What is Cell Cycle?
- The cell cycle, also known as the cell-division cycle, is a complex sequence of events that enables a cell to grow and divide, resulting in two daughter cells. This cycle is fundamental for growth, development, and tissue repair in living organisms. It encompasses several critical processes, including cell growth, DNA replication, and the division of cellular components.
- In eukaryotic cells—those possessing a defined nucleus, such as those in animals, plants, fungi, and protists—the cell cycle is predominantly categorized into two principal phases: interphase and the mitotic (M) phase. Interphase serves as a preparatory stage, where the cell undergoes growth and accumulates the necessary nutrients and energy for the impending division. During this phase, the cell also duplicates its DNA, ensuring that each daughter cell inherits a complete set of genetic material.
- Following interphase, the cell enters the M phase, which consists of mitosis and cytokinesis. Mitosis is the process where replicated chromosomes are evenly distributed into two new nuclei, while cytokinesis involves the physical separation of the cytoplasm and organelles, culminating in the formation of two distinct daughter cells. This orderly progression through the cell cycle is meticulously regulated by various control mechanisms, known as cell cycle checkpoints, which assess the integrity and readiness of the cell to proceed to the next phase.
- In contrast, prokaryotic cells—such as bacteria and archaea—exhibit a different cell cycle structure. Their cycle comprises three main periods: B, C, and D. The B period spans from the conclusion of cell division to the onset of DNA replication. During the C period, the actual replication of DNA occurs, followed by the D period, which encompasses the time from the completion of DNA replication to the division of the prokaryotic cell into two daughter cells.
- For unicellular organisms, the cell cycle is vital for reproduction and the continuation of species. In multicellular organisms, such as plants and animals, multiple cell-division cycles facilitate development from a fertilized egg into a fully formed organism. This process also supports ongoing regeneration and repair of tissues, allowing for the replacement of cells in skin, blood, and various organs, except for certain specialized cells, like neurons, which exhibit limited regenerative capabilities.
- Although the distinct phases of interphase—G1 (first gap), S (synthesis), and G2 (second gap)—are not typically distinguishable under a microscope, each phase plays a crucial role in preparing the cell for division. G1 involves cellular growth and metabolism, S is characterized by DNA synthesis, and G2 prepares the cell for mitosis. Each of these stages includes specific biochemical processes that ensure proper cellular function and readiness for division.
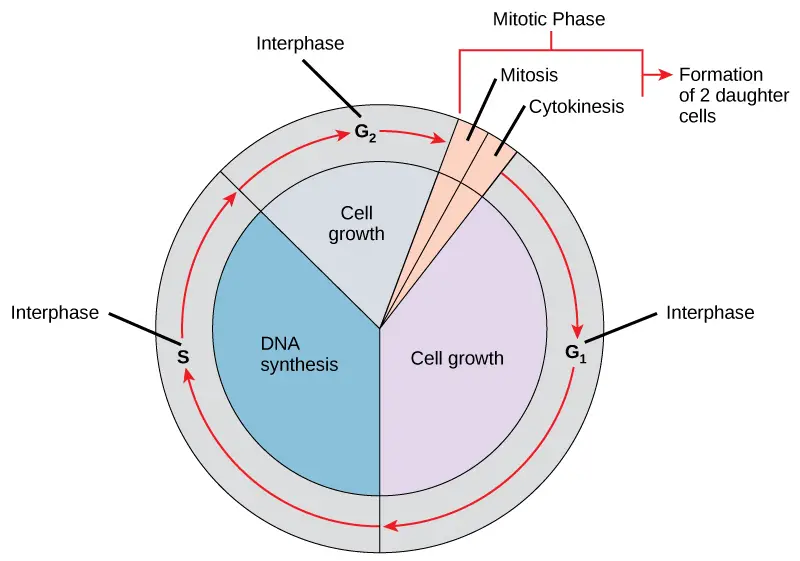
Definition of Cell Cycle
The cell cycle is a series of sequential events that occur in a cell, leading to its growth, DNA replication, and division into two daughter cells. It consists of interphase, where the cell prepares for division, and the M phase, which includes mitosis and cytokinesis. This process is crucial for growth, development, and tissue repair in organisms.
Phases of Cell Cycle
The cell cycle comprises a series of phases that govern the growth, replication, and division of cells. This cycle is crucial for cellular reproduction, development, and tissue maintenance across various organisms. A typical eukaryotic cell cycle includes two main phases: interphase and the mitotic (M) phase.
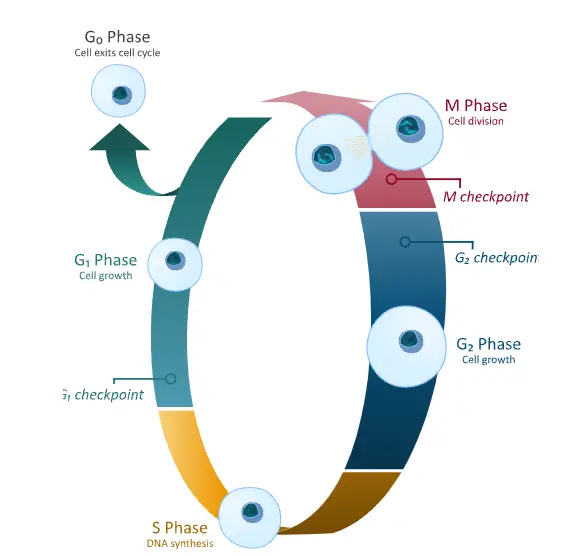
- Interphase
- Interphase represents the preparatory phase of the cell cycle, during which the cell undergoes growth and DNA replication, occupying approximately 95% of the total cycle time.
- G1 Phase (Gap 1)
- This phase occurs immediately after mitosis. The cell is metabolically active, growing, and performing its regular functions without DNA replication.
- During G1, cells accumulate the resources needed for DNA synthesis, preparing for the next stage.
- S Phase (Synthesis)
- The S phase is characterized by the replication of DNA. Each chromosome is duplicated, resulting in two sister chromatids connected at the centromere.
- The centrosome also duplicates during this phase, providing essential components for the mitotic spindle required for chromosome segregation.
- G2 Phase (Gap 2)
- In G2, the cell continues to grow and synthesizes proteins and other macromolecules necessary for mitosis.
- This phase includes the replication of organelles and the disassembly of the cytoskeleton, preparing the cell for the division process.
- M Phase (Mitotic Phase)
- The M phase involves both mitosis and cytokinesis, where the cell divides its duplicated genetic material and cytoplasm into two daughter cells.
- Mitosis
- Mitosis consists of several stages: prophase, prometaphase, metaphase, anaphase, and telophase.
- Prophase: The nuclear envelope begins to disintegrate, the chromatin condenses into visible chromosomes, and the mitotic spindle starts to form.
- Prometaphase: The nuclear envelope fully breaks down, and spindle fibers attach to the kinetochores on the chromosomes.
- Metaphase: Chromosomes align at the metaphase plate, ensuring that each sister chromatid is positioned for separation.
- Anaphase: Sister chromatids are pulled apart toward opposite poles of the cell, becoming individual chromosomes.
- Telophase: Chromosomes decondense back into chromatin, and the nuclear envelope re-forms around each set of chromosomes.
- Mitosis consists of several stages: prophase, prometaphase, metaphase, anaphase, and telophase.
- Cytokinesis
- Cytokinesis occurs concurrently with telophase, marking the final separation of the cytoplasm.
- In animal cells, a contractile ring forms and pinches the cell membrane, creating a cleavage furrow that divides the cell.
- In plant cells, the Golgi apparatus produces vesicles that coalesce at the metaphase plate to form a cell plate, which ultimately develops into a new cell wall.
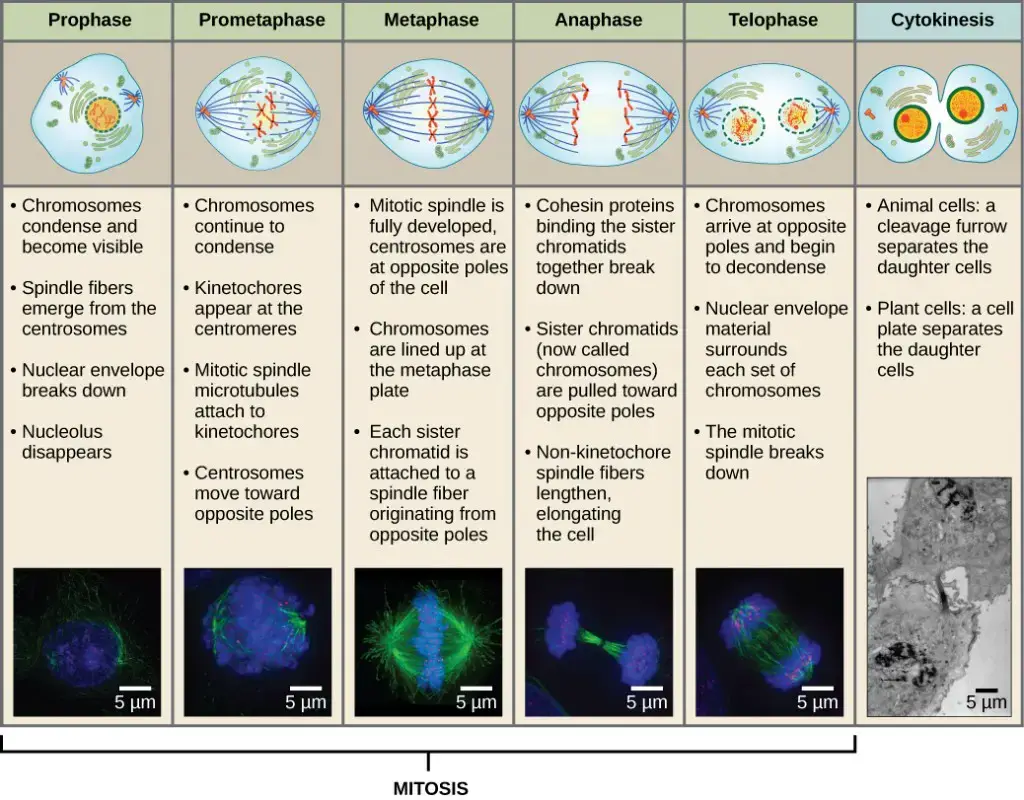
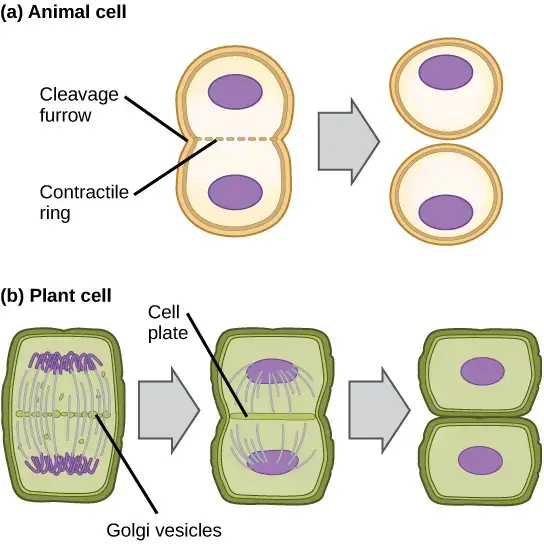
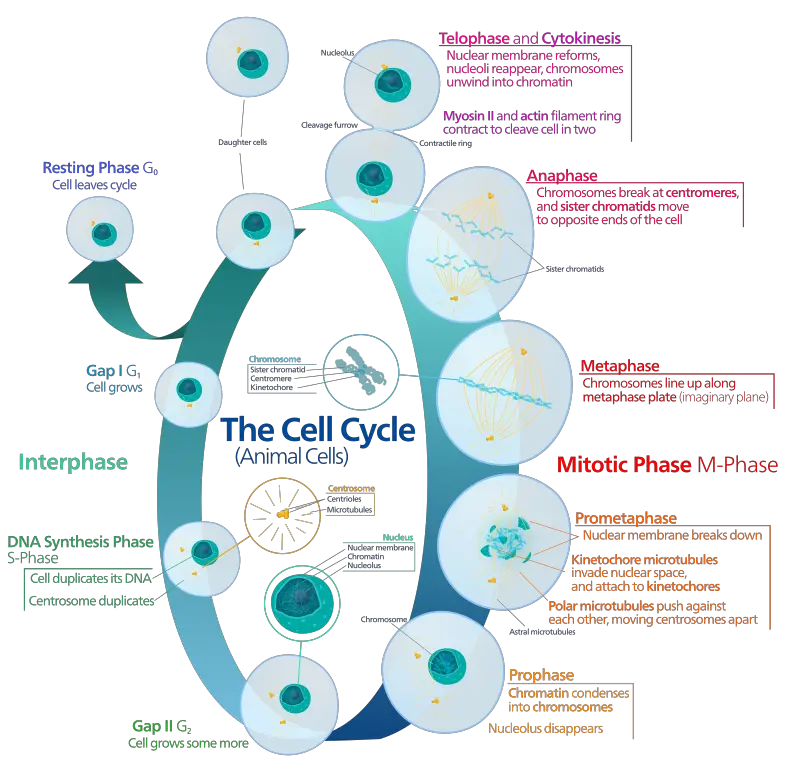
What is G0 Phase?
- Not all cells follow the conventional cell-cycle pattern, which calls for a freshly produced daughter cell to enter interphase right away and then quickly enter the mitotic phase.
- The G0 phase is when a cell is not actively preparing to divide.
- The cell has completed its cell cycle and is now in a quiescent (inactive) stage. Some cells briefly enter G0 before the start of G1 is signalled by an outside source.
- Other cells, such as mature heart muscle and nerve cells, that never or infrequently divide always remain in the G0 state.
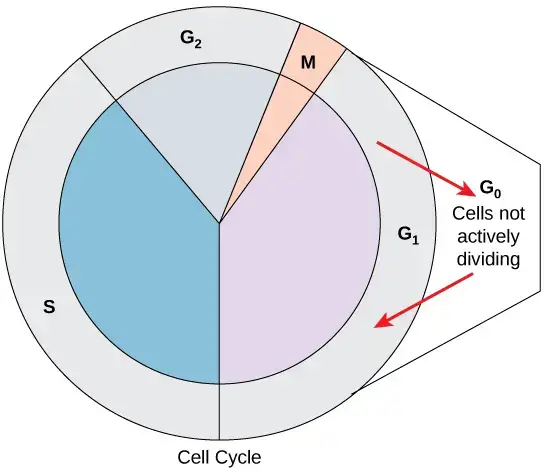
Checkpoints in the Cell Cycle
Checkpoints in the cell cycle serve as critical regulatory mechanisms that ensure cells progress through the cycle accurately and only when conditions are favorable. These checkpoints prevent errors in cell division, which can lead to issues such as cancer or developmental defects. The three primary checkpoints—G1, G2, and M—are strategically positioned to monitor different phases of the cycle, each focusing on distinct criteria essential for healthy cellular function.
- G1 Checkpoint (Restriction Checkpoint):
- Location: This checkpoint occurs at the end of the G1 phase, just before the cell transitions into the S phase.
- Function: The G1 checkpoint is pivotal as it determines whether the cell is ready to divide. It assesses several critical factors:
- Cell Size: The cell evaluates if it has reached a sufficient size to support division.
- Nutrient Availability: The presence of adequate energy reserves and nutrients is crucial for successful division.
- Growth Factors: Cells check for external signals, such as growth factors, that indicate a supportive environment for division.
- DNA Integrity: Any damage to the DNA is scrutinized, as uncorrected damage can lead to problems in daughter cells.
- Outcome: If the cell meets all criteria, it commits to the cycle and enters the S phase irreversibly. However, if it fails to meet any of these conditions, the cell may exit into a resting state known as G0, where it can remain until conditions improve.
- G2 Checkpoint:
- Location: Situated at the transition between the G2 phase and the M phase, this checkpoint acts as a safeguard before mitosis.
- Function: The G2 checkpoint primarily ensures the fidelity of DNA replication:
- DNA Damage Assessment: The cell checks for any potential damage to its DNA.
- Replication Completeness: It verifies that DNA replication has been completed correctly during the S phase.
- Outcome: If the DNA is found to be damaged or incompletely replicated, the cell will halt the cycle, allowing time for repair. Should the damage be deemed irreparable, the cell may undergo apoptosis, effectively preventing the transmission of faulty genetic material to daughter cells.
- M Checkpoint (Spindle Checkpoint):
- Location: This checkpoint occurs during the M phase, specifically at the transition from metaphase to anaphase.
- Function: The M checkpoint ensures proper chromosomal segregation by examining:
- Chromosome Attachment: The cell verifies that all sister chromatids are correctly attached to the spindle apparatus.
- Correct Positioning: It monitors for any misaligned chromosomes that may not be properly engaged with spindle microtubules.
- Outcome: If any chromosome is not attached correctly, the cell will pause mitosis, providing time for the spindle apparatus to capture and align the mispositioned chromosomes. This precaution is crucial as the separation of sister chromatids is an irreversible step.
Cell Cycles in Vivo
Within a multicellular plant or animal, the ability of different cell types to grow and divide is one of the characteristics that sets them apart from one another. Cells can be divided into three general categories:
- neurons, muscle cells, or red blood cells are examples of highly specialised cells that cannot divide. These cells stay in their differentiated condition till they pass away.
- cells that ordinarily do not divide, but when given the right stimuli, can be made to start synthesising DNA and divide. This group includes lymphocytes and liver cells, which can both be stimulated to multiply by interacting with the right antigen or by surgically removing a portion of the liver.
- cells with a typically moderately high mitotic activity level. This group includes stem cells from several adult tissues, including hematopoietic stem cells, which develop into red and white blood cells, and stem cells at the base of multiple epithelia, which cover the surface and cavities of the body. Rapid and ongoing cell division is also seen in the comparatively unspecialized cells of apical meristems found close to the terminals of plant roots and stems. The ability to divide asymmetrically is a crucial trait that stem cells possess that is not shared by most other cells. When a cell divides asymmetrically, the two daughter cells have different shapes, makeups, or destiny. One daughter cell that results from the asymmetric division of a stem cell is still an uncommitted stem cell like its parent, and the other daughter cell has begun the process of differentiating into a cell of that tissue. In other words, stem cells can engage in both self-renewal and the development of differentiated tissue cells through asymmetric divisions.
Cell cycles in cleaving frog embryos, which lack both G 1 and G 2 phases, can last only 30 minutes, whereas those in slowly developing tissues, such the mammalian liver, can last many months.
Many cells in the body are considered to be quiescent, which means they are not currently undergoing cell division but yet have the capacity to do so if the situation warrants it. Cells that have ceased dividing are, with a few notable exceptions, stalled in a phase before DNA synthesis begins.
Control of the Cell Cycle
- Even within a single organism’s cells, the length of the cell cycle varies greatly.
- In humans, cell turnover varies from a few hours during early embryonic development to an average of two to five days for epithelial cells, or to a full human lifetime for specialised cells like cortical neurons or cardiac muscle cells, which spend their entire existence in the G0 state.
- The amount of time a cell spends in each stage of the cell cycle varies as well. The cycle lasts roughly 24 hours when fast-diverging mammalian cells are cultured in culture (outside the body under ideal growing circumstances).
- The G1 phase of rapidly dividing human cells with a 24-hour cell cycle lasts roughly 11 hours.
- Events in the cell cycle are timed according to both internal and exterior to the cell mechanisms.
Regulation at Internal Checkpoints
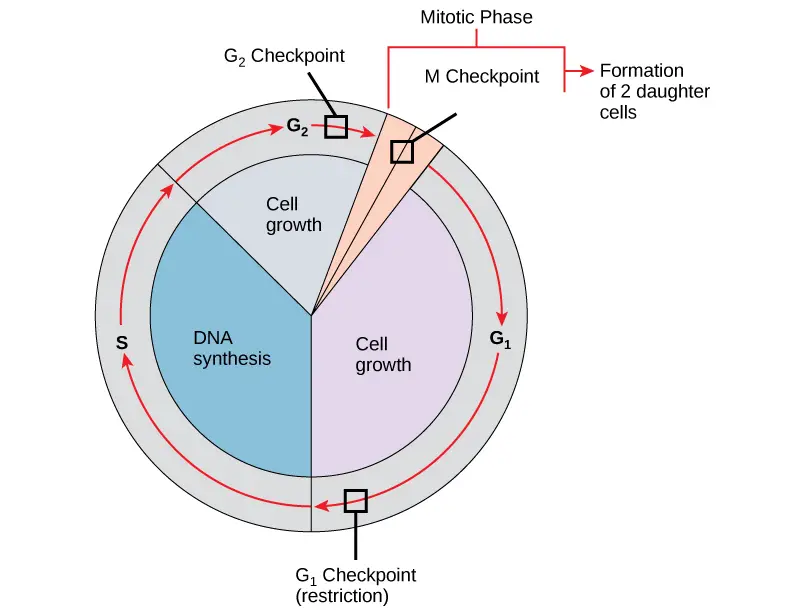
Regulation at internal checkpoints within the cell cycle is crucial for maintaining cellular integrity and ensuring the accurate replication and distribution of genetic material. These checkpoints serve as quality control mechanisms that monitor key processes and conditions, allowing cells to halt progression through the cycle when necessary. The three primary checkpoints—G1, G2, and M—each perform specific functions that safeguard the cell’s ability to produce identical daughter cells.
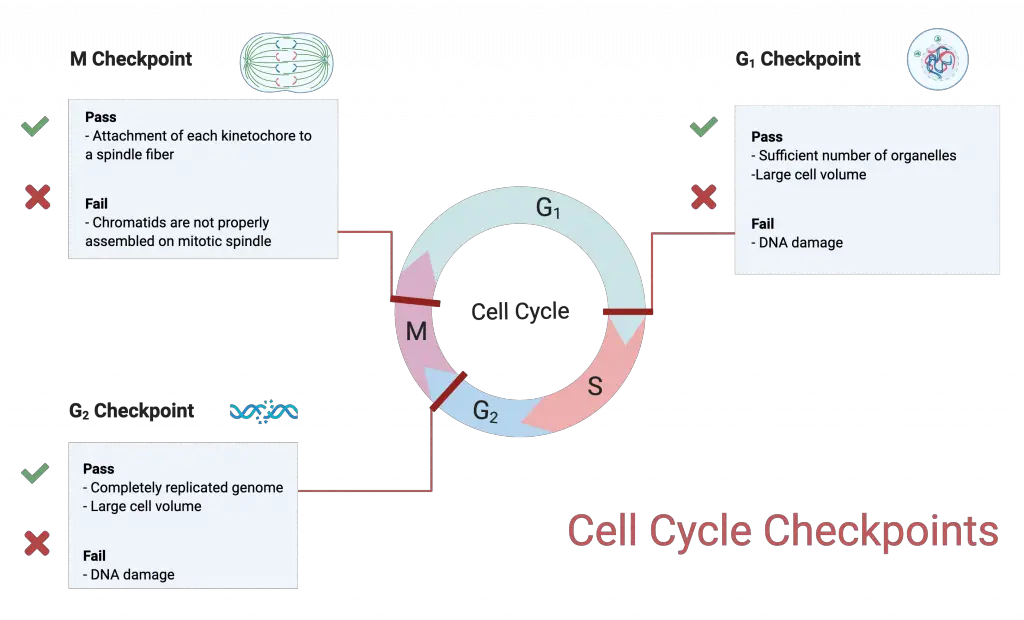
- G1 Checkpoint (Restriction Point):
- Location and Function: The G1 checkpoint occurs at the end of the G1 phase, just prior to the transition into the S phase. This is a critical decision point where the cell evaluates whether to commit to division.
- Criteria for Progression:
- Cell Size: The cell assesses whether it has attained an adequate size to divide effectively.
- Nutrient Availability: Sufficient energy reserves and nutrients are essential for the cell’s metabolic needs during division.
- External Signals: The presence of growth factors from surrounding cells influences the decision to proceed with division.
- DNA Integrity: The cell inspects its genomic DNA for damage, as any errors could jeopardize the viability of daughter cells.
- Outcome: Cells that do not meet these conditions can either attempt to repair any deficiencies or enter a quiescent state known as G0, from which they can re-enter the cell cycle when conditions improve. If all conditions are satisfied, the cell irreversibly commits to the S phase and prepares for DNA replication.
- G2 Checkpoint:
- Location and Function: The G2 checkpoint occurs at the end of the G2 phase, immediately before the cell enters mitosis (M phase). It serves as another crucial quality control point.
- Criteria for Progression:
- Cell Size and Protein Reserves: Similar to the G1 checkpoint, the cell checks its size and the availability of necessary proteins.
- DNA Replication Completeness: The primary function of the G2 checkpoint is to confirm that all chromosomes have been accurately replicated during the S phase and that there is no DNA damage.
- Outcome: If the mechanisms detect any issues, the cell cycle is halted, allowing time for the completion of DNA replication or repair of damaged DNA. In cases where the damage is irreparable, the cell may initiate programmed cell death (apoptosis) to prevent the propagation of mutations.
- M Checkpoint (Spindle Checkpoint):
- Location and Function: The M checkpoint takes place during metaphase, at the transition from metaphase to anaphase. This checkpoint focuses on the proper alignment and attachment of chromosomes.
- Criteria for Progression:
- Chromosome Attachment: The cell verifies that all sister chromatids are correctly attached to the spindle microtubules, which are crucial for their proper segregation during anaphase.
- Kinetochores Status: Each pair of sister chromatids must be anchored to at least two spindle fibers emanating from opposite poles of the cell.
- Outcome: If any sister chromatid is improperly attached, the cell will pause mitosis until all chromosomes are correctly positioned. This pause is vital, as the separation of sister chromatids is an irreversible step in the cell cycle.
The Checkpoints
The checkpoints in the cell cycle play a crucial role in ensuring the fidelity of cell division, thereby safeguarding genomic integrity and preventing oncogenesis. These checkpoints are regulatory pathways that monitor various aspects of the cell cycle, including cell size, DNA integrity, and the proper alignment of chromosomes. Understanding these mechanisms is vital for grasping how cells maintain homeostasis and how dysregulation can lead to disease.
- Cell Size Control
- Cells must double their contents to ensure that each daughter cell receives the appropriate genetic material and biosynthetic components before division.
- Cell Size Checkpoints: These checkpoints are active during the G1 and G2 phases. Research has shown that the size of daughter cells influences their progression through the cell cycle; larger cells tend to accelerate their cycle progression, while smaller cells may delay it.
- In budding yeast, the cyclin Cln3 is critical for initiating the cell cycle based on size, while in fission yeast, proteins Cdc25 and Wee1 respond to both cell size and nutritional status, regulating the Cdc2-cyclin B complex.
- Proposed Mechanisms: One hypothesis suggests that cells monitor protein translation, where ribosomal mass correlates with cell size. This “translational sizer” is thought to control cell cycle progression. The PKA and TOR signaling pathways are also implicated in linking nutrient availability to cell growth and division.
- Additionally, cell geometry may be monitored, as seen in the cylindrical shape of fission yeast, where the protein Pom1 halts cell cycle progression based on cell length.
- DNA Damage Responses
- DNA damage can arise from intrinsic sources (e.g., replication errors) or extrinsic sources (e.g., radiation). In response, cells employ checkpoints to halt the cycle and enable repair.
- Chk1 Pathway: This highly conserved pathway is activated by DNA damage, particularly during S and G2 phases. Chk1 inhibits cyclin-dependent kinases (CDKs) until the damage is repaired. It is activated by single-stranded DNA bound by Replication Protein A (RPA), recruiting proteins like ATR and the 9-1-1 complex.
- p53 Role: In higher organisms, p53 is pivotal in DNA damage responses, particularly during G1. It activates genes that inhibit CDKs, thus preventing the cycle from progressing until damage is resolved. Furthermore, p53 can dictate cell fate decisions such as apoptosis or senescence.
- Monitoring DNA Replication
- During the S phase, cells face challenges in DNA replication that require tight regulation to prevent the propagation of damage.
- Replication Origin Control: Initiation of DNA replication is strictly controlled to occur once per cell cycle through phosphorylation of regulatory proteins Cdt1 and Cdc6.
- Intra-S-phase Checkpoint: The kinases Cds1 in fission yeast and Chk2 in humans stabilize the replication machinery upon encountering obstacles, ensuring the replisome remains intact. This prevents disassociation and potential collapse of the replication fork, which could lead to genomic instability.
- S–M Dependency
- The transition from S phase to mitosis is tightly regulated to ensure complete DNA replication. If DNA damage or replication issues arise, checkpoints inhibit entry into mitosis.
- Role of Chk1 and Cds1: If replication is stalled due to DNA damage, the Chk1 pathway is activated, whereas depletion of dNTPs can also halt mitosis without activating Chk1. This complexity highlights the intertwined nature of replication stability and cell cycle progression.
- The Mitotic Spindle Checkpoint
- The proper segregation of sister chromatids during anaphase is under the mechanical control of the mitotic spindle, composed of microtubules and motor proteins.
- Spindle Checkpoint Functionality: This checkpoint prevents the activation of the Anaphase-Promoting Complex/Cyclosome (APC/C) until all kinetochores are correctly attached and under tension. The spindle checkpoint protein Mad2 inhibits Cdc20, preventing premature anaphase entry.
- The involvement of kinases like Bub1 and the regulation of the APC by Cdc20 and Cdh1 ensures the correct timing of mitosis, maintaining genomic integrity throughout cell division.
Regulator Molecules of the Cell Cycle
Regulator molecules of the cell cycle play a pivotal role in ensuring that cellular division occurs accurately and efficiently. These molecules can be categorized into two main groups: positive regulators, which promote the progression of the cell cycle, and negative regulators, which halt the cycle until specific conditions are met. Understanding the intricate interplay between these regulatory proteins is essential for comprehending how cells maintain genomic integrity and avoid uncontrolled division.
Positive Regulation of the Cell Cycle
Positive regulation of the cell cycle is essential for ensuring that cells progress through their life cycle in an orderly and timely manner. This process is primarily governed by two key groups of proteins: cyclins and cyclin-dependent kinases (Cdks). Their dynamic interactions dictate the advancement of the cell through various checkpoints, facilitating cell division and maintaining cellular integrity.
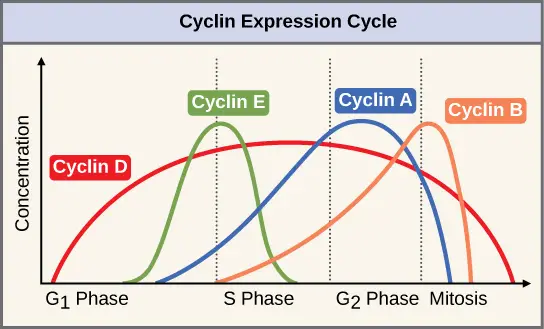
- Role of Cyclins and Cdks:
- Cyclins: Cyclins are proteins whose levels fluctuate predictably throughout the cell cycle. There are four main types of cyclins, each associated with specific phases of the cell cycle:
- Cyclin D: Levels increase during the G1 phase and decrease at the end of mitosis.
- Cyclin E: Concentrations rise during G1 and decrease during the S phase.
- Cyclin A: Levels increase during the S phase and decline during mitosis.
- Cyclin B: Concentrations rise in the S phase and fall during mitosis.
- Cdk Activation: For cyclins to effectively regulate the cell cycle, they must bind to Cdks. This interaction forms a Cdk/cyclin complex that is essential for cell cycle progression. To achieve full activation, the Cdk/cyclin complex requires additional phosphorylation at specific sites, which alters the shape of the protein and enables its functional activity.
- Cyclins: Cyclins are proteins whose levels fluctuate predictably throughout the cell cycle. There are four main types of cyclins, each associated with specific phases of the cell cycle:
- Mechanism of Action:
- Phosphorylation: Cdks act as kinases, meaning they phosphorylate other proteins, thereby activating them for roles in advancing the cell cycle. The phosphorylation event is critical, as it not only activates the target proteins but also changes their conformational structure to enable new functions. The proteins that Cdks phosphorylate are integral to moving the cell to the next phase of the cell cycle.
- Concentration Stability: While the levels of Cdk proteins remain relatively stable throughout the cycle, cyclin concentrations vary. This fluctuation is a crucial factor that determines the formation of active Cdk/cyclin complexes at specific points during the cell cycle.
- Checkpoint Regulation:
- The cyclin/Cdk complexes are responsible for regulating the three major checkpoints of the cell cycle: G1, G2, and M phases. Each complex’s formation and activity are timed with respect to the phases of the cell cycle, ensuring that each transition is adequately controlled.
- If the concentrations of fully activated cyclin/Cdk complexes fall below specific thresholds, the cell cycle cannot proceed through the checkpoints. This regulation is vital for preventing premature or uncontrolled cell division.
- Interactions with Negative Regulators:
- Although cyclins are the primary drivers of cell cycle progression, their activity can be modulated by negative regulatory mechanisms. These include molecules known as Cdk inhibitors, which prevent the full activation of Cdks under adverse conditions. These inhibitors serve as checks to the cell cycle, ensuring that cells do not proceed unless all conditions are favorable for division.
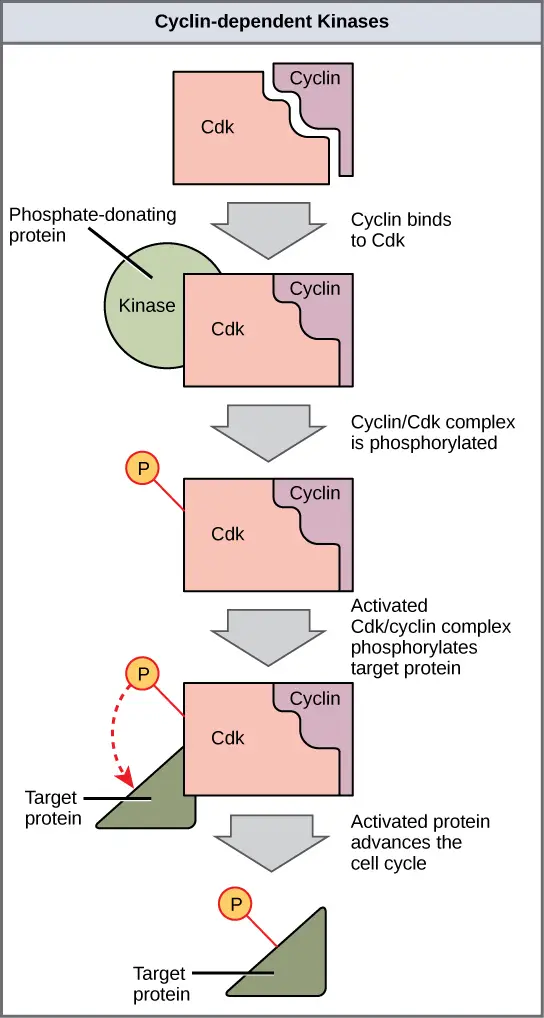
Negative Regulation of the Cell Cycle
Negative regulation of the cell cycle plays a crucial role in maintaining cellular integrity and preventing uncontrolled cell division. This regulation is primarily achieved through a set of proteins that act as checkpoints, ensuring that cells do not progress through the cycle under unfavorable conditions. The most well-studied negative regulators include retinoblastoma protein (Rb), p53, and p21, which collectively halt the cell cycle when necessary, particularly at the G1 checkpoint.
- Retinoblastoma Protein (Rb):
- Rb is a pivotal tumor-suppressor protein that monitors cell size and the overall readiness of the cell for division. In its active, dephosphorylated state, Rb binds to transcription factors, most notably E2F.
- This binding inhibits E2F’s ability to activate genes necessary for the G1/S transition, effectively blocking progression to the S phase of the cell cycle.
- As the cell grows and increases in size, Rb gradually undergoes phosphorylation. This phosphorylation leads to the inactivation of Rb, allowing it to release E2F. Consequently, E2F can then promote the transcription of proteins required for the cell to transition from G1 to S phase.
- p53 Protein:
- p53 is a multi-functional protein that serves as a crucial regulatory checkpoint in response to DNA damage. It plays a significant role in the commitment of the cell to division during the G1 phase.
- When DNA damage is detected, p53 halts the cell cycle and activates pathways that recruit enzymes responsible for DNA repair.
- If the damage is irreparable, p53 can initiate apoptosis, or programmed cell death, thereby preventing the replication of damaged genetic material. As p53 levels increase in response to cellular stress, it triggers the expression of p21.
- p21 Protein:
- p21 acts as an inhibitor of cyclin-dependent kinases (Cdks). Following its production triggered by p53, p21 binds to the active Cdk/cyclin complexes and inhibits their activity.
- This inhibition reinforces the halt in the cell cycle established by p53, ensuring that the cell does not progress into the S phase while damaged DNA is present.
- As cellular stress increases, levels of both p53 and p21 rise, further suppressing the cell’s ability to transition into DNA synthesis.
- Implications of Negative Regulation:
- The interplay between Rb, p53, and p21 forms a critical safety mechanism in cellular regulation. Each protein contributes to the comprehensive control of the cell cycle, preventing progression under harmful conditions that could lead to genomic instability or oncogenesis.
- The failure or dysfunction of these negative regulators is often implicated in the development of cancer, as their inability to properly halt the cell cycle can result in uncontrolled cellular proliferation.
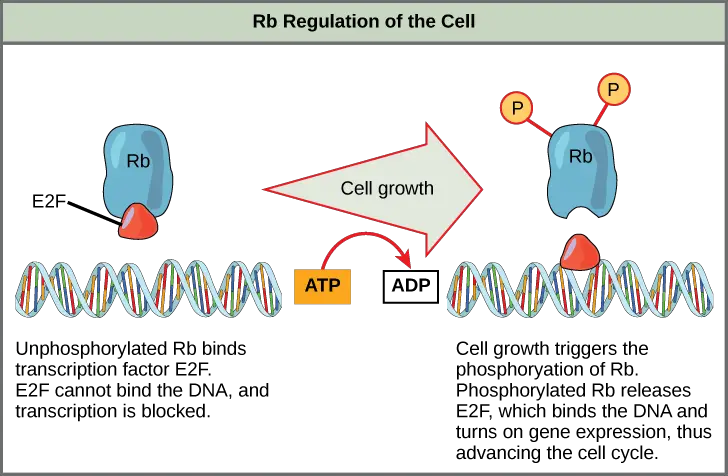
Cancer and the Cell Cycle
Cancer is characterized by uncontrolled cell growth and division, resulting from a series of genetic mutations that disrupt normal regulatory mechanisms within the cell cycle. This process is intricately linked to both positive and negative regulators of the cell cycle, which play crucial roles in maintaining cellular homeostasis. The failure of these regulatory systems leads to the transformation of normal cells into cancerous cells.
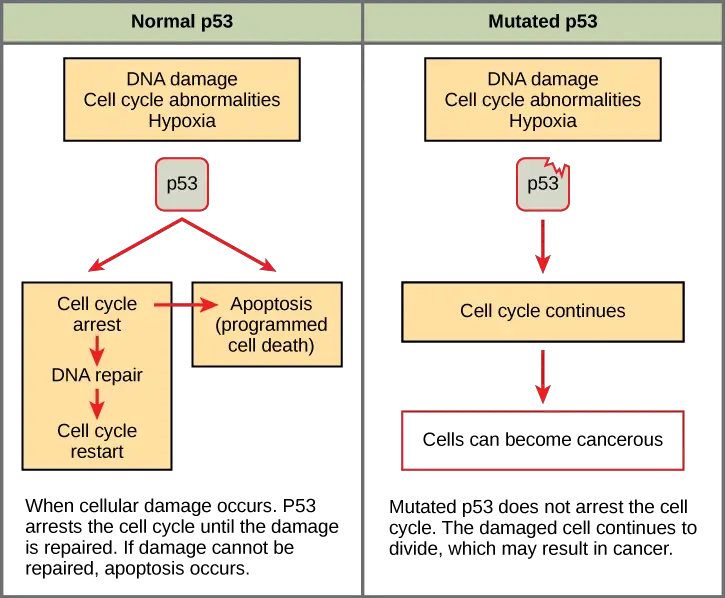
- Cell Cycle Control Mechanisms:
- The cell cycle is meticulously regulated by checkpoints, primarily during the G1, S, and G2 phases. These checkpoints ensure that cells only progress to the next phase when conditions are optimal and all necessary preparations have been made.
- However, errors can occur during these processes, particularly during DNA replication in the S phase. Even when checkpoints function correctly, some replication errors, known as mutations, can escape repair mechanisms and be passed on to daughter cells.
- Role of Gene Mutations:
- All cancers originate from mutations that lead to the production of faulty proteins involved in cell reproduction. Such mutations may affect proto-oncogenes, which are normal genes that, when mutated, can become oncogenes—genes that promote cancerous growth.
- These mutations can lead to less functional or non-functional proteins, potentially preventing the completion of the cell cycle. While this situation is often harmless, mutations can also increase the activity of positive regulators. For instance, a mutation might allow cyclin-dependent kinases (Cdks) to be activated without cyclin, thereby bypassing crucial checkpoints and allowing cells to progress prematurely through the cycle.
- Proto-Oncogenes and Oncogenes:
- Proto-oncogenes code for proteins that positively regulate the cell cycle. When these genes mutate into oncogenes, they can cause an increase in cell cycle progression rates.
- The alteration in the DNA sequence may lead to proteins that push the cell cycle forward, regardless of whether all conditions are met. If these atypical daughter cells can continue dividing, they may accumulate further mutations, amplifying the risk of cancerous transformation.
- Tumor Suppressor Genes:
- Tumor suppressor genes are critical for regulating the cell cycle by coding for negative regulatory proteins, such as retinoblastoma protein (Rb), p53, and p21. These proteins act as brakes on the cell cycle, preventing uncontrolled division until certain conditions are met.
- Mutations in these genes can compromise their function, similar to malfunctioning brakes in a vehicle. For example, more than half of all human tumors exhibit mutations in the p53 gene. This protein plays a vital role in detecting DNA damage and initiating repair processes or apoptosis if the damage is irreparable.
- Consequences of p53 Mutation:
- A defective p53 may fail to identify DNA errors, leading to the propagation of damaged genetic material through cell divisions. Even if some p53 function remains, it might not signal repair enzymes effectively. As a result, damaged DNA persists, and the cell cycle continues unabated.
- Without functional p53, the production of p21 may also decrease, which would eliminate an essential barrier against Cdk activation. Consequently, cells can bypass the G1 checkpoint and enter the S phase under suboptimal conditions, leading to the formation of daughter cells that inherit not only the mutated p53 gene but potentially other oncogenes and inactivated tumor suppressor genes.
- Tumor Growth and Accumulation of Mutations:
- As daughter cells continue to divide without the appropriate regulatory controls, they are more likely to acquire additional mutations. This accumulation can result in the rapid growth of tumors as the normal mechanisms that restrict cell division are effectively dismantled.
- Ultimately, cancer represents a culmination of genetic mutations affecting both positive and negative regulatory pathways, underscoring the delicate balance required for healthy cell cycle regulation.
The Fate of Checkpoint Dysfunction in Human Disease
Checkpoint dysfunction plays a pivotal role in the pathogenesis of various human diseases, particularly cancer. The ability of cells to monitor and respond to DNA damage and other cellular abnormalities is critical for maintaining genomic integrity. When checkpoint mechanisms fail, the consequences can range from cell death to the reprogramming of the cell cycle, ultimately leading to uncontrolled cell proliferation.
- Impact of p53 Dysfunction:
- The loss of p53, one of the most frequently mutated genes in cancer, significantly affects cell fate decisions. This loss disrupts the regulation of cyclin-dependent kinases (CDKs) by p21, which is essential for maintaining the G1 checkpoint.
- In the absence of functional p53, cells lose the ability to effectively arrest the cell cycle in response to DNA damage, which can lead to unregulated progression into the S phase. However, p53 also plays a role in directing cells toward apoptosis (programmed cell death) or senescence (a state of permanent cell cycle arrest).
- Thus, the dysfunction of p53 not only contributes to cancer development but also affects the responsiveness of cancer cells to therapeutic interventions.
- Role of the Chk1 Pathway:
- In cancer cells with defective p53, there is often an increased reliance on the Chk1 pathway, which is essential for cell viability under conditions of DNA damage. Chk1 is frequently up-regulated in various cancers, suggesting that targeting this pathway may offer therapeutic potential.
- Inhibition of Chk1 and its substrate Wee1 is being explored as a promising treatment strategy, as these pathways are crucial for controlling cell cycle progression and maintaining genome integrity.
- Consequences of Checkpoint Loss:
- In model organisms such as fission yeast, the absence of Chk1 leads to significant defects only when cells encounter external DNA damage or possess inherent DNA repair deficiencies. Without adequate checkpoint activation, cells can enter mitosis with damaged or fragmented chromosomes, leading to catastrophic outcomes such as cell death or severe chromosomal instability.
- This instability is further illustrated in mammals, where the Chk1 pathway is critical during early embryonic development. Disruption of this pathway can be lethal in certain tissues, highlighting the dependency of rapid cell division on functional checkpoint mechanisms.
- Aneuploidy and Tumorigenesis:
- Many solid tumors exhibit aneuploid karyotypes, characterized by an abnormal number of chromosomes. This aneuploidy can result from the loss or rearrangement of chromosomes, facilitating tumor suppressor gene loss and oncogene activation.
- Although mutations in spindle checkpoint genes are relatively rare in human cancers, the implications of checkpoint dysfunction can be profound. Even modest disruptions in mitotic checkpoint function can lead to severe chromosomal abnormalities, contributing to tumorigenesis.
- The Balance of Cell Cycle Regulation:
- The intricate relationship between DNA damage responses and cell cycle checkpoints emphasizes the importance of maintaining genomic integrity. High levels of DNA damage typically induce cell death; however, when checkpoints are dysfunctional, cells may continue to divide despite severe genomic alterations.
- Consequently, the dynamics of spindle microtubules and their regulation during mitosis become critical. Abnormalities in spindle function can result in chromosomal missegregation, further exacerbating genomic instability.
- Yang, N., & Sheridan, A. M. (2014). Cell Cycle. Encyclopedia of Toxicology, 753–758. doi:10.1016/b978-0-12-386454-3.00273-6
- Lew, D. J. (2013). Cell Cycle. Brenner’s Encyclopedia of Genetics, 456–464. doi:10.1016/b978-0-12-374984-0.00206-0
- Novak, Bela & Sible, Jill & Tyson, John. (2003). Checkpoints in the Cell Cycle. 10.1038/npg.els.0001355.
- Barnum KJ, O’Connell MJ. Cell cycle regulation by checkpoints. Methods Mol Biol. 2014;1170:29-40. doi: 10.1007/978-1-4939-0888-2_2. PMID: 24906307; PMCID: PMC4990352.
- Lew, D. (2001). Cell Cycle. Encyclopedia of Genetics, 286–296. doi:10.1006/rwgn.2001.1557
- Ventura, E., & Giordano, A. (2019). Cell Cycle. Reference Module in Life Sciences. doi:10.1016/b978-0-12-809633-8.90189-4
- Poon, R. Y. C. (2015). Cell Cycle Control☆. Reference Module in Biomedical Sciences. doi:10.1016/b978-0-12-801238-3.98748-8
- Alberts B, Johnson A, Lewis J, et al. Molecular Biology of the Cell. 4th edition. New York: Garland Science; 2002. An Overview of the Cell Cycle. Available from: https://www.ncbi.nlm.nih.gov/books/NBK26869/
- https://opentextbc.ca/biology/chapter/6-2-the-cell-cycle/
- https://phiab.com/resources/the-cell-cycle/
- https://www.khanacademy.org/science/ap-biology/cell-communication-and-cell-cycle/cell-cycle/a/cell-cycle-phases
- https://en.wikipedia.org/wiki/Cell_cycle
- https://reactome.org/content/detail/R-HSA-69620
- https://www.ptglab.com/products/featured-products/cell-cycle-and-checkpoint-controls/
- https://www.khanacademy.org/science/biology/cellular-molecular-biology/stem-cells-and-cancer/a/cell-cycle-checkpoints-article
- https://en.wikipedia.org/wiki/Cell_cycle_checkpoint
- https://courses.lumenlearning.com/suny-wmopen-biology1/chapter/cell-cycle-checkpoints/