What is X-Ray Spectroscopy?
- X-ray spectroscopy is a powerful scientific technique that allows researchers to investigate the properties and composition of materials by utilizing X-ray radiation. It encompasses a range of spectroscopic methods that utilize the excitation of X-rays to gain insights into the atomic and molecular structure of substances.
- The discovery of X-rays by Wilhelm Conrad Rontgen in 1895 marked a groundbreaking advancement in the field of physics. It didn’t take long for other scientists and medical professionals to recognize the potential of this new technology. Charles Barkla, a British physicist, conducted extensive research between 1906 and 1908, which revealed that X-rays could be utilized as a distinct tool for identifying specific substances. His contributions to the field earned him the Nobel Prize in physics in 1917.
- However, it was the work of the father and son duo, William Henry Bragg and William Lawrence Bragg, that laid the foundation for the application of X-ray spectroscopy. In 1912, the Braggs used spectroscopy to study how X-ray radiation interacted with crystal atoms, leading to the development of a method known as X-ray crystallography. This technique became widely adopted in the field and proved instrumental in determining the atomic and molecular structures of various materials. In recognition of their significant contributions, the Braggs were awarded the Nobel Prize in physics in 1915.
- X-rays themselves are a form of electromagnetic radiation, characterized by wavelengths ranging from 0.01 to 10 nanometers. This corresponds to frequencies in the range of 30 petahertz to 30 exahertz (3×10^16 Hz to 3×10^19 Hz) and energies typically between 100 electron volts (eV) to 100 kiloelectron volts (keV). X-rays are generated through the deceleration and acceleration of high-energy electrons.
- X-ray spectroscopy encompasses various methods that leverage the excitation of X-rays to probe the composition and properties of materials. These techniques include X-ray fluorescence spectroscopy, X-ray absorption spectroscopy, and X-ray photoelectron spectroscopy, among others. Each method utilizes different principles to analyze the interaction between X-rays and matter and provide valuable information about the atomic and electronic structure, chemical composition, and bonding characteristics of materials.
- In X-ray fluorescence spectroscopy, X-rays are used to excite the inner-shell electrons of atoms in a sample, causing them to emit characteristic fluorescent X-rays when they return to their ground state. By analyzing the energy and intensity of these emitted X-rays, researchers can identify the elements present in the sample and determine their concentrations.
- X-ray absorption spectroscopy involves measuring the absorption of X-rays by a material as a function of energy. This technique provides information about the electronic structure and chemical environment of the atoms in the sample. By analyzing the energy dependence of the absorption, scientists can gain insights into the oxidation states, coordination geometry, and local bonding environment of specific elements.
- X-ray photoelectron spectroscopy utilizes the photoelectric effect to investigate the electronic structure of a material. X-rays are used to eject electrons from the inner shells of atoms in the sample, and the resulting photoelectrons are measured. By analyzing the kinetic energy and intensity of these photoelectrons, valuable information about the elemental composition, chemical state, and electronic properties of the sample can be obtained.
- Overall, X-ray spectroscopy plays a vital role in various scientific fields, including materials science, chemistry, physics, and biology. Its ability to provide detailed information about the atomic and molecular structure of materials has enabled significant advancements in understanding the fundamental properties of substances and has applications in fields such as drug discovery, catalysis, nanotechnology, and forensic science.
History of X-ray spectroscopy
The history of X-ray spectroscopy dates back to the late 19th and early 20th centuries, with notable contributions from pioneering scientists. Here is an overview of the key milestones:
- Wilhelm Conrad Röntgen and the Discovery of X-rays (1895): German physicist Wilhelm Conrad Röntgen is credited with the discovery of X-rays in 1895. His groundbreaking work involved studying the properties of cathode rays and led to the unexpected observation of a new type of penetrating radiation. Röntgen’s discovery earned him the first Nobel Prize in physics in 1901.
- Charles Barkla and Characteristic X-rays (1906-1908): British physicist Charles Barkla conducted research between 1906 and 1908 that expanded our understanding of X-rays. He discovered that X-rays emitted by different substances could be characteristic and unique to each material. Barkla’s work in this area earned him the Nobel Prize in physics in 1917.
- William Henry Bragg and William Lawrence Bragg: X-ray Crystallography (1912): In 1912, British physicists William Henry Bragg and his son William Lawrence Bragg began their pioneering work in X-ray crystallography. They used spectroscopy to study the interaction of X-ray radiation with atoms in crystals. By analyzing the diffraction patterns formed when X-rays passed through crystals, they were able to determine the arrangement of atoms within the crystal lattice. Their technique revolutionized the field and became the standard method for studying crystal structures. The Braggs were jointly awarded the Nobel Prize in physics in 1915 for their contributions to X-ray crystallography.
The discoveries and advancements made by these scientists laid the foundation for X-ray spectroscopy and crystallography. Over the years, the field of X-ray spectroscopy has further developed with the introduction of new techniques, instrumentation, and applications. Today, X-ray spectroscopy continues to be a vital tool in various scientific disciplines, enabling researchers to gain insights into the atomic and molecular structures of materials.
Characteristic of X-ray spectroscopy
- Characteristic X-ray spectroscopy is a technique used to analyze the elemental composition of a specimen by examining the X-ray emission spectrum produced when electrons in the inner shells of atoms are excited and subsequently return to their lower energy levels.
- When an electron in the inner shell of an atom absorbs the energy of a photon, it undergoes an excitation and moves to a higher energy level. As the excited electron returns to its lower energy level, it releases the previously gained energy in the form of a photon. This emitted photon has a wavelength that is specific to the element from which it originated, and there may be multiple characteristic wavelengths associated with each element.
- The analysis of the X-ray emission spectrum provides qualitative information about the elemental composition of the specimen. By comparing the spectrum of the specimen with the spectra of samples of known composition, quantitative results can be obtained, usually after applying mathematical corrections for factors such as absorption, fluorescence, and atomic number.
- Different methods can be employed to excite atoms and generate characteristic X-rays. High-energy beams of charged particles, such as electrons or protons, can be used, or a beam of X-rays can be employed. These methods allow for the analysis of elements from the entire periodic table, excluding hydrogen (H), helium (He), and lithium (Li).
- In electron microscopy, for example, an electron beam is used to excite X-rays, and two primary techniques are employed to analyze the spectra of characteristic X-ray radiation: energy-dispersive X-ray spectroscopy (EDS) and wavelength-dispersive X-ray spectroscopy (WDS).
- In energy-dispersive X-ray spectroscopy (EDS), a semiconductor detector measures the energy of incoming photons. Cooling the detector, typically with liquid nitrogen or Peltier cooling, is necessary to maintain detector integrity and resolution. EDS is commonly used in electron microscopes where imaging is the main task, as well as in more affordable and portable X-ray fluorescence (XRF) units.
- Wavelength-dispersive X-ray spectroscopy (WDS) involves the use of a single crystal to diffract photons according to Bragg’s law, after which they are collected by a detector. By moving the diffraction crystal and detector relative to each other, a wide region of the spectrum can be observed. To cover a large spectral range, three or four different single crystals may be necessary. WDS offers superior spectral resolution and sensitivity compared to EDS but is slower and more sensitive to sample positioning. WDS is extensively utilized in microprobes where X-ray microanalysis is the primary task, as well as in XRF.
- In addition to EDS and WDS, X-ray transmission (XRT) spectroscopy is another approach that captures the equivalent atomic composition (Zeff) based on the photoelectric and Compton effects.
- Overall, characteristic X-ray spectroscopy techniques play a crucial role in determining the elemental composition of materials, with applications in various scientific fields such as materials science, geology, chemistry, and forensic science.
X Ray Spectroscopy Principle – Principle of x ray spectroscopy
The principle of X-ray spectroscopy is based on the interactions between electron beams and X-rays with samples. It takes advantage of the behavior of atoms when they interact with radiation, specifically X-rays.
When a material is exposed to high-energy, short wavelength radiation such as X-rays, the atoms within the material can become ionized. In other words, they lose or gain electrons, resulting in changes in their electronic configuration. This excitation of atoms occurs when an electron from the inner shell of an atom absorbs the energy of a photon (in this case, an X-ray photon), causing it to move to a higher energy level.
Subsequently, when the excited electron returns to its original, lower energy level, it releases the previously gained energy as a photon. This emitted photon has a wavelength that is characteristic of the element from which it originated. It’s important to note that each element may have several characteristic wavelengths associated with it.
The phenomenon of atomic X-rays being emitted during electronic transitions to inner shell states is particularly relevant for elements with modest atomic numbers. These emitted X-rays possess energies that are directly related to the atomic number of the element. As a result, each element exhibits a unique X-ray spectrum that can be utilized to identify and distinguish it from other elements.
X-ray spectroscopy techniques, such as X-ray fluorescence spectroscopy (XRF), make use of these principles. In XRF, a sample is exposed to high-energy X-rays, which cause the atoms in the sample to become excited. As the excited electrons return to their lower energy levels, they emit characteristic X-rays specific to the elements present in the sample. By analyzing the energy and intensity of these emitted X-rays, scientists can identify the elements within the sample and determine their concentrations.
The principle of X-ray spectroscopy, with its focus on electronic transitions and the subsequent emission of characteristic X-rays, forms the foundation for the analysis and identification of elements in various materials. By harnessing the unique X-ray signatures associated with different elements, X-ray spectroscopy enables researchers to gain insights into the composition, atomic structure, and electronic properties of materials, contributing to advancements in fields such as materials science, chemistry, and biology.
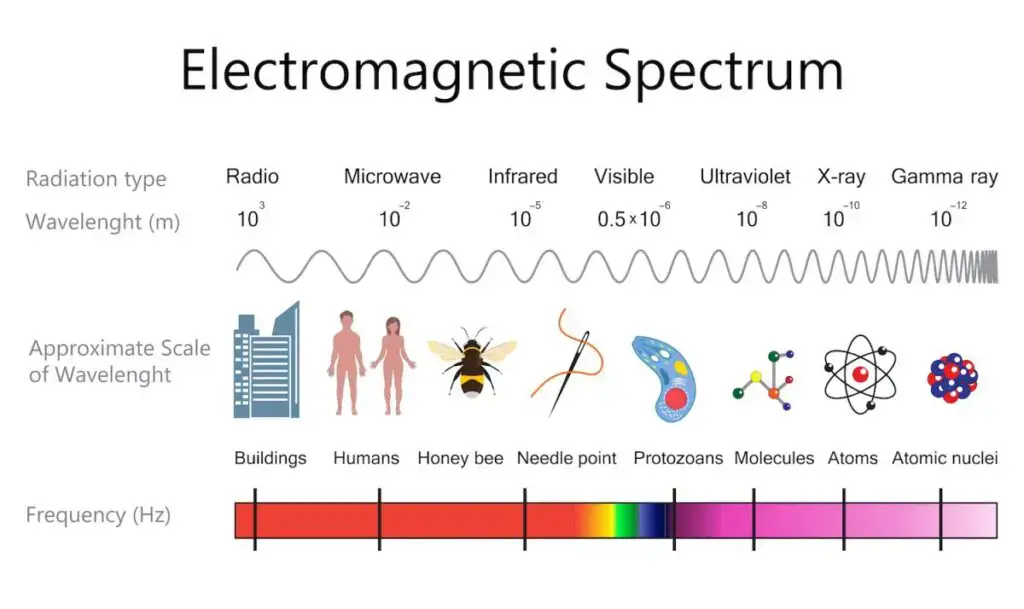
How X-ray spectroscopy works
- Incident Beam: An XRF (X-ray fluorescence) spectrometer illuminates the sample with an intense X-ray beam called the incident beam. This beam interacts with the atoms in the sample.
- Absorption and Scattering: When the incident X-ray beam strikes the sample, some of its energy is scattered, while some is absorbed within the sample. The absorption and scattering depend on the chemical composition of the sample.
- X-ray Emission: As a result of the interaction, the sample emits X-rays. These emitted X-rays have a spectrum of wavelengths that are characteristic of the types of atoms present in the sample.
- Characteristic Wavelengths: If the sample contains multiple elements, a Wavelength Dispersive Spectrometer (WDS) can be employed. This spectrometer allows the separation of the complex emitted X-ray spectrum into characteristic wavelengths for each element present in the sample.
- Detectors: Various types of detectors are used to measure the intensity of the emitted X-ray radiation. These detectors capture the emitted X-rays and quantify their energy.
- Intensity Measurement: The intensity of the energy measured by the detectors is directly proportional to the abundance of the corresponding element in the sample. Higher intensities indicate higher concentrations of the element.
- Quantification: To obtain the exact values for each element, standards derived from prior analyses using other techniques are utilized. These standards provide reference values against which the measured intensities can be compared, enabling the quantification of the elements present in the sample.
By employing these principles, X-ray spectroscopy allows scientists and researchers to analyze and characterize the elemental composition of various materials. It finds applications in fields such as materials science, geology, archaeology, environmental science, and forensic analysis.
X Ray Spectroscopy Instrumentation
The instrumentation of X-ray spectroscopy involves several key components and techniques:
- X-ray generating equipment (X-ray tube):
- X-rays are generated using an X-ray tube, which is a vacuum tube that accelerates electrons released by a hot cathode to high velocities.
- The accelerated electrons collide with a metal target (anode), resulting in the production of X-rays.
- Collimators:
- Collimators are devices used to narrow and align the beam of X-rays.
- They consist of parallel metal plates or a bundle of tubes to direct the X-rays in a specific direction, making them more collimated or parallel.
- Monochromators:
- Monochromators are utilized to select and transmit a narrow portion of the X-ray beam, allowing for the separation of specific wavelengths.
- They can partially polarize an unpolarized X-ray beam and are used to achieve spectral purity.
- Types of monochromators include metallic filter types and diffraction grating types.
- X-ray detectors:
- X-ray detectors are crucial for measuring and capturing the intensity of the X-rays emitted from the sample.Two commonly employed types of X-ray detectors are solid-state detectors and scintillation detectors.
- Solid-state detectors use semiconductors to detect X-rays.Incident radiation in the semiconductor material produces electron-hole pairs, and the resulting charge is collected and measured as an electrical pulse.Examples of solid-state detectors include silicon drift detectors (SDD) and silicon strip detectors (SSD).
- Scintillation detectors consist of a scintillator material that emits light when struck by X-rays.
- The emitted light is converted into an electrical signal by a device such as a photomultiplier tube (PMT).
- PMTs contain photocathodes, dynodes, and an anode to amplify the electrical signal.
- The signal amplification occurs as electrons are emitted from the photocathode, accelerated through the dynodes, and collected at the anode.
These components and techniques form the basis of X-ray spectroscopy instrumentation. They enable the generation, manipulation, and detection of X-rays, allowing scientists and researchers to analyze the composition and properties of materials in various fields of study.
Applications of X-Ray Spectroscopy
X-ray spectroscopy finds numerous applications across various fields. Some notable applications include:
- Petrology Research: X-ray spectrometry is used in the study of igneous, sedimentary, and metamorphic petrology. It helps analyze the composition and mineralogy of rocks, aiding in the understanding of geological processes and formations.
- Soil Surveys: X-ray spectroscopy is employed in soil surveys to determine the elemental composition of soils. This information is crucial for agricultural practices, environmental assessments, and land management.
- Mining: X-ray spectrometry plays a vital role in mining operations. It enables the measurement of the grade and quality of ores, helping in the identification of valuable minerals and the optimization of mining processes.
- Cement Production: X-ray spectroscopy is used in the cement industry for quality control and compositional analysis of raw materials, clinker, and cement products. It ensures compliance with specifications and facilitates process optimization.
- Ceramic and Glass Manufacturing: X-ray spectrometry assists in the quality control and characterization of ceramic and glass materials. It helps ensure consistency, identify impurities, and assess the elemental composition of these materials.
- Metallurgy: X-ray spectrometry finds application in metallurgical processes for quality control and material characterization. It helps determine the elemental composition of metals and alloys, aiding in product development and ensuring compliance with standards.
- Environmental Studies: X-ray spectroscopy is utilized in environmental studies to analyze particulate matter on air filters. It helps identify and quantify pollutants, assess air quality, and monitor environmental contamination.
- Petroleum Industry: X-ray spectrometry is employed in the petroleum industry to determine the sulfur content of crude oils and petroleum products. It assists in quality control, compliance with regulations, and optimization of refining processes.
- Field Analysis: Portable, hand-held XRF spectrometers are used for field analysis in geological and environmental studies. They enable on-site analysis of samples, allowing for quick assessments, rapid decision-making, and data collection in remote locations.
These applications demonstrate the versatility and importance of X-ray spectroscopy in various scientific, industrial, and environmental contexts. The technique’s ability to provide rapid and accurate elemental analysis contributes to advancements in research, resource exploration, quality control, and environmental monitoring.
Advantages of X-Ray Spectroscopy
X-ray spectroscopy offers several advantages that make it a valuable method for structural determination and analysis. Some key advantages include:
- Structural Determination: X-ray spectroscopy is particularly effective in determining the structure of a compound. When other spectral methods fail to reveal the identity of a compound, X-ray spectroscopy is often the method of choice. It provides detailed information about the arrangement of atoms in a crystal lattice, including bond lengths and bond angles.
- High Resolution: X-ray spectroscopy offers high resolution, allowing for precise measurements of crystal structures. It can provide accurate data on atomic positions within a crystal lattice, leading to a comprehensive understanding of the compound’s structure.
- Non-Destructive Analysis: X-ray spectroscopy is a non-destructive analytical technique. It does not alter or damage the sample during the analysis, preserving its integrity for further investigations or additional experiments.
- Wide Applicability: X-ray spectroscopy has broad applicability across various fields, including chemistry, materials science, solid-state physics, and biochemistry. It can be used to analyze a wide range of materials, from inorganic compounds to organic molecules, minerals, and biological macromolecules.
- Quantitative Analysis: X-ray spectroscopy enables quantitative analysis of the elemental composition of a sample. By measuring the intensities of characteristic X-ray emissions, it allows for the determination of the abundance and concentration of different elements present in the sample.
- Sensitivity to Light Elements: X-ray spectroscopy is particularly sensitive to light elements, such as carbon, nitrogen, and oxygen. It can accurately detect and analyze these elements, which is crucial for studying organic compounds, biomolecules, and complex materials containing lighter elements.
- Fast and Efficient: X-ray spectroscopy provides relatively fast results compared to other structural analysis techniques. With modern instrumentation and data processing methods, it is possible to obtain high-quality data in a relatively short time, enabling rapid decision-making and analysis.
- Complementary Technique: X-ray spectroscopy is often used in conjunction with other analytical techniques to complement and validate the results. It can be combined with techniques like infrared spectroscopy, mass spectrometry, and nuclear magnetic resonance (NMR) spectroscopy to provide a comprehensive characterization of a compound.
These advantages highlight the importance of X-ray spectroscopy in structural determination and analysis. Its ability to provide detailed information about the arrangement of atoms in a compound, its non-destructive nature, and its broad applicability make it a valuable tool in scientific research, material characterization, and various industries.
Limitations of X-Ray Spectroscopy
X-ray spectroscopy, despite its many advantages, also has certain limitations that should be considered. These limitations include:
- Single Crystal Requirement: One of the primary limitations of X-ray spectroscopy is the requirement for a compound to be available as a single crystal. The technique relies on the diffraction of X-rays by the crystal lattice, and obtaining a single crystal suitable for analysis can be challenging. This limitation restricts the application of X-ray spectroscopy to compounds that can be crystallized, which may not always be feasible.
- Tedious and Time-Consuming: The process of obtaining a single crystal suitable for X-ray analysis can be tedious and time-consuming. It involves careful preparation and optimization of crystallization conditions, which may require multiple attempts and considerable effort. This aspect of X-ray spectroscopy can be perceived as a drawback, especially when quick results are desired.
- Skillful Hand Requirement: The handling of single crystals for X-ray analysis requires a skillful hand. Mounting the crystal on the diffractometer, aligning it accurately, and collecting high-quality data demand expertise and precision. The technical aspects of the experimental setup and data collection process can pose challenges for researchers without prior experience in X-ray spectroscopy.
- Sensitivity to Crystal Quality: The quality of the crystal used for X-ray analysis is crucial for obtaining accurate and reliable results. Imperfections in the crystal, such as twinning, dislocations, or defects, can affect the diffraction pattern and introduce errors in the structural determination. Ensuring high-quality crystals can be a limitation, particularly for compounds that are difficult to crystallize or inherently exhibit crystal imperfections.
- Inapplicability to Amorphous Materials: X-ray spectroscopy is primarily applicable to crystalline materials. Amorphous substances lack a well-defined crystal lattice, making it challenging to obtain diffraction patterns for structural analysis. Consequently, X-ray spectroscopy is not suitable for studying the structure of amorphous materials, limiting its scope in certain areas of research.
- Radiation Damage: The high-intensity X-ray beam used in spectroscopic analysis can cause radiation damage to the sample. Overexposure to X-rays can induce structural changes or alter the sample’s properties, leading to inaccurate or misleading results. Careful control of exposure times and beam intensity is necessary to minimize radiation damage.
- Limited to Solids: X-ray spectroscopy is primarily employed for the analysis of solid-state materials. It is not well-suited for the study of liquids, gases, or solutions. The requirement for a well-defined crystal lattice restricts the application of X-ray spectroscopy to solid-state samples, limiting its use in certain areas of chemistry and biology.
Despite these limitations, X-ray spectroscopy remains a valuable technique for structural analysis, particularly when dealing with crystalline materials. The advancements in crystallographic instrumentation and techniques have mitigated some of these limitations, making X-ray spectroscopy a powerful tool in understanding the arrangement of atoms in a crystal lattice.
FAQ
What is X-ray spectroscopy?
X-ray spectroscopy is a technique used to study the interaction between X-rays and matter. It involves analyzing the emitted or scattered X-rays to obtain information about the elemental composition, chemical bonding, and structural characteristics of a material.
How does X-ray spectroscopy work?
X-ray spectroscopy works by irradiating a sample with an intense X-ray beam. When the X-rays interact with the atoms in the sample, they cause the emission or scattering of X-rays with characteristic energies and wavelengths. By analyzing the emitted or scattered X-rays, scientists can determine the elemental composition and other properties of the material.
What are the different types of X-ray spectroscopy techniques?
Some common types of X-ray spectroscopy techniques include X-ray fluorescence (XRF), X-ray photoelectron spectroscopy (XPS), X-ray absorption spectroscopy (XAS), and X-ray emission spectroscopy (XES).
What are the applications of X-ray spectroscopy?
X-ray spectroscopy has a wide range of applications, including materials science, chemistry, geology, environmental analysis, pharmaceuticals, forensic science, and archaeology. It is used for elemental analysis, identification of unknown substances, structural characterization, quality control, and many other purposes.
What are the advantages of X-ray spectroscopy?
X-ray spectroscopy offers several advantages, including its non-destructive nature, high sensitivity to trace elements, ability to provide both qualitative and quantitative analysis, and its capability to analyze a wide range of materials, from solids to liquids and gases.
What are the limitations of X-ray spectroscopy?
One limitation of X-ray spectroscopy is that it requires access to a pure crystalline sample for certain techniques like X-ray crystallography. Additionally, X-ray spectroscopy techniques may not be suitable for analyzing light elements such as hydrogen and helium.
What are the different components of an X-ray spectroscopy setup?
An X-ray spectroscopy setup typically consists of an X-ray source (e.g., X-ray tube), sample holder, detectors, collimators, monochromators, and data analysis software.
How is data analysis done in X-ray spectroscopy?
Data analysis in X-ray spectroscopy involves comparing the measured X-ray signals with reference spectra and using mathematical algorithms to determine the elemental composition, bonding information, and other relevant properties of the sample.
Is X-ray spectroscopy safe?
X-ray spectroscopy techniques are generally safe when conducted by trained professionals and with proper safety precautions. However, it is important to follow radiation safety guidelines and minimize unnecessary exposure to X-rays.
How has X-ray spectroscopy contributed to scientific advancements?
X-ray spectroscopy has played a crucial role in various scientific advancements. It has helped in understanding the structure of crystals, identifying unknown compounds, developing new materials, studying the electronic properties of materials, and advancing fields such as materials science, chemistry, and solid-state physics.
References
- Balci, Metin (2005). Basic 1H- and 13C-NMR Spectroscopy Volume 446 || Introduction. , (), 3–8. doi:10.1016/b978-044451811-8.50001-2
- Feng, X., Zhang, H. & Yu, P. (2020). X-ray fluorescence application in food, feed, and agricultural science: a critical review. Crit Rev Food Sci Nutr, 1-11.10.1080/10408398.2020.1776677
- Smyth, M. S. & Martin, J. H. (2000). x-ray crystallography. Mol Pathol, 53, 8-14.10.1136/mp.53.1.8
- Franklin, R. E. & Gosling, R. G. (1953). Molecular Configuration in Sodium Thymonucleate. Nature, 171, 740-741.https://doi.org/10.1038/171740a0
- https://www.iucr.org/__data/assets/pdf_file/0013/733/chap16.pdf
- https://www.azolifesciences.com/article/X-Ray-Spectroscopy-An-Overview.aspx
- http://instructor.physics.lsa.umich.edu/adv-labs/X-Ray_Spectroscopy/x_ray_spectroscopy_v2.pdf
- https://new.bhu.ac.in/Content/Syllabus/Syllabus_3006312820200416111005.pdf
- https://www.iaea.org/topics/x-ray-spectrometry
- http://www.issp.ac.ru/ebooks/books/open/X-Ray_Spectroscopy.pdf
- http://websites.umich.edu/~jphgroup/XAS_Course/Harbin/Lecture1.pdf