What are Mitochondria?
- Mitochondria, often referred to as the cellular powerhouses, are essential membrane-bound organelles found ubiquitously within the cytoplasm of eukaryotic cells. These organelles are pivotal in the generation of adenosine triphosphate (ATP), the primary molecule that stores and transfers energy in cells.
- The structure of a mitochondrion is intricate, characterized by a double-membrane system. The outer membrane serves as a protective barrier, while the inner membrane, folded into numerous cristae, houses the machinery for ATP synthesis. The space between these membranes is known as the intermembrane space, and the innermost compartment, enclosed by the inner membrane, is termed the matrix. Each of these compartments plays a distinct role in various mitochondrial functions.
- Historically, the term “mitochondrion” is derived from the Greek words “mitos,” meaning thread, and “chondrion,” signifying granule. This nomenclature was influenced by the organelle’s appearance under the microscope. The discovery of mitochondria can be traced back to the 19th century, with significant contributions from scientists like Richard Altmann and Carl Benda.
- One of the most intriguing aspects of mitochondria is their possession of a unique genome, separate from the nuclear DNA of the cell. This mitochondrial genome is reminiscent of bacterial DNA, leading to the widely accepted endosymbiotic theory. This hypothesis postulates that mitochondria originated from free-living prokaryotes that entered into a symbiotic relationship with ancestral eukaryotic cells. Over evolutionary time, this relationship became so integrated that the prokaryote evolved into the modern mitochondrion, a critical component of eukaryotic cells.
- Beyond their role in energy production, mitochondria are multifunctional entities. They are involved in a plethora of cellular processes, including signaling pathways, cell differentiation, and regulation of the cell cycle. Moreover, they play a crucial role in apoptosis, or programmed cell death, ensuring the removal of damaged or unnecessary cells. Given their myriad functions, it is unsurprising that mitochondrial dysfunctions are implicated in various human diseases, ranging from specific mitochondrial disorders to broader conditions like cardiac ailments and neurodegenerative diseases.
- In summary, mitochondria are indispensable organelles in eukaryotic cells, central to energy production and various cellular processes. Their unique evolutionary history and multifaceted roles underscore their significance in cellular biology and human health.
Definition of Mitochondria
Mitochondria are membrane-bound organelles in eukaryotic cells responsible for producing adenosine triphosphate (ATP), the primary energy currency of the cell.
Organization and Distribution
Mitochondria, often termed the cellular powerhouses, are intricate organelles that play a pivotal role in energy production. Their organization and distribution within cells are both diverse and dynamic, reflecting their adaptability to various cellular demands.
- General Organization: Mitochondria, though minuscule and typically invisible under standard microscopy, can be specifically stained to reveal their presence. These organelles are dynamic in nature, undergoing processes of fusion (where two mitochondria merge) and fission (where a mitochondrion divides). Collectively, the entire population of mitochondria within a cell is referred to as the “chondriome.” Intriguingly, mitochondria often form intricate networks, intertwining with the cell’s cytoskeleton and frequently associating with the endoplasmic reticulum. Their distribution often aligns with microtubules, ensuring strategic placement within the cell.
- Diverse Distribution and Structure: The morphology and distribution of mitochondria can vary significantly based on the organism, cell type, and cellular function. Some illustrative examples include:
- Mammalian Sperm Cells: These cells house between 50-75 crescent-shaped mitochondria. These mitochondria are uniquely arranged in the middle piece of the sperm, wrapping around the tail’s outer dense fibers in a helical pattern, forming what is known as the “mitochondrial sheath.”
- Myocytes: Muscle cells, or myocytes, are replete with mitochondria strategically positioned between the myofibrils. For instance, a typical ventricular cardiac myocyte can harbor up to 7,000 mitochondria, which can occupy nearly 35% of the cell’s volume.
- Mature Erythrocytes: These red blood cells are distinctive in that they lack mitochondria entirely. As a result, they rely solely on anaerobic respiration to meet their energy needs.
- Fibroblasts: These cells often contain elongated mitochondria, resulting from fusion, which can extend up to 50 μm in length.
- Plant Protoplasts: Typically, these cells possess hundreds of discrete mitochondria, which are uniformly distributed, a phenomenon facilitated by cyclosis.
- Exceptions: While most eukaryotic organisms possess mitochondria, there are notable exceptions. For instance, the eukaryote Monocercomonoides and the multicellular organism Henneguya salminicola are devoid of these organelles.
In summary, the organization and distribution of mitochondria within cells underscore their adaptability and functional versatility. Their dynamic nature, both in terms of structure and positioning, reflects their essential role in meeting the diverse energy demands of cells across various organisms and cell types.
Morphology of Mitochondria
The morphology of mitochondria, the cellular powerhouses, is a testament to their adaptability and functional diversity. Delving into the specifics of their number, shape, and size provides insights into their significance and dynamic nature within cells.
- Number: The quantity of mitochondria within a cell is not uniform but varies based on the cell’s type and its functional demands. For instance:
- Protozoans like Amoeba and Chaos chaos boast an impressive count of approximately 50,000 mitochondria.
- Sea urchin eggs contain a staggering 140,000 to 150,000 mitochondria.
- Amphibian oocytes surpass this with a count of around 300,000.
- In mammals, rat liver cells house between 500 and 1600 mitochondria.
- Green plant cells, due to the presence of chloroplasts that assume some of the mitochondria’s roles, generally have fewer mitochondria.
- Some algal cells are unique in that they may contain only a singular mitochondrion.
- Shape: The shape of mitochondria is not static but is highly dynamic, influenced by the cell’s physiological state. They can manifest in various forms:
- Filamentous or granular structures.
- Club, racket, vesicular, ring, or spherical shapes.
- In specific cells, such as primary spermatocytes or rat cells, mitochondria appear granular, while in liver cells, they adopt a club-like shape.
- Observations using time-lapse microcinematography have revealed the incredible dynamism of mitochondria. They continuously alter their structure, undergoing fusion and subsequent fission. For instance, certain euglenoid cells exhibit mitochondria that merge into a reticulate structure during daylight and dissociate during nighttime. Yeast species have also demonstrated similar morphological changes in response to varying culture conditions.
- Size: Typically, mitochondria measure between 0.5 μm to 2.0 μm, rendering them invisible under standard light microscopes. However, in some instances, they can extend up to 7 μm in length.
In conclusion, the morphology of mitochondria underscores their adaptability and functional versatility. Their dynamic nature, both in number and structure, reflects their pivotal role in meeting the diverse energy demands of cells across species.
Mitochondria Structure
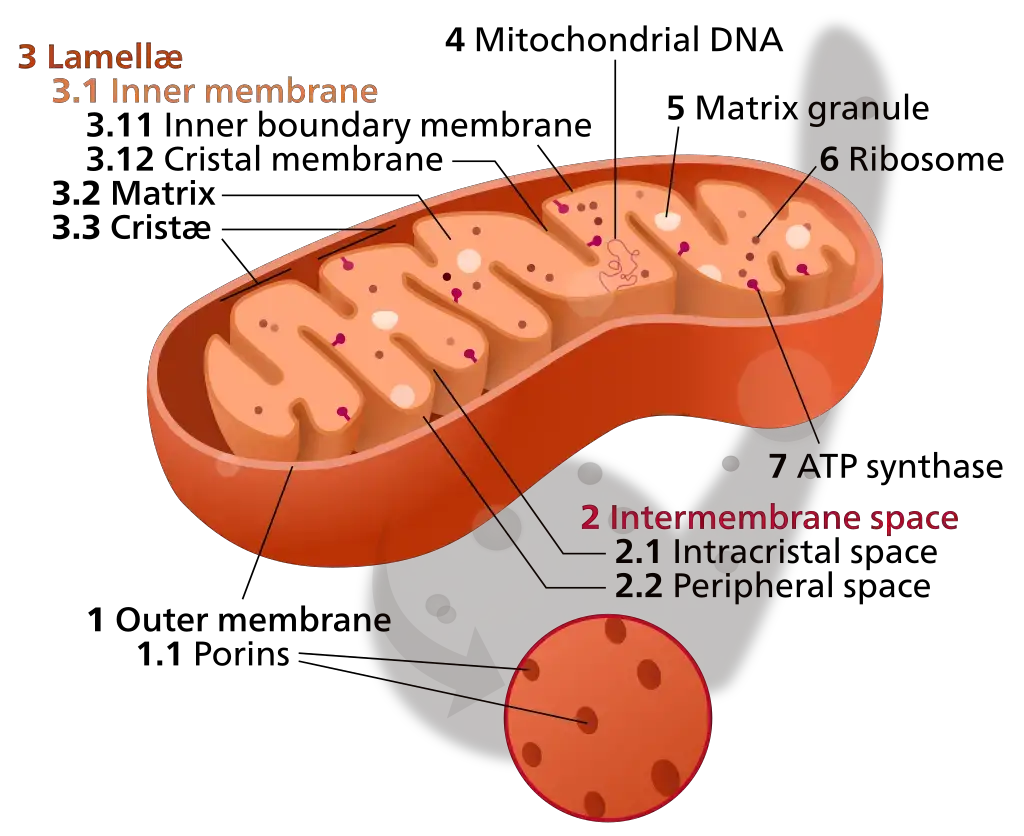
Mitochondria, often termed the “powerhouses” of the cell, are intricate organelles with a unique structural composition that facilitates their primary function: energy production. The structural intricacies of mitochondria can be delineated into five primary components:
- Outer Membrane: The outermost layer of the mitochondrion, the outer membrane, serves as a protective barrier and demarcates the organelle from the cytosol. Composed of a phospholipid bilayer interspersed with proteins, this membrane is permeable to molecules weighing less than 1000 kDa. Key components include:
- Porins: Protein structures facilitating the passage of ions, nucleotides, and small proteins.
- Translocase: Involved in transporting specific larger proteins.
- Metabolic Enzymes: These include mono-amine oxidase, kynurenine hydroxylase, and fatty-acid CoA ligase.
- MAM (Mitochondria-associated ER-membrane): These domains bridge the mitochondria and the endoplasmic reticulum, playing roles in lipid transport, calcium homeostasis, and apoptosis.
- Intermembrane Space: Situated between the outer and inner membranes, the intermembrane space plays pivotal roles in protein and ion transport, inner membrane protein assembly, and cellular respiration. This compartment accumulates protons pumped from the mitochondrial matrix during the electron transport chain’s redox reactions.
- Inner Mitochondrial Membrane: The inner membrane, larger than its outer counterpart, forms numerous folds to fit within the confines of the outer membrane. This convoluted structure is vital for oxidative phosphorylation, as it houses the electron transport chain complexes. Unlike the outer membrane, the inner membrane is highly selective, lacking porins, ensuring the maintenance of the proton gradient essential for ATP synthesis.
- Cristae: These are infoldings of the inner mitochondrial membrane, studded with proteins from the electron transport chain. Cristae augment the surface area, optimizing the efficiency of oxidative phosphorylation. Cells with heightened ATP demands typically exhibit mitochondria with an increased number of cristae.
- Matrix: Encased by the inner membrane, the mitochondrial matrix is a viscous fluid containing multiple copies of mitochondrial DNA, ribosomes, and a plethora of metabolic enzymes. The Krebs cycle, a central metabolic pathway, unfolds within this space, underscoring its significance in cellular energetics.
In summation, the structural design of mitochondria is a testament to its pivotal role in cellular metabolism. Each component, from the protective outer membrane to the enzyme-rich matrix, collaboratively ensures the efficient production of ATP, the cell’s energy currency.
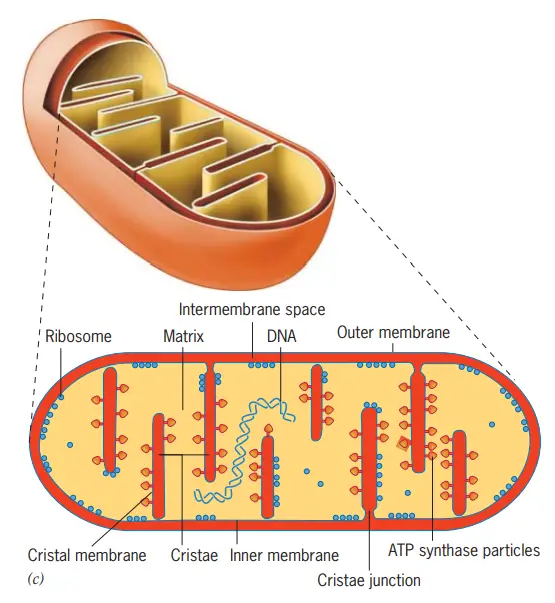
Origin and Evolution of Mitochondria
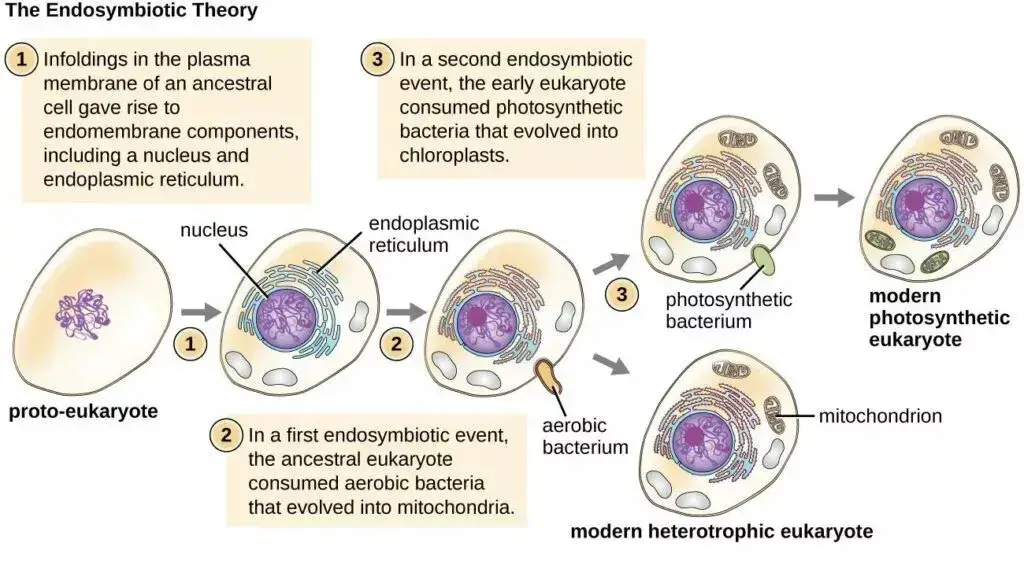
The origin and evolution of mitochondria have long been subjects of scientific inquiry, given the organelle’s pivotal role in eukaryotic cells. Mitochondria are present in the majority of eukaryotic cells, encompassing both plant and animal cells. However, they are absent in prokaryotic organisms, including bacteria.
Two primary hypotheses have been proposed to elucidate the evolutionary trajectory of mitochondria:
- Endosymbiotic Hypothesis: This theory is the most widely endorsed among the scientific community. It posits that the mitochondria we observe today evolved from a free-living aerobic prokaryote, likely an α-proteobacterium. This prokaryotic organism was engulfed by an ancestral pre-eukaryotic cell. Instead of being digested, this engulfed bacterium established a symbiotic relationship with its host. As evolution progressed, this internalized bacterium underwent significant changes: it shed its cell wall and transferred or lost much of its DNA that wasn’t beneficial to the host. This hypothesis is buttressed by several compelling similarities between mitochondria and bacteria, including:
- Both possess circular DNA.
- The ribosomes in mitochondria (70S) closely resemble those in bacteria in terms of size and structure.
- The outer mitochondrial membrane and bacterial cell membrane both contain porins.
- Cardiolipin is present in both the inner mitochondrial membrane and the bacterial cell membrane.
- Mitochondria replicate through binary fission, independent of the host cell’s replication mechanisms.
- Autogenous Hypothesis: This theory suggests that mitochondria emerged from the ancestral eukaryotic cell itself. The genesis of mitochondria is proposed to have resulted from the clustering of specific genes from the primary genome, followed by compartmentalization due to the invagination of the plasma membrane.
While the endosymbiotic theory is the more widely accepted of the two, it’s worth noting that there are exceptions in nature. For instance, Monocercomonoides, a eukaryotic species, has been identified as lacking mitochondria. Instead of relying on mitochondria for energy production, it metabolizes nutrients directly from its surroundings.
In conclusion, while the endosymbiotic hypothesis remains the predominant theory explaining the origin of mitochondria, the evolutionary history of this organelle continues to be a dynamic field of study. The intricacies of its relationship with host cells and the nuances of its evolutionary trajectory underscore the complexity and marvel of cellular evolution.
Components of Mitochondria
Component | Description | Key Features/Components |
---|---|---|
Outer Membrane | The outermost barrier of the mitochondrion, separating the contents of the intermembrane space from the cytosol. | – Composed of a phospholipid bilayer with proteins and enzymes. – Permeable to molecules up to 1000 kDa. – Contains Porins, Translocase, Metabolic Enzymes, and MAM (Mitochondria-associated ER-membrane). |
Intermembrane Space | The space located between the outer and inner mitochondrial membranes. | – Essential for protein and ion transport. – Houses protons pumped from the mitochondrial matrix during redox processes of the electron transport chain. |
Inner Mitochondrial Membrane | Larger than the outer membrane and appears wrinkled due to its many folds. | – Primary site for oxidative phosphorylation. – Contains electron transport chain complexes. – Highly impermeable and lacks porins. – Maintains proton gradient for ATP synthesis. |
Cristae | Compartments formed by the many folds of the inner mitochondrial membrane. | – Studded with electron transport chain proteins. – Increases surface area for oxidative phosphorylation. – Cells with higher ATP/energy requirements have more cristae. |
Matrix | The space enclosed within the inner mitochondrial membrane. | – Houses mitochondrial DNA and various metabolic enzymes. – Site of the Krebs (TCA) cycle. |
Mitochondrial DNA
Mitochondria, often termed the “powerhouses” of the cell, possess a distinctive feature: their own independent genome. This mitochondrial DNA (mtDNA) is distinct from the nuclear DNA and has several unique attributes. Here, we delve into the intricacies of mitochondrial DNA, with a particular focus on its structure and function in humans.
- Diversity in Structure: Mitochondrial DNA varies in its structure across different species. While humans possess a circular form of mtDNA, certain plants, fungi, and protists have linear mtDNA. This diversity in structure is a testament to the evolutionary adaptability of mitochondria.
- Size Variation: The size of the mitochondrial genome can differ considerably among organisms. Human mitochondrial genomes are relatively compact, encompassing approximately 16 kb. In contrast, yeast and plants have more expansive mitochondrial genomes, measuring around 80 kb and 200 kb, respectively. Notably, the larger size does not necessarily equate to a higher gene count.
- Human Mitochondrial DNA Features:
- Base Pairs: Human mtDNA comprises 16,569 base pairs, forming a compact genetic structure.
- Gene Count: The human mitochondrial genome houses a total of 37 genes.
- Oxidative Phosphorylation: Out of these 37 genes, 13 are dedicated to coding proteins that play a pivotal role in oxidative phosphorylation, the primary energy-producing process in cells.
- Ribosomal RNA (rRNA): Two of the genes are responsible for coding rRNA molecules, specifically the 16S and 12S rRNA. These rRNAs are integral to the mitochondrial ribosomes and protein synthesis.
- Transfer RNA (tRNA): 22 genes in the mitochondrial genome code for tRNA molecules. These tRNAs are essential for translating the mitochondrial mRNA into proteins.
In essence, mitochondrial DNA is a compact, efficient genome that encodes essential components for the mitochondrion’s primary function: energy production. Its unique structure and gene composition set it apart from nuclear DNA, underscoring the evolutionary significance and adaptability of mitochondria.
Transmission of mitochondrial DNA
The transmission of mitochondrial DNA (mtDNA) in humans and many other organisms predominantly follows a matrilineal pattern. This unique inheritance mechanism has several key characteristics:
- Maternal Inheritance: In the vast majority of cases, offspring inherit their mitochondrial DNA solely from the mother. This is because the mitochondria in the sperm, which are located in the tail, are typically discarded during fertilization. As a result, only the mitochondria present in the egg contribute to the mtDNA of the developing embryo.
- Heteroplasmy: While it is generally accepted that mtDNA is maternally inherited, there have been rare documented instances of heteroplasmy. This refers to the presence of multiple types of mitochondrial DNA within an individual, suggesting that, on rare occasions, paternal mtDNA might not be completely excluded and can coexist with maternal mtDNA.
- Mitochondrial Eve: The concept of maternal inheritance has led to the identification of the “mitochondrial Eve.” This theoretical woman, who lived approximately 100,000 to 200,000 years ago, is considered the most recent common matrilineal ancestor of all modern humans. By analyzing variations in mtDNA, scientists can trace back human maternal lineages to this single individual.
- Exceptions in Other Species: While maternal inheritance of mtDNA is the norm in humans, there are exceptions in other organisms. Some species exhibit biparental inheritance, where both parents contribute to the offspring’s mtDNA. However, the implications and evolutionary reasons for these exceptions remain subjects of ongoing research.
In conclusion, while the primary mode of mtDNA transmission in humans is through the mother, the mechanisms and exceptions to this rule in other species highlight the complexity and adaptability of genetic inheritance.
Transcription and Replication of mitochondrial DNA
Mitochondrial DNA (mtDNA) possesses unique characteristics that differentiate its transcription and replication processes from those of nuclear DNA. The mitochondria have evolved to maintain their own distinct genetic machinery, which is reminiscent of their ancestral bacterial origins.
- Replication Machinery: Unlike nuclear DNA, which utilizes a variety of DNA polymerases for replication, mtDNA relies primarily on DNA polymerase gamma complex for its replication. This complex is encoded by the POLG gene. This specialized polymerase ensures the accurate duplication of the mitochondrial genome.
- Transcriptional Variations: The transcription process of mtDNA exhibits certain deviations from the universal genetic code. These “violations” or variations in the genetic code are believed to be remnants of the evolutionary history of mitochondria, which are thought to have originated from an ancient symbiotic relationship between primitive eukaryotic cells and bacteria.
- Codon Variations: The codons in mtDNA sometimes code for different amino acids or functions compared to their counterparts in nuclear DNA. This divergence in the genetic code can lead to different protein sequences and functions. The table below illustrates some of these codon variations:
Codon | Universal Code | Mitochondrial DNA |
---|---|---|
UGA | Stop codon | Tryptophan |
AUA | Isoleucine | Methionine |
AGA/AGG | Arginine | Stop codon |
In conclusion, the transcription and replication mechanisms of mitochondrial DNA underscore its unique evolutionary lineage and its semi-autonomous nature within eukaryotic cells. These processes highlight the intricate balance between the evolutionary remnants of ancient symbiosis and the integrated cellular functions of modern eukaryotes.
Differences between the mitochondrial and nuclear DNA in humans
In human cells, DNA is primarily located in two distinct regions: the nucleus and the mitochondria. While both types of DNA carry genetic information vital for cellular function, they exhibit several differences in terms of structure, function, and inheritance. Here, we elucidate the key distinctions between mitochondrial DNA (mtDNA) and nuclear DNA (nDNA):
- Structure:
- Mitochondrial DNA: Circular in shape, reminiscent of bacterial DNA.
- Nuclear DNA: Linear, organized into chromosomes.
- Size:
- Mitochondrial DNA: Contains a concise 16,569 base pairs.
- Nuclear DNA: Vastly larger, encompassing approximately 3 billion base pairs.
- Copy Number per Cell:
- Mitochondrial DNA: Multiple copies can be found within a single mitochondrion, and a cell can have thousands of mtDNA copies.
- Nuclear DNA: Each cell contains a singular copy of the nuclear genome.
- Protection:
- Mitochondrial DNA: Lacks an enveloping membrane.
- Nuclear DNA: Encased within the nuclear envelope, providing an added layer of protection.
- Organization:
- Mitochondrial DNA: Open structure, without the intricate packaging seen in nuclear DNA.
- Nuclear DNA: Organized into chromatin, with DNA wound around histone proteins.
- Dependency:
- Mitochondrial DNA: Relies on certain gene products from nuclear DNA for its function.
- Nuclear DNA: Operates independently of mtDNA.
- Genetic Code:
- Mitochondrial DNA: Utilizes a slightly different genetic code than the universal one.
- Nuclear DNA: Adheres to the universal genetic code.
- Gene Arrangement:
- Mitochondrial DNA: Features overlapping genes.
- Nuclear DNA: Genes are distinct, without overlap.
- Noncoding Regions:
- Mitochondrial DNA: Minimal noncoding regions.
- Nuclear DNA: A substantial portion, approximately 93%, consists of noncoding sequences.
- Inheritance:
- Mitochondrial DNA: Exclusively maternally inherited, passed down from mother to offspring.
- Nuclear DNA: Derived from both parents, with chromosomes inherited from each.
- Association with Histone Proteins:
- Mitochondrial DNA: Does not associate with histone proteins, lying free in a manner similar to bacterial nucleoids.
- Nuclear DNA: Interacts with histone proteins, forming nucleosomes that aid in DNA packaging and regulation.
In summary, while both mitochondrial and nuclear DNA are integral to human genetics and cellular function, they exhibit stark differences in their structure, function, and inheritance patterns. These distinctions underscore the evolutionary origins and specialized roles of these two DNA types within the cell.
Feature | Mitochondrial DNA | Nuclear DNA |
---|---|---|
Structure | Circular | Linear |
Size | 16,569 base pairs | 3 billion base pairs |
Copy Number per Cell | Multiple copies | One copy |
Protection | Non enveloped | Enveloped |
Organization | Open DNA | DNA packed in chromatin |
Dependency | Dependent on certain nuclear DNA gene products | Independent of mitochondrial DNA |
Genetic Code | Does not follow the universal genetic code | Follows the universal genetic code |
Gene Arrangement | Genes are overlapping | Genes are not overlapping |
Noncoding Regions | Very few noncoding regions | 93% of DNA is noncoding |
Inheritance | Maternal inheritance | Both maternal and paternal inheritance |
Association with Histone Proteins | No involvement of histone proteins; mtDNA lies histone-free like bacterial cells | Histone proteins are involved in the packaging of nuclear DNA into nucleosomes |
Isolation of Mitochondria
The study of mitochondria has been approached through three primary methodologies:
- Direct Observation of Mitochondria:
- Mitochondria possess a low refractive index, making their study in living cells a challenge.
- Despite this, they become discernible in cells cultured in a petri dish, especially when observed using phase contrast or darkfield microscopy.
- The application of the vital stain Janus green significantly aids in this examination. This stain colors living mitochondria a greenish-blue hue due to its interaction with the cytochrome oxidase system present in the mitochondria. The stain remains in its oxidized (colored) form within the mitochondria, while in the surrounding cytoplasm, it is reduced to a colorless leukobase.
- For in vivo studies of mitochondrial metabolism, fluorescent dyes like rhodamine 123 have been employed due to their heightened sensitivity.
- Cytochemical Marking of Mitochondrial Enzymes:
- Histochemical markers are enzymes that are specific to different mitochondrial compartments. Examples include cytochrome oxidase for the inner membrane, monoamine oxidase for the outer membrane, malate dehydrogenase for the matrix, and adenylate kinase for the intermembrane space.
- Isolation:
- Differential centrifugation is a technique used to separate mitochondria from other cellular components.
- Homogeneous fractions of mitochondria have been successfully isolated from various tissues, including the liver, skeletal muscle, heart, among others.
- During differential centrifugation, mitochondria sediment at densities ranging from 5,000 to 24,000 g. However, in living cells, they settle intact at the centrifugal pole at densities between 20,000 to 400,000 g during ultracentrifugation.
- Density gradient centrifugation has been employed to segregate the inner and outer mitochondrial membranes. This involves inducing swelling to separate the two layers by rupturing the outer membrane and allowing the inner membrane and matrix to contract.
- Detergents like digitonin and lubrol are commonly used for this purpose. The outer membrane, being lighter and more fragile than the inner membrane, requires centrifugal force for separation.
- The removal of the outer membrane using digitonin results in the formation of mitoplasts, which consist of an inner membrane with unfolded cristae and a matrix. This “folded-bag” appearance of the isolated outer membrane is evident upon negative staining.
- The distinction between these two membranes and compartments has facilitated the precise localization of specific mitochondrial enzyme systems.
Chemical Composition of Mitochondria
Mitochondria, the cellular powerhouses, exhibit varied chemical compositions across different animal and plant cells. However, a general composition reveals that mitochondria consist of:
- Proteins (65%-70%):
- The distribution of proteins varies across the mitochondrial structure. For instance, in liver mitochondria, 67% of the protein is located in the matrix, 21% in the inner membrane, 6% in the outer membrane, and 6% in the intermembrane space.
- Each of these regions houses specific proteins that facilitate distinct cellular functions.
- Lipids (25%-30%):
- The lipid content predominantly comprises phospholipids (90%), including lecithin and cephalin.
- Cardiolipin, a unique phospholipid abundant in the inner membrane, serves as a barrier, restricting the movement of numerous ions and molecules.
- The lipid composition also includes cholesterol (less than 5%) and a combination of free fatty acids and triglycerides (5%).
- RNA (0.5-1%):
- Essential for protein synthesis within the mitochondria.
- DNA (Trace amounts):
- Mitochondrial DNA is crucial for encoding certain proteins and enzymes necessary for cellular respiration.
Enzymatic Distribution:
- Outer Membrane Enzymes:
- Houses enzymes vital for mitochondrial lipid synthesis and the conversion of lipid substrates for matrix processing.
- Key enzymes include monoamine oxidase, rotenone-insensitive NADH-cytochrome-C-reductase, kynurenine hydroxyalase, and fatty acid CoA ligase.
- Intermembrane Space Enzymes:
- This region contains enzymes that phosphorylate other nucleotides using the ATP exiting the matrix.
- Notable enzymes include adenylate kinase and nucleoside diphosphate kinase.
- Inner Membrane Enzymes:
- This membrane is the site for the redox reactions of the respiratory chain.
- It houses ATP synthase, succinate dehydrogenase, -hydroxybutyrate dehydrogenase, ubiquinone or coenzyme Q10, non-heme copper and iron, and enzymes involved in electron transport pathways.
- Mitochondrial Matrix Enzymes:
- The matrix is densely packed with enzymes essential for pyruvate and fatty acid oxidation, as well as the citric acid or Krebs cycle.
- Key enzymes include malate dehydrogenase, isocitrate dehydrogenase, fumarase, aconitase, citrate synthase, -keto acid dehydrogenase, and -oxidation enzymes.
In addition to these primary components, the mitochondrial matrix also contains inorganic electrolytes, nucleotides, and nucleotide coenzymes, all of which play pivotal roles in cellular metabolism and energy production.
Dysfunction and Disease
Mitochondria, often referred to as the powerhouses of the cell, play a pivotal role in cellular energy production. Given their significance, it is unsurprising that any malfunction within these organelles can lead to a spectrum of diseases. The root causes of mitochondrial dysfunction can be multifaceted, ranging from mutations in the mitochondrial genome, alterations in the nuclear genome that encodes vital mitochondrial components, to the detrimental impacts of specific drugs.
Mitochondrial Diseases:
Mitochondrial diseases predominantly arise due to accumulated mutations in the mitochondrial genome. Some of the notable diseases include:
- Leber’s Hereditary Optic Neuropathy (LHON): A condition that primarily affects vision.
- Leigh’s Syndrome: A severe neurological disorder that typically arises in the first year of life.
- NARP Syndrome (Neurogenic muscle weakness, Ataxia, and Retinitis Pigmentosa): A condition that affects various parts of the body, especially the nervous system.
- Kearns-Sayre Syndrome: A disorder that affects many parts of the body, particularly the eyes.
- CPEO (Chronic Progressive External Ophthalmoplegia): A condition characterized by slowly progressive weakness of the external eye muscles.
- MELAS Syndrome (Mitochondrial Encephalomyopathy, Lactic Acidosis, and Stroke-like episodes): The most prevalent mitochondrial disorder, which affects many of the body’s systems, especially the brain and nervous system.
- Pearson’s Syndrome: A condition that affects the bone marrow and pancreas.
It’s worth noting that these diseases are maternally inherited, meaning they are passed down exclusively from the mother. Typically, tissues with high energy demands, such as the brain, eyes, and skeletal muscles, are most affected by mitochondrial diseases due to their heavy reliance on mitochondria for energy.
Mitochondrial Replacement Therapy (MRT):
In recent advancements, the Mitochondrial Replacement Therapy (MRT) has emerged as a groundbreaking technique, especially in the realm of In Vitro Fertilization (IVF). MRT was notably employed when a Jordanian couple, with the mother suffering from Leigh’s syndrome, sought to have a genetic child. The procedure involves retaining the cytoplasm with healthy mitochondrial DNA from a donor’s ovum while transferring the nuclear DNA from the intended mother, thereby excluding the defective mitochondrial genome. The resultant hybrid ovum is then fertilized with the father’s sperm. The embryo thus produced possesses healthy mitochondrial DNA from the donor and nuclear DNA from both parents. This technique marked a significant milestone when the first child was born using MRT on 6th April 2016 in Mexico.
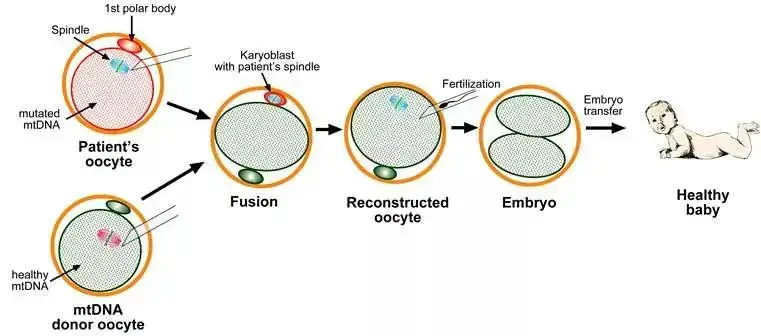
Relationships to aging
The intricate relationship between mitochondria and aging remains a subject of extensive research, with many facets yet to be fully elucidated. Several mechanisms have been postulated to explain the role of mitochondria in the aging process, emphasizing the importance of these cellular powerhouses in determining longevity and age-related changes. Here, we delve into some of the proposed mechanisms:
- Generation of ROS: Mitochondria are the primary producers of reactive oxygen species (ROS). ROS can inflict damage on macromolecules, including DNA. This oxidative damage has been linked to aging, with DNA mutations leading to mitochondrial dysfunction and further ROS release.
- Decreased Respiratory Capacity: Over time, a decline in mitochondrial respiratory activity is observed. This decline disrupts cellular metabolism and is implicated in aging. Moreover, reduced functional capacity can also enhance ROS production.
- Mitophagy: Mitophagy refers to the selective degradation of mitochondria by autophagy. It helps regulate the number of dysfunctional mitochondria. As age advances, mitophagy diminishes, leading to an increase in malfunctioning mitochondria.
- Mitochondrial Mutations: Mitochondrial DNA is more susceptible to mutations compared to nuclear DNA, primarily due to less efficient DNA repair mechanisms. Over time, these mutations accumulate, resulting in a functional decline of mitochondria.
- Decline in Stem Cell Population: Stem cells play a pivotal role in tissue maintenance. Mitochondria influence stem cell function, and a decline in stem cell populations is observed with aging.
- Cellular Senescence: Mitochondria play a role in regulating cellular aging by modulating the cell’s metabolic profile. Significant changes in the cellular metabolome are closely linked to the cell’s senescent state.
- Mitochondrial Unfolded Protein Response (UPRmt): UPRmt is activated by mitochondrial stress, leading to protein misfolding within mitochondria. The nucleus detects this and elevates proteases and chaperones to address the misfolded proteins. A decline in protease levels is associated with aging and age-related diseases, including cardiovascular disease and diabetes.
- DAMPs (Damage-Associated Molecular Patterns): Released during stress, DAMPs include mitochondrial DNA, which is released into the bloodstream during mitochondrial stress. Elevated mitochondrial DNA levels in the blood are associated with age and a chronic low-grade inflammatory state.
- Mitochondrial Derived Peptides: Peptides like humanin and MOTS-c, derived from mitochondria, have been linked to preventing Alzheimer’s disease and age-related insulin resistance. They are also correlated with increased longevity.
- Mitochondrial Metabolism: Mitochondria produce NAD, a substrate for sirtuins, proteins associated with longevity. As age progresses, cellular NAD levels decrease.
In summary, mitochondria play a multifaceted role in the aging process, influencing various cellular mechanisms and responses. Understanding these relationships is crucial for developing interventions to mitigate age-related changes and promote healthy aging.
Mitochondria Functions
Mitochondria, often referred to as the “powerhouses” of the cell, play pivotal roles in a myriad of cellular functions. Their significance extends beyond energy production, encompassing various aspects of cellular metabolism and regulation. Here, we elucidate the multifaceted functions of mitochondria:
- ATP Synthesis: The paramount function of mitochondria is the synthesis of adenosine triphosphate (ATP), the cell’s primary energy currency. Through a process termed oxidative phosphorylation, ATP molecules are generated. This process involves a series of redox reactions in the electron transport chain, located in the inner mitochondrial membrane. Electrons, donated by molecules like NADH and FADH2, are transferred through a series of complexes, creating an electrochemical gradient. This gradient drives the FOF1 ATP synthase complex, facilitating ATP synthesis.
- Thermogenesis: In certain tissues, such as brown adipose tissue, mitochondria release energy as heat rather than storing it in ATP. This uncoupling of oxidative phosphorylation is crucial for thermoregulation in young individuals.
- Calcium Homeostasis: Mitochondria, in conjunction with the endoplasmic reticulum, are pivotal in maintaining intracellular calcium levels. The domains between mitochondria and the endoplasmic reticulum, known as MAM, play a crucial role in this regulation, ensuring cellular survival and optimal function.
- Immune Regulation: Mitochondria influence various aspects of the immune response, including immune cell activation, differentiation, and survival. They achieve this through metabolic alterations, inflammatory responses, mitochondrial dynamics, and signaling between mitochondria and the endoplasmic reticulum.
- Apoptosis: Mitochondria are central to the intrinsic pathway of apoptosis. DNA damage and other cues can trigger this pathway, leading to an imbalance in pro- and anti-apoptotic proteins. This results in the release of cytochrome-c from mitochondria, initiating a cascade that culminates in cell death.
- Stem Cell Regulation: Mitochondrial metabolism is integral to the homeostasis of somatic stem cells. Mutations in mitochondrial DNA can compromise this homeostasis, leading to tissue degeneration and accelerated aging.
- Biochemical Synthesis: Mitochondria are involved in the synthesis of various biochemicals. Intermediates from the TCA cycle serve as precursors for the synthesis of certain amino acids and other compounds. For instance, oxaloacetate and α-ketoglutaric acid are precursors for aspartic acid and glutamic acid, respectively.
In summary, mitochondria are multifunctional organelles that play a central role in cellular energy production, regulation, and homeostasis. Their diverse functions underscore their importance in maintaining cellular health and function.
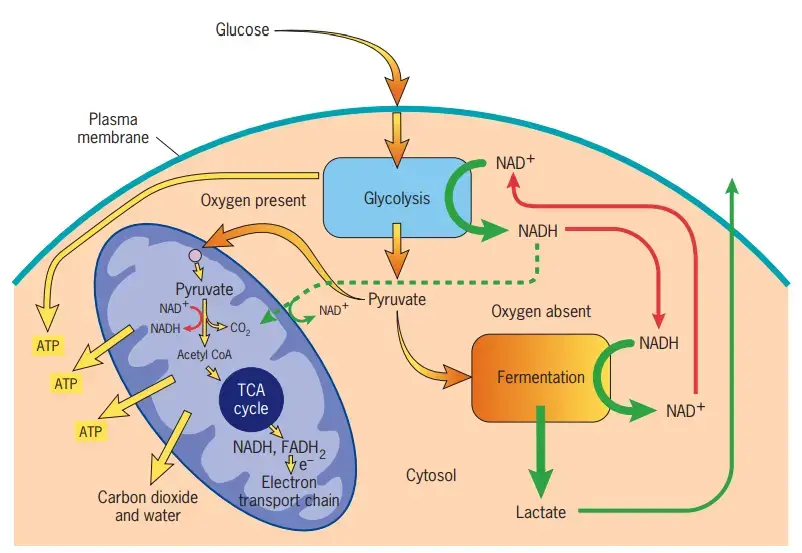
Quiz
What is the primary function of mitochondria in cells?
a) Protein synthesis
b) ATP production
c) DNA replication
d) Lipid storage
Which part of the mitochondria is responsible for oxidative phosphorylation?
a) Outer membrane
b) Matrix
c) Cristae
d) Intermembrane space
How is mitochondrial DNA primarily inherited?
a) Paternally
b) Maternally
c) Both from mother and father
d) Randomly
Which of the following is NOT a function of mitochondria?
a) Cellular respiration
b) Photosynthesis
c) Calcium homeostasis
d) Apoptosis regulation
What is the shape of mitochondrial DNA in humans?
a) Linear
b) Circular
c) Triangular
d) Hexagonal
Which enzyme is specific to the outer membrane of mitochondria and plays a role in cellular metabolism?
a) Citrate synthase
b) Cytochrome c oxidase
c) Monoamine oxidase
d) ATP synthase
Which process helps in maintaining the number of dysfunctional mitochondria in check?
a) Glycolysis
b) Mitophagy
c) Fermentation
d) Krebs cycle
Which syndrome is NOT associated with mitochondrial DNA mutations?
a) Down syndrome
b) Leigh’s syndrome
c) Kearns Sayre syndrome
d) MELAS syndrome
Which of the following is a unique lipid found in the inner mitochondrial membrane?
a) Cholesterol
b) Phosphatidylcholine
c) Cardiolipin
d) Sphingomyelin
Which organelle is believed to have a symbiotic relationship with ancient eukaryotic cells, leading to the formation of mitochondria?
a) Lysosome
b) Golgi apparatus
c) Bacteria
d) Endoplasmic reticulum
FAQ
What are mitochondria?
Mitochondria are organelles found in most eukaryotic cells that are responsible for producing energy in the form of ATP through cellular respiration.
What is the structure of mitochondria?
Mitochondria have an outer membrane, an inner membrane, and a matrix. The inner membrane is highly folded, forming structures called cristae, which increase the surface area for energy production.
What is the function of mitochondria?
The primary function of mitochondria is to produce ATP through cellular respiration, which involves breaking down glucose and other molecules to release energy.
How do mitochondria produce ATP?
Mitochondria produce ATP through a process called oxidative phosphorylation, which involves a series of chemical reactions that take place in the inner membrane.
How many mitochondria are in a cell?
The number of mitochondria in a cell can vary depending on the cell type and its energy needs. For example, muscle cells have many more mitochondria than skin cells.
Can mitochondria reproduce?
Yes, mitochondria have their own DNA and can reproduce through a process called fission.
What is mitochondrial disease?
Mitochondrial disease is a group of rare genetic disorders that affect the function of mitochondria and can lead to a wide range of symptoms, including muscle weakness, seizures, and developmental delays.
Can mitochondrial dysfunction contribute to aging?
Some research suggests that mitochondrial dysfunction may play a role in aging and age-related diseases, such as Alzheimer’s and Parkinson’s.
Can mitochondria be targeted with drugs?
Yes, drugs that target mitochondria are being developed for a variety of conditions, including cancer, neurodegenerative diseases, and metabolic disorders.
What is the endosymbiotic theory of mitochondria?
The endosymbiotic theory suggests that mitochondria were once free-living bacteria that were engulfed by another cell and evolved a symbiotic relationship with their host, eventually becoming integrated into the cell as an organelle.
References
- Cooper GM. The Cell: A Molecular Approach. 2nd edition. Sunderland (MA): Sinauer Associates; 2000. Mitochondria. Available from: https://www.ncbi.nlm.nih.gov/books/NBK9896/
- Gray, M. W. (2013). Mitochondria. Brenner’s Encyclopedia of Genetics, 430–432. doi:10.1016/b978-0-12-374984-0.00957-8
- Gray, M. W. (2001). Mitochondria. Encyclopedia of Genetics, 1215–1217. doi:10.1006/rwgn.2001.0835
- Manoli, I., Alesci, S., & Chrousos, G. P. (2007). Mitochondria. Encyclopedia of Stress, 754–761. doi:10.1016/b978-012373947-6.00559-6
- Liao, P.-C., Bergamini, C., Fato, R., Pon, L. A., & Pallotti, F. (2019). Isolation of mitochondria from cells and tissues. Methods in Cell Biology. doi:10.1016/bs.mcb.2019.10.002
- https://biologydictionary.net/mitochondria/
- https://www.sciencedirect.com/topics/physics-and-astronomy/mitochondria
- https://www.livescience.com/50679-mitochondria.html
- https://www.biologyonline.com/dictionary/mitochondrion
- https://www.genome.gov/genetics-glossary/Mitochondria#:~:text=Mitochondria%20are%20membrane%2Dbound%20cell,called%20adenosine%20triphosphate%20(ATP).
- https://www.britannica.com/science/mitochondrion