What is Plant Tissue Culture?
- Plant tissue culture represents a revolutionary advancement in plant breeding methodologies, emerging prominently around the 1950s. Traditional breeding techniques often failed to meet the increasing demands for crop production, thereby necessitating the development of more efficient approaches. Tissue culture, a method that utilizes specific plant parts, allows for the rapid generation of multiple plant copies in a controlled environment, significantly enhancing productivity and consistency.
- At its core, plant tissue culture exploits the concept of totipotency, which is the ability of plant cells to differentiate and develop into an entire plant. This means that any cell derived from any part of a plant can potentially regenerate a complete organism. The process involves cultivating explants—fragments of differentiated tissues—under in vitro conditions, where environmental factors can be precisely controlled. This method not only facilitates the cultivation of isolated cells, tissues, or entire organs but also enables the growth of whole plants in a sterile medium that is rich in essential nutrients.
- For effective tissue culture, it is crucial to maintain ideal conditions, including optimal nutrient supply, pH, and temperature. These factors create a conducive environment for plant growth and proliferation. As a result, plant tissue culture has become integral to crop improvement strategies, enabling the large-scale production of plants in a significantly reduced timeframe. Many economically valuable plant species have benefitted from this technique, leading to enhanced yield and resilience.
- Moreover, plant tissue culture has expanded our understanding of the underlying factors influencing growth, metabolism, morphogenesis, and differentiation in plants. The technique has been instrumental in propagating disease-free plant varieties and enhancing nutritional quality. Additionally, it has enabled the development of plants that exhibit increased tolerance to environmental stresses, such as salinity, drought, and cold temperatures.
- The applications of plant tissue culture extend beyond mere propagation. It has proven effective in the production of secondary metabolites, which are vital for various agricultural and pharmaceutical applications. Furthermore, this technique plays a crucial role in the conservation of endangered, threatened, and rare plant species, providing a method for their successful growth and preservation.
Plant tissue culture Definition
Plant tissue culture is a biotechnology technique that involves growing plant cells, tissues, or organs in a controlled, sterile environment on a nutrient medium. This method exploits the totipotency of plant cells, allowing for the rapid propagation of plants, production of disease-free specimens, and conservation of rare species.
History of Plant tissue culture
The history of plant tissue culture is marked by a series of pivotal discoveries and developments that have shaped the field. Below is a chronological overview of key contributions and milestones in the evolution of this significant biotechnological technique:
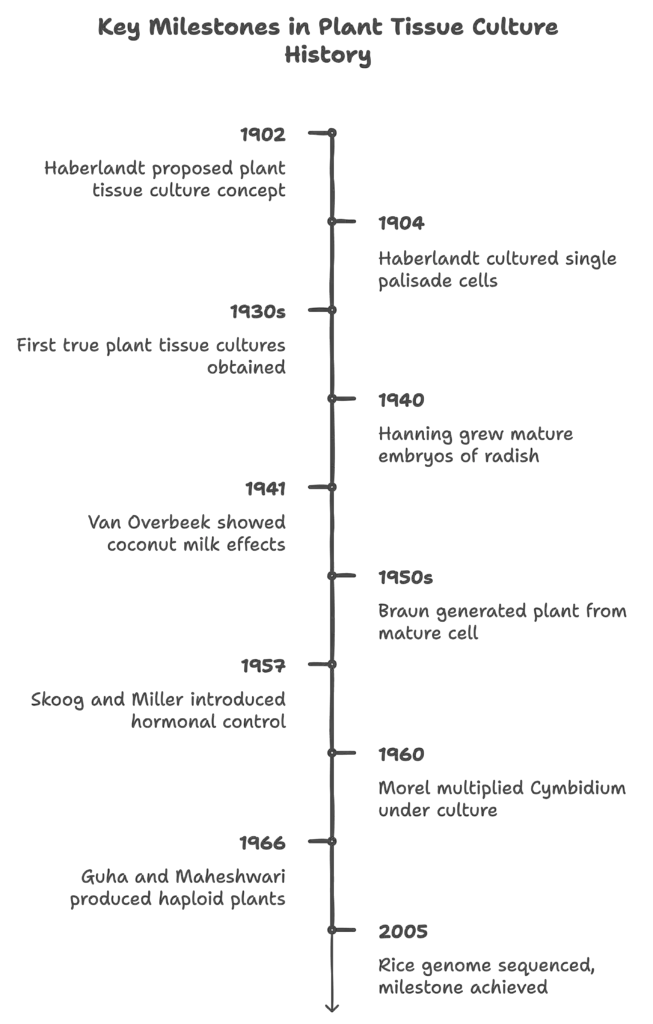
- 1902: German botanist Guttlieb Haberlandt first proposed the concept of plant tissue and cell culture. He cultured tissues from Lamium purpureum and Eichhornia crassipes, utilizing a salt solution enriched with sucrose. His pioneering work laid the foundation for future tissue culture techniques, although he noted that the highly differentiated cells lacked the necessary growth hormones for division.
- 1904: Haberlandt succeeded in culturing single palisade cells from leaves, observing their growth without cell division. He is often regarded as the “father of plant tissue culture” due to his contributions.
- 1930s: The first true plant tissue cultures were obtained by researchers like Gautheret, who raised cambial tissue from Acer pseudoplatanus and cultured explants from Ulmus campestre and Robinia pseudoacacia using agar-solidified media. These early experiments marked significant advancements in plant tissue culture methodologies.
- 1940: Hanning excised mature embryos of Raphanus sativus and successfully grew them on mineral salts and sugar solutions, contributing to the understanding of embryo culture.
- 1941: Van Overbeek demonstrated the stimulatory effect of coconut milk on embryo development and callus formation in Datura, indicating the importance of certain natural substances in tissue culture.
- 1950s: Braun successfully generated a plant from a mature plant cell, paving the way for commercial applications of tissue culture. In 1954, Muir broke callus tissues into single cells, further advancing the techniques.
- 1957: Skoog and Miller introduced the concept of hormonal control in organ formation, emphasizing the role of growth regulators in plant development.
- 1960: Morel laid the groundwork for commercial plant tissue culture by successfully multiplying Cymbidium, an orchid species, under cultured conditions.
- 1966: Guha and Maheshwari produced haploid plants from pollen grains of Datura, demonstrating the potential for genetic research and improvement in plants.
- 1970s: The development of protoplast fusion techniques allowed for the creation of somatic hybrids, expanding the possibilities for plant breeding and genetic manipulation. In 1972, Carlson produced the first interspecific hybrid of Nicotiana tobacum through protoplast fusion.
- 1980s and 1990s: Significant advancements included the introduction of various methods for genetic modification, such as electroporation and microinjection. The focus on improving transformation efficiency and reducing production costs for transgenic plants became paramount.
- 2005: The rice genome was sequenced under the International Rice Genome Sequencing Project, marking a significant milestone in the application of plant tissue culture in genetic engineering.
Equipments Required for Plant Tissue Culture
Plant tissue culture requires specialized equipment to ensure a sterile environment and controlled growth conditions. The laboratory setup is crucial for avoiding contamination and facilitating the successful growth and maintenance of plant tissues. The following is a detailed overview of the essential equipment required for plant tissue culture:
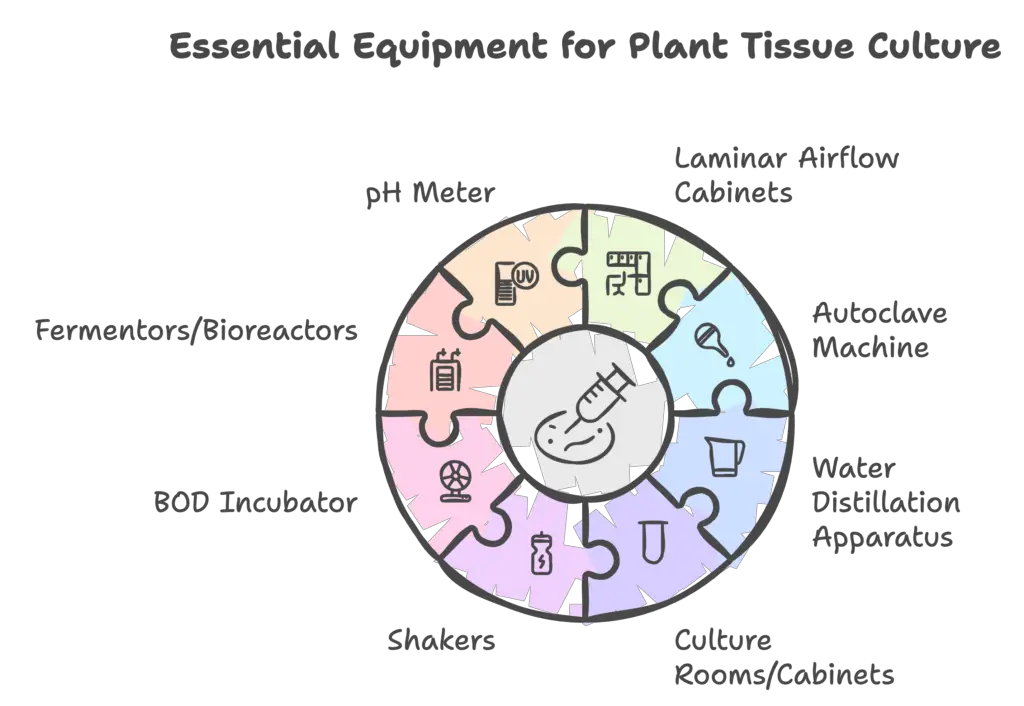
- Laminar Airflow Cabinets: These are essential for maintaining a contaminant-free environment, a critical aspect of plant tissue culture. The cabinet provides an aseptic workspace by filtering air to remove dust and microcontaminants, ensuring that the culture process remains sterile.
- Autoclave Machine: An autoclave is used for sterilizing equipment and culture media. Through the application of pressurized steam, it eliminates bacteria, fungi, and viruses. It can also sterilize scalpels, glassware (e.g., Petri dishes and pipettes), and other materials, ensuring that all tools and mediums are free from contaminants.
- Water Distillation Apparatus: This apparatus is necessary for producing distilled or deionized water, which is crucial for preparing sterile culture media and cleaning laboratory equipment.
- Culture Rooms/Cabinets: These are designed to maintain controlled environmental conditions, including temperature, humidity, and light. Adjustable photoperiods and light intensity ensure that cultures receive appropriate light exposure, while temperature controls facilitate optimal growth.
- Shakers: Suspension cultures require constant movement to ensure even distribution of nutrients and oxygen. Rotary or reciprocal shakers are used to maintain the necessary motion in liquid cultures.
- BOD Incubator: A Biological Oxygen Demand (BOD) incubator provides a controlled environment with consistent temperatures, crucial for culturing microorganisms and maintaining plant tissue cultures.
- Fermentors/Bioreactors: These are used in large-scale operations to cultivate plant cells for the production of secondary metabolites, biochemicals, or other valuable compounds. They allow for the mass production of plant-based products under controlled conditions.
- pH Meter: Maintaining the correct pH level of the culture medium is critical for cell growth. A pH meter ensures the precise adjustment of the medium’s acidity or alkalinity, supporting optimal culture conditions.
- Weighing Balance: This is used for accurately measuring various components of the nutrient medium, such as chemicals, salts, and other substances needed for plant growth.
- Freezer: A freezer is essential for storing chemicals, reagents, and stock solutions at appropriate temperatures to prevent degradation and contamination.
- Spirit Burner/Gas Micro Burner: These are used for flame sterilization of instruments, ensuring that tools like scalpels and forceps are sterile before use in the culture process.
- Incubation Room/Incubator: An incubator provides a controlled environment with the ideal temperature, light, and humidity levels required for plant tissue growth and differentiation. Light intensity and photoperiod duration can be adjusted, and shelves are designed to allow even exposure to these conditions across all culture vessels.
- Glassware: A variety of glassware is required for the preparation, storage, and cultivation of cultures. These include culture vessels, large test tubes (25×150 mm), conical flasks, graduated pipettes, measuring cylinders, beakers, filters, funnels, and Petri dishes.
- Sterilization Tools (Scissors, Scalpels, Forceps): These are used for preparing explants from excised plant tissues. Each tool must be sterilized regularly to prevent contamination during handling.
Basic Steps in Plant Tissue Culture
Plant tissue culture is the in-vitro technique of cultivating plant cells, tissues, or organs in a controlled environment to regenerate a complete plant. The process requires careful attention to sterility, preparation, and environmental factors. Here is a detailed breakdown of the fundamental steps involved in plant tissue culture:
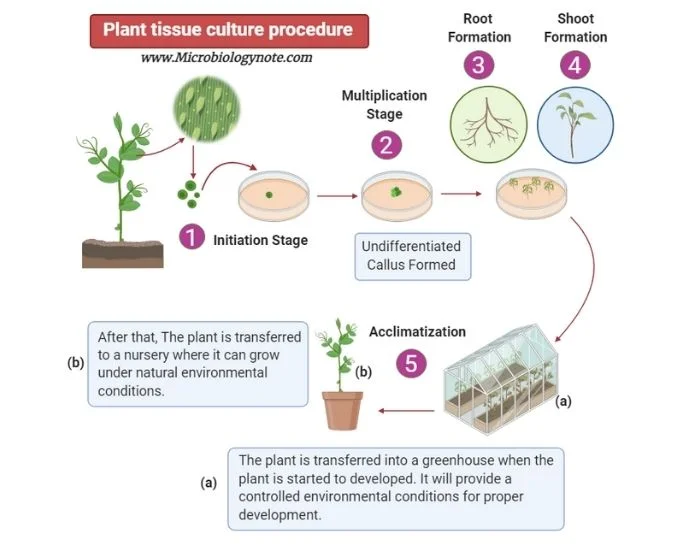
- Selection and Sterilization of Explant:
- The process begins with selecting a suitable explant from the donor plant. An explant refers to a small piece of tissue taken from the plant, which will serve as the starting material for tissue culture.
- After excising the explant, it is sterilized to eliminate any potential contaminants. The sterilization process involves using disinfectants like mercuric chloride (0.1-1%), calcium hypochlorite (1-2%), and alcohol (70%).
- The explant is immersed in the sterilizing agent for a period ranging from 10 seconds to 15 minutes, followed by thorough washing with distilled water to ensure complete removal of the sterilizing chemicals.
- Preparation and Sterilization of Culture Medium:
- A suitable culture medium is prepared based on the type of explant being cultured. The culture medium typically contains nutrients and hormones necessary for plant growth.
- The prepared medium is poured into sterilized vessels, which are then sterilized using an autoclave. This step ensures that the medium remains free from microbial contamination.
- Inoculation:
- Inoculation refers to the process of transferring the sterilized explant onto the prepared culture medium. This must be done under strictly aseptic conditions to avoid introducing contaminants during the transfer.
- Incubation:
- The inoculated cultures are placed in a culture room where they are incubated under carefully controlled conditions of light, temperature, and humidity. These environmental factors are critical for promoting optimal growth and development of the cultured tissue.
- Subculturing:
- As the cultured cells grow and develop, they need to be periodically transferred, or subcultured, into fresh nutrient media. This process ensures continuous nutrient supply and prevents overcrowding, which can inhibit growth. Subculturing is essential for producing plantlets from the growing tissue.
- Transfer of Plantlets:
- Once plantlets are sufficiently developed, they must undergo a hardening process, where they are gradually acclimatized to the external environment. This step prepares the plantlets for survival outside the controlled conditions of the culture room.
- After hardening, the plantlets are transferred either to a greenhouse or directly to pots, where they continue to grow into mature plants.
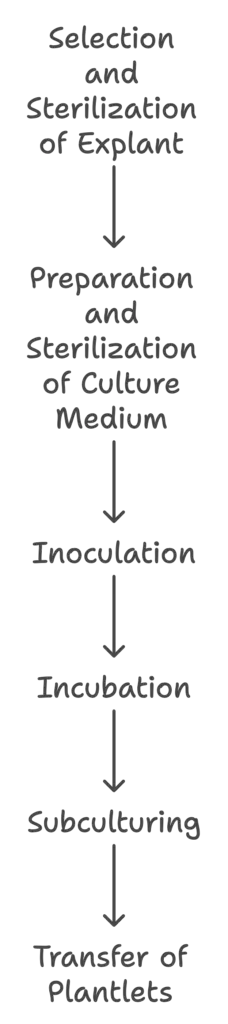
Conditions for Plant Tissue Culture
Plant tissue culture relies on a controlled environment to ensure successful growth and regeneration of plant tissues. Several key conditions must be maintained, including the composition of the culture medium, environmental factors, and sterility. The following are the essential conditions required for effective plant tissue culture:
- Nutrient Composition of Culture Medium:
- The culture medium is the foundation for plant growth in tissue culture. It contains macronutrients and micronutrients essential for cellular functions and development.
- Macronutrients: These include nitrogen, potassium, phosphorus, calcium, magnesium, and sulfur, which are required in larger amounts to support the growth of plant tissues.
- Micronutrients: These are required in smaller quantities but are equally important. Elements such as iron, manganese, zinc, boron, copper, molybdenum, and cobalt are typical micronutrients.
- Vitamins: Vitamins are necessary for metabolic processes in cultured tissues. Commonly used vitamins include thiamine (B1), pyridoxine (B6), and nicotinic acid (niacin). Sometimes, biotin, folic acid, ascorbic acid (vitamin C), and tocopherol (vitamin E) are added.
- Carbon Source: Since plant tissues in vitro lack access to photosynthesis, an external carbon source is provided. Sucrose is the most commonly used sugar, though other sources like glucose, maltose, and sorbitol may also be used.
- pH: The pH of the culture medium is critical as it affects nutrient uptake and the biochemical processes within the plant tissue. Most media are adjusted to a pH range of 5.2–5.8, though specific cultures may require higher pH levels.
- The culture medium is the foundation for plant growth in tissue culture. It contains macronutrients and micronutrients essential for cellular functions and development.
- Plant Tissue Culture Media Types:
- The type of medium used depends on the plant species and tissue type. Common media include:
- Murashige and Skoog (MS) medium: The most widely used medium for vegetative propagation.
- McCown’s Woody Plant Medium (WPM): Suitable for tree tissue cultures.
- Knudson’s Medium: Often employed for culturing orchids and ferns.
- The type of medium used depends on the plant species and tissue type. Common media include:
- Plant Growth Regulators (PGRs):
- PGRs are vital in regulating the development of plant cells and tissues. The balance and concentration of different PGRs, such as auxins and cytokinins, determine whether root or shoot development occurs.
- Auxins: Promote root formation. Common auxins include indoleacetic acid (IAA), indolebutyric acid (IBA), naphthaleneacetic acid (NAA), and 2,4-dichlorophenoxyacetic acid (2,4-D).
- Cytokinins: Stimulate cell division and shoot formation. Popular cytokinins include benzylaminopurine (BAP), zeatin, kinetin, and isopentenyl adenine (2-ip). When auxin and cytokinin levels are balanced, a mass of undifferentiated cells called a callus is formed.
- Gibberellins: Also used occasionally to promote cell elongation and growth in specific cultures.
- PGRs are vital in regulating the development of plant cells and tissues. The balance and concentration of different PGRs, such as auxins and cytokinins, determine whether root or shoot development occurs.
- Sterility:
- Maintaining a sterile environment is one of the most critical conditions in plant tissue culture. Plant tissues are highly susceptible to contamination from bacteria and fungi, which can outcompete the plant tissue for nutrients and destroy cultures.
- Surface Sterilization: Explants are sterilized using disinfectants like sodium hypochlorite, ethanol, or fungicides. The duration of sterilization varies depending on the tissue type, with leaf tissues requiring shorter exposure times than hard-coated seeds.
- Laminar Flow Hood: All tissue culture procedures are conducted in a laminar flow hood, which filters air using dust filters and HEPA (High-Efficiency Particulate Air) filters to create a sterile work environment. Regular cleaning with 70% alcohol is necessary to maintain sterility.
- Maintaining a sterile environment is one of the most critical conditions in plant tissue culture. Plant tissues are highly susceptible to contamination from bacteria and fungi, which can outcompete the plant tissue for nutrients and destroy cultures.
- Environmental Conditions:
- Light: Light intensity and photoperiod (light/dark cycle) play an essential role in plant growth. A photoperiod of 16 hours of light and 8 hours of darkness is often optimal. Cool white fluorescent lamps are typically used, providing a light intensity of 25–50 µmol m²/s¹.
- Temperature: The temperature in the growth chamber must be maintained at 22–25°C to ensure optimal growth.
- Humidity: A relative humidity level of 50–60% is maintained in most growth chambers. Some plant cultures are incubated in darkness to promote specific developmental processes.
- Ethylene Management:
- Ethylene is a gaseous hormone produced by plants, which can accumulate in closed culture vessels and negatively affect growth. To mitigate this, ethylene inhibitors such as silver nitrate, aminoethoxyvinylglycine (AVG), and silver thiosulfate can be added to the culture to reduce its harmful effects.
- Culture Vessels:
- Plant cultures can be grown in various vessels, including test tubes, flasks, Petri dishes, and bottles. These containers must be sterile, and their choice depends on the type and size of the culture being propagated.
Types of Plant Tissue Culture Media
There are present different types of Plant Tissue Culture Media such as;
- White’s medium: White’s medium was for the root culture.
- Murashige and Skoog (MS) medium: This medium was developed by Murashige and Skoog to induce organogenesis, and regeneration of plants in cultured tissues.
- B5 medium: Gamborg developed this medium for cell suspension and callus cultures. In modern-day it is used for protoplast culture.
- N6 medium: the medium was developed by Chu for the cereal anther culture, besides other tissue cultures.
- Nitsch’s medium: Nitsch formulated this medium to frequently be used for other cultures. Among the media mentioned above, MS medium is widely employed in plant tissue culture work due to its success with several plant species and culture systems.
Composition of Media for Plant Tissue Culture
The composition of media plays a pivotal role in plant tissue culture, as it directly influences the growth and morphogenesis of plant tissues under in vitro conditions. Various media have been developed to suit different plant species and types of explants, which can be parts like roots, stems, leaves, or meristematic tissues. Below is a detailed breakdown of the principal components commonly used in plant tissue culture media:
- Macronutrients:
- Macronutrients are essential for plant cell growth and development. These include elements such as nitrogen (N), phosphorus (P), potassium (K), calcium (Ca), magnesium (Mg), and sulfur (S).
- Nitrogen is usually supplied as ammonium nitrate (NH4NO3) or potassium nitrate (KNO3), while potassium and phosphorus are provided as potassium phosphate (KH2PO4).
- Stock solutions of macronutrients are typically prepared at 10 times the concentration of the working medium for ease of storage and use.
- Micronutrients:
- Micronutrients are required in smaller amounts but are equally critical for plant metabolism. These include iron (Fe), manganese (Mn), zinc (Zn), boron (B), copper (Cu), and molybdenum (Mo).
- Iron is often provided as Fe-EDTA, a chelated form to prevent precipitation in the medium. Other micronutrients are usually added in very small quantities (ppm levels), and stock solutions are made at 100 times the working concentration.
- Carbon Source:
- Sucrose is the most common carbon source used in plant tissue culture media, typically ranging from 2% to 6%. It provides the necessary energy for tissue growth and development, as plant cells in vitro are heterotrophic and rely on an external carbon source.
- Organic Supplements:
- Vitamins: Thiamine (B1), nicotinic acid (B3), and pyridoxine (B6) are common vitamins included in tissue culture media. These vitamins play important roles in enzymatic activities and cellular metabolism. Other vitamins like biotin and folic acid are added in specific media types.
- Amino Acids: Organic nitrogen sources such as casein hydrolysate, L-glutamine, and L-glycine are used to promote growth in protoplast cultures and stimulate cellular activities. Amino acids are crucial for protein synthesis and help in establishing robust cell lines.
- Other Organic Supplements: Coconut milk, yeast extract, and protein hydrolysates may be added to enhance growth and differentiation. Sometimes activated charcoal is included to adsorb toxic compounds produced during culture, thereby promoting healthier growth.
- Growth Regulators:
- Growth regulators are essential for promoting organogenesis, differentiation, and development of tissues in vitro.
- Auxins such as indole acetic acid (IAA), naphthalene acetic acid (NAA), and 2,4-dichlorophenoxyacetic acid (2,4-D) encourage cell elongation and root formation.
- Cytokinins like 6-benzylaminopurine (BAP) and zeatin stimulate cell division and shoot proliferation. The balance between auxins and cytokinins in the medium dictates the type of organogenesis—higher auxin levels promote rooting, while higher cytokinin levels favor shoot formation.
- Gibberellins like GA3 are used to elongate shoots and enhance callus growth in certain cultures.
- Gelling Agent:
- A gelling agent like agar, derived from seaweeds, is often used to solidify the medium. The concentration typically ranges from 0.5% to 1%, depending on the desired texture of the medium.
- Some cultures use liquid media, but semisolid media offer the advantage of holding explants in place, ensuring stable conditions for growth.
- pH Adjustment:
- The pH of the culture medium is another critical factor that influences nutrient availability and plant growth. It is typically adjusted to a range between 5.4 and 5.8 before sterilization, as this range is optimal for most plant tissues.
Examples of Commonly Used Plant Tissue Culture Media
- Murashige and Skoog (MS) Medium (1965): Widely used for a range of tissue culture applications, including organogenesis and regeneration. It contains high levels of nitrogen, potassium, and other essential nutrients.
- Gamborg’s B5 Medium (1968): Developed for callus and cell suspension culture, this medium features higher nitrate and potassium levels but lower ammonia content.
- Nitsch and Nitsch (NN) Medium (1969): Designed for anther culture, it is rich in thiamine, biotin, and folic acid, promoting effective in vitro anther culture for species like Nicotiana.
- White’s Medium (1963): Formulated for root culture, White’s medium contains lower concentrations of salts and a higher concentration of MgSO4, making it suitable for specific plant tissues.
- Chu’s N6 Medium (1975): Used primarily for rice anther culture, this medium facilitates the initiation and differentiation of callus and has been valuable in hybridization programs.
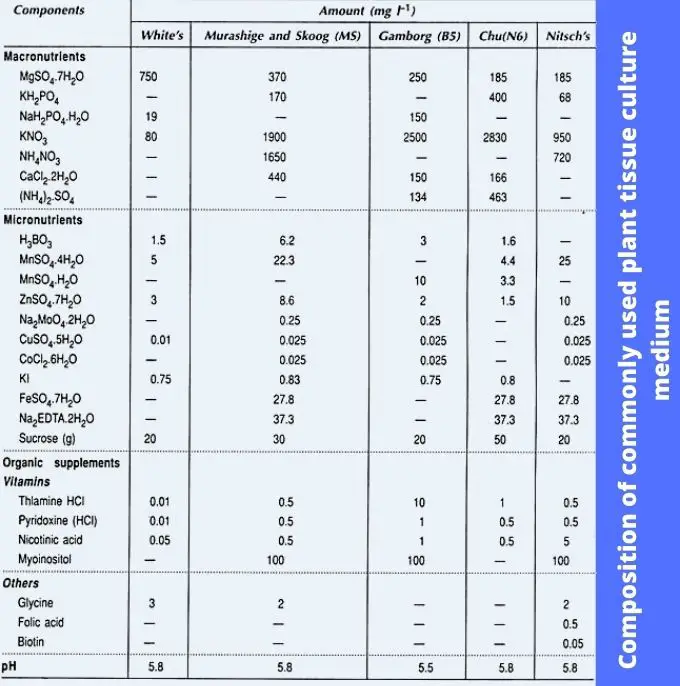
Preparation of Plant Tissue Culture Media
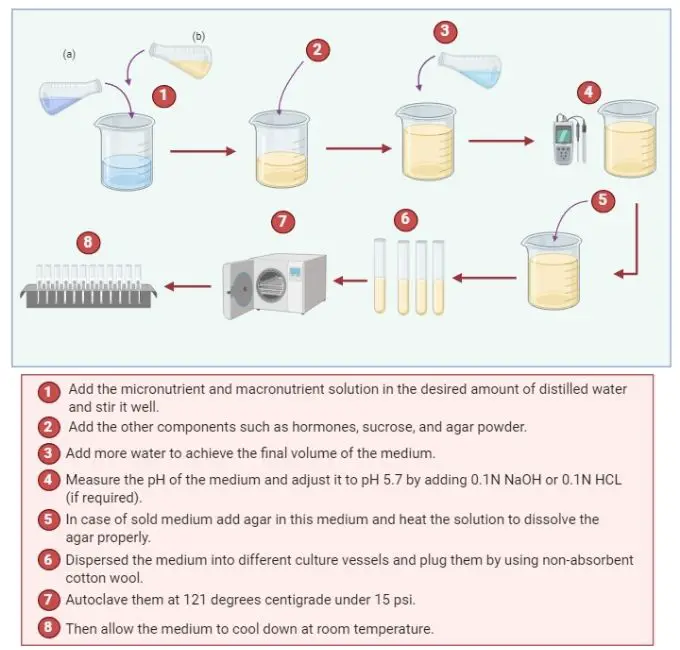
- Prepare the solution of macronutrients and micronutrients separately.
- Add the micronutrient and macronutrient solution in the desired amount of distilled water and stir it well.
- Add the other components such as hormones, sucrose, and agar powder. Add vitamins and auxins after autoclave to achieve better results.
- Add more water to achieve the final volume of the medium.
- Measure the pH of the medium and adjust it to pH 5.7 by adding 0.1N NaOH or 0.1N HCL (if required).
- In case of sold medium add agar in this medium and heat the solution to dissolve the agar properly.
- Dispersed the medium into different culture vessels and plug them by using non-absorbent cotton wool.
- After that autoclave them by wrapping them into cheese-cloth or with any other suitable closer.
- Autoclave them at 121 degrees centigrade under 15 psi.
- Then allow the medium to cool down at room temperature.
Sterilization of Plant Tissue Culture Media
The transmission of plant parasites can be reduced by preventing the contamination of tissue culture media. To control the contamination of culture medium we should follow these steps;
- Add antimicrobial agents, acidification agents to the medium (less employed in plant tissue culture media).
- Use microporous filters for the filtration of the medium.
- To decrease the chances of contamination, it is suggested that sterilization rooms should have the most limited number of openings.
- Perform the Media preparation and sterilization in a separate compartment or in a laminar airflow cabinet.
- The sterilization space should additionally possess walls and floor that withstand moisture, temperature, and vapor.
- Autoclave the medium at a temperature ranging from 115o – 135o C.
Types of Plant tissue culture
Plant tissue culture offers several techniques to propagate, regenerate, or manipulate plant tissues under controlled conditions. The types of plant tissue culture vary based on the specific plant tissues or cells being cultured and the desired outcome. Each method provides unique advantages for plant breeding, genetic studies, and commercial propagation. The following outlines the major types of plant tissue culture:
- Callus Culture:
- A callus is an unorganized mass of cells that forms when plant tissues are wounded. In callus culture, an explant (a piece of plant tissue) is placed on a culture medium containing auxins and cytokinins, which promote the growth of undifferentiated cells. This culture method is essential for generating large amounts of tissue for further manipulation.
- Function: Callus culture can continuously multiply through the influence of plant growth hormones or can be directed to develop organs (organogenesis) or somatic embryos (embryogenesis).
- Cell Suspension Culture:
- Cell suspension culture involves growing small fragments of a loose, friable callus in a liquid medium. This process is facilitated by placing the callus in a liquid medium and continuously shaking the culture, allowing single cells to grow in suspension.
- Function: Cell suspensions can be maintained over extended periods as batch cultures in flasks. The growth rate of cells in suspension is typically higher than that in solid culture media. This method is used to study plant metabolism, secondary metabolite production, and gene expression.
- Anther/Microspore Culture:
- Anther culture involves the in-vitro cultivation of anthers or isolated microspores (immature pollen cells) to produce haploid plants. These haploid plants can undergo chromosome doubling to create homozygous plants, which are genetically uniform.
- Function: This method shortens the time required to produce homozygous plants compared to traditional breeding. The plants produced from anther or microspore culture are valuable in plant breeding and genetic studies.
- Protoplast Culture:
- Protoplasts are plant cells without cell walls. These can be isolated from various plant tissues by removing the cell walls using mechanical or enzymatic methods. Protoplasts have the capacity to regenerate into whole plants when placed in an appropriate culture medium.
- Function: Protoplast culture is useful for creating somatic hybrids, transferring genes, and regenerating plants from a single cell. Protoplasts are particularly delicate but can be successfully cultured in either liquid or solid media, with higher success when embedded in an alginate matrix before culturing.
- Embryo Culture:
- This technique involves the in-vitro cultivation of embryos excised from immature ovules or seeds. Embryo culture is particularly useful for rescuing embryos from species with seed dormancy, sterility, or other growth challenges.
- Function: Embryo culture enables the direct regeneration of plants and is widely used in plant breeding to ensure successful hybridization. Additionally, somatic embryos or embryogenic calluses can be developed from immature embryos.
- Meristem Culture:
- Meristem culture involves the use of the apical meristem, a region of actively dividing cells at the tip of the shoot or root, to propagate plants. Depending on the part of the meristem used, this technique can be referred to as meristem culture, meristem tip culture, or shoot tip culture.
- Function: Meristem culture is instrumental in producing virus-free plants, as apical meristems are often free from viral infections. This method is commonly used in the propagation of disease-free clones, making it an essential tool in commercial plant propagation and the conservation of endangered species.
Totipotency
Totipotency is a fundamental concept in plant tissue culture, referring to the ability of a single plant cell to regenerate into a whole new multicellular organism. This potential relies on the inherent capacity of plant cells to undergo differentiation and to form various types of cells and tissues. Totipotency is essential for the successful regeneration of plants from explants, small portions of a plant that are cultured in controlled environments. The concept was first proposed in the 19th century, based on observations of plant regeneration, and it was further demonstrated by Muir in 1953 when plants were regenerated from isolated cells, confirming the validity of cellular totipotency.
- Definition and Origin:
- Totipotency refers to the ability of a plant cell to differentiate into any cell type and develop into a complete organism. This capacity depends on the cell’s ability to undergo differentiation, a process where cells mature and specialize.
- The term “totipotency” was coined by Morgan in 1901, and the theory emerged from earlier hypotheses about plant regeneration, which were proposed in the mid-19th century.
- Muir’s work in 1953 provided experimental evidence of totipotency, showing that isolated plant cells could regenerate into whole plants.
- Key Processes in Totipotency:
- Dedifferentiation: This process involves mature, specialized cells reverting to an unspecialized, dividing state. Dedifferentiation is a necessary step for a cell to regain its ability to form different cell types.
- Redifferentiation: Following dedifferentiation, cells undergo redifferentiation, where they re-specialize to form plant organs or even a whole plant. This cycle of dedifferentiation and redifferentiation is crucial for expressing totipotency.
- Transdifferentiation: In some cases, differentiation may occur directly from one organ to another without going through the undifferentiated state. This phenomenon, known as transdifferentiation, also plays a role in the expression of totipotency in certain plant systems.
- Varying Totipotent Abilities in Plants:
- Different plant parts exhibit varying levels of totipotency. In most cases, somatic cells in the plant body retain the ability to regenerate into whole plants. However, the degree of totipotency may vary between species, organs, or cell types, making some cells more capable of regeneration than others.
- Applications of Cellular Totipotency:
- Crop Improvement: Totipotency allows for the genetic modification of plants, which can lead to the development of improved crop varieties with enhanced resistance to diseases, pests, or environmental stresses.
- Micro-propagation: Totipotency is harnessed to rapidly propagate commercially valuable plants, ensuring consistent and large-scale production of desired species.
- Artificial or Synthetic Seeds: Totipotency aids in the production of synthetic seeds, which are used for the propagation of plants that are difficult to cultivate by traditional methods.
- Conservation of Germplasm: Through totipotent cells, the genetic diversity of plant species can be preserved and maintained, particularly for species at risk of extinction.
- Production of Haploids: Totipotency enables the creation of haploid plants, which are essential for breeding programs and research in plant genetics.
- Somatic Hybrids and Cybrids: The fusion of somatic cells or cytoplasmic hybrids, made possible by totipotency, contributes to the production of new plant varieties with combined traits from different species.
- Cultivation of Difficult-to-Germinate Plants: Totipotency allows for the cultivation of plants with seeds that are either minute or challenging to germinate through conventional means.
- High-Scale Production of Secondary Metabolites: The totipotent nature of plant cells supports the large-scale production of valuable secondary metabolites used in pharmaceuticals and other industries.
- Genotypic Modifications: Totipotent cells can be genetically modified to produce plants with specific traits or characteristics, further expanding the possibilities for plant improvement and innovation.
Organogenesis
Organogenesis is the process through which organs such as roots, shoots, and flowers are regenerated from explants or callus tissues in plant tissue culture. This phenomenon plays a critical role in the formation of a complete and organized plant structure from cultured cells or tissues. Organogenesis is induced by a combination of factors, including substances present in the culture medium, compounds from the explants, and substances produced during culturing. It can occur through either direct or indirect pathways, depending on whether callus formation is involved.
- Definition and Foundation:
- Organogenesis refers to the development or regeneration of plant organs such as roots, shoots, and flowers directly from explants or callus. It begins with the formation of meristematic cells (meristemoids), which initiate the creation of primordia. These primordia eventually develop into shoots or embryoids.
- The concept of organogenesis was first proposed by Torrey in 1966, while the differentiation of shoot buds was earlier demonstrated by White in 1939.
- Direct and Indirect Organogenesis:
- Direct Organogenesis: In this mode, organs like adventitious shoots form directly from the explant without the intermediate step of callus formation.
- Indirect Organogenesis: This process involves the formation of callus first, followed by the differentiation of organs from the callus. Both pathways require dedifferentiation and redifferentiation to form a complete plant structure.
- Explants and Organogenesis:
- Organogenesis has been achieved using various plant tissues, including cotyledons, hypocotyls, stems, leaves, shoot apices, roots, young inflorescences, flower petals, petioles, and embryos. The process involves two essential steps:
- Dedifferentiation: The explant tissue forms callus, which consists of undifferentiated cells.
- Redifferentiation: Specific primordia develop from the callus, eventually giving rise to plant organs.
- Organogenesis has been achieved using various plant tissues, including cotyledons, hypocotyls, stems, leaves, shoot apices, roots, young inflorescences, flower petals, petioles, and embryos. The process involves two essential steps:
- Caulogenesis and Rhizogenesis:
- The two main components of organogenesis are:
- Caulogenesis: This is the formation of shoots and is initiated by the induction of adventitious shoot buds. It is regulated by cytokinin concentrations in the culture medium.
- Rhizogenesis: This is the process of root formation, which generally follows caulogenesis. Rhizogenesis is influenced by the balance of growth regulators, especially auxins.
- The two main components of organogenesis are:
- Culture Media Used in Organogenesis:
- Organogenesis is supported by different culture media formulations, including:
- MS medium (Murashige and Skoog, 1962)
- B5 medium (Gamborg, 1969)
- White’s medium (White, 1963)
- SH medium (Schenk and Hildebrandt, 1972)
- Organogenesis is supported by different culture media formulations, including:
- Factors Regulating Organogenesis:
- Organogenesis is regulated by a variety of chemical and physical factors:
- Explants: The physiological state and size of the explant influence its ability to undergo organogenesis.
- Environmental Conditions: Light and temperature play key roles in the process. High light intensity can inhibit shoot-bud formation, while blue light promotes shoot differentiation and red light enhances rooting. A temperature around 33°C supports callus growth, while lower temperatures (e.g., 18°C) encourage shoot-bud differentiation.
- Growth Regulators: The concentration of growth hormones such as auxins and cytokinins critically impacts organogenesis. Skoog and Miller (1957) demonstrated that the balance between these hormones controls the formation of roots and shoots. A high concentration of auxins promotes callus formation and root differentiation, while cytokinins favor the development of shoot buds.
- Organogenesis is regulated by a variety of chemical and physical factors:
- Role of Growth Regulators:
- Auxins: A moderate to high concentration of auxin (such as 2,4-D) induces callus formation by stimulating cell proliferation. Auxins favor root differentiation at higher concentrations.
- Cytokinins: These hormones promote shoot-bud differentiation, especially when present in relatively high concentrations compared to auxins.
- Significance of Organogenesis:
- Organogenesis provides valuable insights into the mechanisms of hormone action, cell and tissue differentiation, plant organ development, and molecular pathways involved in plant growth. It is an essential tool for understanding the complex interplay of factors that regulate plant morphogenesis.
Embryogenesis or Embryogenic Differentiation
Embryogenesis, or embryogenic differentiation, refers to the process by which embryos regenerate from somatic cells of plant organs. This process, known as somatic embryogenesis, involves the development of embryos from somatic cells of the embryo sac or the surrounding tissues, without the fusion of gametes. Somatic embryos, also referred to as embryoids, can be generated in vitro, and this technique has broad applications in plant propagation and preservation.
- Definition and Foundation:
- Somatic embryogenesis involves the formation of embryos from somatic (non-reproductive) cells rather than from zygotes. These embryos, called somatic embryos or embryoids, are formed in vitro without gametic fusion, thus bypassing the need for sexual reproduction.
- The process was first observed in carrot (Daucus carota) by Steward and colleagues in 1958. Since then, somatic embryos have been successfully developed in several plant species, including Citrus, Coffea, and Zea mays.
- Methods of Inducing Somatic Embryogenesis:
- Somatic embryogenesis can be achieved through two main methods:
- Direct Embryogenesis: In this approach, embryos develop directly from the cells of explants or immature embryos. This pathway bypasses the callus formation stage.
- Indirect Embryogenesis: This method involves an intermediate step where callus tissue is first produced from the explant. The embryogenic callus is then transferred to a proliferation medium to induce embryo development.
- Somatic embryogenesis can be achieved through two main methods:
- Steps in Somatic Embryogenesis:
- Somatic embryogenesis follows a multi-step process that includes:
- Induction of Embryogenesis: The initial phase involves the induction of embryogenesis from explant tissues, often facilitated by the presence of specific growth regulators in the culture medium. Auxin is particularly important during this stage.
- Embryo Development: After induction, the embryoids begin to develop, resembling the stages of normal zygotic embryo development.
- Embryo Maturation: The somatic embryos undergo maturation, eventually giving rise to structures capable of developing into fully functional plants.
- Somatic embryogenesis follows a multi-step process that includes:
- Factors Influencing Embryogenesis:
- Physiological Conditions: The success of somatic embryogenesis is influenced by the physiological state of the explant tissue.
- Genotype of the Plant: Different plant species, and even different genotypes within a species, exhibit varying levels of embryogenic potential.
- Type of Explants: The specific tissue or organ used as the explant source plays a critical role in determining the efficiency and outcome of somatic embryogenesis.
- Growth Regulators in Embryogenesis:
- Growth regulators, particularly auxins, are essential for inducing embryogenesis. The concentration and balance of these regulators in the culture medium are critical for initiating and supporting the embryogenic process.
- Importance of Somatic Embryos:
- Large-Scale Propagation: Somatic embryos provide a valuable resource for the large-scale propagation of germplasm, making it possible to propagate plants rapidly and efficiently without relying on sexual reproduction.
- Cryo-Storage of Germplasm: Somatic embryos serve as an important tool for cryo-preservation, allowing the long-term storage of plant embryos and valuable genetic resources.
- Rapid Plant Propagation: Since somatic embryogenesis bypasses the fertilization process, it facilitates the rapid multiplication of plants, offering significant advantages in agricultural and horticultural applications.
Protoplast Culture
Protoplast culture refers to the in-vitro cultivation of protoplasts—plant cells that have had their cell walls removed, leaving a plasma membrane-bound vesicle. This technique is significant for somatic hybridization, where protoplasts from somatic cells of the same or genetically different plants are fused to create a hybrid. The process allows for the development of a whole plant from the fused protoplast. Historically, the technique has enabled breakthroughs in plant regeneration and hybridization.
- Definition and Isolation:
- A protoplast is a plant cell without its cell wall, consisting only of the plasma membrane and its contents. Protoplasts can be isolated from various plant tissues, such as leaf and mesophyll cells. The process of isolation can be carried out mechanically or enzymatically.
- Mechanical Isolation: The first mechanical isolation of protoplasts was achieved by J. Klercker in 1892 from the plasmolyzed cells of Stratiotes aloides. Later, E. Kuster isolated protoplasts from fruits of various plants using a physiological method involving cell wall hydrolysis.
- Enzymatic Isolation: E.C. Cocking developed the first enzymatic method in 1960, using cellulase enzyme to isolate protoplasts from Lycopersicon esculentum root tip cells. Takebe, Otsuki, and Aoki (1968) advanced this method by sequentially using cellulase and macerozyme enzymes to isolate protoplasts from tobacco mesophyll cells. Power and Cocking (1968) later demonstrated that a mixture of these two enzymes could be used simultaneously for a one-step protoplast isolation method.
- Protoplast Isolation Process:
- To isolate protoplasts, the cell wall is enzymatically digested using cellulase and pectinase enzymes, followed by placing the protoplast in a hypertonic solution (usually 13% mannitol) to prevent plasmolysis. After isolation, the protoplasts are purified, and their viability is tested before they are cultured in a suitable nutrient medium, either liquid or semi-solid.
- Protoplast Culture and Fusion:
- Once isolated, protoplasts can be cultured and regenerated into whole plants. Protoplast fusion, or somatic hybridization, involves fusing the protoplasts from two different plant species or varieties. This fusion can be induced mechanically or chemically:
- Mechanical Fusion: Protoplasts are brought into physical contact using tools like a micromanipulator and perfusion micropipette under a microscope.
- Chemical Fusion: Fusogens such as Polyethylene Glycol (PEG), high concentrations of calcium ions (Ca²⁺), Dextran, Polyvinyl Alcohol (PVA), or synthetic phospholipids are used. These fusogens bind with the lipid membrane, reducing the negative charge on the protoplasts, which otherwise repel each other due to their natural electronegativity. As a result, the protoplasts fuse.
- Electrofusion: This method involves applying an electric current to align the protoplasts, followed by a high-intensity electrical pulse to induce fusion.
- Once isolated, protoplasts can be cultured and regenerated into whole plants. Protoplast fusion, or somatic hybridization, involves fusing the protoplasts from two different plant species or varieties. This fusion can be induced mechanically or chemically:
- Hybrid Cell Formation:
- After fusion, the resultant hybrid cell contains two nuclei from the parent protoplasts, a condition known as heterodikaryon. These cells can be identified using staining techniques such as Carbol fuchsin, aceto-orcein, or aceto-carmine. Fluorescent staining techniques using non-toxic fluorochromes like FITC (fluorescein isothiocyanate) or RITC (rhodamine isothiocyanate) can also be employed. Heterokaryotic cells fluoresce apple-green under FITC and red under RITC.
- Factors Affecting Protoplast Viability:
- Several factors influence the viability and regeneration of protoplasts, including light conditions, temperature, humidity, nutrition, water availability, and the age of the plant tissue used for isolation. The photoperiod (light duration) and the physiological state of the plant tissue also play a role in the success of protoplast culture and regeneration.
Importance of Protoplast Culture
The following points outline the key practical applications and importance of protoplast culture.
- Improvement of Plant Species:
- Protoplast culture has immense potential for enhancing plant species and varieties with significant economic value. This technique serves as a foundation for developing new cultivars that may better meet agricultural demands.
- Overcoming Sexual Incompatibility:
- One of the most notable advantages of protoplast culture is its capability to create somatic hybrids between sexually incompatible species. This property proves to be a robust method for transcending the barriers of sexual incompatibility, thus broadening the genetic pool available for plant breeding.
- Cytoplasmic Hybrids (Cybrids):
- Protoplast fusion facilitates the formation of cybrids, which are hybrids that contain the nuclear genome of one parent and the cytoplasmic genome of another. This technique allows for the development of cytoplasmic male sterile (CMS) lines, enhancing breeding programs.
- Genetic and Cytoplasmic Modification:
- Isolated protoplasts serve as an excellent substrate for conducting genetic and cytoplasmic modification experiments. This aspect of protoplast culture is vital for understanding plant genetics and improving traits through targeted modifications.
- Direct Gene Transfer:
- Protoplasts are ideal targets for direct gene transfer techniques. Researchers can successfully transfer genes responsible for traits such as disease resistance, nitrogen fixation, improved growth rates, protein quality, frost hardiness, and drought resistance through this method.
- Inducing Genetic Variation:
- In vegetatively propagated plants, protoplast fusion can induce genetic variation by combining genetic material from two species, varieties, or different genera. This application opens pathways to create new genetic combinations and enhance biodiversity.
- Hybrid Vigor and Apomictic Development:
- The production of plants exhibiting greater hybrid vigor has been achieved using somatic hybrids formed through protoplast fusion. Furthermore, successful apomictic development of somatic hybrids has been recorded in economically important crops like orange (Citrus spp.) and mango (Mangifera indica).
- Plant Regeneration in Forestry:
- Protoplast culture facilitates the regeneration of plants across various forest tree taxa. Species such as Eucalyptus, Populus alba, Picea, Abies, Larix, and Pinus taeda can benefit from this technology, which supports reforestation and conservation efforts.
- Fusion with Yeast Cells:
- Protoplast fusion technology extends beyond plants; it also encompasses the fusion of plant cells with yeast cells. This cross-organism fusion is essential for characterizing yeast artificial chromosomes (YAC), which serve as promising vehicles for gene transfer.
- Cereal Crop Improvement:
- Protoplast culture has played a pivotal role in improving cereal crops, including wheat and maize. The successful production of tetraploids and triploids in orange (Citrus spp.) illustrates the effectiveness of protoplast fusion in developing plants with desirable traits. Additionally, intergeneric hybrids in Brassica have been developed through this innovative culture technique.
Advantages of Plant tissue culture
The following highlights the key benefits of plant tissue culture:
- Totipotency: Plant cells possess the ability to regenerate into whole plants, a property known as totipotency. This characteristic allows for the growth of entire plants from individual cells or tissues, facilitating large-scale propagation.
- Nutritional and Metabolic Control: In tissue culture, the nutritional needs of plant cells are precisely managed. The culture media can be tailored to provide specific macronutrients, micronutrients, vitamins, and growth regulators essential for optimal growth and development.
- Morphogenesis and Regeneration: Plant tissue culture techniques, such as organogenesis and somatic embryogenesis, enable efficient morphogenesis, which is the process through which plants regenerate from cells or tissues. This capability supports rapid plant regeneration and the production of multiple clones from a single explant.
- Creation of Variations: In vitro culture techniques can generate variations in plant characteristics, contributing to the development of new cultivars with desirable traits. This process is crucial for breeding programs aimed at enhancing yield, disease resistance, and stress tolerance.
- Haploid Production: The use of anther and pollen culture allows for the production of haploid plants, which can be valuable in breeding programs. Haploids expedite the process of obtaining homozygous lines, facilitating genetic research and the development of improved plant varieties.
- Wide Hybridization: Plant tissue culture supports wide hybridization efforts by enabling the culture of ovules, ovaries, and embryos. This approach helps overcome pre-zygotic and post-zygotic sterility mechanisms, allowing for the successful hybridization of otherwise incompatible species.
- Micropropagation: One of the most significant advantages of plant tissue culture is the ability to micropropagate plant materials. This technique allows for the rapid multiplication of disease-free plants, ensuring uniformity and quality in commercial production.
- In Vitro Selection of Mutants: Plant tissue culture enables the selection of mutants that exhibit tolerance to biotic and abiotic stresses. This selective breeding approach can enhance the resilience of crops to environmental challenges, pests, and diseases.
- Secondary Metabolite Biosynthesis: The in vitro culture of plant tissues can promote the biosynthesis of secondary metabolites, which are often valuable for medicinal and industrial purposes. By manipulating culture conditions, researchers can optimize the production of these compounds.
- Genetic Engineering: Plant tissue culture techniques are essential for plant genetic engineering, allowing for the transfer of DNA into plant cells. This facilitates the development of genetically modified organisms (GMOs) with enhanced traits, such as improved nutritional content or pest resistance.
Disadvantages of Plant Tissue Culture
These disadvantages can affect the cost, efficiency, and outcomes of the process.
- Labor-Intensive: Plant tissue culture requires a high degree of manual labor, particularly in the preparation of explants, media, and the maintenance of sterile conditions. Each step in the process—from selecting the appropriate explant to transferring plantlets—demands precision, attention to detail, and skill. Therefore, the labor intensity involved in tissue culture contributes to its complexity.
- Expensive Process: The costs associated with plant tissue culture can be prohibitively high, especially when compared to traditional propagation methods. The need for specialized equipment, including laminar flow hoods, autoclaves, and growth chambers, as well as expensive chemicals like growth regulators and gelling agents, drives up the expenses. Besides, the cost of maintaining sterile environments, particularly in large-scale operations, adds further to the financial burden.
- Vulnerability to Contamination: One of the most significant challenges in plant tissue culture is contamination. Fungi, bacteria, and other microorganisms can easily infiltrate the culture environment, compromising the success of the entire process. Even slight lapses in sterility can lead to the loss of cultures, resulting in wasted time and resources. Explants, being inherently susceptible to microbial contamination, must be thoroughly disinfected to avoid such risks.
- Sensitivity to Environmental Conditions: The success of tissue culture depends heavily on controlled environmental factors, such as temperature, light, and humidity. Any deviation from the optimal conditions can adversely affect the growth and development of plant tissues. Moreover, in vitro cultures may be highly sensitive to minor changes in media composition, pH, or the concentration of growth regulators, making them prone to developmental abnormalities.
- Somaclonal Variation: Although genetic uniformity is one of the goals of plant tissue culture, somaclonal variation—genetic changes occurring during tissue culture—can lead to unintended variations. While this may sometimes result in beneficial traits, it can also produce undesirable mutations that affect the quality or characteristics of the regenerated plants. This variability can reduce the reliability of tissue culture for producing true-to-type clones.
- Acclimatization Challenges: Plantlets produced through tissue culture often face difficulties when transferred to soil environments. Since these plantlets are grown in a highly controlled in vitro environment, they may lack the necessary adaptations to survive in natural field conditions. The hardening process, which prepares plantlets for outdoor conditions, can be time-consuming and requires careful management.
- Expertise Required: The success of plant tissue culture hinges on a high level of technical expertise. Operators must be well-versed in the techniques of sterilization, media preparation, and handling plant tissues. Any errors or lack of knowledge can result in failed cultures, limiting the accessibility of the technique to only those with advanced training and experience.
Applications of Plant Tissue Culture
Below are the major applications of plant tissue culture, highlighting its broad impact.
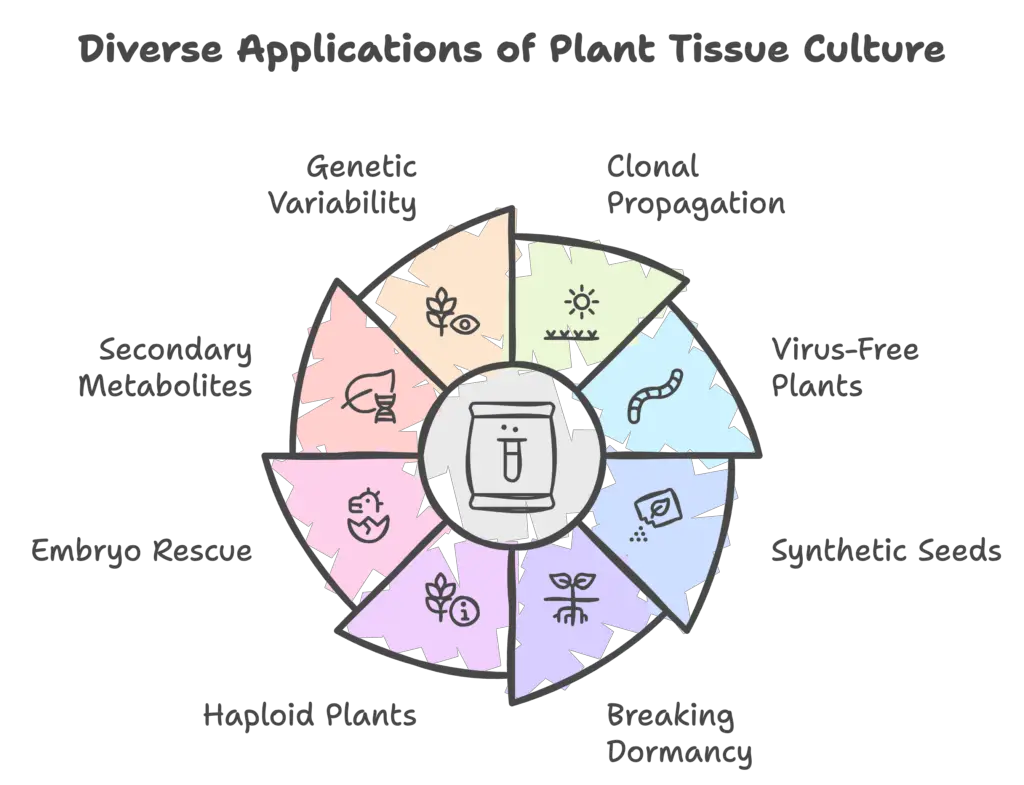
- Clonal Propagation:
- Tissue culture is widely used for clonal propagation, allowing rapid, large-scale multiplication of genetically identical plants (clones) through asexual reproduction. Apical shoots, axillary buds, and meristems are cultured to regenerate multiple plantlets. This method is particularly beneficial in horticulture and forestry, providing plant material in bulk, regardless of seasonal changes. Disease-resistant plants and sterile hybrids can be multiplied, and it helps to overcome seed dormancy in tree species. Examples include successful propagation of apple, pears, strawberry, cardamom, and orchids.
- Production of Virus-Free Plants:
- Tissue culture enables the production of virus-free plants through meristem-tip culture. Since meristems are generally free from infections, they are cultured to obtain plants devoid of viruses. Commercially, this method has been used to eliminate viruses like Potato virus X and cassava mosaic virus. Additionally, in vitro propagation of economically valuable tree species, such as Acacia nilotica, Tectona grandis, and Pinus sylvestris, has been achieved through micropropagation.
- Production of Synthetic Seeds:
- Somatic embryos generated by tissue culture are encapsulated in a matrix (such as sodium alginate) to form synthetic seeds. These seeds are useful for rapid mass propagation, easy handling, and long-term storage. They can be sown directly into the soil without greenhouse acclimatization, making them a practical alternative to natural seeds.
- Breaking Dormancy:
- Tissue culture techniques, such as zygotic embryo culture, help in reducing seed dormancy and shortening the breeding cycle. In some species, this enables two generations of flowering within a year. The life cycle of plants like Iris has been reduced from 2-3 years to less than a year through this method, significantly speeding up plant breeding processes.
- Haploid Plants:
- Haploid plants, which possess a single set of chromosomes, can be obtained via anther or pollen culture (androgenesis) or through ovary and ovule culture (gynogenesis). These haploids are essential for producing homozygous diploid or polyploid lines in a short time without extensive backcrossing. Chromosome doubling can restore the fertility of haploids, making them pure breeding lines with desired traits. Haploid plants are valuable in plant breeding for developing resistance to stresses and other desirable characteristics.
- Embryo Rescue:
- Some seeds fail to germinate or take an extended time to do so. Tissue culture allows for the rescue of immature embryos through the embryo rescue technique, where embryos are cultured on a nutrient medium to grow into complete plants. This process ensures that plants with long dormancy periods or non-viable seeds can still be propagated.
- Production of Secondary Metabolites:
- Secondary metabolites, which are valuable for pharmaceuticals, agriculture, and industry, are produced in large quantities by cultured plant cells. These compounds, such as alkaloids, glycosides, terpenoids, and tannins, possess antimicrobial, insecticidal, and pharmacological properties. Tissue culture provides controlled conditions to enhance the production of these biochemicals, making it a powerful tool in commercial biotechnology.
- Genetic Variability (Somaclonal Variations):
- Plants regenerated from tissue culture often exhibit somaclonal variation, heritable differences in both qualitative and quantitative traits. These variations, which arise due to changes in ploidy levels or cultural conditions, are valuable for crop improvement. Somaclonal variants may exhibit traits such as disease resistance, herbicide tolerance, stress tolerance, and improved agronomic qualities like yield and plant height.
- Somatic Hybrids:
- Tissue culture facilitates somatic cell hybridization or protoplast fusion, allowing the combination of desirable traits from two species or genera that cannot hybridize sexually. This technique is crucial for producing somatic hybrids with disease resistance and other valuable traits, such as resistance to viruses like Tobacco mosaic virus and club rot disease.
- Transgenic Plants:
- Transgenic plants, which have been genetically modified to incorporate foreign genes, can be produced via tissue culture techniques. Genes for traits such as insect resistance, herbicide tolerance, improved oil quality, and enhanced nutrient content can be introduced through methods like electroporation, microinjection, or Agrobacterium-mediated transformation. Genetic engineering in crops is facilitated by tissue culture, making it a key tool in agricultural biotechnology.
- Plant Germplasm Conservation:
- Tissue culture plays an important role in the conservation of plant germplasm, preserving the genetic diversity of crops and related species. Germplasm can be conserved through in situ methods (biosphere reserves) or ex situ methods, where seeds or in vitro cultures are stored in gene banks for long-term use. This preservation is vital for maintaining valuable genetic traits that could be lost due to environmental changes or extinction.
- Biomass Production:
- Tissue culture enhances biomass production by optimizing culture conditions and nutrient media. Increased biomass yields are achievable through this technique, making it applicable in fields that require high plant biomass for commercial use.
Secondary Metabolite Production
Secondary metabolites, recognized for their significant economic and pharmaceutical value, are by-products generated by plants during metabolism. These compounds, comprising less than 1% of the plant’s dry weight, exhibit considerable variation depending on the plant’s developmental stage and physiological condition. With approximately 25% of pharmaceutical compounds sourced from plants and over 11% of essential drugs derived exclusively from flowering plants, the demand for efficient production methods for these metabolites is paramount. In this context, plant tissue culture has emerged as a pivotal technique for enhancing the production of secondary metabolites.
- Historical Background: The concept of secondary metabolites dates back to 1873 when Sach identified that plants produce various metabolic by-products. These secondary metabolites are of great interest due to their medicinal properties.
- Importance of Secondary Metabolites:
- These compounds play a crucial role in plant defense, pigmentation, and interaction with the environment.
- The medical relevance of secondary metabolites has led to extensive in-vitro studies aimed at optimizing the production of commercially valuable chemicals.
- Role of Tissue Culture:
- Tissue culture techniques have been instrumental in amplifying the production of secondary metabolites. They enable consistent and controlled conditions that are conducive to maximizing yield.
- The process not only circumvents seasonal fluctuations and variations in quality but also offers a sustainable alternative to protect endangered plant species.
- Examples of Secondary Metabolites:
- Noteworthy metabolites obtained from plant tissue cultures include:
- Vincristine and Vinblastine from Catharanthus roseus
- Paclitaxel (Taxol), podophyllotoxin, camptothecin, morphine, and diosgenin are among other significant compounds sourced from cultured plants.
- Noteworthy metabolites obtained from plant tissue cultures include:
- Advantages of Plant Cell Cultures:
- Shortens the maturity time required for plants to produce secondary metabolites.
- Reduces the quantity of plant material needed for large-scale production.
- Facilitates rapid production of significant quantities of valuable compounds.
- Aids in the preservation of valuable natural resources.
- Suspension cultures can enhance yield for desired products.
- Offers the potential to discover novel compounds through metabolic engineering, metabolite mapping, and recombinant DNA technology.
- Allows the conversion of specific substrates into more valuable products via enzymatic activities.
- Notable Examples of Secondary Metabolite Production:
- Increased nicotine production in Nicotiana tabacum.
- Enhanced levels of anthocyanins in Euphorbia milii.
- Production of anthraquinones in Morinda citrifolia, Crocus sativa, Asperula, Galium, and Sherardia.
- Generation of shikonins in Lithospermum erythrorhizon.
- Production of phenolic compounds in Acer pseudoplatinus.
- Synthesis of L-hydroxyquiphenylalanine (L-Dopa) in Stizolobium hassjoo.
- Creation of ferruginol in Salvia miltorrhiza.
- Sanguinarine produced from Papaver somniferum.
- Ajmalicine and serpentine sourced from Catharanthus roseus.
- Rosmarinic acid from Coleus blumei.
- Diosgenin produced by Dioscorea deltoids.
- Ginseng sourced from Panax ginseng.
- Commercial Production via Bioreactors:
- The large-scale production of secondary metabolites and industrially valuable chemicals can be efficiently achieved using bioreactor systems. These systems provide a controlled environment for biological reactions and the production of desired compounds.
- Bioreactors allow for the cultivation of microorganisms or biochemically active substances, facilitating optimized chemical processes.
- Commercially Important Secondary Metabolites:
- Table 12.1 lists several key secondary metabolites produced through tissue culture, including:
- Vasine from Adhatoda vasica
- Artemisinin from Artemisia annua
- Azadirachtin from Azadirachta indica
- Capsaicin from Capsicum annum
- Ginseng saponin from Panax notoginseng
- Podophyllotoxin from Podophyllum hexandrum
- Taxane and Paclitaxel from Taxus chinensis
- Shikonin from Lithospermum erythrorhizon
- Berberine from Coscinium fenestratum
- Sennosides from Cassia senna
- Cathin from Brucea javanica
- Ajmalicine and Vincristine from Catharanthus roseus
- Table 12.1 lists several key secondary metabolites produced through tissue culture, including:
Haploid production through androgenesis and gynogenesis
Haploids are plants that contain a single set of chromosomes, distinguishing them from the typical diploid plants, which have two sets. The process of haploid production is significant in plant breeding and genetics, as it enables the creation of homozygous lines, which are essential for breeding programs and research. This discussion will explore the two primary methods of haploid production: androgenesis and gynogenesis.
- Haploids in Nature:
- Higher plants generally exhibit diploid characteristics with two sets of chromosomes in somatic cells.
- Natural haploids can arise through parthenogenesis, a process where normal sexual reproduction malfunctions.
- These haploids are typically sterile due to their single set of chromosomes but can be converted into homozygous diploids through spontaneous or induced chromosome doubling.
- Historical Background:
- The first documented production of haploids occurred in 1921 by Bergner in Datura stramonium.
- Guha and Maheshwari further advanced the field by reporting the development of haploid embryos and plantlets from microspores of Datura innoxia.
- Androgenesis:
- Over 200 species, including major crops such as cereals, mustard, potato, and tomato, have been developed using androgenesis.
- The process begins with the excision of anthers containing microspores at the late uninucleate stage from surface-sterilized buds.
- The anthers are then cultured on nutrient media, where temperature plays a crucial role:
- A low temperature (4-5°C) is typically maintained for the first 2-3 days to enhance the androgenic response.
- In certain species, such as Brassica, a higher temperature (30-35°C) is necessary.
- The microspores undergo repeated divisions, forming multicellular structures that can develop into embryos directly or form a callus from which plants are regenerated through organogenesis or embryogenesis.
- A summary of the standard protocol for raising androgenic haploids can be referenced in associated diagrams or figures.
- Gynogenesis:
- Gynogenesis is another technique for producing haploids in vitro. This method involves culturing unfertilized ovules, which can be either egg cells (parthenogenesis) or other haploid cells of the embryo sac (apogamy).
- The process bypasses fertilization, leading to the formation of embryos directly from the cultured ovules.
- Gynobasic haploids were first developed from ovary cultures of Hordeum vulgare (barley) by San Noem in 1976.
- This technique has also been successfully applied to other cereals, including wheat, rice, maize, and crops like sugar beet, tobacco, and sunflower.
- Sterility and Diploidization:
- Both androgenesis and gynogenesis result in haploid plants that possess an incomplete set of homologous chromosomes, rendering them sterile and incapable of seed production.
- To achieve fertility, haploids must undergo a process of diploidization, resulting in fertile homozygous diploid plants.
Applications of Haploids
The following points outline the primary applications of haploids:
- Rapid Generation of Homozygous Plants:
- The production of homozygous plants can be achieved in a relatively short time compared to conventional breeding methods.
- By utilizing colchicine treatment, chromosomes can be doubled, restoring fertility to haploid plants. This process enables the development of double haploids, which have the potential to become pure breeding new cultivars.
- Detection of Recessive Mutations:
- Haploids are valuable for detecting recessive mutations that may remain unexpressed in heterozygous diploids.
- This application allows researchers to identify and study specific genetic traits that could enhance breeding programs.
- Streamlining Breeding Procedures:
- The use of haploids alleviates the need for laborious and lengthy inbreeding procedures, making breeding more efficient.
- This efficiency is particularly beneficial for breeders seeking to develop new varieties quickly.
- Gametoclonal Variation:
- In vitro androgenesis allows breeders to observe gametoclonal variation, which occurs due to recombination and segregation during meiosis.
- This insight can be used to explore genetic diversity and identify desirable traits within plant populations.
- Production of Super Males:
- Anther culture techniques contribute to the production of super males, which are male populations exhibiting desirable traits associated with male plants.
- The ability to produce these specialized male plants can enhance breeding outcomes, especially in crops where male traits are advantageous.
- Introduction of Alien Chromosomes or Genes:
- Due to their chromosomal instability, haploids can be utilized for the introduction of alien chromosomes or genes into breeding programs.
- This application enables the incorporation of beneficial traits from unrelated species, enhancing genetic diversity and resilience in crops.
- Genetic Transformation and Stress Resistance:
- Genetically transformed haploids have shown promising results by exhibiting resistance to various biotic and abiotic stresses.
- This characteristic is crucial for developing crop varieties that can withstand environmental challenges, pests, and diseases.
- https://egyankosh.ac.in/bitstream/123456789/86107/1/Unit-11.pdf
- https://byjus.com/biology/plant-tissue-culture/
- https://egyankosh.ac.in/bitstream/123456789/86108/1/Unit-12.pdf
- https://www.isaaa.org/resources/publications/pocketk/14/default.asp
- https://www.ncbi.nlm.nih.gov/pmc/articles/PMC7088179/
- https://www.apsnet.org/edcenter/disimpactmngmnt/labexercises/PlantBiotechnology/Documents/PlantTissueCulture.pdf
- https://ncert.nic.in/textbook/pdf/lebt107.pdf
Great information to me