A centrifuge uses centrifugal force in order to separate different components from a fluid. This is done by spinning fluid at high speeds within a container. It can then separate fluids with different densities (e.g. Cream from milk, or liquids and solids. It causes particles and substances that are denser to move in the radial direction. Conversely, objects with a lower density are displaced and moved to the centre. A laboratory centrifuge using sample tubes causes the radial acceleration to cause denser particles settle to the bottom of a tube while lower-density substances rise up to the top. A centrifuge is a powerful filter that can separate contaminants from main fluid.
In manufacturing and waste treatment, industrial centrifuges are used to remove suspended solids or separate immiscible fluids. One example of this is the cream separator used in dairy plants. Ultracentrifuges and high-speed centrifuges that can provide high accelerations are capable of separating fine particles down to the nanoscale and molecules with different masses. For example, large centrifuges can be used to simulate high acceleration or gravity environments (for pilot training). For the extraction of water from fabrics, medium-sized centrifuges can be found in washing machines as well as at swimming pools. Gas centrifuges can be used to separate isotopes, such as enriching nuclear fuel for fissile elements.
Benjamin Robins (1707-1751), an English military engineer, invented a whirling-arm apparatus to measure drag. Antonin Prandtl, an 1864 inventor of the dairy centrifuge that separates cream from milk, proposed the idea. Alexander Prandtl, his brother, put the idea into practice and displayed a functioning butterfat extraction machine in 1875.
Definition of Centrifugation
Centrifugation is a process that involves the use of a centrifuge to separate particles from a solution based on their size, shape, density, or other physical properties. A centrifuge is a device that rotates a sample at high speeds to generate a centripetal force, which causes the heavier particles to sediment to the bottom of a tube or rotor while the lighter particles remain suspended.
There are several types of centrifuges, including high-speed centrifuges, low-speed centrifuges, and ultracentrifuges. High-speed centrifuges are used to separate cells and other biological materials, while low-speed centrifuges are used to separate non-biological materials such as suspensions of crystals or inks. Ultracentrifuges are used to separate very small particles or to measure the size, shape, and density of particles.
Centrifugation is a widely used technique in research, industry, and clinical laboratories, and it has many applications, including the purification of biological samples, the separation of cells and organelles, the analysis of proteins and nucleic acids, and the preparation of diagnostic specimens.
Centrifuge definition
A centrifuge is a device that uses centrifugal force to separate particles from a solution based on their size, shape, density, or other physical properties. It consists of a motor, a rotating head or rotor, and a series of tubes or cups that hold the sample. When the rotor is rotated at high speeds, the centripetal force generated causes the heavier particles to sediment to the bottom of the tubes or cups while the lighter particles remain suspended.
There are several types of centrifuges, including high-speed centrifuges, low-speed centrifuges, and ultracentrifuges. High-speed centrifuges are used to separate cells and other biological materials, while low-speed centrifuges are used to separate non-biological materials such as suspensions of crystals or inks. Ultracentrifuges are used to separate very small particles or to measure the size, shape, and density of particles.
Centrifuges are widely used in research, industry, and clinical laboratories, and they have many applications, including the purification of biological samples, the separation of cells and organelles, the analysis of proteins and nucleic acids, and the preparation of diagnostic specimens.
What is Relative Centrifugal Force (RCF)?
- Relative centrifugal force is a measurement for the rate of strength in rotors that are of different sizes and types.
- The force is that is exerted on the inside of the rotor by the force of the rotor’s rotation.
- RCF is the force perpendicular to the surface applied to the sample, which is always in relation to the gravity of the earth.
- The RCF of different centrifuges is a good tool for analysis of rotors and allowing to select the best centrifuge to fulfill a specific task.
The formula used to calculate the force of centrifugal relative (RCF) could be written as follows:
RCF (g Force)= 1.118 × 10-5 × r × (RPM)^2
where r is the radius of the rotor (in centimeters), and RPM is the speed of the rotor in rotation per minute.
Objectives of Centrifugation
The main objectives of centrifugation are to separate particles from a solution based on their size, shape, density, or other physical properties, and to purify or isolate specific components of the sample. Centrifugation is a widely used technique in research, industry, and clinical laboratories, and it has many applications, including the following:
- Separation of cells and organelles: Centrifugation can be used to separate cells from tissue samples, or to isolate specific organelles such as mitochondria, nuclei, or ribosomes.
- Purification of biological samples: Centrifugation can be used to remove contaminants or unwanted materials from a sample, such as cells, proteins, or nucleic acids.
- Analysis of proteins and nucleic acids: Centrifugation can be used to separate proteins or nucleic acids based on their size, charge, or other physical properties, allowing for the analysis of specific molecules.
- Preparation of diagnostic specimens: Centrifugation is used in clinical laboratories to prepare diagnostic specimens, such as blood or urine, for analysis.
- Clarification of suspensions: Centrifugation can be used to remove suspended particles from a solution, resulting in a clearer sample.
- Separation of immiscible liquids: Centrifugation can be used to separate immiscible liquids, such as oil and water, based on their densities.
Centrifugation Principle/how does centrifugation work?
- The principle of centrifugation is based on the concept that particles in a solution, which have a higher density than the surrounding solvent, tend to sink or sediment, while particles with lower density tend to float or rise to the top. The speed at which the particles move is directly proportional to the difference in density. Under isopycnic conditions, where there is no difference in density, the particles remain stationary.
- Centrifugation takes advantage of these density differences to separate various particles in a solution. Instead of relying on gravity, which may be relatively weak, centrifugation employs the much stronger centrifugal force generated by a centrifuge. A centrifuge is a specialized piece of equipment designed to rotate an object around a fixed axis, applying a powerful outward force perpendicular to the axis of spin.
- The centrifuge operates based on the sedimentation principle, wherein the centripetal acceleration caused by the rotation of the centrifuge causes denser substances and particles to move outward in the radial direction. Simultaneously, objects that are less dense are displaced and move towards the center.
- In a laboratory centrifuge equipped with sample tubes, the radial acceleration exerted on the solution causes denser particles to settle at the bottom of the tube, forming a pellet, while substances with lower density rise to the top. This separation allows researchers to isolate and collect different components of the solution based on their relative densities.
- By using centrifugation, scientists and researchers can separate and purify a wide range of materials, including cells, organelles, proteins, nucleic acids, and other particles. It is a fundamental technique employed in various fields such as biology, biochemistry, chemistry, and clinical diagnostics, enabling efficient and precise separation and analysis of complex mixtures.
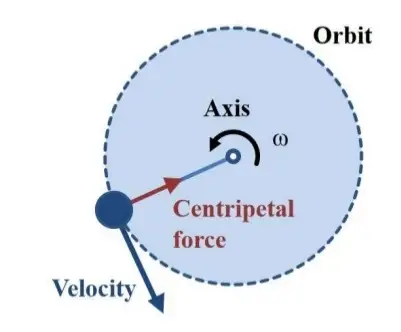
Which factors have an influence on centrifugation :
- Density of both samples as well as the solution
- Temperature/viscosity
- Displacement of particles’ distance
- The speed of rotation
Examples of centrifugation
Centrifugation finds application in various everyday processes and scientific disciplines. Here are some examples of how centrifugation is used in different contexts:
- Extraction of fat from milk: Centrifugation is employed in the production of skimmed milk. Whole milk is subjected to centrifugation, which causes the separation of the cream (containing fat) from the rest of the milk. The centrifugal force pushes the denser fat globules towards the outer edge, allowing for their removal and subsequent production of skimmed milk.
- Removal of water from lettuce: A salad spinner is a common kitchen tool that utilizes centrifugation to remove excess water from washed lettuce or other leafy vegetables. By spinning the basket containing the lettuce at high speed, the centrifugal force generated expels the water, leaving the lettuce drier and ready for consumption or further preparation.
- Spin-drying in washing machines: Washing machines incorporate a spin-drying cycle that employs centrifugation to remove water from clothing after the wash cycle. By spinning the drum at high speed, the centrifugal force extracts water from the clothes, expelling it through the drainage system. This process reduces the drying time required for the laundry.
- Separation of blood and urine materials in laboratories: Centrifugation plays a vital role in clinical and forensic laboratories for the separation of solid components from blood and urine samples. When blood or urine is subjected to centrifugation, the denser components such as red blood cells, platelets, and sedimented materials settle at the bottom of the tube, forming a pellet. The remaining liquid, known as plasma in the case of blood, or supernatant in the case of urine, is separated from the solid materials for further analysis or testing.
These examples illustrate how centrifugation is utilized in a variety of practical scenarios, ranging from food processing to laundry and scientific research. The ability of centrifugation to separate substances based on their densities makes it an essential technique in numerous industries and scientific fields.
Centrifugation Process
- The centrifuge is an container inside which an amalgamation of liquid and solid or two liquids are placed. Then, the container is turned at a very high speed. As the container rotates at high speed, the mixture is separated into its components by the effect of centrifugal force upon their density.
- The liquid or solid with greater specific gravity is thrown into the word with more force.
Sedimentation Principle of Centrifuge
In a solution, the particles with a density that is greater than that of the sink (sediment) and those which are lighter than the sink, float up to the top. The more different density, the more quickly they travel. If there isn’t any distinction between the intensity (isopyknic conditions) the particles remain in a steady state.
To make the most of tiny variations in density, to distinguish different particles within a solution gravity is replaced by the more potent “centrifugal force” that is provided by centrifuge.
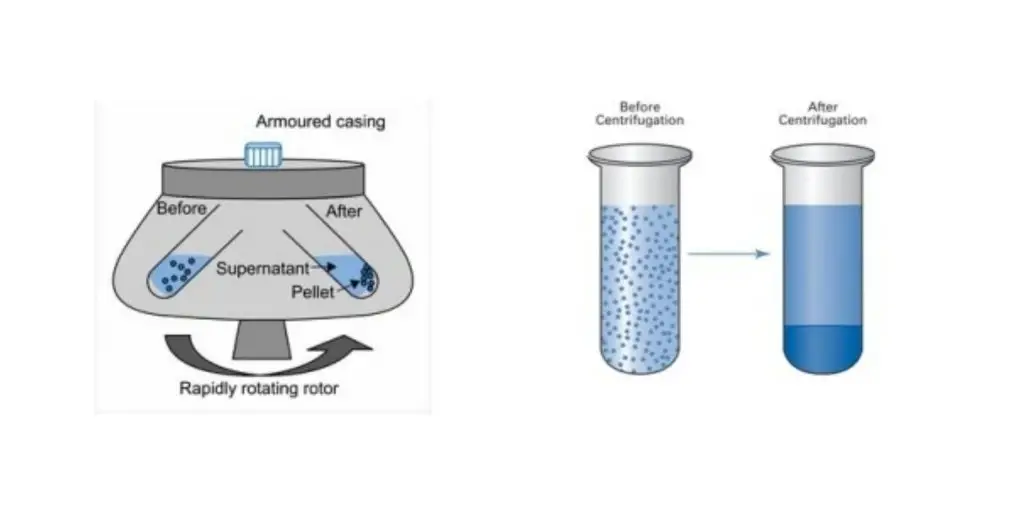
The forces works during the centrifugation
Two forces oppose the centrifugal force that acts on suspended particles:
- Buoyant force: force by which particles have to move away from the liquid medium into which they settle.
- Frictional force: force created by particles when they move throughout the liquid.
Particles are able to move off the plane of motion in the field of a centrifugal force only when the force of centrifugal forces exceeds the buoyant and frictional counteracting forces, resulting in the sedimentation of particles in a steady rate.
Relative Centrifuge Force (RCF) expressed in xg (multiple of earth gravitational force)
RFC = 1,118 x R x (rpm /1000)²
R is radius in cm
rpm : Speed in Revolutions per minute
As a result, for the same speed, a radius 20% bigger gives a number of xg 20% higher.
How to convert between times gravity (×g) and centrifuge rotor speed (RPM)?
Certain procedures require specific centrifugation parameters, which need to be defined using the term the relative centrifugal force (RCF) measured in terms of gravity units (times gravity, or grams). Most microcentrifuges are equipped with the ability to set the speed (revolutions per minute (RPM, also known as RPM) but not the absolute centrifugal force. Therefore, a formula for conversion is needed for ensuring that the correct setting is utilized in the experiment. The relation with RPM as well as RCF is as the following:
g = (1.118 × 10-5) x R x S²
In which g is the ratio of centrifugal force and it is the radius the rotor has measured in centimeters while S represents the speed in rotations per minute. The RCF values in units of the times gravity (x g) for the most common microcentrifuges rotor radius are shown in the table below. In the example below, centrifugation at 5,000 RPM inside microcentrifuge with the rotor has 7 cm radius will produce a centrifugal strength of 1 957 x grams.
Speed and duration of centrifugation typically aren’t the most important factors when it comes to routine procedures for handling samples using the use of a benchtop microcentrifuge. In general the speed and time are adequate to ensure that the cells, debris or resin are effectively pelleted It doesn’t matter whether the speed is greater or the duration is longer than the time needed. For the same reason most protocols do not define the specific centrifugal force that should be used, but rather an overall guideline, such as “centrifuge at a high speed for one minute.”
Types of Centrifuge
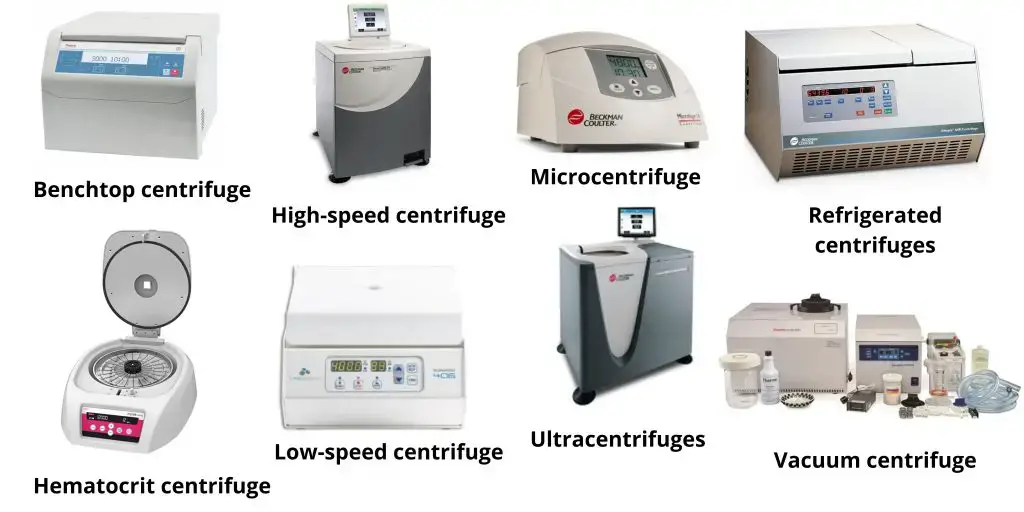
1. Low-Speed Centrifuge
- Low-speed centrifuges are the most common centrifuges commonly used in labs to perform the regular sorting of the particles.
- The centrifuges are operated at the maximum speed of 4000-5000 rpm.
- They are typically operated at ambient temperature since they don’t have control mechanisms to regulate the speed or the temperature of the process.
- Fixed angle and swinging bucket types of rotors are used in these centrifuges.
- They are compact and easy to use centrifuges, which are great for the analysis of blood samples as well as different biological sample.
- The centrifuge that operates at low speed follows the same principle that is used in all other centrifuges, however the application is limited to removal between simpler and more efficient solutions.
2. High-Speed Centrifuges
- High-speed centrifuge as the name implies is the type of centrifuge which can operate at speeds that are slightly higher.
- Speed of the centrifuge with high speed may vary between 15,000 and 30,000 rpm.
- The high-speed centrifuge is typically employed in laboratories that are more sophisticated for biochemical applications and requires a fast speed of operation.
- High-speed centrifuges have an instrument for controlling both the temperature and speed of the procedure that is crucial to analyze sensitive biological molecules.
- The high-speed centrifuges are equipped with different adapters to fit samples tubes of different dimensions and volumes.
- The three types of rotors may be used to create the centrifugation process of these centrifuges.
3. Ultracentrifuges
- Ultracentrifuges run at very high speeds which allow separation of smaller molecules like proteins, ribosomes and even viruses.
- This is the highest advanced type of centrifuge which allows to separate molecules which can’t be separated by other centrifuges.
- Refrigeration systems are found in these centrifuges to help control the heat that is generated by the spinning.
- They could be up to 150,000 rpm.
- It can be used to aid in both analytical and preparation work.
- Ultracentrifuges can be used to separate molecules in large batches , and in a continuous flow.
- Apart from separation, ultracentrifuges may also be employed for the analysis of macromolecules’ characteristics, including the shape, size, and density.
4. Microcentrifuges
- Microcentrifuges can be employed to separate small volumes of samples that range from 0.5 and 2 ul.
- Microcentrifuges usually operate at around 12,000-13,000 rpm.
- This is utilized for to separate molecularly organelles in cells like DNA, nuclei and nuclei as well as for extraction with phenol.
- Microcentrifuges also referred to as microfuge, utilize test tubes which are smaller as compared to the traditional test tubes that are utilized in larger centrifuges.
- Certain microcentrifuges have adapters that allow making use of bigger tubes with smaller ones.
- Microcentrifuges with temperature controls are available for the operation of temperature-sensitive samples.
5. Benchtop centrifuge
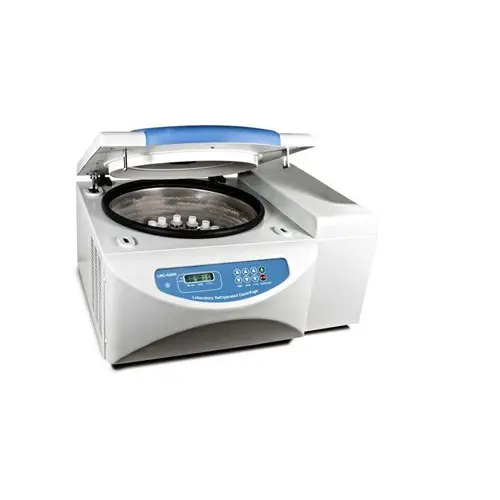
- Benchtop centrifuges are compact centrifuge, which is widely used in research and clinical laboratories.
- It is powered through an electric motor. the tubes rotate around an undetermined axis, which results in force perpendicularly towards the tubes.
- Because they are small, they can be useful for smaller labs that have smaller space.
- A variety of centrifuges for benchtop use are available on the market for a variety of uses.
- A benchtop centrifuge is equipped with an rotor that has racks for the tubes that are used to test and a lid that covers the unit that is used to operate the centrifuge.
6. Continuous flow centrifuge
- Continuous flow centrifuge can be described as a centrifuge that permits the centrifugation of huge volumes of samples, without impacting the rate of sedimentation.
- This kind of centrifuge permits the separation of an enormous amount of samples with high centrifugal force, thereby getting rid of the laborious task of emptying and filling tubes after each cycle.
- They have a shorter length of path that makes it easier for getting the solid portion from the supernatant thereby allowing for a faster pace that the operation can be carried out.
- They also have bigger capacities, which can speed up the process as the sample does not have to be loaded and unloaded repeatedly again , as in conventional centrifuges.
- One liter of sample can be removed using this centrifuge in a that lasts 4-hours or less.
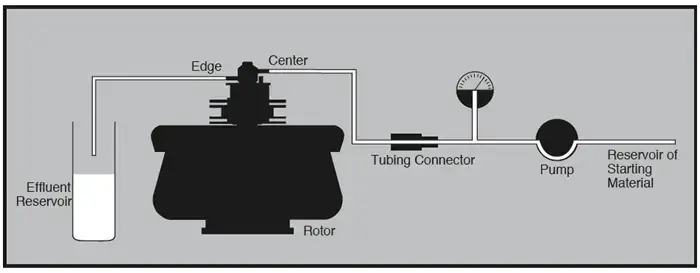
7. Gas centrifuge
- A gas centrifuge can be described as a device specifically designed for the separation of gases that are based on isotopes.
- The centrifuge works on the principle of centrifugal force the same as other centrifuges in which it is possible to separate the molecular on basis of their mass.
- The centrifuge is used principally to extract and separate of uranium-238 and uranium-235.
- The gas centrifuge is based according to the plan of constant flow of gases into and out of the centrifuge unlike other centrifuges that work with batch processing.
- They are placed in cascades in order they can be split into two parts based on their isotopes. They are then transferred to the next centrifuge to continue processing.
- Gas centrifuges are replacing other gaseous diffusion techniques because they produce more gas concentration than previous methods.
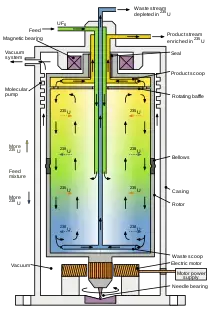
8. Hematocrit centrifuge
- Hematocrit centrifuges are specially designed centrifuges that are used to determine the amount of the volume fraction of the erythrocytes (RBCs) within a specific blood sample.
- The centrifuge can provide hematocrit levels which can be used for tests in biochemistry, immune as well as blood tests, among other tests in general clinical use.
- Hematocrit centrifuges can be used to determine the cause of bleeding as well as polycythemia (an an elevation of the number of erythrocytes to higher than normal levels) anemia bone marrow dysfunction, leukemia, and myeloma.
- Microhematocrit’s centrifuge is able to reach rates of around 11,000 rpm, and RCFs up to 15,000 g for spin tubes with samples.
- A hematocrit centrifuge’s parts centrifuge for hematocrit are similar to those of the benchtop centrifuge, however this particular centrifuge is designed specifically to work with blood samples.
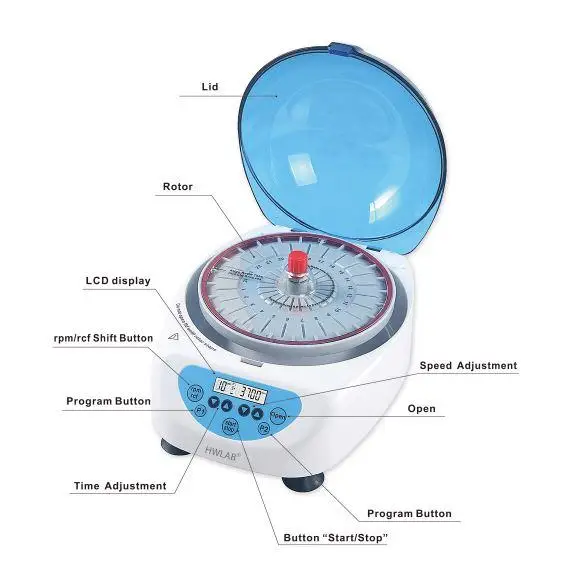
9. Refrigerated centrifuges
- Centrifuges that are refrigerated that come with temperature controls that span from -20degC up to 30degC.
- A different type of centrifuge is available with the control of temperature that is crucial for different processes that require lower temperatures.
- Refrigerated centrifuges are equipped with an instrument for controlling temperature as well as racks and rotors for the test tubes.
- These centrifuges offer an RCF that can reach 60,000 xg which is perfect for the separation of different biological molecules.
- They are commonly employed to collect substances which are rapidly separated, like the yeast cell, chloroplasts and erythrocytes.
- The refrigerated centrifuge’s chamber is shut by a barrier to ensure that it is suitable for the operation.
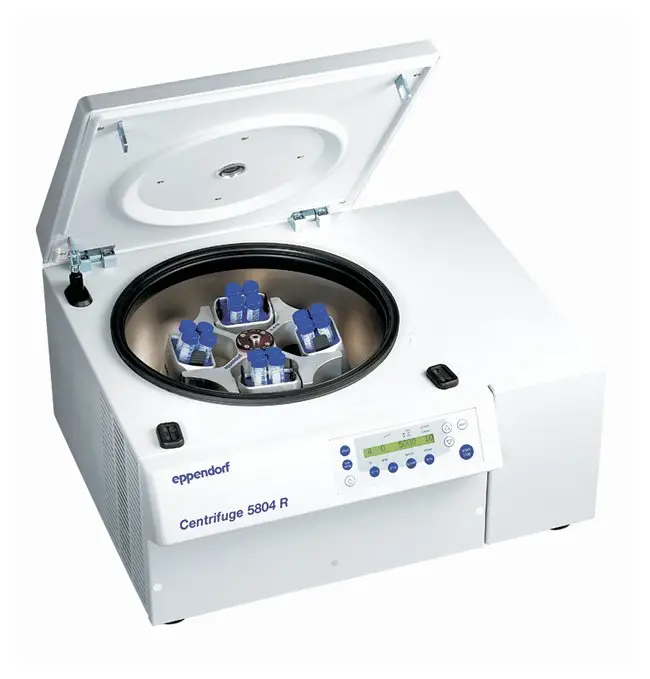
10. Vacuum centrifuge/ Concentrators
- Vacuum centrifuge makes use of centrifugal force and vacuum heat to speed up lab evaporation of the samples.
- These centrifuges can be used for processing large numbers of sample (up to up to 148 samples at once).
- This type of centrifuge can be employed in both biological and chemical laboratories to facilitate the efficient extraction of solvents from samples, thereby concentration of the samples.
- These are often employed in high-throughput labs for samples that may contain an abundance of solvents.
- A rotary evaporator can be used to eliminate solvents that are not needed and reduce bumping in the solvent.
- The centrifuge functions by lowering the chamber’s pressure and also reducing temperature of sample.
- The solvents will evaporate, causing the particles and causing them to separate.
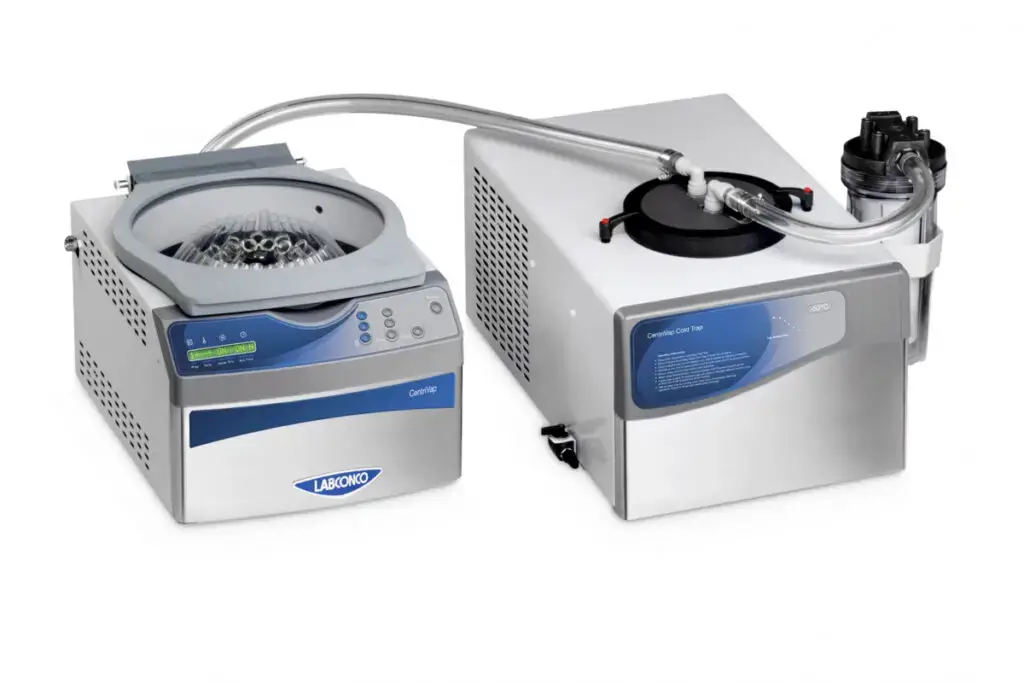
Types of Centrifugation
1. Analytical Centrifugation
- Analytical centrifugation is a powerful separation technique that enables the analysis and characterization of particles in a sample based on their density and the centrifugal force they encounter. One commonly used method for analytical centrifugation is analytical ultracentrifugation (AUC). AUC is a versatile and robust approach specifically designed for the quantitative analysis of macromolecules in solution.
- In AUC, a sample containing macromolecules such as proteins, nucleic acids, or complexes is loaded into a specialized rotor, which is then placed inside an ultracentrifuge. The rotor is subjected to high-speed rotation, generating a strong centrifugal field. As the rotor spins, the centrifugal force acting on the sample causes the particles to sediment, moving towards the bottom of the centrifuge cell.
- The rate at which the particles sediment is influenced by their size, shape, and density. Heavier particles sediment faster, while lighter particles sediment more slowly. By monitoring the sedimentation process over time, valuable information about the particles’ properties and behavior can be obtained.
- A key technique employed in AUC is sedimentation velocity analysis. This involves continuously monitoring the position of the particle boundary or meniscus as it sediments in the centrifuge cell. The movement of the boundary provides data that can be used to calculate the sedimentation coefficient of the particles. The sedimentation coefficient is related to the particle’s size and shape, allowing for the determination of molecular weight, shape, and even the presence of multiple components within a complex mixture.
- Another approach in AUC is sedimentation equilibrium analysis. In this method, equilibrium is reached between the sedimentation and diffusion of particles, resulting in a stable concentration gradient. By measuring the concentration of particles across the gradient, information about their molecular weight, association or dissociation constants, and molecular interactions can be determined.
- Analytical centrifugation, particularly AUC, offers several advantages in the field of molecular analysis. It provides quantitative information about the size, shape, and molecular weight of macromolecules in their native state, without the need for labeling or modification. It can also be used to study protein-protein interactions, protein-nucleic acid interactions, and other molecular interactions. Furthermore, AUC is highly versatile, accommodating a wide range of sample types and allowing for the analysis of complex mixtures.
- Overall, analytical centrifugation, especially AUC, is a valuable technique for studying macromolecules, providing important insights into their structure, behavior, and interactions in solution. It has significant applications in biochemistry, biophysics, pharmaceutical research, and other scientific fields where a comprehensive understanding of macromolecular properties is crucial.
Principle of Analytical Centrifugation
- The principle of analytical centrifugation is rooted in the differential sedimentation of particles based on their density and size under the influence of centrifugal force. It is observed that particles with higher density settle or sediment faster, while larger molecules experience greater movement in the centrifugal field compared to smaller ones.
- Analytical ultracentrifugation is a technique commonly used to determine the relative molecular mass of macromolecules, and it can be conducted through two primary approaches: sedimentation velocity and sedimentation equilibrium.
- In sedimentation velocity analysis, the sedimentation process is monitored in real-time. The movement of a concentration boundary or meniscus formed by the particles within the centrifuge cell is tracked. The rate of sedimentation provides valuable data to calculate the sedimentation coefficient, which is a measure of the hydrodynamic properties of the macromolecules. The sedimentation coefficient can be used to determine the relative molecular mass of the macromolecule and can also indicate changes in size and shape under different experimental conditions.
- Sedimentation equilibrium analysis, on the other hand, focuses on reaching an equilibrium between the sedimentation and diffusion of particles. This equilibrium is characterized by a stable concentration gradient. By measuring the concentration distribution across the gradient, information about the macromolecule’s molecular weight, association or dissociation constants, and molecular interactions can be obtained.
- To facilitate precise and selective observation of the sedimentation process in real-time, analytical ultracentrifuges employ three optical systems: absorbance, interference, and fluorescence. These optical systems allow for the monitoring of the sedimentation boundary or meniscus and provide detailed information about the sample being analyzed.
- In summary, the principle of analytical centrifugation is based on the differential sedimentation of particles according to their density and size. Analytical ultracentrifugation techniques, such as sedimentation velocity and sedimentation equilibrium, exploit this principle to determine the relative molecular mass and hydrodynamic properties of macromolecules. The sedimentation coefficient derived from these analyses is useful in characterizing the size, shape, and changes in macromolecules under varying experimental conditions. Optical systems in analytical ultracentrifuges enable precise and selective observation of the sedimentation process, aiding in the real-time analysis of samples.
Steps of Analytical Centrifugation
Analytical centrifugation involves several steps to analyze the properties of biomolecules. Here are the key steps involved in the process:
- Sample Preparation: Small sample sizes, typically ranging from 20-120 mm³, are collected and prepared for analysis. These samples contain the biomolecules of interest and are placed in specialized analytical cells or tubes.
- Loading the Analytical Cells: The prepared samples are loaded into the analytical cells, which are designed to withstand the high speeds and forces generated during centrifugation. These cells are then carefully positioned inside the ultracentrifuge rotor.
- Centrifugation: The ultracentrifuge is operated to generate a centrifugal force that causes the biomolecules in the sample to migrate through the solvent. This migration occurs radially outwards from the center of rotation. The centrifugation is performed at high speeds, typically in the range of tens of thousands to hundreds of thousands of revolutions per minute (rpm).
- Optical Observation: During centrifugation, the position of the biomolecules relative to the center of rotation is determined using an optical system, such as the Schlieren optical system. The Schlieren optical system allows for the visualization and measurement of the concentration gradient and movement of the solutes in the sample.
- Data Collection: As the biomolecules migrate radially, their distance from the center of rotation is recorded or monitored using the optical system. The data obtained includes the solute concentration and the squared radial distance from the center.
- Analysis and Determination: Based on the data collected, a graph is constructed, plotting the solute concentration against the squared radial distance from the center of rotation. This graph, known as a concentration gradient profile, provides valuable information about the molecular mass and distribution of the biomolecules in the sample.
- Molecular Mass Determination: By analyzing the concentration gradient profile, the molecular mass of the biomolecules can be determined. This is achieved by comparing the experimental data with theoretical models or reference standards. The relationship between the solute concentration and the squared radial distance helps in calculating the molecular mass of the biomolecules.
These steps allow for the analysis of biomolecules using analytical centrifugation. By observing the migration and distribution of the biomolecules under the influence of centrifugal force, valuable information about their molecular mass and other properties can be obtained. This technique is widely used in various fields of research, including biochemistry, biophysics, and pharmaceutical sciences, to study macromolecules and their interactions.
Uses of Analytical Centrifugation
Analytical centrifugation is a versatile technique that finds various uses in scientific research and analysis. Here are some key applications of analytical centrifugation:
- Purity Determination: Analytical centrifugation can be utilized to assess the purity of macromolecules. By analyzing the sedimentation behavior of a sample, it is possible to identify and quantify impurities or contaminants present in the macromolecule preparation. This information is crucial for evaluating the quality and integrity of the sample.
- Examination of Molecular Mass Changes: Analytical centrifugation allows for the examination of changes in the molecular mass of supramolecular complexes. Supramolecular complexes are formed when multiple molecules interact to create a higher-order structure. Through centrifugation, researchers can monitor the sedimentation behavior of these complexes and determine if any changes occur in their molecular mass under different conditions or in response to specific stimuli.
- Determination of Relative Molecular Mass: One of the primary applications of analytical centrifugation is the determination of the relative molecular mass of solutes in their native state. By monitoring the sedimentation behavior of macromolecules in solution, the relative molecular mass can be calculated based on the sedimentation coefficient. This information provides insights into the size, shape, and composition of the macromolecules, helping researchers understand their structure-function relationships.
- Characterization of Macromolecular Interactions: Analytical centrifugation enables the study of macromolecular interactions. By analyzing the sedimentation behavior of a sample containing interacting molecules, researchers can gain insights into the stoichiometry, affinity, and kinetics of these interactions. This information is crucial for understanding the mechanisms of molecular recognition, complex formation, and functional associations.
- Assessment of Protein Conformational Changes: Analytical centrifugation can also be employed to examine conformational changes in proteins. By subjecting proteins to different experimental conditions, such as changes in temperature, pH, or ligand binding, the sedimentation behavior of the protein can be monitored. These observations provide valuable information about conformational transitions and structural changes in the protein molecule.
Examples of Analytical Centrifugation
Analytical centrifugation is a technique that uses a centrifuge to measure the size, shape, and density of particles in a sample. Some examples of analytical centrifugation techniques are:
- Sedimentation velocity centrifugation: This technique uses a centrifuge to measure the size and shape of particles based on their sedimentation rate. The sample is placed in a tube or rotor and rotated at high speeds, causing the particles to sediment through a fluid. The sedimentation rate is measured using a detector, such as a laser or interferometer, and the data is used to calculate the size and shape of the particles.
- Sedimentation equilibrium centrifugation: This technique uses a centrifuge to measure the size, shape, and density of particles based on their sedimentation equilibrium. The sample is placed in a tube or rotor and rotated at high speeds, causing the particles to sediment through a fluid until they reach a state of equilibrium. The concentration of the particles is measured using a detector, such as a UV spectrophotometer, and the data is used to calculate the size, shape, and density of the particles.
- Analytical ultracentrifugation: This technique uses an ultracentrifuge to measure the size, shape, and density of very small particles, such as proteins or nucleic acids. The sample is placed in a tube or rotor and rotated at high speeds, causing the particles to sediment through a fluid. The sedimentation rate is measured using a detector, such as a laser or interferometer, and the data is used to calculate the size, shape, and density of the particles.
- Dynamic light scattering: This technique uses a laser to measure the size and shape of particles based on their scattering of light. The sample is placed in a cuvette and illuminated with a laser, and the scattered light is detected and used to calculate the size and shape of the particles.
- Electrophoretic light scattering: This technique uses a laser to measure the size and shape of particles based on their electrophoretic mobility. The sample is placed in a cuvette and illuminated with a laser, and the scattered light is detected and used to calculate the size and shape of the particles.
Advantages Analytical Centrifugation
- High resolution: Analytical centrifugation allows for the measurement of the size, shape, and density of particles with a high degree of resolution.
- Versatility: Analytical centrifugation can be used to measure a wide range of particles, including proteins, nucleic acids, cells, and organelles.
- Non-invasive: Many analytical centrifugation techniques are non-invasive, meaning they do not alter or damage the sample during the measurement process.
- High sensitivity: Analytical centrifugation techniques are highly sensitive and can detect even small amounts of sample.
Disadvantages Analytical Centrifugation
- Complexity: Analytical centrifugation techniques can be complex and require specialized equipment and expertise.
- Limited to measuring particles based on size, shape, and density: Analytical centrifugation is limited to measuring particles based on their size, shape, and density, and it may not be suitable for measuring other physical properties.
- Time-consuming: Analytical centrifugation techniques can be time-consuming, particularly if multiple measurements are required.
- Expense: Analytical centrifugation techniques require specialized equipment and consumables, which can be expensive.
2. Density gradient centrifugation
- Density gradient centrifugation is a powerful technique used for the separation and purification of molecules based on their density. It takes advantage of the principle that molecules will migrate through a density gradient when subjected to centrifugal force, with the rate of migration determined by their density.
- The process of density gradient centrifugation involves creating a density gradient within a centrifuge tube. This gradient is achieved by layering solutions of different densities, typically in the form of a sucrose or cesium chloride gradient. The sample containing the molecules to be separated is carefully layered on top of the density gradient.
- When the tube is placed in the centrifuge and spun at high speeds, the molecules in the sample experience a centrifugal force that drives them through the density gradient. As the molecules migrate, they encounter regions of the gradient with increasing or decreasing density, depending on their own density. Consequently, molecules of different densities will reach different positions in the tube and form distinct bands or zones.
- The separation is based on the principle that molecules with higher density will sediment or move towards the bottom of the tube faster than molecules with lower density. As a result, molecules of different densities will eventually form separate bands or layers along the length of the gradient.
- After centrifugation, the tube is carefully removed from the centrifuge, and the distinct bands or layers are visualized and collected. Each band represents a specific population of molecules with similar densities. This technique allows for the purification and isolation of molecules with specific density characteristics.
- Density gradient centrifugation has a wide range of applications in various fields of research. It can be used to separate and purify different biomolecules, such as proteins, nucleic acids, lipids, and subcellular organelles, based on their densities. It is also utilized for virus purification and isolation, as viruses often have distinct densities that can be exploited for separation.
- Furthermore, density gradient centrifugation is employed in studies of molecular biology, biochemistry, and biophysics to investigate the structure and composition of macromolecular complexes. By analyzing the distribution of molecules along the density gradient, researchers can gain insights into their composition, interactions, and molecular characteristics.
- In summary, density gradient centrifugation is a technique that exploits the density differences of molecules to achieve their separation. By creating a density gradient and subjecting the sample to centrifugal force, molecules migrate through the gradient and form distinct bands or layers based on their densities. This technique is widely used for purification, isolation, and characterization of biomolecules, as well as in the study of molecular complexes and virus particles.
Principle of Density gradient centrifugation
The principle of density gradient centrifugation is based on the behavior of molecules under the influence of centrifugal force and their interaction with a density gradient medium. The key aspects of this principle are as follows:
- Sedimentation under Centrifugal Force: When a sample containing molecules is subjected to centrifugal force in a centrifuge, the molecules experience a sedimentation force that causes them to move through the sample solution. This sedimentation occurs as the molecules attempt to reach a region with a density matching their own.
- Density Gradient Medium: To facilitate separation based on density, a density gradient medium is employed. This medium can have either decreasing or increasing density along its length. Typically, sucrose or cesium chloride solutions are used to create the density gradient. The gradient is formed by layering solutions of different densities, and the sample is placed on top of the gradient.
- Migration through the Density Gradient: As the sample is subjected to centrifugal force, the molecules within it start migrating through the density gradient. The rate of migration is determined by the density of the molecules relative to the density of the gradient at each point.
- Separation based on Density: Molecules with higher density than the surrounding medium will gradually settle towards the bottom of the tube or container as they move through the density gradient. As they reach a point where their density matches that of the surrounding medium, they become suspended or evenly distributed at that position. Molecules with lower density will remain suspended at higher levels within the density gradient.
- Layer Formation and Recovery: The separation process results in the formation of distinct layers or bands along the length of the density gradient. Each layer contains molecules with a similar density. These layers can be carefully recovered by various methods such as fraction collection or gradient fractionation techniques.
Density gradient centrifugation allows for the separation and purification of molecules based on their density. By exploiting the principle that molecules will settle to a region with a matching density, different molecules can be separated into distinct layers or bands within the density gradient. This technique is widely used in various fields, including biochemistry, molecular biology, and cell biology, for the isolation of biomolecules, purification of subcellular components, and characterization of macromolecular complexes.
Steps of Density gradient centrifugation
Density gradient centrifugation involves several steps to separate particles based on their density. Here are the key steps involved in density gradient centrifugation:
- Preparation of Density Gradient: A density gradient is created by layering a medium with varying concentrations of a solute in a centrifuge tube. The lower concentration solution is gently layered over the higher concentration solution to form a gradient. Common density gradient media include sucrose or cesium chloride solutions.
- Sample Application: The sample containing the particles to be separated is carefully applied on top of the density gradient. This can be done using a pipette or other suitable methods to ensure the sample is layered without disturbing the gradient.
- Ultracentrifugation: The tubes containing the density gradient and sample are placed in an ultracentrifuge. The centrifuge is operated at high speeds to generate a strong centrifugal force. This force causes the particles in the sample to migrate through the density gradient.
- Particle Migration: As the centrifuge spins, the particles in the sample begin to migrate through the density gradient. The rate of migration is determined by the relative density of the particles compared to the surrounding medium. Heavier particles will settle towards the bottom of the tube, while lighter particles will remain suspended at higher levels within the gradient.
- Equilibrium and Fractionation: Over time, the particles reach a point in the density gradient where their density matches that of the surrounding medium. At this point, they become evenly distributed or suspended within the gradient. This state of equilibrium allows for the separation of particles based on their density.
- Fraction Collection: After the equilibrium is reached, the centrifuge is stopped, and the tubes are carefully removed. The tubes are then fractionated by collecting fractions from different levels of the density gradient. Each fraction contains particles with a specific density.
- Particle Isolation: The collected fractions are processed further to isolate the particles of interest. Depending on the nature of the particles and the experimental requirements, various techniques such as centrifugation, filtration, or chromatography can be employed to isolate the particles as individual units.
By following these steps, density gradient centrifugation enables the separation and isolation of particles based on their density. This technique is widely used in various fields, including biochemistry, cell biology, and molecular biology, for the purification of biomolecules, separation of subcellular components, and isolation of specific particles from complex mixtures.
Uses of Density gradient centrifugation
Density gradient centrifugation is a versatile technique with a wide range of applications in various fields of research. Here are some key uses of density gradient centrifugation:
- Purification of Biomolecules: Density gradient centrifugation is commonly employed for the purification of biomolecules, such as proteins, nucleic acids, and subcellular organelles. By exploiting the differences in density, the technique enables the separation of these biomolecules from impurities or contaminants. It is particularly useful for purifying large volumes of biomolecules efficiently and effectively.
- Virus Purification and Study: Density gradient centrifugation is extensively used in virology for the purification of different types of viruses. Viruses often have specific densities, and by using density gradient centrifugation, researchers can separate and isolate viruses from other components in a sample. This purification step is crucial for further studies on virus structure, composition, and function.
- Separation of Particles: Density gradient centrifugation serves as a powerful separation technique for particles with different densities. It enables the separation of particles based on their density differences, allowing for the isolation of specific particles from complex mixtures. This can be applied to separate various types of particles, such as cellular components, subcellular organelles, and biological macromolecules.
- Determination of Particle Densities: In addition to its separation capabilities, density gradient centrifugation can also be used to determine the densities of particles. By creating a density gradient and analyzing the positions at which particles equilibrate within the gradient, their density can be estimated. This information is valuable for characterizing particles, assessing their composition, and understanding their behavior in different environments.
- Study of Particle Interactions: Density gradient centrifugation can be utilized to investigate particle interactions. By subjecting particles to density gradient centrifugation under different conditions or in the presence of specific ligands or substrates, researchers can observe changes in their distribution along the gradient. This provides insights into the nature of particle interactions, including molecular recognition, complex formation, and binding affinities.
Examples of Density gradient centrifugation
Density gradient centrifugation has been employed in several notable experiments and applications. Here are a couple of examples:
- Meselson-Stahl Experiment on DNA Replication: Density gradient centrifugation played a crucial role in the landmark Meselson-Stahl experiment, which provided evidence for the semi-conservative nature of DNA replication. In this experiment, different isotopes of nitrogen were used to label DNA. By subjecting the DNA samples to density gradient centrifugation, it was possible to separate DNA molecules based on their density. The experiment demonstrated that after one round of DNA replication in the presence of heavy nitrogen (15N), the DNA molecules formed a hybrid band with intermediate density. This result supported the semi-conservative model, where each newly synthesized DNA strand contains one original strand and one newly synthesized strand.
- Isolation of Microsomal Fraction and Membrane Vesicles: Density gradient centrifugation is widely used in cell biology to isolate specific cellular components and organelles. One example is the isolation of the microsomal fraction from muscle homogenates. Microsomes are small vesicles derived from the endoplasmic reticulum (ER) and contain membrane-bound proteins and other ER components. By subjecting the muscle homogenate to density gradient centrifugation, the microsomes can be separated based on their density. Subsequently, the membrane vesicles within the microsomal fraction can be further separated based on their differing densities, allowing for the isolation of specific membrane components for further analysis and study.
These examples demonstrate how density gradient centrifugation has been applied in significant experiments and practical applications. By utilizing the principle of particle separation based on density, this technique has contributed to advancing our understanding of DNA replication and facilitated the isolation and study of specific cellular components and membrane vesicles.
Advantages Density gradient centrifugation
- High resolution: Density gradient centrifugation allows for the separation of molecules with a high degree of resolution, making it useful for the purification of specific molecules or organelles.
- High efficiency: Density gradient centrifugation is highly efficient at separating molecules based on their size, shape, and density, allowing for the separation of large quantities of sample in a short amount of time.
- Gentle on samples: Density gradient centrifugation is relatively gentle on samples, as it does not rely on physical forces such as filtration or sedimentation.
- Versatility: Density gradient centrifugation can be used to separate a wide range of molecules, including proteins, nucleic acids, cells, and organelles.
Disadvantages Density gradient centrifugation
- Complexity: Density gradient centrifugation requires the preparation of a gradient, which can be time-consuming and requires specialized equipment and expertise.
- Limited to separating molecules based on density: Density gradient centrifugation is limited to separating molecules based on their density, size, and shape, and it may not be suitable for separating molecules based on other physical properties.
- Limited to separating molecules with a narrow size range: Density gradient centrifugation is most effective at separating molecules with a narrow size range, and it may not be suitable for separating molecules with a wide size range.
- Expense: Density gradient centrifugation requires specialized equipment and consumables, which can be expensive.
3. Differential centrifugation
Differential centrifugation is a commonly used technique in cell biology and biochemistry to separate cellular components based on their size, shape, and density. The principle behind differential centrifugation involves subjecting a sample to a series of increasing centrifugal forces, allowing different components to settle at different rates.
Principle of Differential centrifugation
Differential centrifugation operates based on the principle of differences in sedimentation rate, size, and density of biological particles. Here are the key principles involved in the process:
- Sedimentation Rate: Differential centrifugation takes advantage of the fact that particles of different sizes and densities settle at different rates under the influence of centrifugal force. Larger and denser particles tend to settle more rapidly, while smaller and lighter particles take longer to sediment.
- Centrifugal Force: By applying increasing centrifugal forces during the centrifugation process, particles experience greater sedimentation forces. The centrifugal force causes particles to move outward from the center of rotation, and the settling rate depends on the force applied.
- Sedimentation Steps: In differential centrifugation, a series of centrifugation steps is employed, with each step involving different centrifugal forces and durations. Initially, at lower forces, the larger and denser particles settle down, forming a pellet at the bottom of the centrifuge tube. As the centrifugal force is increased, smaller particles with slower sedimentation rates settle progressively.
- Size and Density Differences: The settling behavior of particles in differential centrifugation depends on their size and density relative to the surrounding medium. Larger and denser particles sediment more quickly and at lower centrifugal forces, while smaller and less dense particles take longer and require higher forces to settle.
- Pellet Formation: The differential sedimentation rates lead to the formation of distinct pellets or sedimented fractions at the bottom of the centrifuge tube. The pellet typically contains the largest and densest particles, while the smaller-sized structures and lighter particles remain in the supernatant.
- Floating Particles: In some cases, particles that are less dense than the surrounding medium may float instead of settling to the bottom. This can occur when the buoyant force acting on the particles is greater than the sedimentation force. These floating particles can be collected from the upper layers of the centrifuge tube.
By exploiting the differential sedimentation rates based on size and density, differential centrifugation enables the separation and fractionation of biological particles into distinct fractions. This technique has been widely used to isolate and study specific components, such as organelles, subcellular structures, and various cellular particles, contributing to our understanding of cell biology and enabling further analysis and characterization of these components.
Steps of Differential centrifugation
Differential centrifugation involves a series of steps to separate particles based on their size and density differences. Here is an outline of the steps involved in the process:
- Sample Homogenization: The sample solution, which may contain cells, organelles, or other biological particles, is homogenized in a buffer medium. The buffer helps maintain the stability and integrity of the sample during centrifugation.
- Initial Centrifugation: The homogenized sample is placed in a centrifuge tube and subjected to centrifugation at a specific centrifugal force, duration, and temperature. This initial centrifugation step is designed to separate the largest and densest particles from the rest of the sample.
- Pellet Formation: As a result of the initial centrifugation, the larger and denser particles sediment and form a pellet at the bottom of the centrifuge tube. The pellet contains the separated particles, while the supernatant, which is the liquid portion above the pellet, contains the remaining particles.
- Supernatant Transfer: The supernatant, which contains smaller particles and components, is carefully transferred to a new centrifuge tube. This allows for further separation and isolation of specific particles in subsequent steps.
- Repeat Centrifugation: The transferred supernatant is subjected to another round of centrifugation, but at a different centrifugal force, duration, and temperature. This step is aimed at separating the next fraction of particles based on their size and density differences.
- Pellet Collection: After each centrifugation step, a new pellet forms at the bottom of the tube, and the supernatant is once again collected. This process is repeated multiple times, with each subsequent centrifugation step separating particles of decreasing size and density.
- Particle Identification: Once all the desired separations have been performed, the collected pellets and supernatant fractions can be further analyzed. The separated particles can be identified through various methods, such as biochemical assays, microscopy, or specific staining techniques that target unique indicators or markers of the specific particles of interest.
By systematically adjusting the centrifugal force, duration, and temperature in each step, differential centrifugation allows for the separation and isolation of particles based on their size and density. This technique has been widely used in biological research and diagnostics to study organelles, subcellular components, and various cellular particles, providing valuable insights into their structure, function, and composition.
Uses of Differential centrifugation
Differential centrifugation finds several applications in biological research and laboratory settings. Here are some key uses of this technique:
- Organelle and Membrane Separation: One of the primary applications of differential centrifugation is the separation of cell organelles and cellular membranes. By adjusting the centrifugal force and duration, different organelles such as mitochondria, lysosomes, peroxisomes, and endoplasmic reticulum can be isolated. This allows for the study of their structure, function, and biochemical properties.
- Nucleus Separation: While not providing high-resolution separation, differential centrifugation can be employed for the low-resolution separation of the nucleus. This allows for the isolation of nuclear components and the study of nuclear processes.
- Purification of Extracts: Differential centrifugation is useful for the purification of biological extracts containing larger-sized impurities. By removing the larger particles or debris through successive centrifugation steps, the target molecules or components of interest can be obtained in a more purified form.
- Subcellular Fractionation: The technique enables the fractionation of complex mixtures into distinct subcellular fractions based on their size and density differences. This facilitates the study of specific cellular compartments and their associated functions.
- Enzyme and Protein Studies: Differential centrifugation can be applied to separate enzymes and proteins based on their subcellular localization. By isolating specific organelles or subcellular fractions, researchers can investigate the distribution, activity, and regulation of enzymes and proteins within different cellular compartments.
- Diagnostic Applications: In clinical laboratories, differential centrifugation is used for the separation and isolation of specific components in bodily fluids, such as blood or urine. This enables the detection and analysis of disease-related markers, cell types, or infectious agents.
4. Isopycnic centrifugation
Isopycnic centrifugation, also known as equilibrium density gradient centrifugation, is a technique used to separate particles based on their density. Unlike differential centrifugation, which separates particles based on their size and density differences, isopycnic centrifugation relies solely on the density of the particles.
Principle of Isopycnic centrifugation
The principle of isopycnic centrifugation, also known as equilibrium centrifugation, is based on the density of particles rather than their sizes. Here are the key principles underlying this technique:
- Density-Based Separation: Isopycnic centrifugation separates particles solely based on their densities. Unlike differential centrifugation, which considers both size and density, isopycnic centrifugation relies on the buoyant density of the particles.
- Equilibrium Reached: As the sample is subjected to centrifugal force, the particles move through a density gradient medium in the centrifuge tube. The density of the medium increases gradually as one moves towards the bottom of the tube.
- Particle Migration: The particles migrate through the density gradient in the tube, moving towards the bottom. The rate of migration depends on the size of the particles, with larger particles moving faster than smaller ones.
- Equilibrium Bands: The migration of particles continues until each particle reaches a region in the gradient where its density matches the density of the surrounding medium. At this point, the particles become buoyant and form distinct bands or layers within the density gradient.
- Density-Dependent Separation: The separation achieved through isopycnic centrifugation is based on the density of the particles rather than their sizes. Denser particles settle at the bottom of the tube, while less dense particles form bands above them.
- True Equilibrium: Isopycnic centrifugation is considered a true equilibrium because the separation is directly dependent on the buoyant densities of the particles, not their sizes. Once the particles reach regions with matching densities, their movement ceases, resulting in a stable equilibrium.
By exploiting the principle of density-based separation, isopycnic centrifugation enables the isolation and purification of particles with similar sizes but different densities, such as nucleic acids, viruses, subcellular organelles, and biomolecules. The resulting separated bands or fractions can be further analyzed to study the composition, structure, and functional properties of the isolated particles.
Steps of Isopycnic centrifugation
The steps involved in performing isopycnic centrifugation are as follows:
- Gradient Preparation: A density gradient is prepared in the centrifuge tube by layering a solution with an increasing density towards the bottom of the tube. This can be achieved by carefully layering solutions of varying densities or using a pre-formed gradient.
- Sample Distribution: The biological sample solution, along with the appropriate salt solution, is uniformly distributed onto the top of the gradient in the centrifuge tube. The sample is carefully layered onto the gradient to prevent mixing.
- Centrifugation: The centrifuge tube is placed in the centrifuge, and the centrifuge is operated at a specific speed and duration. As the centrifugal force is applied, the particles in the sample start moving down the tube.
- Density Gradient Formation: The salt in the gradient creates a density gradient in the tube. The density increases gradually as one moves towards the bottom of the tube.
- Particle Migration and Equilibrium: The particles in the sample migrate through the density gradient. Each particle moves down the tube until it reaches a region where its density matches the density of the surrounding medium. At this point, the particle reaches equilibrium and settles down.
- Particle Separation and Identification: After centrifugation, the tube is carefully removed from the centrifuge. The separated particles are present in distinct bands or layers in the tube, based on their respective densities. These bands or layers can be identified visually or through various analytical techniques, such as spectrophotometry or immunodetection. The particles of interest can be collected and further analyzed or subjected to additional purification steps, if required.
The steps of isopycnic centrifugation allow for the separation and isolation of particles based on their densities. By utilizing the density gradient, this technique enables the precise separation and identification of particles with similar sizes but different densities, providing valuable insights into their composition and characteristics.
Uses of Isopycnic centrifugation
Isopycnic centrifugation finds several uses in various scientific and biomedical applications. Some of its key uses include:
- Purification of Biomolecules: Isopycnic centrifugation is widely employed for the purification of biomolecules, including proteins, nucleic acids, and subcellular organelles. By taking advantage of the density differences between different biomolecular components, isopycnic centrifugation can separate and purify these molecules from complex mixtures.
- Density Determination: Isopycnic centrifugation serves as a valuable technique for determining the density of particles. By analyzing the position of particles within the density gradient, researchers can precisely measure and characterize the densities of various particles, such as macromolecules, viruses, or cellular components. This information is crucial for understanding their structural and functional properties.
- Fractionation of Particles: Isopycnic centrifugation allows for the fractionation of particles based on their densities. This technique can be employed to separate and isolate specific particle populations from complex mixtures, enabling further analysis and characterization. Fractionation based on density is particularly useful for studying and separating particles that have similar sizes but different densities.
- Study of Biomolecular Interactions: Isopycnic centrifugation can provide insights into biomolecular interactions. By subjecting mixtures of interacting molecules to isopycnic centrifugation, researchers can analyze the behavior and distribution of the interacting components within the density gradient. This information aids in understanding molecular interactions, such as protein-protein interactions or protein-nucleic acid interactions.
- Virus Purification: Isopycnic centrifugation is extensively used for the purification and concentration of viruses. Viral particles can be separated and purified from host cell components, contaminants, and debris by exploiting their distinct density characteristics. This purification step is crucial for studying the structure, composition, and function of viruses and for vaccine development and viral research.
5. Rate-zonal density gradient centrifugation/ Moving Zone Centrifugation
Rate-zonal density gradient centrifugation a form of centrifugation that is used to separate particles based on their size as well as shape. It follows the same principle of density gradient centrifugation , however it operates in a different manner. It’s also known as move zone centrifugation.
Principle of Rate-zonal density gradient centrifugation
Rate-zonal density gradient centrifugation is a technique used to separate particles based on both their size and shape. The principle of this method involves layering a sample in a restricted zone on top of a pre-formed density gradient, which is then subjected to centrifugation. The density gradient consists of densities significantly lower than those of the particles being centrifuged.
During centrifugation, all particles present in the sample migrate into the density gradient due to the difference in density. The rate at which particles sediment through the gradient depends primarily on their size and shape. Larger particles sediment more rapidly, while particles with a more spherical shape also tend to sediment more rapidly.
The separation in rate-zonal density gradient centrifugation is achieved based on the sedimentation coefficient of the particles. The sedimentation coefficient reflects the rate at which a particle moves through the medium under the influence of centrifugal force. The particles sediment at different rates, resulting in their fractionation within the density gradient.
Unlike differential centrifugation, where the sample is distributed throughout the medium, rate-zonal centrifugation involves the initial presence of the sample as a narrow band on top of the density gradient. This restricted zone allows for a distinct separation of particles based on their sedimentation properties.
By applying the principle of rate-zonal density gradient centrifugation, particles can be fractionated according to their size and shape. This technique is particularly useful for studying and separating particles with different sedimentation characteristics, such as macromolecules, subcellular organelles, and cellular components. The resulting fractions can be further analyzed and studied to gain insights into their structural and functional properties.
Steps of Rate-zonal density gradient centrifugation
The steps involved in rate-zonal density gradient centrifugation are as follows:
- Preparation of density gradient: A density gradient is prepared in a centrifuge tube by layering solutions of different densities. The density increases gradually from the top to the bottom of the tube. This can be achieved by using solutions with varying concentrations of sucrose, cesium chloride, or other suitable density gradient media.
- Layering the sample: The sample to be fractionated is carefully layered on top of the density gradient in the form of a narrow band. This ensures that the sample is initially concentrated in a restricted zone on the gradient.
- Centrifugation: The tube containing the density gradient and the layered sample is placed in a centrifuge and subjected to centrifugal force. The centrifuge is operated at a specific speed and duration, allowing the particles to migrate through the gradient.
- Separation of particles: During centrifugation, particles with larger sizes and more spherical shapes sediment more rapidly. As a result, different particles in the sample separate into distinct bands at different positions along the density gradient. The faster-moving particles form bands closer to the bottom, while slower particles form bands higher up in the gradient.
- Collection of fractions: After centrifugation, the tube is carefully removed from the centrifuge. The separated particles are obtained by collecting the fractions from different bands. This is usually done by puncturing the tube at specific points or by carefully aspirating the fractions from the top.
The collected fractions can be further analyzed and studied to gain insights into the size, shape, and sedimentation properties of the particles. The separation is primarily based on the sedimentation coefficient of the particles, which reflects their rate of sedimentation through the density gradient.
Rate-zonal density gradient centrifugation is a powerful technique used in various fields of research, including biochemistry, molecular biology, and cell biology. It allows for the separation and purification of particles based on both their size and shape, providing valuable information about their structural and functional characteristics.
Uses of Rate-zonal density gradient centrifugation
Rate-zonal density gradient centrifugation has various applications in the field of molecular biology and biochemistry. Some of its uses include:
- Separation of viruses: Rate-zonal density gradient centrifugation is a valuable tool for separating different viruses based on their size and density. Viruses often have unique components that vary in size and density, making this technique useful for their separation and purification.
- Fractionation of RNA: This method has been widely employed for the fractionation of RNA molecules on sucrose gradients. It allows for the separation of different RNA species based on their size and secondary structure, enabling researchers to study their functions and characteristics.
- Separation and purification of DNA: Rate-zonal density gradient centrifugation has been utilized for the separation, purification, and fractionation of DNA molecules from viruses and bacteria. By exploiting the differences in size and shape, DNA molecules can be separated into distinct fractions, aiding in their analysis and further research.
- Fractionation of polysomes and ribosome subunits: One of the earliest applications of rate-zonal density gradient centrifugation was in the fractionation of polysomes and ribosome subunits. This technique allows for the separation and isolation of these important cellular components, facilitating studies on protein synthesis and translational regulation.
6. Differential velocity (Moving Boundary) centrifugation
Differential velocity centrifugation (DVC) is a kind of centrifugation technique where components are separated and placed into the centrifuge tube using an increasing number of velocities.
Principle of Differential velocity (Moving Boundary) centrifugation
Differential velocity (Moving Boundary) centrifugation is a technique used to separate biological particles based on their size and density by taking advantage of their differential sedimentation rates. The principle of this method can be summarized as follows:
- Differences in sedimentation rate: The separation in this method is based on the fact that particles of different sizes and densities have different sedimentation rates when subjected to centrifugal force. Larger and denser particles sediment more rapidly than smaller and less dense particles.
- Centrifugation process: The sample containing the mixture of particles is placed in a centrifuge tube and subjected to increasing rotational speed. As the speed of the centrifuge increases, larger and denser particles start to settle first due to their faster sedimentation rate.
- Particle distribution: As the centrifugation continues, particles of different sizes and densities begin to settle at different levels in the tube. The largest and densest particles form a pellet at the bottom of the tube, leaving smaller-sized particles suspended in the supernatant.
- Pellet collection: Once the centrifugation is completed, the tube is carefully removed from the centrifuge, and the pellet at the bottom is collected. This pellet contains the larger and denser particles that have settled out of the suspension.
- Further separation: The supernatant, which contains smaller particles, can be subjected to additional centrifugation steps at higher speeds or longer durations to separate out even smaller particles. This process can be repeated multiple times to obtain particles of progressively smaller sizes.
- Floating particles: In the case of particles that are less dense than the surrounding medium, they do not settle at the bottom but instead float at the top of the tube during centrifugation. These particles can be collected separately based on their floating properties.
Differential velocity (Moving Boundary) centrifugation is a widely used technique for fractionating and isolating particles of different sizes and densities. It allows for the separation of biological samples into distinct fractions, enabling researchers to study and analyze specific components of interest.
Steps of Differential velocity (Moving Boundary) centrifugation
The steps of Differential velocity (Moving Boundary) centrifugation can be summarized as follows:
- Sample preparation: The sample solution, containing the mixture of particles to be separated, is homogenized in a buffer medium. The buffer helps maintain the stability and integrity of the particles during centrifugation.
- Initial centrifugation: The homogenized sample is then carefully layered or added to a centrifuge tube. The tube is placed in the centrifuge and operated at a lower rotor speed for a specific duration and temperature. This initial centrifugation step allows for the larger and denser particles to settle down and form a pellet at the bottom of the tube.
- Pellet separation: Once the centrifugation is complete, the tube is carefully removed from the centrifuge. The pellet, containing the larger and denser particles, is separated from the supernatant (the liquid portion of the sample) by gently decanting or aspirating the supernatant.
- Subsequent centrifugation: The supernatant, which now contains smaller particles, is transferred to a new centrifuge tube. The tube is then centrifuged again, but at a different rotor speed or for a different duration and temperature. This step allows for further separation of the smaller particles from the supernatant.
- Supernatant and pellet separation: After the second centrifugation, the supernatant and any subsequent supernatants obtained from additional centrifugation steps are separated from the pellets formed. The pellets may vary in size and density, depending on the particles present in the sample.
- Repeat steps: The steps of subsequent centrifugation, separation of supernatant and pellet, can be repeated multiple times if necessary to achieve the desired level of separation and isolation of particles. Each centrifugation step may utilize different conditions (speed, duration, temperature) to target specific particle sizes or densities.
- Particle identification: Once the separation steps are completed, the isolated particles can be identified and characterized further. This can be done by testing for specific indicators or markers that are unique to the particles of interest. Various analytical techniques, such as microscopy, immunoassays, or biochemical assays, can be employed for particle identification.
Uses of Differential velocity (Moving Boundary) centrifugation
Differential velocity (Moving Boundary) centrifugation, also known as differential centrifugation, has several important uses in various scientific fields. These include:
- Separation of cell organelles and membranes: One of the primary applications of differential centrifugation is the isolation and separation of specific cell organelles and membranes. By subjecting cellular homogenates to sequential centrifugation steps at different speeds and durations, different components of the cell, such as mitochondria, endoplasmic reticulum, and lysosomes, can be separated based on their sedimentation rates. This technique allows for the purification and enrichment of specific cellular components for further analysis or study.
- Low-resolution separation of the nucleus: Differential centrifugation can be employed to obtain a low-resolution separation of the nucleus from other cellular components. By applying appropriate centrifugation conditions, the larger and denser nucleus can be sedimented, while the remaining cytoplasmic components stay in the supernatant. Although this method provides a relatively crude separation, it is useful for obtaining enriched nuclear fractions for basic research or diagnostic purposes.
- Particle size identification and comparison: Since differential centrifugation separates particles based on their sizes, it can be used to identify and compare particles of different sizes in a sample. By subjecting the sample to centrifugation at different speeds and durations, particles with different sedimentation rates will settle at different positions in the centrifuge tube. This enables the characterization and comparison of particles based on their differential sedimentation patterns, aiding in the analysis of particle size distributions or the presence of distinct populations of particles in a sample.
7. Equilibrium density gradient centrifugation
Equilibrium Density Gradient Centrifugation is an altered and specific form of centrifugation using a density gradient.
Principle of Equilibrium density gradient centrifugation
Equilibrium density gradient centrifugation, also known as isopycnic centrifugation, relies on the principle of density-based separation of particles in a solution. The following principles govern the process:
- Separation based on density: The fundamental concept of equilibrium density gradient centrifugation is that particles in a solution will separate based on their densities. The density gradient is established within the centrifuge tube, with higher density at the bottom and lower density at the top.
- Migration through the density gradient: The particles in the sample are introduced into the tube containing the density gradient. As the centrifuge is operated, the particles move through the gradient under the influence of centrifugal force.
- Equilibrium point: Each particle will migrate until it reaches a region in the gradient where the density of the surrounding medium matches its own density. At this equilibrium point, the centrifugal force acting on the particle is balanced by the buoyant force pushing the particle upward. As a result, the particle comes to a halt and remains suspended at a specific position within the gradient.
- Layer formation: The density of the medium in the gradient increases gradually as we move towards the bottom of the tube. Consequently, particles with higher densities will settle down at the bottom of the tube, while particles with lower densities will form bands at higher positions in the gradient. This layering allows for the separation and fractionation of particles based on their density.
By carefully controlling the density gradient and centrifugation conditions, particles can be positioned in specific regions of the gradient according to their density. This technique enables the separation and purification of particles with different densities, facilitating the study of their characteristics, interactions, and properties.
In summary, equilibrium density gradient centrifugation employs the principle of density-based separation, where particles migrate through a density gradient until they reach a position of equilibrium based on their density. The resulting layered pattern allows for the separation and isolation of particles with distinct densities for further analysis and characterization.
Steps of Equilibrium density gradient centrifugation
The steps involved in equilibrium density gradient centrifugation are as follows, based on the provided information:
- Gradient preparation: A density gradient is prepared in a centrifuge tube, typically with increasing density towards the bottom of the tube. This gradient can be prepared manually by layering different concentrations of a suitable salt or by using pre-formed density gradients.
- Sample distribution: The solution containing the biological sample, along with any necessary salt or buffer, is uniformly distributed throughout the centrifuge tube. This ensures an even distribution of the sample within the density gradient.
- Centrifugation: The centrifuge tube containing the sample and density gradient is placed inside the centrifuge, and the centrifugation process is initiated. As the centrifuge spins, a centrifugal force is applied, causing the particles to move down the tube through the density gradient.
- Equilibrium position: As the particles migrate through the density gradient, they reach a region where the density of the surrounding medium matches their own density. At this point, the particles come to a stop and become suspended in the gradient, achieving equilibrium. The position at which each particle settles is determined by its density.
- Separation and identification: Once equilibrium is reached, the tube is carefully removed from the centrifuge. The different particles are now separated into distinct layers based on their densities. These layers can be carefully collected, and the particles within each layer can be further analyzed or subjected to additional processes for identification, characterization, or further purification.
It’s important to note that the specific techniques and processes used for separation and identification can vary depending on the nature of the particles and the desired outcome of the experiment.
In summary, the steps of equilibrium density gradient centrifugation involve the preparation of a density gradient, uniform distribution of the sample, centrifugation to achieve equilibrium, and subsequent separation and identification of particles based on their densities within the gradient.
Uses of Equilibrium density gradient centrifugation
Equilibrium density gradient centrifugation has various uses in the field of biological research. Here are some applications based on the provided information:
- Purification of biomolecules: Equilibrium density gradient centrifugation is commonly employed for the purification of large volumes of biomolecules. The density gradient allows the separation of different particles based on their densities. By carefully collecting specific layers or bands containing the desired biomolecules, researchers can achieve purification of the target molecules from complex mixtures.
- Determination of particle densities: Equilibrium density gradient centrifugation serves as a valuable technique for determining the densities of various particles. By observing the position at which different particles settle within the density gradient, researchers can infer their relative densities. This information is useful for characterizing and comparing particles, such as organelles, macromolecules, or subcellular components, based on their density properties.
- Analysis of complex mixtures: Equilibrium density gradient centrifugation can be utilized to analyze and study complex mixtures of particles. By separating particles into distinct layers or bands according to their densities, researchers can obtain fractions enriched with specific components of interest. This allows for further analysis, identification, or characterization of individual particles or groups of particles within the mixture.
- Fractionation of subcellular components: This technique is particularly useful for fractionating subcellular components, such as organelles, into distinct layers based on their densities. By carefully collecting specific layers, researchers can isolate and study individual organelles or subcellular structures. This enables the investigation of their functions, molecular composition, and interactions within the cell.
8. Sucrose gradient centrifugation
Sucrose gradient centrifugation is one kind of density gradient centrifugation in which it is made from sucrose by altering the sucrose concentration.
Principle of Sucrose gradient centrifugation
Sucrose gradient centrifugation is a technique used for the separation and purification of molecules based on their densities. Here is an explanation of the principle behind sucrose gradient centrifugation based on the provided information:
- Density-based separation: Sucrose gradient centrifugation takes advantage of the principle that molecules or particles will settle under a centrifugal force until they reach a region of the medium where the density matches their own. In this case, a density gradient is established using a sucrose solution.
- Sucrose density gradient: The sucrose gradient is prepared in a centrifuge tube with a higher density at the bottom and a lower density at the top. The gradient is created by layering sucrose solutions of different concentrations, typically starting with lower concentrations at the top and increasing concentrations towards the bottom.
- Centrifugal force: The sample containing the molecules of interest is layered onto the top of the sucrose gradient in the centrifuge tube. As the centrifuge is operated, the sample is subjected to a centrifugal force, causing the molecules to move through the sucrose medium.
- Movement through the gradient: The molecules in the sample begin to move through the sucrose gradient due to the centrifugal force. The more dense molecules tend to settle towards the bottom of the tube as they traverse through the density gradient.
- Equilibrium suspension: As the molecules move through the gradient, they eventually reach a point where their density matches the density of the surrounding sucrose medium. At this point, the molecules become suspended, forming distinct layers at specific regions of the sucrose gradient.
- Layer separation: The molecules with different densities are separated into different layers along the sucrose gradient. These layers can be visualized as bands within the tube. The layers containing molecules of interest can be recovered by carefully collecting specific bands using techniques such as fractionation or aspiration.
Sucrose gradient centrifugation allows for the separation and purification of molecules based on their densities. By creating a sucrose density gradient and subjecting the sample to centrifugation, molecules with different densities are separated into distinct layers within the gradient. This technique is widely used in various biological and biochemical applications for the isolation and characterization of molecules, such as proteins, nucleic acids, organelles, and subcellular components, based on their density properties.
Steps of Sucrose gradient centrifugation
Sucrose gradient centrifugation involves several steps to separate and isolate particles based on their densities. Here are the steps involved in sucrose gradient centrifugation:
- Density gradient preparation: A density gradient is prepared using sucrose solutions of varying concentrations. Typically, higher concentrations of sucrose are layered at the bottom of the centrifuge tube, and lower concentrations are layered on top, creating a sucrose density gradient.
- Sample application: The sample containing the particles of interest is gently layered onto the top of the sucrose gradient in the centrifuge tube. Care should be taken to avoid disrupting the gradient.
- Ultracentrifugation: The tubes containing the sample and sucrose gradient are placed in an ultracentrifuge and spun at high speeds. The centrifugal force causes the particles to sediment and move through the sucrose density gradient.
- Equilibrium sedimentation: As the particles travel through the sucrose gradient, they reach a point where their density matches the density of the surrounding sucrose medium. At this point, the particles become suspended and form distinct bands or fractions within the gradient.
- Fraction collection: After the centrifugation is complete, the centrifuge tubes are carefully removed from the ultracentrifuge. The fractions containing the particles of interest are collected by carefully aspirating or pipetting them from specific regions of the sucrose gradient. Each fraction may contain particles with similar densities.
- Particle isolation: The collected fractions are further processed or analyzed to isolate and characterize the particles of interest. This may involve additional purification steps, such as dialysis, concentration, or further separation techniques.
Sucrose gradient centrifugation is a versatile technique used in various biological and biochemical applications to separate and purify particles based on their densities. By creating a sucrose density gradient, particles migrate through the gradient until they reach equilibrium with the surrounding medium, allowing for their isolation in distinct bands or fractions. This technique is commonly employed in the isolation of subcellular organelles, separation of macromolecules like proteins and nucleic acids, and characterization of particles based on their density properties.
Uses of Sucrose gradient centrifugation
Sucrose gradient centrifugation is a widely used technique with various applications in the field of molecular biology and biochemistry. Here are some of the key uses of sucrose gradient centrifugation:
- Separation of DNA and RNA: Sucrose gradient centrifugation is commonly employed to separate DNA and RNA molecules based on their size and density. By subjecting a mixture of nucleic acids to sucrose gradient centrifugation, different DNA or RNA fragments can be separated into distinct bands or fractions according to their densities. This technique is particularly useful for analyzing DNA or RNA samples, studying their structure, and determining their sizes.
- Analysis of protein complexes: Sucrose gradient centrifugation is an important tool for studying protein complexes and their interactions. By subjecting a protein mixture to centrifugation in a sucrose gradient, the protein complexes of different sizes and densities can be separated and visualized as distinct bands. This technique allows researchers to analyze the composition, size, and stoichiometry of protein complexes and investigate their functions and interactions within biological systems.
- Determination of macromolecule density: Sucrose gradient centrifugation enables the determination of the density of macromolecules. By comparing the migration of macromolecules of interest with known density standards within a sucrose gradient, their densities can be estimated. This information is valuable for characterizing macromolecules, studying their properties, and assessing their purity.
- Size determination of macromolecules: Sucrose gradient centrifugation can also provide valuable insights into the size distribution of macromolecules. By examining the positions of different macromolecular species in the sucrose gradient, researchers can infer their relative sizes. This information is particularly useful for characterizing macromolecules such as DNA, RNA, proteins, and protein complexes.
- Fractionation and purification: Sucrose gradient centrifugation can be employed for the fractionation and purification of various macromolecules. By collecting specific fractions from the sucrose gradient, researchers can isolate macromolecules of interest based on their density and size characteristics. This technique facilitates the separation of macromolecules from contaminants and impurities, enabling their further analysis or downstream applications.
Types of centrifuge used in pharmaceutical industry
There are four types of centrifuges that are used in pharmaceutical production. They all have different characteristics.
A industrial centrifuge is an equipment that uses centrifugal force to separate fluids or particles. There are three types of centrifuges: liquid-liquid separation, liquid-liquid-solid separation and liquid-liquid–solid separation. This article will discuss four types of centrifuges used in the pharmaceutical industry.
1. Horizontal peeler centrifuges
The principle of centrifugal force is used to separate solids from liquids using density difference. You can use this method to separate salt from water, or urea from the unconverted carbamate mixture. High rotation speeds provide the centrifugal force necessary to allow suspended solids settle on the drum’s inner surface. All washing steps are done at the same speed and in the exact same centrifuge vessel.
Its unique feature is its constant basket speed. This allows for all operations such as feeding and drying, drying and discharging to be done at full basket speed. Horizontal peeler centrifuges are available in both batch and continuous mode.
This centrifuge’s peeler is controlled by a hydraulic piston and a peeler shaft. The blade and mounting arrangement are located above the discharge chute, so that scraped cakes can be directly deposited into the chute.
2. Top discharge centrifuges
They are an economical alternative to pharmaceutical production. Top discharge centrifuges are easily adaptable to product characteristics. They can handle a variety of products in small and large batches. The machine can discharge gentle solids without causing particle destruction.
This type usually has an automatic clean in-place (CIP), and a lossless filter cakes discharge. Cleaning is easy for machinery parts in direct contact with the product.
3. Vertical peeler centrifuges
Vertical peeler centrifuges operate under discontinuous filtration and are well-suited for the efficient separation of pharmaceutical and fine chemicals. This centrifuge can be used for small batches and products that change frequently.
Because the peeling process is done at a slower speed, there are no crystal breaks. The process housing opens/closes automatically, allowing for complete inspection of all parts within the area. The cleaning process is performed by a fully automated CIP system. It can also flood the process enclosure, if required.
This centrifuge machinery is suited for separating Amino acids, benzoic acid, benzene, sulphur, calcium hypochloride, hexachlorocyclohexane, insulin, penicillin, and starch, for example.
4. Inverting filter centrifuges
The filtration system can automatically discharge the particulates collected. This filtration system is ideal for filtering process waste or difficult-to-filter products. It has a gentle product discharge and leaves no residue heel. The particulates are kept in their original shape by the discharge method, which does not require pressing or scraping. Hydraulic systems can be contaminated by the mechanically controlled system. It spins at high speeds with maximum basket loads and consumes relatively little washing medium.
Centrifuge rotor
Rotors in centrifuges are the motor devices that house the tubes with the samples. Centrifuge rotors are designed to generate rotation speed that can bring about the separation of components in a sample. There are three main types of rotors used in a centrifuge, which are:
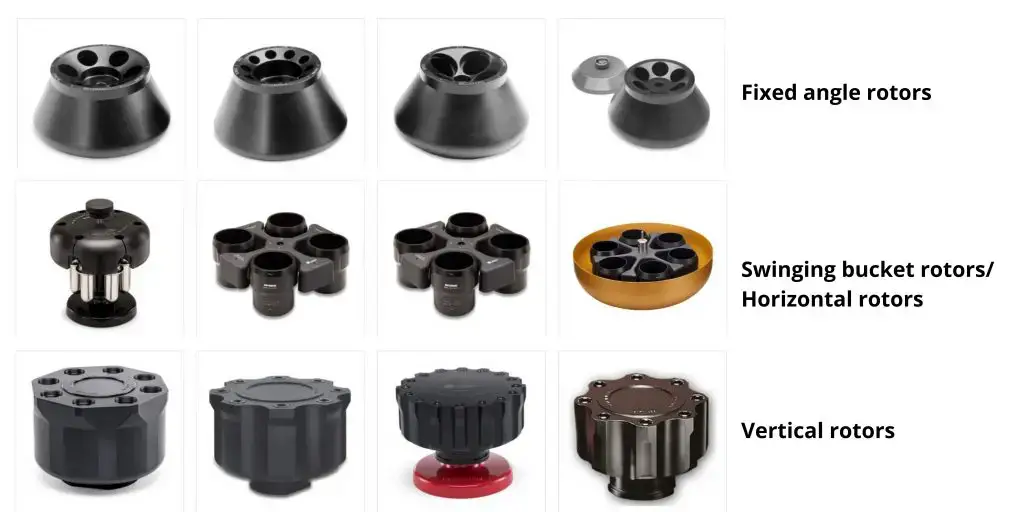
1. Fixed angle rotors
These rotors hold the sample tubes at an angle of 45° in relation to the axis of the rotor. In this type of rotor, the particles strike the opposite side of the tube where the particles finally slide down and are collected at the bottom. These are faster than other types of rotors as the pathlength of the tubes increases. However, as the direction of the force is different from the position of the tube, some particles might remain at the sides of the tubes.
Fixed-angle rotors are centrifuge rotors that have a fixed angle of inclination and are used in centrifuges to separate particles based on size and density. Fixed-angle rotors are typically used for high-speed separations, and are capable of reaching very high speeds.
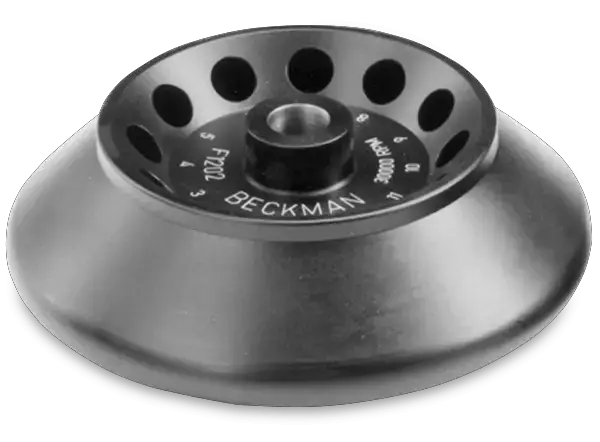
There are several advantages to using fixed-angle rotors:
- High speed: Fixed-angle rotors are capable of reaching very high speeds, making them ideal for separating particles based on size and density.
- Efficient separation: Because the samples are suspended at a fixed angle, fixed-angle rotors are generally more efficient at separating particles compared to swing-bucket rotors.
- Variety of sizes: Fixed-angle rotors come in a range of sizes and designs, making them suitable for a variety of applications and sample types.
- Versatility: Fixed-angle rotors can be used for a wide range of applications, including separating proteins, nucleic acids, cells, and other particles.
- Durability: Fixed-angle rotors are generally more durable and long-lasting compared to other types of rotors.
It is important to choose the appropriate fixed-angle rotor for your specific application, as different rotors are optimized for different types of separations and sample volumes.
2. Swinging bucket rotors/ Horizontal rotors
Swinging bucket rotors hold the tubes at an angle of 90° as the rotor swings as the process is started. In this rotor, the tubes are suspended in the racks that allow the tubes to be moved enough to acquire the horizontal position. In this type of rotors, the particles are present along the direction or the path of the force that allows the particles to be moved away from the rotor towards the bottom of the tubes. Because the tubes remain horizontal, the supernatant remains as a flat surface allowing the deposited particles to be separated from the supernatant.
3. Vertical rotors
Vertical rotors provide the shortest pathlength, fastest run time, and the highest resolution of all the rotors. In vertical rotors, the tubes are vertical during the operation of the centrifuge. The yield of the rotor is not as ideal as the position of the tube doesn’t align with the direction of the centrifugal force. As a result, instead of settling down, particles tend o spread towards the outer wall of the tubes. These are commonly used in isopycnic and density gradient centrifugation.
Vertical rotors are designed for use in vertical centrifuges, which are laboratory instruments used to separate particles based on their size and density. Vertical rotors are typically smaller and more compact than horizontal rotors, and are designed for use with smaller volumes of samples.
There are several advantages to using vertical rotors:
- High speed: Vertical rotors are capable of reaching very high speeds, making them ideal for separating particles based on size and density.
- Compact size: Vertical rotors are typically smaller and more compact than horizontal rotors, making them ideal for use in smaller laboratories or when space is limited.
- Efficient separation: Because the samples are suspended in a vertical position, vertical rotors are generally more efficient at separating particles compared to horizontal rotors.
- Variety of sizes: Vertical rotors come in a range of sizes and designs, making them suitable for a variety of applications and sample types.
- Versatility: Vertical rotors can be used for a wide range of applications, including separating proteins, nucleic acids, cells, and other particles.
It is important to choose the appropriate vertical rotor for your specific application, as different rotors are optimized for different types of separations and sample volumes.
4. Horizontal rotors
These rotors are designed for high-capacity separation and are often used for separating large volumes of samples.
Horizontal centrifuge rotors are designed for use in horizontal centrifuges, which are laboratory instruments used to separate particles based on their size and density. Horizontal rotors are typically larger and more robust than other types of rotors, and are designed for use with large volumes of samples.
There are several advantages to using horizontal centrifuge rotors:
- High capacity: Horizontal rotors are capable of separating large volumes of samples, making them ideal for use in high-throughput applications.
- Gentle separation: Because the samples are suspended in a horizontal position, horizontal rotors are generally less stressful on the samples compared to vertical rotors. This can be beneficial for sensitive samples, such as cells or proteins.
- Easy loading and unloading: Horizontal rotors typically have a front-loading design, which makes it easy to load and unload samples.
- Versatility: Horizontal rotors come in a range of sizes and designs, making them suitable for a variety of applications and sample types.
- Durability: Horizontal rotors are generally more durable and long-lasting compared to other types of rotors.
It is important to choose the appropriate horizontal rotor for your specific application, as different rotors are optimized for different types of separations and sample volumes.
5. Specialty rotors
There are many other types of specialty rotors available, including those designed for use with microtubes, tubes with large diameters, and tubes with irregular shapes.
Specialty centrifuge rotors are designed for specific applications or types of samples. Some examples of specialty rotors include:
- Microcentrifuge rotors: These rotors are designed for use with microtubes and are often used for high-speed separations of small volumes of samples.
- Large-capacity rotors: These rotors are designed for use with tubes with large diameters and are often used for separating large volumes of samples.
- Irregular-shape rotors: These rotors are designed for use with tubes with irregular shapes, such as round-bottom tubes or tubes with tapered ends.
- Preparative rotors: These rotors are designed for use in preparative centrifugation, which is a process used to purify or concentrate samples.
- Thermal rotors: These rotors are designed to maintain a constant temperature during the centrifugation process, which is important for certain types of samples, such as enzymes or proteins.
- Ultracentrifuge rotors: These rotors are designed for use in ultracentrifugation, which is a high-speed centrifugation process used to separate particles based on their size and density.
It is important to choose the appropriate specialty rotor for your specific application, as different rotors are optimized for different types of separations and sample volumes.
6. Other Types of Centrifuge rotor
- Drum rotors: Drum-rotors are specialized, high-centrifugal rotors. The samples are placed in a cassette, and then vertically into the rotating rotor. The samples will not move from their vertical position. The sedimentation process works in a similar way to swing-bucket, but at higher speeds. These rotors are very efficient at density separation, but they are not suitable for pelleting.
- Zonal Rotors: Zonel rotors can be either batch- or continuous-flow. They are more commonly used than the latter and are intended to reduce the wall effect in fixed angle and swinging bucket rotors and increase the sample size.
- Elutriator Rotors: The elutriator rotor is a type of continuous flow rotor. It has recesses that hold one conical shaped separation room, whose apex points away from the axis. There’s also a bypass chamber that acts as a counterbalance and provides the fluid outlet.
Care of Rotors
The anodized protective coating on the aluminium rotor is extremely thin and does not offer much protection against corrosion. Rotors should be treated with care to avoid scratching. It is important to thoroughly wash rotors with de-ionized water. Since moisture can cause corrosion, it is best to allow them to dry upside down in warm conditions. After drying, they should be kept dry in a dry, clean environment. Only the outer surfaces of rotors can be protected with lanolin or silicone polish.
However, swiping-bucket rotors should not be fully submerged in water. The bucket hanging system can be difficult to dry. The rotors made of titanium are resistant to corrosion. To avoid vibration-induced damage to the drive shaft of a centrifuge, it is important to balance the sample loads within the specified limits. The balance of the rotor should be maintained by not running the swiping-bucket rotor with any bucket or cap removed.
Metal fatigue can occur when the rotor is being decelerated or accelerates. This can be caused by cyclic stretching or relaxing of metal. To prevent overstressing the motor and ensure safe operation, it is important to keep a detailed record of all its usage. This includes speed, duration, and number of runs. The manufacturer can then either de-rate the motor after a specified number of runs, or replace it after a specific time.
How To Operate The Centrifuge Tubes
Centrifuge tubes are cylindrical-shaped, calibrated glass containers that can be used to analyze and separate various materials in the laboratory. For the experiments, they had to fit in the slots of the centrifuge.
These devices are used by lab technicians to test liquids and solid-liquid samples. They first deposit the substances in the tubes, then secure them within the slots. The lab technicians then spin the tubes at the right speeds to ensure that the denser particles of each substance collect at the point closest to the axis, and vice versa.
There are many options for laboratory centrifuge tubes in plastic, copolymers and polypropylene.
Sizes
You can find everything from small tubes with caps for micro-centrifuges to large tubes that can hold 50,000 grams in frigerated centrifuges.
Types of centrifuge tubes
Different types of centrifuge tubes are widely used for various purposes, such as:
- Conical Bottom Tubes
- Microcentrifuge Tubes- Snap Cap
- Microcentrifuge Tubes – Screw Cap and So On
How to use a centrifuge safely
These are the key guidelines to follow when operating a centrifuge tube to avoid damage and potential injuries to lab technicians or doctors.
- You should always work on an even surface. You should ensure that the surface is level and firm.
- Balance the centrifuge tubes within the rotor is safe. For example, if you need to run a centrifuge with 20mL liquid, you can put another tube with 20mL water in the opposite hole. If the liquid is of a different density than water, it is best to balance the tubes using mass and not volume.
- Do not open the lid when the rotor is in motion. This can sometimes prove to be dangerous. There are centrifuges with safety shutoffs. The lid can be opened to prevent the rotor from spinning. Because of its inertia, the rotor will continue to spin so it is best to be cautious.
- The centrifuge tubes rotate, so there is some vibration. However, excessive wobbling could be dangerous. Double check that the tubes are properly balanced. You should not continue to run a centrifuge that is shaking, wobbling, or spinning while it is spinning.
- It is always a good idea to wear safety glasses or a face shield when working in a laboratory. It is also a good idea to wear safety glasses if you work near a centrifuge.
- While the centrifuge is still spinning, do not touch, bump, or move it.
- To avoid any disruptions, it is better to clean your centrifuge and rotor after every use.
Applications of Centrifugation
- Separations in Laboratory: There are many laboratory-scale centrifuges that can be used to separate suspended liquids or immiscible liquids in biology, chemistry, and clinical medicine.
- Separation of Isotope: There are other centrifuges that separate isotopes. The first being the Zippe type centrifuge. These centrifuges can be used in nuclear power or nuclear weapon programs.
- Industrial centrifugal separator: This coolant filtration system is used to separate particles from liquids like grinding coolant or machining coolant. It is used to separate non-ferrous particles such as silicon, glass and ceramic. It does not use any consumable parts such as filter bags. This saves the earth.
- Geotechnical centrifuge modeling: Geotechnical Centrifuge Modelling is used to test models that involve soils. To scale models, centrifuge acceleration is used to create prototype scale stresses. Problems like building foundations, earth dams and tunnels, as well as slope stability.
- Materials Synthesis: The high gravity conditions created by the centrifuge can be used in the chemical industry, casting and material synthesis. Gravitational conditions can greatly affect convection and mass transfers. Researchers found that high-gravity levels can have a significant impact on the product’s phase composition and morphology.
- Commercial applications:
- Hand-washed clothes can be dried in a standalone centrifuge, usually with a water outlet.
- To get rid of water from laundry loads, washing machines can be used as centrifuges.
- In Mission: SPACE at Epcot, Walt Disney World, centrifuges are used to propel riders. This attraction uses a combination of a centerifuge and motion simulator to give the illusion of going into space.
- Centrifuges use centrifugal acceleration in soil mechanics to match soil stresses in scale models to real-life conditions.
- Many large industrial centrifuges can be used to dry sludges in wastewater and water treatment. The resultant dry product is commonly called cake. After most solids have been removed, the centrifuge leaves behind what is known as centrate.
- For the removal of solids from drilling fluid, large industrial centrifuges can also be used by the oil industry.
- Some companies use disc-stack centrifuges to separate bitumen from water and other solids.
- Centrifuges can be used to separate cream from milk (remove fat). See Separator (milk).
References
- https://en.wikipedia.org/wiki/Centrifuge
- https://www.discoveryscientificsolutions.com/item/51
- https://www.sigmaaldrich.com/IN/en/technical-documents/technical-article/protein-biology/protein-pulldown/centrifugation-separations
- https://www.beckman.com/resources/technologies/centrifugation/principles/rotor-types
- http://origin.who.int/medical_devices/innovation/hospt_equip_8.pdf
- Serwer, BACTERIOPHAGES: SEPARATION OF, Editor(s): Ian D. Wilson, Encyclopedia of Separation Science, Academic Press, 2000, Pages 2102-2109,
- https://www.westlab.com/blog/2018/12/27/3-things-to-consider-when-choosing-a-centrifuge-tube#:~:text=Centrifuge%20tubes%20are%20available%20in,resistance%20to%20most%20organic%20solvents.
- https://www.biologydiscussion.com/biochemistry/centrifugation/centrifuge-introduction-types-uses-and-other-details-with-diagram/12489
- https://handling-solutions.eppendorf.com/sample-handling/centrifugation/safe-use-of-centrifuges/basics-in-centrifugation/
- https://www.news-medical.net/Life-Science-and-Laboratory/Microcentrifuges
- https://adarshsomani02.wordpress.com/tag/types-of-centrifuge-tubes/
- https://laboglob.com/en/centrifuge-tubes/
- https://www.mybiosource.com/learn/testing-procedures/rate-zonal-centrifugation/
- https://henderson-biomedical.co.uk/blog/centrifuge-tubes/
- Wilson, K., Walker, J. (2018). Principles and Techniques of Biochemistry and Molecular Biology. Eighth edition. Cambridge University Press: New York.
- https://www.pharmtech.com/view/centrifuges-used-pharmaceutical-manufacturing-primer