What is Mass Spectrometry (MS)?
- Mass Spectrometry (MS) stands as a pivotal analytical technique in the realm of scientific research, offering insights into the molecular composition of samples. This method is instrumental in discerning the mass-to-charge ratio (m/z) of ions, thereby facilitating the identification of chemical constituents within a sample.
- The core principle of MS revolves around the ionization of a sample, transforming it into charged particles. This ionization typically ensues through the bombardment of the sample with electrons, converting it into a stream of positively charged ions. Some of these ions might fragment, leading to a myriad of charged particles, each with distinct mass-to-charge ratios.
- Following ionization, the ions are propelled through an electric or magnetic field. Their trajectory within this field is contingent upon their mass-to-charge ratio. Ions with similar m/z values will exhibit analogous deflection patterns. Subsequent to their journey through the field, these ions are detected, often employing mechanisms like electron multipliers. The culmination of this process is the generation of a mass spectrum, a graphical representation delineating the intensity of detected ions against their respective mass-to-charge ratios.
- The mass spectrum serves as a fingerprint of the sample, revealing its elemental or isotopic signature. Not only does it provide data on the masses of individual particles and molecules, but it also sheds light on the chemical structures of various compounds present in the sample. By juxtaposing the obtained mass spectrum with known spectra, one can ascertain the identity of molecules, even elucidating their structural intricacies.
- The versatility of MS is evident in its widespread application across diverse scientific domains. From chemistry and biology, where it aids in the identification of unknown compounds, to forensic science, where it assists in the detection of substances, the utility of MS is undeniable. High-resolution mass spectrometry, equipped with state-of-the-art ionization and detection apparatus, can pinpoint the formula and structure of nearly any compound with remarkable precision.
- Historically, MS has played a pivotal role in groundbreaking discoveries, such as the identification of neon isotopes. By analyzing the mass spectrum of neon, scientists were able to discern the presence of isotopes, underscoring the technique’s capability to not only detect but also differentiate between isotopes based on their mass.
- In summation, Mass Spectrometry is an indispensable tool in the scientific arsenal, offering unparalleled insights into the molecular world. Through its ability to measure the mass-to-charge ratio of ions and generate detailed mass spectra, it provides a comprehensive understanding of the chemical composition and structure of samples.
Definition of Mass Spectrometry (MS)
Mass Spectrometry (MS) is an analytical technique used to identify the chemical composition of a sample by measuring the mass-to-charge ratio of its ions, resulting in a mass spectrum that reveals the molecular and structural information of the sample.
Mass Spectrometry Principle
Mass Spectrometry operates on a foundational principle rooted in the behavior of ions under the influence of electric and magnetic fields. At its core, this technique involves the ionization of molecules, typically achieved by subjecting them to a beam of high-energy electrons. This bombardment results in the fragmentation of molecules, producing a myriad of ions, predominantly positive in nature. Each of these ions possesses a specific mass-to-charge (m/e) ratio, which, for the majority of ions with a singular charge, equates to their molecular mass.
Upon ionization, these ions are channeled through a series of electric and magnetic fields. Drawing from Newton’s second law of motion, the trajectory and behavior of these ions within the fields are intrinsically linked to their mass. Specifically, the law elucidates the relationship between an object’s mass and its acceleration when subjected to a force. In the context of Mass Spectrometry, ions of differing m/e ratios exhibit distinct deflections when traversing through the electric or magnetic fields.
Ultimately, these ions converge on a detector, where their presence is registered and subsequently translated into a mass spectrum. This spectrum serves as a graphical representation, plotting the intensity of detected ions against their respective mass-to-charge ratios. Through this, the molecular composition and structural intricacies of the sample under investigation are unveiled.
Working of Mass Spectrometry (MS)
- Sample Introduction: The sample, which can be solid, liquid, or gas, is introduced into the mass spectrometer.
- Ionization: The sample molecules are ionized by bombarding them with high-energy electrons, causing them to break into charged fragments or ions.
- Separation: The ions are separated based on their mass-to-charge ratio (m/e ratio) by subjecting them to an electric or magnetic field while accelerating them.
- Deflection: Ions with different mass-to-charge ratios experience different levels of deflection in the electric or magnetic field. Lighter ions undergo greater deflection, while heavier ions undergo less deflection.
- Detection: The separated ions are detected by a charged particle detector, such as an electron multiplier. The detector records the signals produced by the ions as they reach it.
- Mass Spectrum: The recorded signals are used to generate a mass spectrum, which displays the relative abundance of ions as a function of their mass-to-charge ratio.
- Interpretation: The mass spectrum is analyzed to identify the atoms or molecules in the sample. Known masses can be correlated with identified masses, or characteristic fragmentation patterns can be used for identification.
By following these steps, mass spectrometry enables scientists to characterize and identify molecules, determine molecular masses, study chemical reactions, and gain insights into the composition and structure of substances.
Instrumentation of Mass Spectrometry (MS)
The instrumentation of mass spectrometry (MS) involves several key components and processes. Here’s an overview of the main parts and steps involved:
1. Sample Inlet:
- The sample, stored in a reservoir, is introduced into the mass spectrometer.
- Molecules from the sample reach the ionization chamber at low pressure in a steady stream through a pinhole called a “molecular leak.”
2. Ionization:
- The atoms in the sample are ionized, typically by bombarding them with a stream of electrons.
- This ionization process involves knocking off one or more electrons, resulting in the formation of positive ions.
- Most of the positive ions carry a charge of +1.
- Various ionization techniques are utilized, such as electron ionization (EI-MS), chemical ionization (CI-MS), and desorption techniques (FAB).
3. Acceleration:
- The ions produced are accelerated to ensure they all have the same kinetic energy.
- Positive ions pass through three slits with voltages in decreasing order.
- The middle slit carries intermediate and final ions at zero volts.
4. Deflection:
- Ions are deflected by a magnetic field based on their masses.
- Lighter ions are deflected more than heavier ions.
- The deflection is also influenced by the number of positive charges carried by an ion, with ions carrying more positive charges experiencing more deflection.
5. Detection:
- The beam of ions passing through the mass analyzer is detected by a detector based on the mass-to-charge ratio (m/e ratio).
- When an ion hits the metal box in the detector, the charge is neutralized by an electron jumping from the metal onto the ion.
- Various types of analyzers are used in mass spectrometry, including magnetic sector mass analyzers, double-focusing analyzers, quadrupole mass analyzers, time-of-flight analyzers (TOF), ion trap analyzers, and ion cyclotron analyzers.
Mass Spectrometry Diagram
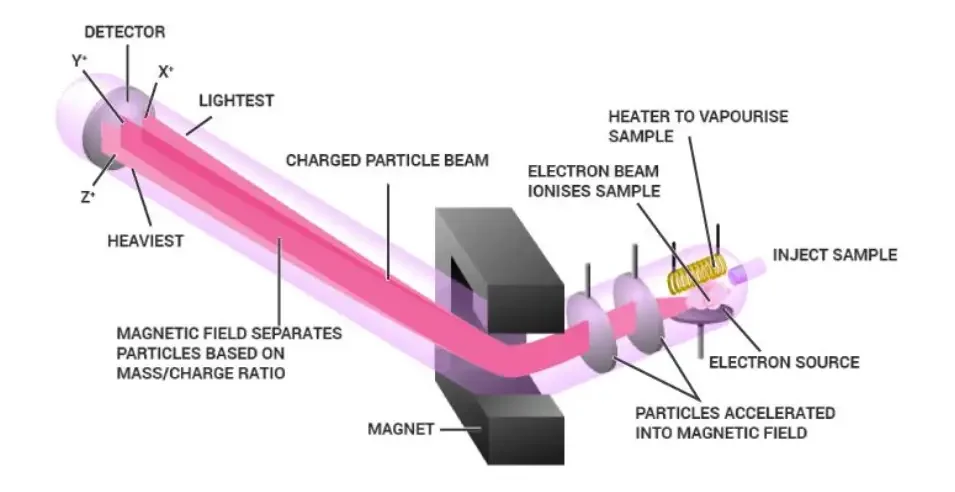
How Does Mass Spectrometry Work?
Mass spectrometry is a versatile analytical technique used to determine the composition, structure, and properties of molecules. Here is an explanation of how mass spectrometry works:
- Ionization (Ion Source): The sample is first vaporized and ionized to form charged particles or ions. Various ionization techniques can be employed, such as electron impact ionization, electrospray ionization, or matrix-assisted laser desorption ionization (MALDI), depending on the nature of the sample. The ions may carry a positive or negative charge, depending on the ionization method used.
- Ion Separation/Isolation (Mass Analyzer): The ionized particles are then subjected to a series of electric or magnetic fields within the mass analyzer. The purpose of the mass analyzer is to separate the ions based on their mass-to-charge ratio (m/z). Different types of mass analyzers, such as time-of-flight (TOF), quadrupole, magnetic sector, or ion trap analyzers, can be used to achieve this separation. The ions are deflected or accelerated by the fields in a way that ions with the same m/z ratio follow a specific path or trajectory.
- Detection (Ion Detector): The separated ions are directed towards an ion detector, which measures the abundance of ions as a function of their m/z ratio. The detector records the signals produced by the ions and converts them into electrical signals that can be further processed and analyzed. The resulting data is typically displayed as a mass spectrum, where the x-axis represents the m/z values and the y-axis represents the relative abundance of ions at each m/z value.
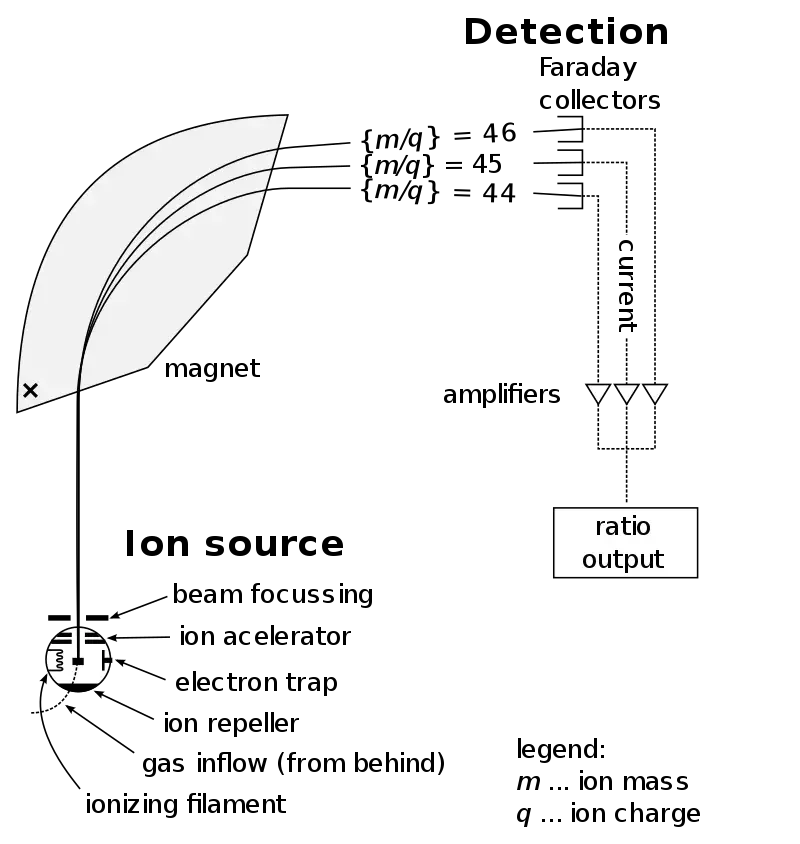
By analyzing the mass spectrum, scientists can identify the atoms or molecules present in the sample by comparing the m/z values with known masses of specific compounds. Additionally, the fragmentation pattern of ions can provide valuable information about the structure and composition of the molecules.
Overall, mass spectrometry enables qualitative and quantitative analysis of chemical substances, allowing researchers to gain insights into the composition, structure, and behavior of a wide range of molecules in various fields such as chemistry, biology, medicine, environmental science, and more.
How To Read A Mass Spectrometry Spectrum
Understanding the information displayed on the graph is required to read a mass spectrometry spectrum. The following is a description of how to read a mass spectrometry spectrum:
- Y-Axis (Relative Intensity or Abundance): The spectrum’s y-axis displays the relative intensity or abundance of ions at certain m/z values. The height of the peaks or bars represents the abundance of ions at specific m/z values. The peak with the greatest intensity is known as the base peak, and it reflects the ion in the spectrum with the greatest relative abundance.
- Base Peak: The base peak is the highest peak in the spectrum and represents the ion with the greatest relative abundance. It may or may not, however, correspond to the molecular ion. The base peak may be a fragment ion rather than the entire molecular ion in some situations.
- Peaks and m/z Values: Each peak on the spectrum corresponds to a different m/z value, which shows the ions’ mass-to-charge ratio. When ions have a single charge, the m/z value is equal to the compound’s molecular mass. On the x-axis of the spectrum, the m/z values rise from left to right.
- Molecular Ion: The molecular ion is often represented as the peak with the highest m/z value. The molecular ion represents the entire molecule under investigation and offers information on its relative molecular mass. It is vital to highlight that the molecular ion may not be noticed in some situations, particularly if the molecule is fragile or quickly split.
- Fragments: In addition to the molecular ion, the spectrum may contain peaks corresponding to fragment ions. Fragment ions are formed when a molecule fragments during the ionisation process. These pieces reveal important details about the molecule’s structure and content. The fragment pattern, together with the molecular ion, can be utilised to identify the sample.
- Database Comparison: When attempting to identify a sample, it is frequently advantageous to compare the mass spectrum to a database of recognised spectra. The National Institute of Standards and Technology (NIST) has a database of mass spectra for comparison and identification.
Scientists can identify the chemicals contained in the sample and get insights into their molecular structures and properties by analysing the peaks, m/z values, relative intensities, and comparing the spectrum to known databases.
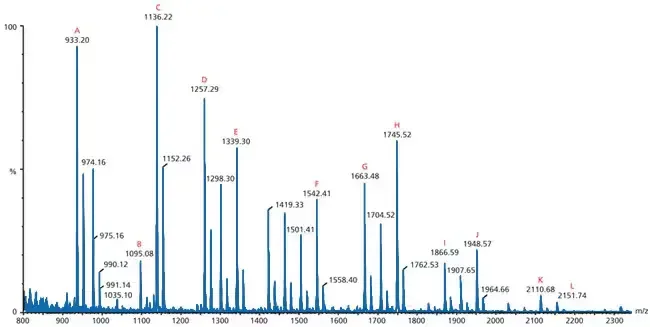
Specimen Requirements and Procedure
For the successful application of mass spectrometry, meticulous specimen preparation is paramount. The objective is to render the sample amenable to ionization, ensuring it is in either the liquid or gaseous phase. This is predominantly achieved through chromatographic techniques, which serve as preliminary separation methods.
- Gas Chromatography (GC):
- Principle: Gas chromatography operates by segregating components of a gaseous mixture. The separation is contingent upon intrinsic molecular attributes such as shape, size, molecular weight, and boiling point.
- Procedure: The specimen is first diluted and subsequently vaporized within the chromatograph. Herein, the components are partitioned based on their differential interactions with the stationary phase. Post-separation, these gaseous constituents are channeled into the mass spectrometer for in-depth analysis.
- Specimen Requirement: It’s imperative that samples destined for gas chromatography are volatile. This ensures that they can transition to the gaseous phase without undergoing decomposition within the mass spectrometry apparatus.
- Liquid Chromatography (LC):
- Principle: This technique hinges on the differential interactions of the sample components with the mobile and stationary phases of the chromatograph.
- Procedure: The polarity of the sample components plays a pivotal role. Molecules with polarities divergent from the mobile phase exhibit accelerated migration through the chromatograph column. This results in the stratification of the sample into distinct bands, each representing a specific component. These separated entities are then primed for mass spectrometric analysis.
Beyond chromatography, other avant-garde techniques have been developed to facilitate sample preparation:
- Electrospray Ionization: This method employs elevated voltages to segregate sample components, rendering them suitable for mass spectrometric analysis.
- Fast Atom Bombardment: Here, an ion beam is directed at the sample, facilitating the generation of ions from a solid phase.
The versatility of mass spectrometry is underscored by the diverse array of samples amenable to analysis. This includes, but is not limited to, proteins, nucleic acids, lipids, and fatty acids.
In essence, the efficacy of mass spectrometry is intrinsically linked to the meticulous preparation of specimens. Through techniques like gas and liquid chromatography, samples are rendered compatible with the mass spectrometer, ensuring accurate and comprehensive analyses.
Testing Procedures in Mass Spectrometry
Mass spectrometry, a cornerstone in analytical chemistry, necessitates a series of intricate steps to elucidate the composition and properties of samples. Herein, we delineate the key components and procedures integral to this technique:
- Ionization:
- Principle: At the heart of mass spectrometry lies the ionization process, wherein atoms are transformed into cations by electron removal. The instrument exclusively analyzes these positive ions. Remarkably, even elements that are inherently non-ionic or negatively charged are converted into cations within the mass spectrometer.
- Mechanism: An internal electric field emits electrons, which, upon collision with sample molecules, engender cations.
- Electron Ionization (EI): Predominantly employed in GC/MS, EI uses a filament subjected to an electric potential, typically around 70 eV. The ensuing high-energy electrons bombard the molecules, culminating in charged molecular ions and fragments. These ions and their relative proportions serve as reproducible markers for compound identification.
- Soft Ionization Techniques: Techniques like Electrospray Ionization (ESI) and Atmospheric Pressure Chemical Ionization (APCI) are conducted at atmospheric pressure, preserving the molecular ion’s integrity. ESI employs voltage, heat, and air to generate progressively smaller droplets, concentrating ions for analysis. APCI, on the other hand, uses heat and a corona discharge to ionize the solvent and subsequently the sample components.
- Acceleration:
- Principle: To ensure uniform kinetic energy, all cations undergo acceleration. These cations traverse through specific slits in the apparatus, entering the ion beam, setting the stage for mass-to-charge ratio-based separation.
- Deflection:
- Principle: A magnetic field steers the cations based on their mass and charge. The relationship is such that lighter ions and those with higher positive charges experience greater deflection.
- Outcome: This deflection mechanism facilitates the determination of the mass-to-charge ratio (m/z). For ions with a charge of 1+, the m/z value typically mirrors the ion’s mass or molecular weight.
- Detection:
- Principle: The detector quantifies the ions, selectively registering positive ions while neutral ones are evacuated by the vacuum.
- Mechanism: The electron multiplier is a prevalent detection method. Here, a cascade of electrons is generated as ions strike a series of dynodes with incrementally increasing potentials. This results in a substantial signal amplification, often exceeding a factor of a million.
- Vacuum:
- Principle: To mitigate ion collisions and ensure optimal separation, mass spectrometers operate under reduced pressure.
- Outcome: The low-pressure environment, akin to a vacuum, ensures that neutral molecules are siphoned off, allowing cations to traverse the apparatus unhindered.
In summation, mass spectrometry is a multi-faceted analytical technique, underpinned by a series of meticulously orchestrated steps. From ionization to detection, each component plays a pivotal role in ensuring accurate and comprehensive sample analysis.
Results, Reporting, and Interpretation in Mass Spectrometry
Mass spectrometry, a cornerstone in analytical chemistry, offers a detailed insight into the mass-to-charge ratio of ions present in a compound. The culmination of this analysis is represented as a mass spectrum, a graphical depiction that plots the relative abundance of ions against their respective mass-to-charge ratios. This spectrum serves as a fingerprint of the compound, revealing its elemental and isotopic composition, thereby elucidating its chemical identity and structure.
Key components of the mass spectrum, integral to its interpretation, include:
- Molecular Ion Peak (Parent Peak):
- Definition: This peak corresponds to the intact molecule or the compound under investigation.
- Significance: The m/z value of the molecular ion peak mirrors the molecular weight of the compound. For instance, in the case of hexane, an m/z value of 86 signifies its molecular weight.
- Base Peak:
- Definition: Among all the ions detected in the sample, the base peak represents the ion with the highest relative abundance.
- Significance: Serving as a reference, the intensity of the base peak is designated as 100%. Subsequently, the intensities of all other peaks in the spectrum are gauged relative to this benchmark.
- M+1 Peak:
- Definition: This peak, albeit of lower intensity, signifies the presence of isotopes in the sample.
- Significance: If a compound has a molecular weight of 96 g/mol, the m/z value would be 96. An m+1 peak at an m/z of 97 indicates the presence of isotopes, such as those of carbon or hydrogen. It might seem counterintuitive for an ion to exhibit a mass surpassing that of the compound, but this anomaly is attributed to the inherent isotopic variations of elements.
- M+2 Peak:
- Definition: Analogous to the m+1 peak, the m+2 peak also denotes isotopic presence.
- Significance: Elements like chlorine and oxygen, which boast multiple isotopes, can manifest as an m+2 peak when present in a compound.
In essence, the mass spectrum, with its array of peaks, offers a comprehensive overview of the compound’s composition. Proper interpretation of these peaks, from the parent peak to the isotopic peaks, is pivotal for accurate identification and characterization of the compound. Through this, mass spectrometry stands as an invaluable tool in the realm of analytical chemistry.
What is a Quadrupole?
- A quadrupole is an important component of many mass spectrometers and is used to analyse ions. It’s a type of mass analyzer that can separate and measure ions depending on their mass-to-charge ratio (m/z).
- The quadrupole is made up of four parallel metal rods placed in a square or rectangular pattern. These rods are often electrically charged and constructed of metal, such as stainless steel. Two of the rods are positively charged, while the other two are negatively charged. The voltages applied to the rods generate an electric field within the quadrupole.
- Ions enter the quadrupole and are subjected to the combined impact of the electric fields produced by the charged rods. The ions follow a precise course through the quadrupole when the electric fields oscillate rapidly. The quadrupole functions as a filter by altering the voltages applied to the rods, allowing ions with specified m/z values to pass through while filtering out others.
- The stability of ion paths within the quadrupole provides the basis for the filtering concept. Ions with specified mass-to-charge ratios that match the quadrupole voltage settings will have stable trajectories and pass through to the detector, whereas ions with different m/z values will be deflected and will not pass through.
- Different ions can be selectively transmitted via the quadrupole by altering the voltages supplied to the rods, allowing for the investigation of a wide range of substances. This procedure is also known as mass filtering or mass selection.
- Quadrupoles are commonly employed in mass spectrometers of many types, such as quadrupole mass spectrometers (QMS) and quadrupole ion trap mass spectrometers (QIT-MS). They have a high selectivity and sensitivity, enabling exact measurement and identification of ions based on their mass-to-charge ratios.
- To summarise, a quadrupole is a key component of mass spectrometry that functions as a mass analyzer. It uses electric fields formed by charged rods to selectively filter ions based on their mass-to-charge ratios, allowing accurate detection of analytes’ composition and structure in a sample.
Mass Spectrometry Detectors
Mass spectrometry detectors are critical components of mass spectrometers, which capture and measure ions in order to create data for analysis. These detectors are critical in transforming ion signals into detectable electrical or optical signals that can be processed and analysed.
Ions are deflected through the mass analyzer based on their mass-to-charge ratios (m/z). The strength and configuration of the applied electric or magnetic fields determine the extent of deflection. A detector is placed at various deflection locations to count the number of ions that reach it.
In mass spectrometry, there are various types of detectors, each with its unique operating principle and advantages. Among the most frequent types of detectors are:
- Electron Multiplier: To enhance the ion signal, this detector employs a succession of electron multiplication stages. When ions collide with a surface coated with a substance that emits secondary electrons upon impact, these electrons are accelerated and multiplied by a cascade effect, resulting in a considerably magnified signal.
- Faraday Cup: Faraday cup detectors collect ions by electrostatically attracting them to a metal surface, typically a cup or collector electrode. When ions touch the surface, their charge is transferred to the cup, resulting in a detectable electric current. This current is proportional to the number of ions and can be measured to estimate the intensity of the ions.
- Ion-to-Photon Detectors: Ion-to-photon detectors transform ions into photons, which are then detected and quantified. A photomultiplier tube (PMT) is a popular example, in which ions create photons through interactions with a scintillation substance. A photomultiplier detects the photons and amplifies the signal for measurement.
Because of the low abundance of ions and the sensitivity necessary for accurate detection, the detectors often produce very weak signals. To overcome this difficulty, the signals are amplified using specialised electronics or amplifiers, allowing even minute ion currents or charges to be precisely monitored and recorded.
After that, the detector output is processed and analysed to provide a mass spectrum. The mass spectrum represents the intensity of ions at different m/z values, providing information on the analytes present in the sample’s composition, structure, and abundance.
In conclusion, mass spectrometry detectors are critical in catching and quantifying ions for examination. They detect ions by measuring the generated charge or current caused by ion collisions on surfaces. Detectors generate mass spectra by amplifying and processing signals, providing significant insights into the chemical composition and properties of analysed substances.
The Mass Analyzer
The mass analyzer is an important component of a mass spectrometer that sorts and separates ions depending on their mass-to-charge ratio (m/z). It is critical in determining the mass and abundance of ions in a sample.
There are several varieties of mass analyzers available, each with its own set of pros and disadvantages, as well as varying capabilities in terms of speed, resolution, and technical criteria. Among the most widely used mass analyzers are:
- Quadrupole Analyzer: A quadrupole analyzer is made up of four parallel metal rods that form an electric field. Ions with certain m/z ratios can flow through the quadrupole filter and reach the detector by adjusting the electric field intensity and frequencies delivered to the rods. Others are filtered out. Quadrupole analyzers are popular because of their versatility, stability, and ability to scan for specific m/z values.
- Time-of-Flight (TOF) Analyzer: TOF analyzers measure the time it takes for ions to traverse a known distance in order to calculate their m/z values. Ions are propelled into a flight tube and separated based on their velocities, with lighter ions reaching the detector first. TOF analyzers provide fast analysis and are especially suitable for analysing big macromolecules.
- Magnetic Sector Analyzers: Magnetic sector analyzers deflect ions based on their m/z ratios using a mix of magnetic and electric fields. Ions follow curved trajectories within the analyzer, and individual ions can be steered towards the detector by altering the magnetic field strength. Magnetic sector analyzers provide accurate m/z readings with great resolution.
- Ion Trap Analyzer: Ion trap analyzers use electromagnetic fields to confine ions in a three-dimensional space. Ions with varying m/z ratios can be selectively expelled and detected by adjusting the trapping fields. Ion trap analyzers are well-known for their versatility, sensitivity, and capacity to conduct tandem mass spectrometry investigations.
- Orbitrap Analyzer: Orbitrap analyzers use an electric and magnetic field combination to capture ions in orbital motion. The ions oscillate, causing a current to be sensed and utilised to calculate their m/z ratios. Orbitrap analyzers provide excellent resolution, precision, and sensitivity, which is especially useful for high-resolution mass measurements.
The type of mass analyzer selected is determined by the individual analytical requirements, such as the necessary resolution, mass range, speed, and sensitivity. The mass analyzer is often used in conjunction with an ion detection device, such as electron multipliers or ion-to-photon detectors, to catch and measure the ions that pass through the analyzer.
Mass spectrometers can provide valuable information about the masses, structures, and abundances of ions in a sample by utilising various mass analyzers and detection systems, allowing identification and characterization of compounds in fields such as chemistry, biology, and materials science.
Applications of Mass Spectrometry (MS)
Mass spectrometry (MS) is a versatile analytical technique with a wide range of applications. Here are some of the most important applications of mass spectrometry:
- Environmental Monitoring and Analysis: Mass spectrometry is used to analyse soil, water, and air pollutants, as well as to assess water quality and monitor environmental toxins.
- Geochemistry: Mass spectrometry is important in determining age, researching soil and rock composition, and conducting surveys for oil and gas exploration.
- Chemical and Petrochemical Industry: Mass spectrometry is used in the chemical and petrochemical industries to ensure the purity and composition of chemicals and petrochemical products.
- Biomolecule Analysis: Mass spectrometry is used to identify the structures of biomolecules such as carbohydrates and nucleic acids. It is also used to sequence biopolymers like proteins and oligosaccharides.
- Molecular Mass Determination: Mass Spectrometry is used to determine the molecular masses of peptides, proteins, and oligonucleotides, which aids in their characterization and identification.
- Breath Analysis: During surgery, mass spectrometry is used to analyse gases in patients’ breath, allowing for real-time monitoring and assessment of the patient’s metabolic state.
- Forensic Analysis: Mass spectrometry is useful in identifying drug misuse and detecting drug metabolites in blood, urine, and saliva samples, which aids forensic investigations.
- Aerosol Particle Analysis: Mass spectrometry is used to analyse aerosol particles, allowing researchers to better understand their composition, origins, and effects on air quality and human health.
- Food Safety and Pesticide Analysis: Pesticide residues in food are determined by mass spectrometry, assuring food safety and regulatory compliance.
- Isotope Analysis: Mass spectrometry is used to identify element isotopes, allowing researchers to analyse isotopic compositions and trace elemental sources.
- Pharmaceutical and Drug Development: Pharmaceutical and drug development rely on mass spectrometry to aid in drug discovery, drug metabolism investigations, and pharmacokinetic analyses.
- Proteomics and Metabolomics: Mass spectrometry is widely employed in proteomics and metabolomics investigations to analyse and quantify proteins and metabolites, offering insights into biological systems and disease causes.
These are only a handful of the numerous applications of mass spectrometry. Its adaptability, sensitivity, and capacity to offer specific molecular information make it a valuable tool in a wide range of scientific disciplines and enterprises.
Lab Techniques Like Mass Spectrometry
In the realm of scientific research, a plethora of analytical techniques exists, each tailored to specific applications and offering unique insights into the nature of samples. Mass spectrometry stands as a cornerstone among these, but several other methodologies share its objective of elucidating the composition and properties of substances. Herein, we delve into some of these instrumental techniques:
- Gas Chromatography (GC): This technique involves the volatilization of a sample, which is then transported by an inert gas through a column packed with a stationary phase. As the sample constituents traverse the column, they separate based on their interaction with the stationary phase. A detector at the column’s end registers the separated components, enabling their identification and quantification.
- Liquid Chromatography (LC): Analogous to gas chromatography, this method employs a liquid mobile phase to transport the sample through a column. The differential interactions between the sample components and the column’s stationary phase facilitate their separation. Post-separation, the constituents can be subjected to further analytical techniques for comprehensive analysis.
- Secondary Ion Mass Spectrometry (SIMS): Tailored for the examination of solid surfaces and thin films, SIMS involves bombarding the sample with a primary ion beam. This bombardment results in the ejection of secondary ions from the sample’s surface. The mass-to-charge ratios of these secondary ions are then analyzed, providing insights into the sample’s surface composition and structure.
- Tandem Mass Spectrometry (MS/MS): This advanced technique employs two sequential mass spectrometers. The first spectrometer ionizes the sample and selects a specific ion of interest. This ion is then fragmented, and the resulting product ions are analyzed in the second spectrometer. Predominantly used in proteomics and metabolomics, MS/MS offers detailed molecular information, especially concerning complex biological molecules.
- Inductively Coupled Plasma Mass Spectrometry (ICP-MS): Renowned for its sensitivity, ICP-MS vaporizes the sample using an inductively coupled plasma source. The resultant ions are then introduced into a mass spectrometer. Given its heightened sensitivity to trace metals, ICP-MS finds extensive application in environmental science, particularly in assessing water quality.
In summation, while mass spectrometry remains a pivotal tool in analytical chemistry, a suite of complementary techniques exists, each catering to specific research needs and offering nuanced insights into the nature of samples.
Clinical Significance of Mass Spectrometry
Mass spectrometry (MS) stands as a pivotal analytical tool, with its applications spanning a myriad of scientific domains, from forensic toxicology to clinical research. The technique’s versatility is underscored by its capacity to elucidate the mass-to-charge ratio of ions, which is subsequently represented in a mass spectrum. This spectrum, plotting ions’ relative abundance against their mass-to-charge ratios, serves as a molecular fingerprint, revealing the elemental and isotopic composition of compounds.
Disease Diagnosis and Biomarkers:
- MS is instrumental in clinical settings, primarily for disease diagnosis via biomarker analysis. Biomarkers, indicators of normal or pathological processes, play a crucial role in diagnosis, prognosis, and therapeutic monitoring.
- Enzymes, such as amylase, serve as biomarkers for conditions like pancreatitis. Similarly, natriuretic peptides are pivotal in cardiovascular disease prognosis.
- MS can analyze biomarkers ranging from small molecules to macromolecules, with samples derived from diverse biological sources, including plasma, urine, and saliva. Chromatographic techniques, like liquid or gas chromatography, enhance the spectrometer’s sensitivity and specificity, refining clinical decision-making.
Proteomics and Lipidomics:
- Proteins and lipids are quintessential biomarkers. Proteomics, the study of the entire protein set of an organism, leverages MS to quantify proteome differences between biological states. MS facilitates the spectral analysis of proteins in various biological samples.
- Lipidomics, the comprehensive study of lipids, employs MS to characterize lipid oxidation reactions, elucidating the biological properties of lipids within the human body.
COVID-19 Testing:
- Amidst the COVID-19 pandemic, MS emerged as a diagnostic tool. Nasal swab samples were subjected to MS, with the resultant spectrum categorizing patients as COVID-19 positive or negative.
Pharmaceutical Analysis:
- MS plays an indispensable role in pharmaceutical drug analysis. The technique’s ionization capability discerns the molecular constituents of drugs, expediting and enhancing clinical sample analysis.
Glycomics:
- Oligosaccharides, complex molecules comprising multiple monosaccharides, present analytical challenges. MS, however, is revolutionizing glycomics, enabling comprehensive studies of sugar structures and biosynthesis.
Oligonucleotide Analysis:
- Oligonucleotides, linear polymers of nucleotides, undergo various modifications. MS identifies these modifications, determining their structure and position, and aids in oligonucleotide sequencing.
Environmental and Forensic Analysis:
- MS’s applications extend to environmental analysis, encompassing drinking water testing, pesticide quantitation, and pollution monitoring. In forensic science, MS aids in trace evidence analysis, arson investigations, drug abuse confirmations, and explosive residue identifications.
In summation, mass spectrometry’s clinical significance is profound, with its applications permeating diverse scientific realms. From disease diagnosis to environmental monitoring, MS stands as an analytical beacon, driving advancements in myriad scientific disciplines.
Fields of Application of Mass Spectrometry
Mass spectrometry (MS) finds applications in various fields due to its versatility and ability to provide detailed molecular information. Here are some key fields where mass spectrometry is applied:
- Elemental and Isotopic Analysis:
- Physics: Mass spectrometry is used for elemental identification and isotopic abundance measurement in physical sciences.
- Radiochemistry: It aids in the analysis of radioisotopes and their decay products.
- Geochemistry: Mass spectrometry is employed to study elemental and isotopic compositions of rocks, minerals, and environmental samples.
- Organic and Bio-organic Analysis:
- Organic Chemistry: Mass spectrometry helps identify and characterize organic compounds, study reaction mechanisms, and assess purity.
- Polymer Chemistry: It aids in the analysis of polymer structures and composition, as well as the identification of polymer additives.
- Biochemistry and Medicine: Mass spectrometry is extensively used in proteomics, metabolomics, and lipidomics to study biological molecules and their interactions.
- Structural Elucidation:
- Organic Chemistry: Mass spectrometry is employed to determine the structure and connectivity of organic molecules, including the identification of functional groups.
- Polymer Chemistry: It helps characterize the structure and sequence of polymers, including copolymers and block polymers.
- Biochemistry and Medicine: Mass spectrometry aids in the structural characterization of biomolecules, such as proteins, peptides, nucleic acids, and carbohydrates.
- Characterization of Ionic Species and Chemical Reactions:
- Physical Chemistry: Mass spectrometry is used to study ion-molecule reactions, gas-phase kinetics, and energy profiles of ions.
- Thermochemistry: It helps determine thermodynamic properties and reaction energetics of ions and molecules.
- Mass Spectral Imaging:
- Biomedical Studies: Mass spectrometry imaging enables the spatial mapping of molecules within biological samples, aiding in disease diagnosis and understanding biological processes.
- Material Sciences: Mass spectrometry imaging allows the characterization and visualization of molecular distribution on surfaces and materials.
These applications demonstrate the wide range of uses for mass spectrometry across different scientific disciplines, including physics, chemistry, biochemistry, medicine, and material sciences. Mass spectrometry plays a crucial role in elemental analysis, structural elucidation, chemical reaction studies, and imaging, providing valuable insights into the composition, structure, and behavior of various substances.
Advantages of Mass Spectrometry
- Sensitivity: Mass spectrometry is highly sensitive, capable of detecting and analyzing substances at very low concentrations (parts per million or even parts per billion). It can identify trace amounts of compounds in complex mixtures.
- Identification of Unknown Components: Mass spectrometry is an excellent tool for identifying or confirming the presence of unknown components in a sample. It can help in the discovery of new compounds or the characterization of impurities.
- Molecular Mass Determination: Mass spectrometry allows for precise determination of the molecular masses of compounds. This is particularly useful in determining the purity of samples and calculating the molar mass of molecules.
- Structural Analysis: Mass spectrometry can provide valuable structural information about molecules, helping in the elucidation of chemical structures, including the identification of functional groups and the sequencing of biomolecules.
- Versatility: Mass spectrometry is applicable to a wide range of compounds, from small organic molecules to large biomolecules. It can be used in various fields, including chemistry, biochemistry, environmental science, and pharmaceutical analysis.
Disadvantages of Mass Spectrometry
- Isomer Differentiation: Mass spectrometry alone may not be able to distinguish between optical isomers (enantiomers) or geometric isomers, as they produce similar mass spectra. Additional techniques, such as chromatography or nuclear magnetic resonance (NMR), are often required for complete structural characterization.
- Identification of Hydrocarbons: Mass spectrometry may face challenges in accurately identifying hydrocarbons, as many hydrocarbons produce similar fragmented ions. This limitation can be overcome by combining mass spectrometry with complementary techniques like gas chromatography.
- Instrument Complexity: Mass spectrometry instruments can be complex and require specialized training to operate and interpret the data. Maintenance and calibration of the instruments can also be time-consuming and costly.
- Sample Preparation: Sample preparation for mass spectrometry analysis can be demanding, especially for complex matrices. Purification, extraction, and derivatization techniques may be required to enhance detection and separation.
Quiz
What is the primary purpose of mass spectrometry?
a) To measure the volume of a sample
b) To determine the boiling point of a compound
c) To measure the mass-to-charge ratio of ions
d) To determine the solubility of a substance
Which of the following is NOT a component of a mass spectrometer?
a) Ion source
b) Mass analyzer
c) Detector
d) Refractometer
In mass spectrometry, what does the term “m/z” stand for?
a) Mass/Zone
b) Mass/Zero
c) Mass/Charge
d) Molecular/Zone
Which ionization technique is considered a “soft” ionization method?
a) Electron Ionization (EI)
b) Electrospray Ionization (ESI)
c) Fast Atom Bombardment (FAB)
d) Both b and c
In a mass spectrum, which peak represents the ion with the highest relative abundance?
a) Molecular Ion Peak
b) Base Peak
c) M+1 Peak
d) M+2 Peak
Which of the following samples would NOT be suitable for analysis by Gas Chromatography-Mass Spectrometry (GC/MS)?
a) Volatile organic compounds
b) Gaseous samples
c) Large proteins
d) Small drug molecules
What is the primary advantage of Tandem Mass Spectrometry (MS/MS)?
a) It can analyze larger samples.
b) It provides higher resolution.
c) It can further fragment ions for detailed analysis.
d) It operates at higher temperatures.
Which of the following is a common use of mass spectrometry in forensic science?
a) Determining the age of fossils
b) Analyzing trace evidence
c) Studying cellular structures
d) Measuring the pH of a solution
In Electrospray Ionization (ESI), what is primarily responsible for producing smaller droplets from the liquid sample?
a) High pressure
b) Magnetic field
c) Combination of voltage, heat, and air
d) Ultraviolet light
Which of the following is NOT a typical sample type analyzed in mass spectrometry?
a) Plasma
b) Urine
c) Solid metals
d) Saliva
FAQ
What is mass spectrometry?
Mass spectrometry is an analytical technique used to measure the mass-to-charge ratio (m/z) of ions. It provides information about the composition, structure, and abundance of molecules in a sample.
What are the different types of mass analyzers?
Some commonly used mass analyzers are quadrupole, time-of-flight (TOF), ion trap, magnetic sector, and Fourier transform ion cyclotron resonance (FT-ICR). These analyzers differ in their principles of operation and offer different capabilities in terms of resolution, mass range, and scan speed.
How is mass spectrometry used in proteomics?
Mass spectrometry plays a crucial role in proteomics by enabling the identification, quantification, and characterization of proteins. It is used for protein sequencing, post-translational modification analysis, protein-protein interaction studies, and biomarker discovery.
What is tandem mass spectrometry?
Tandem mass spectrometry (MS/MS) involves using multiple stages of mass analysis to obtain more detailed structural information about molecules. It often involves precursor ion selection, fragmentation of selected ions, and analysis of the resulting fragment ions.
What is high-resolution mass spectrometry?
High-resolution mass spectrometry (HRMS) provides enhanced mass accuracy and resolving power compared to conventional mass spectrometry. It allows for more precise determination of molecular masses and the differentiation of closely related compounds.
What types of ionization techniques are used in mass spectrometry?
Common ionization techniques include electron impact ionization (EI), electrospray ionization (ESI), matrix-assisted laser desorption/ionization (MALDI), and atmospheric pressure chemical ionization (APCI). Each technique has its advantages and is suitable for different types of samples.
What are the applications of mass spectrometry?
Mass spectrometry has diverse applications in fields such as chemistry, biology, pharmaceuticals, forensics, environmental analysis, and proteomics. It is used for compound identification, quantification, structural elucidation, and biomolecule analysis, among others.
How does mass spectrometry work?
Mass spectrometry involves ionizing a sample, separating the ions based on their m/z ratio, and detecting and analyzing the ions. The ions are generated, separated, and detected using various components such as ion sources, mass analyzers, and detectors.
How is mass spectrometry used in drug discovery?
Mass spectrometry is used in drug discovery for lead compound identification, pharmacokinetic studies, metabolite identification, and drug metabolism analysis. It helps researchers understand the absorption, distribution, metabolism, and excretion (ADME) of drugs.
What are the advantages of mass spectrometry in analysis?
Mass spectrometry offers high sensitivity, selectivity, and accuracy in compound analysis. It can detect and identify trace amounts of analytes, provide structural information, and is amenable to both qualitative and quantitative analysis. Additionally, it can be coupled with other techniques like chromatography for comprehensive analysis.
References
- Skoog, D. A., Holler, F. J., & Crouch, S. R. (2013). Principles of Instrumental Analysis. Cengage Learning. (Chapter 20: Mass Spectrometry)
- Watson, J. T., & Sparkman, O. D. (2007). Introduction to Mass Spectrometry: Instrumentation, Applications, and Strategies for Data Interpretation. John Wiley & Sons.
- Gross, J. H. (2011). Mass Spectrometry: A Textbook. Springer.
- Marshall, A. G., & Verdun, F. R. (Eds.). (2013). Fourier Transform Mass Spectrometry. Elsevier.
- McLafferty, F. W., & Tureček, F. (1993). Interpretation of Mass Spectra. University Science Books.
- Nikolaev, E. N., & Myshkin, Y. A. (2016). Mass Spectrometry in Proteomics. CRC Press.
- Stroobant, V., & De Hoffmann, E. (Eds.). (2011). Mass Spectrometry: Principles and Applications. John Wiley & Sons.