What is Microevolution?
- Microevolution, in the realm of biology, refers to the subtle shifts in allele frequencies within a specific population. This phenomenon is distinguishable from macroevolution, which encompasses broader evolutionary changes observable between distinct species. While microevolutionary changes can manifest within a single generation, macroevolutionary alterations require extended timeframes.
- At its core, microevolution is driven by four primary mechanisms: mutation, selection (both natural and artificial), gene flow, and genetic drift. These mechanisms induce alterations in allele frequencies over relatively brief evolutionary durations, in contrast to the more prolonged changes associated with macroevolution.
- The field of population genetics offers a mathematical framework to elucidate the intricacies of microevolution. This branch of biology delves into the quantitative aspects of gene frequency changes and their implications.
- Concurrently, ecological genetics focuses on the empirical observation of microevolutionary processes in natural settings. Notably, many instances of observable evolutionary adaptations, such as antibiotic resistance in bacteria, are manifestations of microevolution.
- An allele represents one of multiple versions of a mutation at a specific chromosomal location. It is essentially a variant of a particular gene that dictates a distinct trait within a population, leading to nuanced and subtle variations.
- However, these immediate alterations may not always have a pronounced impact on subsequent generations. From the perspective of the Hardy-Weinberg equilibrium, a deviation in allele frequencies arises when any of its criteria remain unmet.
- It is imperative to understand that microevolution serves as the foundational substrate for macroevolution. The cumulative effects of microevolutionary changes can potentially catalyze speciation, thereby contributing to the broader macroevolutionary landscape.
- Thus, both microevolution and macroevolution operate through analogous mechanisms, with the primary distinction being their respective temporal scales.
- In summary, microevolution is characterized by shifts in allele frequencies within populations, driven by mechanisms such as mutation, selection, gene flow, and genetic drift. The disciplines of population genetics and ecological genetics provide the theoretical and observational insights, respectively, into this intricate evolutionary process.
Definition of Microevolution
Microevolution refers to small-scale changes in allele frequencies within a population over a relatively short period of time, driven by mechanisms such as mutation, selection, gene flow, and genetic drift.
Causes of Microevolution
1. Mutation
- Mutation is a pivotal mechanism in microevolution, representing alterations in the DNA sequence of an organism’s genome. These changes can arise from various sources, including radiation, viruses, transposons, mutagenic chemicals, and inherent errors during processes like meiosis or DNA replication.
- Specifically, during DNA replication, the polymerization of the second strand can introduce errors. Some organisms even induce mutations through cellular processes, such as hypermutation.
- The phenotypic manifestation of an organism can be influenced by mutations, particularly if they transpire within the protein-coding region of a gene. The inherent error rates during DNA replication are typically minuscule, approximately 1 error per 10 to 100 million bases, owing to the meticulous proofreading capabilities of DNA polymerases.
- In the absence of this proofreading function, error rates can surge by a thousandfold. This is evident in many viruses that depend on DNA and RNA polymerases devoid of proofreading capabilities, leading to elevated mutation rates.
- Mutagenic processes, which amplify DNA alteration rates, can be triggered by chemicals that disrupt the base-pairing structure or by UV radiation causing direct DNA damage. Even though cells possess DNA repair mechanisms to rectify mismatches and DNA breaks, these mechanisms occasionally fail, resulting in persistent mutations.
- Chromosomal crossover, a mechanism for DNA exchange and gene recombination, can also be a source of mutations if misalignments occur during meiosis. Such errors can lead to significant structural DNA changes, including duplications, inversions, deletions, or even translocations between chromosomes.
- The consequences of mutations on DNA sequences can be diverse. They might be inconsequential, modify the gene product, or render the gene non-functional. Research on Drosophila melanogaster indicates that mutations altering a gene’s protein product are predominantly detrimental. Approximately 70% of such mutations are harmful, while the rest are neutral or mildly advantageous.
- Given the potential harm of mutations, organisms have evolved DNA repair mechanisms. The ideal mutation rate for a species strikes a balance between the detrimental effects of high mutation rates and the metabolic costs of upholding mutation-reducing systems.
- RNA-based viruses exhibit rapid mutation rates, providing them an evolutionary edge, enabling them to swiftly adapt and evade host defenses, such as the human immune system.
- Gene duplication, often resulting from genetic recombination, is a crucial source for the evolution of new genes. Such duplications provide raw material for the emergence of novel genes, with gene families expanding over time. New genes can originate from the duplication and subsequent mutation of ancestral genes or by merging parts of different genes, leading to new functional combinations.
- In conclusion, mutation, a fundamental driver of microevolution, arises from various sources and can have diverse impacts on an organism’s genome. Whether through errors in replication, external mutagenic agents, or chromosomal rearrangements, mutations provide the genetic variability essential for evolution.
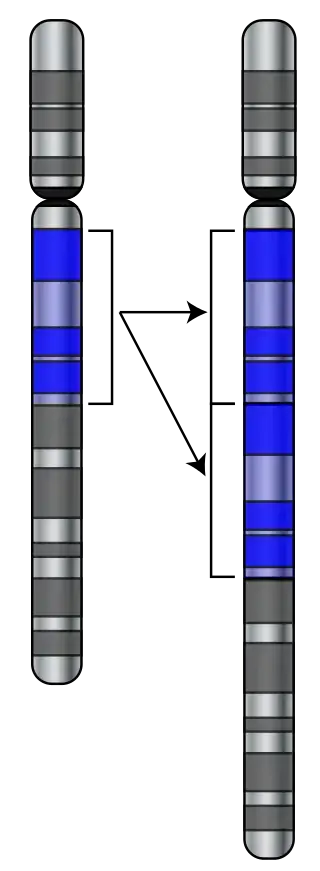
2. Selection
- Selection is a central mechanism in evolutionary biology, dictating the prevalence of heritable traits that enhance an organism’s likelihood of survival and successful reproduction. Over multiple generations, these beneficial traits become increasingly predominant within a population.
- There exists a nuanced distinction between natural selection, which occurs spontaneously in nature, and artificial selection, where humans intentionally select for specific traits. Despite the subtle differences, natural selection remains the predominant force driving evolutionary changes.
- Inherent genetic diversity within a population ensures that certain individuals are better adapted to their environment, leading to differential survival rates. This variation is further influenced by factors impacting reproductive success, a concept extensively explored by Charles Darwin in his discussions on sexual selection.
- While natural selection operates on an organism’s phenotype, it’s the heritable genetic foundation of these observable traits that, when advantageous, becomes more prevalent within a population. Over extended periods, the cumulative effects of natural selection can foster adaptations that finely tune organisms to specific ecological roles, potentially leading to the emergence of new species.
- Darwin, in his seminal 1859 work “On the Origin of Species,” introduced the concept of natural selection, drawing parallels with artificial selection where humans selectively breed organisms for desired traits. It’s noteworthy that during Darwin’s era, the principles of genetics were yet to be elucidated.
- However, the subsequent integration of Darwin’s evolutionary theories with advancements in classical and molecular genetics gave rise to the modern evolutionary synthesis. This synthesis underscores natural selection as the principal mechanism underpinning adaptive evolution in contemporary biological understanding.
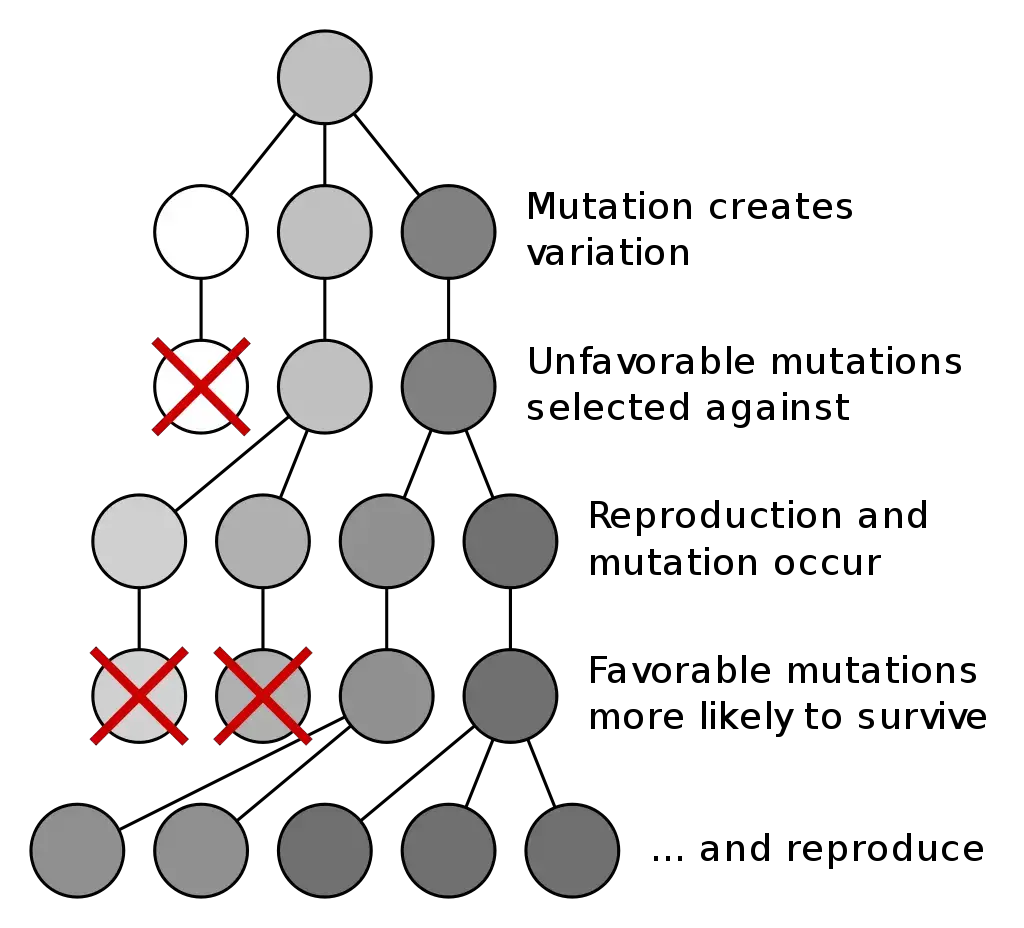
3. Genetic drift
- Genetic drift is an intrinsic evolutionary mechanism characterized by random fluctuations in allele frequencies within a population. Unlike natural selection, which operates based on the reproductive success of specific alleles in response to environmental factors, genetic drift is governed purely by chance. Specifically, the alleles present in offspring are a stochastic sample of those found in their parental generation.
- The concept of allele frequency pertains to the proportion of a specific allele variant in relation to the total number of alleles of that gene within a population. Genetic drift can lead to the complete elimination of certain gene variants, thereby diminishing the genetic diversity of the population.
- The ramifications of genetic drift are more pronounced in smaller populations, where random events can have a disproportionate impact on allele frequencies. Conversely, in larger populations, the effects of genetic drift are typically more subdued due to the sheer number of individuals and alleles present.
- The role and significance of genetic drift in the evolutionary process have been subjects of extensive debate among scientists. Historically, Ronald Fisher posited that genetic drift held minimal evolutionary significance, a perspective that dominated scientific thought for many years. However, in 1968, Motoo Kimura reignited the discourse with his neutral theory of molecular evolution. Kimura’s theory proposed that the majority of genetic changes arise from genetic drift rather than adaptive processes. Nonetheless, recent genomic data suggests that neutral alleles’ frequencies are influenced more by selection at linked sites than by the sampling errors associated with genetic drift.
- In essence, while genetic drift operates independently of adaptive pressures and can be beneficial, neutral, or detrimental, its influence on evolutionary trajectories, especially in smaller populations, cannot be understated.
4. Gene flow
- Gene flow, also known as gene migration, refers to the transfer of genetic material or alleles between distinct populations, typically within the same species. This exchange plays a pivotal role in maintaining genetic diversity and influencing the genetic makeup of populations.
- Gene flow can occur through various mechanisms. Within species, it can result from the migration and subsequent reproduction of organisms or the transfer of pollen between plants. In more complex scenarios, gene transfer can occur between different species, leading to hybrid organisms or through horizontal gene transfer, a phenomenon most common among bacteria.
- Factors such as geographical barriers, like mountains, oceans, deserts, or even human-made structures, can impede gene flow. For instance, the Great Wall of China has been observed to restrict plant gene migration. However, when barriers are absent or permeable, migration can lead to significant changes in allele frequencies within populations. Immigrants can introduce new genetic variants, while emigrants can remove them.
- In certain instances, closely related species might interbreed, producing hybrids like mules from mating horses and donkeys. While such hybrids are often infertile due to chromosomal mismatches during meiosis, they can occasionally be viable and exhibit new or intermediate phenotypes. In plants, hybridization is a notable speciation mechanism, especially since plants can more readily tolerate polyploidy (possessing multiple chromosome sets). This polyploidy in hybrids facilitates reproduction and increases genetic diversity, offering a buffer against inbreeding depression.
- Horizontal gene transfer, especially prevalent among bacteria, involves the direct transfer of genetic material between organisms, bypassing traditional reproduction. This mechanism has significant implications in medicine, particularly in the spread of antibiotic resistance among bacteria. Moreover, horizontal gene transfer isn’t limited to bacteria; evidence suggests such transfers from bacteria to eukaryotes, including yeasts and certain beetles. Viruses, too, can facilitate DNA transfer across diverse organisms, transcending biological domains.
- In essence, gene flow serves as a conduit for the exchange of genetic material, either within or between species. Factors like mobility and geographical barriers influence its rate and extent. While gene flow can counteract speciation by merging diverging gene pools, its absence can promote genetic divergence and potentially lead to speciation.
Difference between microevolution and macroevolution
Microevolution and macroevolution are both fundamental concepts in evolutionary biology, but they operate on different scales and lead to distinct biological outcomes. Here’s a detailed comparison based on the provided content:
Attributes | Microevolution | Macroevolution |
---|---|---|
Evolutionary changes | Changes occur on a small scale within a specific single population. | Changes transpire on a large scale, extending beyond the confines of a single species. |
Leads to | Results in alterations to the gene pool, causing intraspecies genetic variations. | Culminates in the formation of new species. |
Genetic data | Genetic information is rearranged or modified. | New species emerge due to structural changes in genes, such as deletions or additions. |
Time frame | Evolutionary changes manifest over relatively short time spans. | Evolutionary shifts unfold over extended periods, spanning many generations. |
Example | The emergence of new flu virus strains or the variation observed in the peppered moth. | Evolutionary milestones like the development of feathers or the transition from invertebrates to vertebrates. |
In essence, while microevolution focuses on genetic variations within a specific population over shorter time frames, macroevolution examines broader evolutionary trends leading to the emergence of new species over longer durations. Both concepts, though distinct, are interconnected, with microevolutionary changes potentially accumulating over time to drive macroevolutionary shifts.
Examples of Microevolution
- Pesticide Resistance in Insects: Pesticides are formulated to exterminate insects that pose threats to plants. However, with each pesticide application, a subset of insects may exhibit resistance due to specific genetic traits. These resistant individuals survive, reproduce, and consequently amplify the frequency of the resistance-conferring genes within the population. Over time, with repeated pesticide applications, the entire insect population may become resistant, necessitating the development of a new pesticide. The microevolutionary mechanisms at play in this scenario include natural selection and genetic drift, particularly through a bottleneck effect.
- Evolution of Viral Diseases: Viruses exhibit unique characteristics that make them prime candidates for rapid microevolutionary changes. A distinguishing feature of some viruses is their reliance on RNA instead of DNA for storing genetic information. RNA-based replication is prone to higher mutation rates than DNA, leading to frequent generation of novel genes. These new genes, if advantageous, can proliferate through natural selection. Coupled with their vast population sizes and brief generation intervals, viruses can swiftly disseminate beneficial mutations across populations. Furthermore, certain viruses, like influenza and HIV, can undergo recombination, wherein they exchange genetic material with other viral strains. This process can give rise to entirely new viral variants, enhancing the genetic diversity within the viral population. The rapid microevolution observed in viruses, driven by their elevated mutation rates, poses challenges in medical treatments, as viral populations can swiftly evolve resistance to therapeutic drugs.
In summary, microevolution, observable in phenomena like pesticide resistance in insects and the evolution of viral diseases, underscores the dynamic interplay of genetic variations and environmental pressures. These examples highlight the adaptive responses of organisms to changing environments, driven by mechanisms like natural selection and genetic drift.
Quiz
What is microevolution primarily concerned with?
a) Changes in allele frequencies within a population over time.
b) The origin of new species.
c) The history of life on Earth.
d) The study of fossils.
Which of the following is NOT a mechanism of microevolution?
a) Genetic drift
b) Gene flow
c) Natural selection
d) Cellular respiration
Pesticide resistance in insects is an example of which microevolutionary mechanism?
a) Mutation
b) Genetic drift
c) Natural selection
d) Gene flow
Which factor can increase the impact of genetic drift?
a) Large population size
b) Small population size
c) High mutation rate
d) Stable environment
What is the primary cause of gene flow?
a) Mutation
b) Selection
c) Migration
d) Genetic recombination
Which of the following can decrease genetic variation in a population?
a) Mutation
b) Genetic drift
c) Gene flow
d) Natural selection
In which scenario is microevolution most likely to occur rapidly?
a) Stable environment with no changes
b) Large population with no migration
c) Small population with a bottleneck event
d) Absence of mutations
Which of the following is a potential outcome of microevolution?
a) Extinction of a species
b) Changes in individual behaviors
c) Alterations in allele frequencies
d) Formation of a new kingdom
Which process can introduce new genetic material into a population’s gene pool?
a) Genetic drift
b) Mutation
c) Natural selection
d) Stabilizing selection
In the context of microevolution, what does the Hardy-Weinberg equilibrium describe?
a) A population evolving rapidly
b) A population with changing allele frequencies
c) A population not undergoing evolution
d) A population with high mutation rates
FAQ
What is microevolution?
Microevolution refers to small-scale changes in allele frequencies within a population over a few generations, without the formation of new species.
How is microevolution different from macroevolution?
While microevolution focuses on genetic changes within a single population, macroevolution examines broader evolutionary changes that lead to the emergence of new species over longer time spans.
What are the primary mechanisms driving microevolution?
The main mechanisms include natural selection, genetic drift, gene flow (migration), and mutation.
Can microevolution lead to the formation of new species?
Microevolution itself doesn’t lead to new species. However, accumulated microevolutionary changes over long periods can contribute to macroevolutionary processes, potentially leading to speciation.
Why is the study of microevolution important?
Understanding microevolution provides insights into how populations adapt to changing environments, which has implications for conservation, agriculture, and medicine.
Is microevolution observable?
Yes, microevolution can be observed and measured over short periods, even within a few generations, such as in the case of pesticide-resistant insects.
How does genetic drift contribute to microevolution?
Genetic drift, a random change in allele frequencies due to chance events, can lead to certain alleles becoming more common or disappearing entirely, especially in small populations.
What role does mutation play in microevolution?
Mutations introduce new genetic variations into a population. If these mutations confer an advantage, they may increase in frequency through natural selection.
Can human activities influence microevolution?
Yes, activities such as selective breeding, habitat destruction, and the use of pesticides can drive microevolutionary changes in various species.
What is the significance of the Hardy-Weinberg equilibrium in microevolution?
The Hardy-Weinberg equilibrium describes a theoretical population where allele frequencies do not change, meaning the population is not evolving. It provides a baseline to compare with real populations to determine if microevolution is occurring.
References
- Population Genetics (2017) University of Miami. Retrieved from:http://www.bio.miami.edu/tom/courses/bil160/bil160goods/04_popgen.html
- University of California Museum of Paleontology. (2017) Mechanisms of microevolution. In Understanding Evolution. Retrieved from http://evolution.berkeley.edu/evolibrary/article/0_0_0/evo_39
- Evolutionary Biology/Microevolution (2013) Wikibooks. Retrieved from:https://en.wikibooks.org/wiki/Evolutionary_Biology/Microevolution
- Manrubia, S. Lázaro (2006) Viral evolution. Physics of Life Reviews. 2006. 65-92
- Evolution of viruses / viruses. (2017) Khan Academy. Retrieved from:https://www.khanacademy.org/science/biology/biology-of-viruses/virus-biology/a/evolution-of-viruses
- Duffy, S. Shackelton, L. Holmes, E. (2008) Rates of evolutionary change in viruses: patterns and determinants. Nature Reviews Genetics. 9: 267-276