteins
- Palmitoylated (16:0) proteins have been the subject of the greatest research among lipid-anchored proteins. Braun and Rohn identified the first member of the palmitoylated family in the bacterial cell wall of E. coli in 1969.
- Subsequently, palmitoylation of the myelin proteolipid protein was discovered (PLP). PLP is the predominant protein in the myelin sheath, constituting roughly 40% of membrane proteins.
- PLP is a transmembrane protein containing four a-helices and six cysteine residues that are believed to be acylated predominantly with palmitic acid.
- Stearic acid and oleic acid are involved in less acylations. Acylation of a fatty acid to the sulphur side chain of cysteine results in the creation of a thioester in a reversible manner. In fact, the fact that this lipid connection is a reversible fatty acid attachment is perhaps its most crucial characteristic.
- Reversibility contrasts starkly with myristoylation and prenylation. Using acyltransferases and thioesterases, respectively, easy palmitoylation and depalmitoylation have the ability to modulate enzyme activity.
- This strongly resembles the well-known enzyme regulation mechanisms including phosphorylation by kinases and dephosphorylation by phosphatases.
- The ever-expanding family of palmitoylated proteins includes rhodopsin, band three of erythrocytes, and lung surfactant proteolipid.
- Palmitic acid has been observed to be O-acylated to serines and threonines as well as N-acylated to the -amine side chain of lysines. According to reports, palmitic acid is linked to an N-terminal cysteine in the signalling pathway protein Sonic Hedgehog via an amide bond.
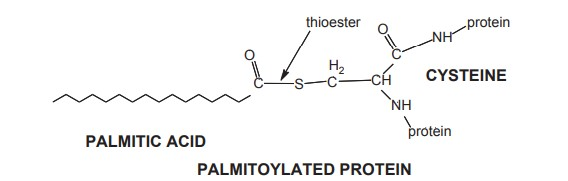
3. Prenylated Lipid-Anchored Proteins
- Integral membrane proteins can also be lipid-anchored via irreversible thioether bonds to extremely hydrophobic isoprene groups. Hence, these proteins are known as prenylated proteins.
- In 1978, Kamiya et al. As described in Chapter 5, prenylated groups are branching 5-carbon repeating units of 3-methyl-2-buten-1-yl and receive their name from isoprene. Despite the fact that it may appear that the primary function of these prenylated chains is to glue the associated protein to the hydrophobic core of membranes, these groups play an additional, very distinct, and crucial role in membranes.
- It has been demonstrated that prenyl groups are essential for protein-protein binding via specialised prenylbinding domains. Consequently, prenylated groups are both lipid-anchors and intimately involved in the localization of particular proteins to particular domains or proteins inside the membrane.
- Membrane prenylation involves either 15-carbon (3 isoprene units) farnesyl or 20-carbon (4 isoprene units) geranylgeranyl covalently linked to cysteine residues at or near the C-terminus of proteins by a thioether.
- Once created, thioethers are considered permanent attachments since they cannot be easily separated. Prenylation, like myristoylation and palmitoylation, attaches the protein to the cytoplasmic (inside leaflet) side of the membrane.
- Protein kinases, nuclear lamins, Ras and Ras-associated G proteins are examples of prenylated proteins.
- Given that prenylation is crucial for the function of numerous proteins involved in cellular signalling and trafficking pathways, the process is becoming an important therapeutic target for a variety of disorders.
- There are already over 20,000 known prenylated proteins, and this number grows constantly. Thus, it is plausible to suppose that many additional prenylated proteins remain to be found.
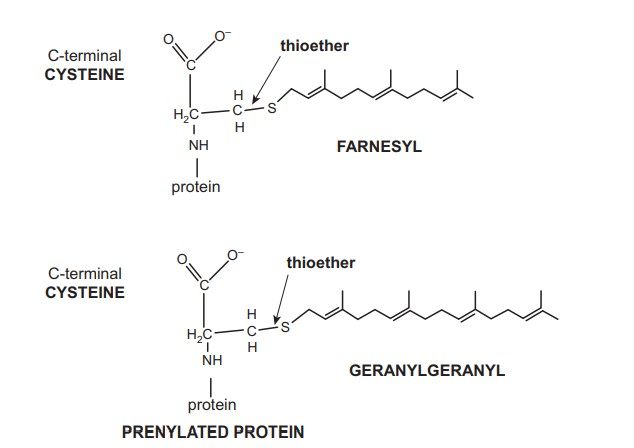
4. Glycosylphosphatidylinositol-Anchored Proteins
- Whereas myristoylation, palmitoylation, and prenylation bind proteins to the cytoplasmic side (inside) of membranes, the acyl chains of GPI anchor some extracellular proteins to the outer surface of cells.
- Ikazawa and Low independently identified GPI-anchored proteins about the same time in 1976. GPI-anchored proteins are not transmembrane and alkaline carbonate cannot remove them. Thus, they are not peripheral proteins by definition.
- Its primary membrane anchor appears to be the acyl chains of phosphatidylinositol (PI), which would be weak without additional electrostatic or hydrophobic contacts. GPI-anchored proteins generally cluster and only face the exterior.
- The C-terminus of the anchored protein is indirectly attached to the membrane via a phosphoethanolamine, a glycan (three mannose sugars), a glucosamine, and lastly an inositol sugar of PI. GPI-anchored proteins are prevalent in eukaryotes but absent in bacteria.
- There are around 45 GPI-anchored proteins in humans, despite the fact that GPIanchors are not a frequent motif of membrane protein structure. In yeast, where all GPI proteins are known, only 15 of the 5790 proteins (about one in 400) include GPI-anchors.
- Many receptor-mediated signal transduction pathways are dependent on GPI-anchored proteins for their enzymatic, antigenic, adhesion, and membrane-organizing properties.
- Carbonic anhydrase, alkaline phosphatase, Thy-1, and BP-3 are examples of well-known GPI-anchored proteins. The GPI-anchored protein can be released from the membrane following hydrolysis by phospholipase C, and it was found in this way.
- GPI proteins have been associated to a number of human genetic illnesses, the majority of which are uncommon. Possibly the most well-known is Marfan Syndrome, whose most famous carrier, according to a 1962 theory, was Abraham Lincoln.
- This intriguing narrative is no longer believed, despite having gotten a tremendous lot of attention. Lincoln most likely had multiple endocrine neoplasia type 2B, which has skeletal characteristics nearly comparable to those of Marfan Syndrome.
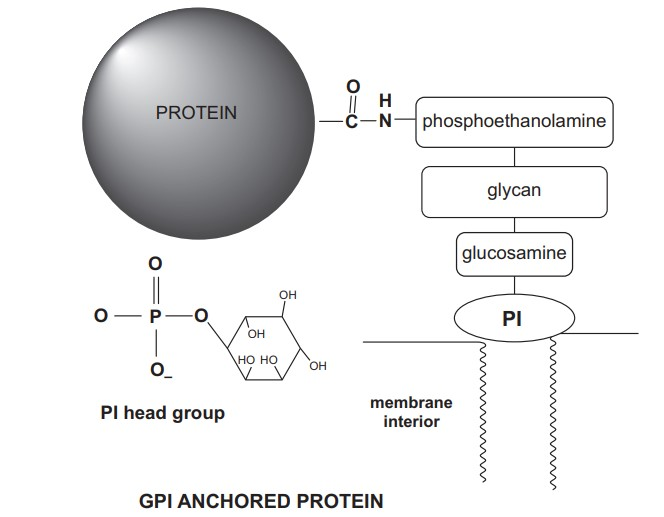
How are membrane proteins studied?
Membrane proteins are studied using a variety of experimental techniques. Here are a few examples:
- X-ray crystallography: This technique is used to determine the three-dimensional structure of membrane proteins at atomic resolution. Membrane proteins are purified and crystallized, and then X-ray diffraction patterns are collected from the crystals to determine the protein structure.
- NMR spectroscopy: Nuclear magnetic resonance (NMR) spectroscopy is used to determine the structure and dynamics of membrane proteins in solution. This technique can provide information about the protein’s interactions with other molecules, its flexibility, and its conformational changes.
- Electron microscopy: Electron microscopy is used to visualize the structure of membrane proteins in their native membrane environment. This technique can reveal the organization of proteins within the membrane and their interactions with other molecules.
- Fluorescence microscopy: Fluorescence microscopy is used to study the localization and dynamics of membrane proteins within cells. This technique can provide information about the protein’s mobility, interactions with other proteins, and its role in cell signaling.
- Proteomics: Proteomics is used to identify and quantify the proteins present in a sample. This technique can be used to study the expression levels and post-translational modifications of membrane proteins.
Overall, the study of membrane proteins requires a combination of biochemical, biophysical, and imaging techniques to fully understand their structure, function, and interactions within the cell.
Features of Membrane Proteins
Membrane proteins come in a wide variety of shapes and sizes and perform a wide range of tasks, but they always rely on a few key characteristics. Some distinguishing characteristics of membrane proteins are as follows.
- Transmembrane Domains: Transmembrane domains are segments of a protein that extend the full length of the lipid bilayer. Hydrophobic amino acid residues are a common feature of these domains, and they mediate interactions with the hydrophobic tails of membrane phospholipids.
- Hydrophobic and Hydrophilic Regions: Membrane proteins contain both hydrophobic and hydrophilic domains, allowing them to communicate with the lipid bilayer and the aqueous milieu on either side.
- Selectivity: A common characteristic of membrane proteins is their ability to regulate the passage of certain molecules or ions. It is the protein’s unique structure and charge that usually accounts for its selectivity.
- Receptor Sites: Areas on membrane proteins called receptors are activated when these proteins engage their respective target molecules or ions. Most of the time, the molecule or ion being detected by a receptor has a binding site on the receptor that is structurally or chemically compatible to the site.
- Conformational Changes: When a membrane protein binds a specific molecule or ion, it often undergoes a conformational change that either initiates a biological reaction or allows the protein to transport the bound molecule across the membrane.
- Anchoring: A variety of mechanisms, including interactions with other proteins and attachment to lipid molecules in the membrane, are available for anchoring membrane proteins to the cell membrane.
- Glycosylation: Carbohydrate chains are bound to several membrane proteins through a process called glycosylation. This alteration can serve as a safeguard against proteolysis and as a signal to downstream proteins in the cell.
Transmembrane domains, hydrophobic and hydrophilic areas, selectivity, receptor sites, conformational changes, anchoring, and glycosylation are all properties of membrane proteins that are crucial to their functions in the cell membrane. Proteins found in membranes are able to transport molecules, send signals, provide structural support, and catalyze reactions because of these properties.
Functions of Membrane Proteins
Membrane proteins are involved in many processes that are crucial to the health of a cell. Some of the primary roles that membrane proteins play are as follows:
- Transport: Several membrane proteins have the ability to transport molecules or ions across the membrane. In other words, these proteins can be selective, letting through only the molecules or ions of your choosing.
- Enzymatic activity: Certain membrane proteins are enzymes, which catalyze chemical reactions on or near the membrane. Enzymes in charge of lipid and carbohydrate biosynthesis and catabolism are just a couple examples.
- Signal transduction: When signaling molecules like hormones or neurotransmitters attach to specific receptors on membranes, this triggers a cellular response through a process called signal transduction. Alterations in the function of other membrane proteins, activation of intracellular signaling pathways, and changes in gene expression are all possible outcomes of this response.
- Cell adhesion: Cell adhesion is the process by which cells adhere to one another or to the extracellular matrix, and membrane proteins can play a part in this process. Tight junctions and desmosomes are two examples of cell-cell junctions that may involve these proteins.
- Structural support: Membrane proteins play a crucial role in the structural integrity of the cell membrane, ensuring that it retains its proper shape and integrity. Proteins that help connect the cytoskeleton to membranes are one such category.
- Ion channels: Ion channels are formed when membrane proteins open up and let ions pass through. The permeability of these channels to ions can be controlled in a number of ways, making them selective.
- Recognition and binding: Membrane proteins have the ability to identify and bind to foreign molecules or ions, like antibodies or antigens. The immune system relies on these interactions, and they also help distinguish between self and non-self molecules.
In conclusion, membrane proteins are crucial to a cell’s health and survival in many different ways. The transport, enzyme activity, signal transduction, cell adhesion, structural support, ion channels, and recognition and binding are all examples of these roles.
FAQ
What are membrane proteins?
Membrane proteins are proteins that are embedded in, or attached to, the cell membrane. They play a crucial role in the functions of the cell membrane, such as cell signaling, transport of molecules, and cell-cell communication.
What is the structure of membrane proteins?
Membrane proteins have a diverse range of structures, but they are generally composed of hydrophobic and hydrophilic regions. The hydrophobic regions interact with the lipid bilayer of the membrane, while the hydrophilic regions face the extracellular or intracellular environment.
How are membrane proteins classified?
Membrane proteins are classified based on their location within the cell membrane and their function. They can be classified as integral membrane proteins, peripheral membrane proteins, transmembrane proteins, or lipid-anchored proteins.
What is the role of integral membrane proteins?
Integral membrane proteins are embedded within the cell membrane and play a crucial role in transport of molecules, cell signaling, and cell adhesion.
What is the role of peripheral membrane proteins?
Peripheral membrane proteins are located on the surface of the cell membrane and are involved in cell signaling, cell adhesion, and enzymatic activity.
What are transmembrane proteins?
Transmembrane proteins span the entire thickness of the cell membrane and are involved in transport of molecules, cell signaling, and cell adhesion.
How do membrane proteins transport molecules across the cell membrane?
Membrane proteins use various mechanisms to transport molecules across the cell membrane, including passive transport, active transport, facilitated diffusion, and osmosis.
What is the significance of membrane proteins in disease?
Membrane proteins are involved in many disease processes, including cancer, cystic fibrosis, and Alzheimer’s disease. Understanding the structure and function of membrane proteins can aid in the development of new treatments for these diseases.
References
- Alberts B, Johnson A, Lewis J, et al. Molecular Biology of the Cell. 4th edition. New York: Garland Science; 2002. Membrane Proteins. Available from: https://www.ncbi.nlm.nih.gov/books/NBK26878/
- Membrane Proteins. (2021, March 6). https://bio.libretexts.org/@go/page/6442
- Yeagle, P. L. (2016). Membrane Proteins. The Membranes of Cells, 219–268. doi:10.1016/b978-0-12-800047-2.00010-3
- Stillwell, W. (2016). Membrane Proteins. An Introduction to Biological Membranes, 89–110. doi:10.1016/b978-0-444-63772-7.00006-3
- https://www.biologyonline.com/dictionary/membrane-protein
- https://flexbooks.ck12.org/cbook/ck-12-biology-flexbook-2.0/section/2.4/primary/lesson/membrane-proteins-bio/
- https://flexbooks.ck12.org/cbook/ck-12-biology-flexbook-2.0/section/2.4/primary/lesson/membrane-proteins-bio/
- https://www.studocu.com/en-us/document/simon-fraser-university/cellular-biology-and-biochemistry/lecture-notes-13-biomembrane-proteins/163761
- https://www.sparknotes.com/biology/cellstructure/cellmembranes/section2/
- https://www.nature.com/scitable/topicpage/cell-membranes-14052567/
- https://www.creative-biolabs.com/blog/index.php/membrane-protein-overview/
- https://ib.bioninja.com.au/standard-level/topic-1-cell-biology/13-membrane-structure/membrane-proteins.html
Hi, some of the pages of write are either Missing or hiding.Also diagrams are not included.Why?
Hi, thank you for informing us. Can you give us links of those pages?, i may be due to we are using some sensor content filter feature, that’s why some words are appearing as **