What is IR Spectroscopy?
- Infrared (IR) spectroscopy, also known as vibrational spectroscopy, is a common analytical technique utilized by inorganic and organic chemists to investigate and identify chemical substances or functional groups. Utilizing the vibrational transitions of molecules to provide information about their structures.
- Infrared spectroscopy utilizes an instrument known as an infrared spectrometer or spectrophotometer, which produces an infrared spectrum. This spectrum illustrates the interaction of infrared radiation with matter via absorption, emission, and reflection. It can be used to analyze solid, liquid, or gaseous samples and is useful for identifying and verifying known and unknown samples, as well as characterizing novel materials.
- Typically, an IR spectrum is depicted as a graph, with absorbance (or transmittance) of infrared radiation on the vertical axis and frequency, wavenumber, or wavelength on the horizontal axis. In IR spectra, wavenumber is typically expressed in reciprocal centimeters (cm1), while wavelength is typically expressed in micrometers (m). These entities are mutually interdependent. In laboratories, Fourier transform infrared (FTIR) spectrometers are commonly used for IR spectroscopy. Additionally, two-dimensional IR spectroscopy is feasible.
- On the basis of their relationship to the visible spectrum, the infrared portion of the electromagnetic spectrum is divided into three regions: near-infrared, mid-infrared, and far-infrared. The near-infrared region has higher energy (approximately 14,000–4,000 cm1 or 0.7–2.5 m wavelength) and can excite overtone or combination vibrational modes of molecules. Commonly, the mid-infrared region (approximately 4,000–400 cm1 or 2.5–25 m) is utilized to investigate fundamental vibrations and concomitant rotational-vibrational structure. Far-infrared wavelengths (approximately 400–10 cm1 or 25–1,000 m) have minimal energy and are suited for rotational spectroscopy and low-frequency vibrations. The region between 2 and 130 cm1 that borders the microwave region is referred to as the terahertz region, which can be used to investigate intermolecular vibrations.
- The peaks of an IR spectrum represent the specific infrared light frequencies absorbed by a molecule. Because these frequencies correspond to the vibration of the bonds within the molecule, they are selectively absorbed by molecules. In the infrared region of the electromagnetic spectrum is the energy necessary to excite these molecular vibrations. Polarity is required for a bond to interact with infrared radiation.
- Polarity is determined by the presence of distinct regions of partial positive and negative charges in a molecule, which permits the electric field component of infrared radiation to excite the vibrational energy of the molecule. Consequently, the molecule’s dipole moment endures a change proportional to the change in vibrational energy. The polarity of the bond determines the intensity of absorption. Non-polar symmetrical bonds, such as NN and O=O, do not absorb infrared radiation because they are incapable of interacting with an electric field.
Principle of IR Spectroscopy
Infrared (IR) spectroscopy operates on the basis of analyzing the interaction between infrared radiation and a molecule. In terms of wavelength, the infrared region most frequently employed for analyzing organic compounds lies between 2,500 and 16,000 nm, corresponding to a frequency range of 1.9 × 10^13 to 1.2 × 10^14 Hz.
In this region, photon energies are insufficient to excite electrons, but they can cause vibrational excitation of covalently bonded atoms and groups within a molecule. In addition to being able to freely rotate around singular bonds, molecules also undergo a variety of vibrational motions that are characteristic of the atoms that compose them.
As a result, nearly all organic compounds have the ability to absorb infrared radiation with energies corresponding to these harmonics. Using infrared spectrometers, which operate similarly to other types of spectrometers, chemists can obtain absorption spectra of compounds that provide unique insights into their molecular structure.
The infrared spectrum is the fundamental measurement in infrared spectroscopy. It is a graphical representation of the measured infrared intensity versus the wavelength (or frequency) of light. IR spectroscopy focuses on measuring the vibrations of atoms within a molecule, and it is possible to determine the functional groups present based on these vibrations.
As expressed by wavenumbers, stronger bonds and lighter elements tend to vibrate at higher stretching frequencies. IR spectroscopy is based on the theory that molecules selectively absorb light frequencies that correspond to their specific molecular structure. The assimilated energy from the incident light can increase the rotational speed or amplify the vibrations of a molecule.
Instrumentation of Infrared Spectroscopy (IR Spectroscopy )
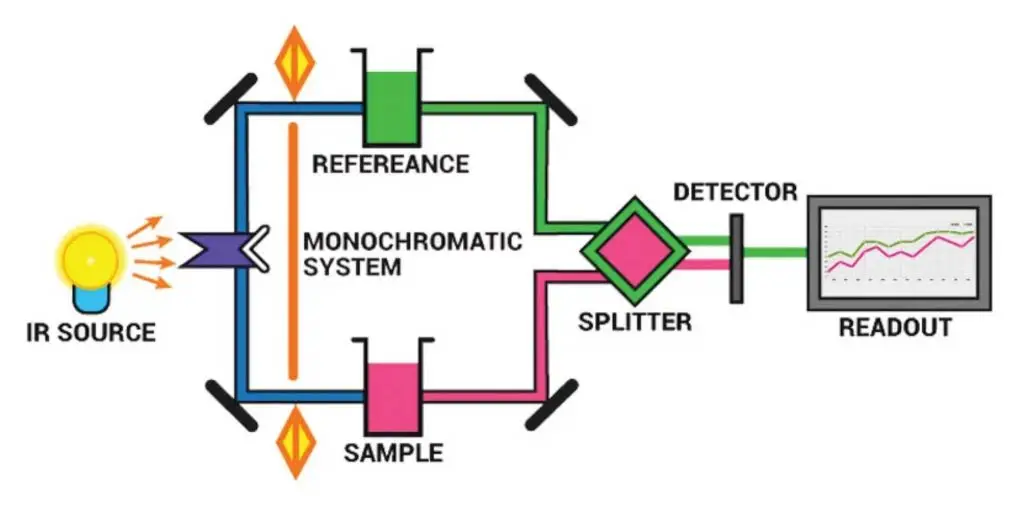
The principal components of IR the spectrometer are:
- Radiation source
- Sample cells and sampling of substances
- Monochromators
- Detectors
- Recorder
1. Radiation sources in IR Spectroscopy
Infrared (IR) sensors rely on a consistent and powerful source of radiant energy that generates IR radiation across the necessary wavelength range. In spectroscopy, numerous sources of IR radiation are routinely employed, including:
- Nernst Glower: The Nernst glower is a ceramic rod constructed of rare earth oxides, such as zirconium or yttrium oxides, that have been doped with trace quantities of rare earth elements. When an electric current passes through the glower, it generates a wide range of infrared radiation. Because of their strong emissivity, Nernst glowers are frequently utilized in the mid-IR spectrum.
- Incandescent Lamp: Incandescent lamps, which are often used as light bulbs in homes, can also be employed as a source of IR radiation. As a consequence of the heating filament, which generates a continuous spectrum in the IR region, these lamps produce IR radiation. Incandescent lights are affordable and widely accessible.
- Mercury Arc: In addition to UV and visible light, mercury arc lamps generate powerful lines of IR radiation. These lamps contain mercury vapor, which is generated by an electric discharge and emits distinctive spectral lines. Mercury arc lamps are often used in UV-Vis-IR spectroscopy.
- Tungsten Lamp: Tungsten lamps, often known as halogen lamps, are commonly employed as IR radiation sources. A tungsten filament is surrounded by a halogen gas, such as bromine or iodine, in these lamps. The halogen gas aids in the regeneration of the tungsten filament, resulting in a longer lamp life and a more steady IR output. Tungsten lights emit a continuous infrared spectrum.
- Globar Source: When heated electrically, a silicon carbide rod produces infrared light. It has a great thermal stability and can offer a continuous spectrum in the infrared area. In Fourier transform infrared (FTIR) spectroscopy, globar sources are often utilized.
- Nichrome Wire: Nichrome wire, a nickel-chromium alloy, may be heated to emit infrared radiation. It is frequently used in laboratories as a basic and low-cost IR source. The strength and spectrum of IR radiation emitted by nichrome wire are temperature dependent.
These IR radiation sources have distinct qualities, such as spectrum range, intensity, and stability, which make them useful for a variety of IR spectroscopy applications. The proper IR source is determined by the precise requirements of the experiment or analysis being performed.
2. Sample cells and sampling of substances
In IR spectroscopy, the characterization of samples can be performed on various forms, including solids, liquids, and gases, each requiring specific techniques for sampling and handling.
- Solid samples are prepared using a variety of techniques to ensure accurate analysis. One common method is the pressed pellet technique, where the solid sample is finely ground, mixed with a suitable matrix material, and compressed into a pellet for measurement. Another approach is the solid run in solution, where the solid is dissolved in a suitable solvent to form a solution, which is then analyzed using IR spectroscopy. Additionally, solid films or thin layers can be created by depositing the sample onto a suitable substrate, allowing for direct analysis.
- Liquid samples are typically analyzed using a liquid sample cell. These cells are often made of alkali halides, such as potassium bromide (KBr), which are transparent in the IR region. However, it is important to note that aqueous solvents cannot be used with alkali halide cells, as they will dissolve the cell material. Instead, organic solvents like chloroform are commonly employed for holding liquid samples in IR spectroscopy.
- Gas sampling in IR spectroscopy follows similar principles to liquid sampling. The gas sample is typically introduced into a suitable gas cell or gas chamber for analysis. The cell may be designed to contain a fixed volume of gas or allow for continuous flow of the sample. The gas cell should be made of a material that is transparent to IR radiation, such as quartz or other suitable materials.
By employing appropriate sampling techniques and utilizing specialized sample cells, IR spectroscopy enables the characterization and analysis of a wide range of substances, including solids, liquids, and gases. Each sample type requires specific considerations to ensure accurate and reliable measurements, allowing researchers to gain valuable insights into the molecular composition and properties of the analyzed samples.
3. Monochromators
- A monochromator is an optical device that isolates and selects a small range of wavelengths from a larger light spectrum. It is widely used in a variety of scientific and technical applications that demand precise control over light wavelength.
- Monochromators come in a variety of shapes and sizes, including prisms, gratings, and filters. Prisms are among the earliest and most basic types of monochromators. They are made of a translucent material having a triangular or wedge-shaped cross-section that may refract light. Prisms used in monochromators are frequently constructed of potassium bromide, sodium chloride, or caesium iodide. These materials have strong optical characteristics and can effectively separate light into its constituent wavelengths.
- Another form of monochromator that is often employed in scientific apparatus is diffraction gratings. They are made up of a surface with a regular pattern of closely spaced parallel lines or grooves. Light diffracts and forms a succession of spectral orders when it travels through a diffraction grating, resulting in the separation of distinct wavelengths. Because of their excellent optical properties, alkali halides such as lithium fluoride are extensively utilized materials for the fabrication of diffraction gratings.
- Filters, sometimes known as interference filters, are used as monochromators. These filters are designed to transmit light solely within a specific wavelength range while blocking or attenuating light outside of that range. Because of its capacity to selectively transmit particular wavelengths of light, lithium fluoride is a frequent substance used in the manufacture of filters.
- Depending on the application, each type of monochromator has its own set of pros and disadvantages. Prisms are generally simple and straightforward to operate, however their spectral range and resolution may be limited. Diffraction gratings have higher spectral resolution and a broader spectral range, but they need more accurate alignment and have more complicated production methods. Filters, on the other hand, give narrow bandwidth selection with good transmission efficiency, but their tunability may be restricted.
- Overall, monochromators are important in many scientific domains, including spectroscopy, microscopy, and analytical chemistry, where precise control and modification of light wavelengths is required for reliable measurements and analysis.
4. Detectors
Detectors play a crucial role in measuring the strength of infrared radiation. In the field of infrared spectroscopy, the detector is a device used to measure the intensity of infrared light that is transmitted through or absorbed by a sample.
Various types of detectors are employed in infrared spectroscopy, including:
- Thermal detectors (Bolometers): These detectors measure the change in temperature of a material caused by the absorption of radiation. They are commonly used to measure the absorption of infrared light by a sample and are sensitive to a wide range of wavelengths.
- Photovoltaic detectors (Photodiodes): These detectors convert absorbed radiation into an electrical current. They are widely used for measuring the absorption of infrared light by a sample and are sensitive to a broad range of wavelengths.
- Photoconductive detectors: These detectors measure the change in electrical conductivity of a material resulting from absorbed radiation. They are commonly used for measuring the absorption of infrared light by a sample and exhibit sensitivity across a wide range of wavelengths.
- Photoresistive detectors: These detectors measure the change in resistance of a material due to absorbed radiation. They are often utilized for measuring the absorption of infrared light by a sample and offer sensitivity across a wide range of wavelengths.
The selection of a specific detector type in infrared spectroscopy depends on the particular requirements of the measurement, including factors such as sensitivity, wavelength range, and dynamic range. In general, detectors that exhibit sensitivity to a broad range of wavelengths and have a high dynamic range are preferred for infrared spectroscopy applications.
5. Recorders
Infrared (IR) spectroscopy relies on recorders to catch and record various components of the IR spectrum. The detector of an infrared spectrometer monitors the intensity of transmitted or absorbed infrared light, while the recorder records the measurement data for subsequent analysis and interpretation.
In IR spectroscopy, various types of recorders are often used:
- Strip chart recorders: Strip chart recorders are devices that record data on a moving strip of paper. They are frequently used to capture a sample’s absorption spectra in real time. Strip chart recorders are simple and inexpensive ways to capture infrared spectra.
- Digital oscilloscopes: Digital oscilloscopes capture and show data on a computer screen in the form of a graph. When compared to strip chart recorders, they have a greater resolution and a broader dynamic range. Digital oscilloscopes are commonly used to capture and evaluate a sample’s absorption spectrum in real time.
- Data acquisition systems: Data acquisition systems are sophisticated machines that gather and store data from various sensors or detectors. They are often used to capture and evaluate a sample’s absorption spectra in real time. Data capture systems provide excellent resolution, a broad dynamic range, and increased data storage capacity.
The selection of an IR spectroscopy recorder is determined by the measurement’s unique requirements, such as the needed resolution, dynamic range, and data storage requirements. Real-time analysis, financial concerns, and the complexity of the recorded data may all impact the choice of the best recorder for a certain IR spectroscopy system.
Graph of the IR spectrum
Below is a small example of the typical Infrared Absorption Frequency.
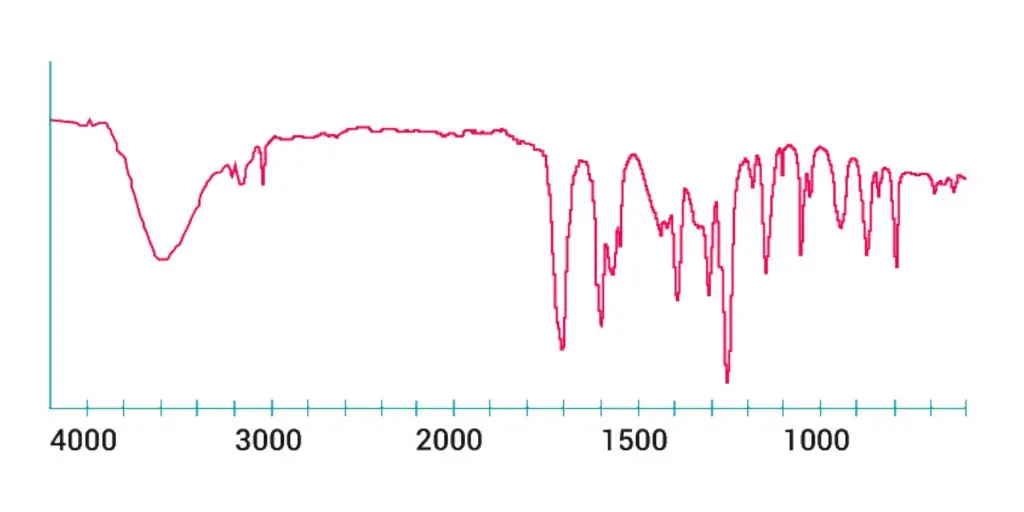
So, IR spectroscopy involves the gathering of absorption data and the analysis of that information as an IR spectrum.
Types of Infrared Spectrophotometry (IR Spectroscopy)
Infrared (IR) spectrophotometry methods of many sorts are used to quantify the absorption of IR light by molecules. Here are several examples:
- Fourier transform infrared (FTIR) spectrophotometry: This approach analyzes the IR spectrum in the frequency domain using the Fourier transform mathematical method. FTIR spectrophotometry has a large dynamic range and great sensitivity. To offer thorough sample analysis, it is usually used in concert with other analytical methods.
- Attenuated total reflectance (ATR) spectrophotometry: This technique is used to analyze solid and liquid materials. The sample is placed in contact with a crystal (such as diamond or zinc selenide) and the absorption of IR light as it travels through the crystal is measured. ATR spectrophotometry is a non-destructive technology that permits analysis without the need for special sample preparation, making it appropriate for delicate or precious samples.
- Transmission spectrophotometry: Transmission spectrophotometry is a method for analyzing liquid and gas samples. The absorption of IR light as it travels through a cell holding the sample is measured. Transmission spectrophotometry is a technique often used to investigate the structure and composition of gases and liquids.
- Reflectance spectrophotometry: This technique is used for examining solid materials. It detects the IR light reflection from the sample’s surface. Reflectance spectrophotometry is very effective for evaluating material surface qualities such as roughness, smoothness, and chemical composition.
Each form of IR spectrophotometry has its own set of uses and benefits. The suitable approach is chosen based on the type of the sample, the information sought, and the expected analytical findings.
Comparison between FTIR, ATR, Transmission spectrophotometry, Reflectance spectrophotometry
Fourier transform infrared (FTIR) spectrophotometry, attenuated total reflectance (ATR) spectrophotometry, transmission spectrophotometry, and reflectance spectrophotometry are all types of infrared (IR) spectrophotometry used to measure the absorption of IR light by molecules and identify and analyze the functional groups present in a variety of materials such as polymers, pharmaceuticals, and chemicals. Here’s a comparison of these methods:
- FTIR spectrophotometry: FTIR spectrophotometry is based on the Fourier transform, which is a mathematical approach that permits the spectrum to be studied in the frequency domain rather than the time domain. It has a large dynamic range and is very sensitive, allowing it to examine a wide range of materials, including liquids, gases, solids, and complicated combinations. However, it necessitates sample preparation for some types of samples, is restricted to specific functional groups, and may be influenced by water interference.
- ATR spectrophotometry: ATR spectrophotometry includes contacting the sample with a crystal, such as diamond or zinc selenide, and measuring the absorption of infrared light as it travels through the crystal. It is a non-destructive approach that does not need sample preparation, making it ideal for examining delicate or precious materials. It is, however, confined to solid and liquid samples and may not be suited for gas analysis.
- Transmission spectrophotometry: This technique includes measuring the absorption of infrared light as it travels through a sample-filled cell. It is frequently employed in the study of the structure and composition of gases and liquids. It does, however, need sample preparation and is confined to liquid and gas samples.
- Reflectance spectrophotometry: This technique includes detecting the reflection of infrared light from the sample’s surface. It is frequently used to investigate material surface qualities such as roughness, smoothness, and chemical composition. It is, however, confined to solid materials and may not be appropriate for testing liquids or gases.
Infrared spectroscopy Table
Infrared (IR) spectroscopy is a technique that measures the vibrational, rotational, and other low-frequency modes of molecules in the infrared region of the electromagnetic spectrum. It is a powerful tool for analyzing the molecular structure of a wide range of materials, including polymers, pharmaceuticals, and chemicals.
The IR spectrum of a molecule is a plot of the absorption of infrared light as a function of wavenumber (the reciprocal of the wavelength). Each molecule has a unique set of vibrational modes, and the IR spectrum can be used to identify the specific bonds and functional groups present in the molecule.
An IR spectrum is typically presented as a table or graph, with the wavenumber on the x-axis and the absorption intensity on the y-axis. The absorption intensity is usually expressed as a percentage of the incident light absorbed by the sample.
The IR spectrum is divided into several regions, each corresponding to a different range of wavenumbers:
- The far-infrared region (10-400 cm-1) corresponds to the lowest energy vibrations, such as lattice modes and rotational modes.
- The mid-infrared region (400-4000 cm-1) corresponds to the vibrations of bonds involving C-H, C-C, N-H, O-H, and other common functional groups.
- The near-infrared region (4000-25000 cm-1) corresponds to vibrations of bonds involving C-O, C=O, N-O, and other functional groups.
An IR spectroscopy table typically lists the wavenumber, intensity, and assignment of each absorption band in the IR spectrum. The assignment refers to the specific bond or functional group responsible for the absorption.
For example, an IR spectroscopy table for an ester might include the following entries:
Wavenumber (cm-1) | Intensity | Assignment |
---|---|---|
2950 | strong | C-H stretch |
1740 | medium | C=O stretch |
1460 | weak | C-O stretch |
This table indicates that the ester has strong absorption at 2950 cm-1 due to C-H stretching, medium absorption at 1740 cm-1 due to C=O stretching, and weak absorption at 1460 cm-1 due to C-O stretching.
Regions of the Infrared spectrum
The infrared (IR) spectrum is the portion of the electromagnetic spectrum that corresponds to wavelengths of light ranging from about 700 nanometers (nm) to 1 millimeter. It is divided into three main regions:
- Far-infrared (FIR) region: This region corresponds to wavelengths from about 700 nm to 30 micrometers (μm) and is also known as the “thermal infrared” region. It is characterized by low energy vibrations, such as lattice modes and rotational modes, and is often used to study the physical properties of solids and liquids.
- Mid-infrared (MIR) region: This region corresponds to wavelengths from about 3 μm to 30 μm and is characterized by the vibrations of chemical bonds involving C-H, C-C, N-H, O-H, and other common functional groups. It is often used to identify and analyze the functional groups present in a molecule.
- Near-infrared (NIR) region: This region corresponds to wavelengths from about 0.7 μm to 3 μm and is characterized by vibrations of bonds involving C-O, C=O, N-O, and other functional groups. It is often used to analyze the composition and structure of materials in the food, pharmaceutical, and chemical industries.
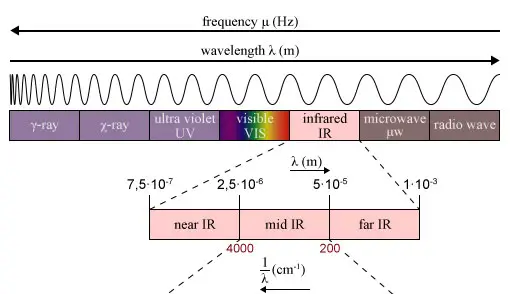
The IR spectrum is typically presented as a plot of the absorption of infrared light as a function of wavenumber (the reciprocal of the wavelength), with the wavenumber on the x-axis and the absorption intensity on the y-axis. Each molecule has a unique set of vibrational modes, and the IR spectrum can be used to identify the specific bonds and functional groups present in the molecule.
Types of Samples used in Infrared Spectroscopy
There are many different types of samples that can be analyzed using infrared (IR) spectroscopy. Some common types of samples include:
- Liquids: IR spectroscopy can be used to analyze the structure and chemical composition of liquids, such as solvents, acids, and bases.
- Gases: IR spectroscopy can be used to study the vibrational modes of gases, such as oxygen, nitrogen, and carbon dioxide.
- Solids: IR spectroscopy can be used to analyze the structure and chemical composition of solids, such as metals, minerals, and polymers.
- Biological samples: IR spectroscopy can be used to study the structure and function of biological molecules, such as proteins, nucleic acids, and lipids.
- Complex mixtures: IR spectroscopy can be used to identify and quantify the individual components of complex mixtures, such as fuels, food products, and pharmaceuticals.
There are several different techniques for preparing samples for IR spectroscopy, depending on the type of sample and the desired level of sensitivity. Some common techniques include:
- KBr pellet method: This method is commonly used for solid samples, and involves grinding the sample with potassium bromide (KBr) and pressing the mixture into a pellet. The pellet is then placed in the IR spectrometer and analyzed.
- ATR method: This method is used for solid and liquid samples and involves placing the sample in contact with a crystal, such as diamond or zinc selenide, and measuring the absorption of IR light as it passes through the crystal.
- Transmission method: This method is used for liquid and gas samples and involves measuring the absorption of IR light as it passes through a cell filled with the sample.
- Reflectance method: This method is used for solid samples and involves measuring the reflection of IR light from the surface of the sample.
What is fourier transform infrared spectroscopy?
- Fourier transform infrared (FTIR) spectroscopy is a type of infrared (IR) spectroscopy that is used to analyze the vibrational, rotational, and other low-frequency modes of molecules. It is a powerful tool for identifying and analyzing the functional groups present in a wide range of materials, including polymers, pharmaceuticals, and chemicals.
- FTIR spectroscopy works by measuring the absorption of IR light as a function of wavenumber (the reciprocal of the wavelength). The sample is placed in the path of an IR beam, and the absorption of the IR light is measured as a function of wavenumber. The resulting spectrum is a plot of the absorption intensity as a function of wavenumber, and can be used to identify the specific bonds and functional groups present in the sample.
- FTIR spectroscopy is based on the Fourier transform, which is a mathematical technique that allows the spectrum to be analyzed in the frequency domain rather than the time domain. This makes it possible to measure the spectrum over a wide range of wavenumbers in a single scan, and allows for more precise and accurate analysis of the sample.
- FTIR spectroscopy is widely used in a variety of fields, including chemistry, biology, material science, and environmental science, and is often used in conjunction with other analytical techniques, such as Raman spectroscopy, to provide a more complete understanding of the sample.
What is near infrared spectroscopy?
Near infrared (NIR) spectroscopy is a type of infrared (IR) spectroscopy that is used to analyze the vibrational modes of molecules in the near-infrared region of the electromagnetic spectrum (wavelengths ranging from about 0.7 micrometers to 3 micrometers). It is a powerful tool for identifying and analyzing the functional groups present in a wide range of materials, including polymers, pharmaceuticals, and chemicals.
NIR spectroscopy works by measuring the absorption of NIR light as a function of wavenumber (the reciprocal of the wavelength). The sample is placed in the path of an NIR beam, and the absorption of the NIR light is measured as a function of wavenumber. The resulting spectrum is a plot of the absorption intensity as a function of wavenumber, and can be used to identify the specific bonds and functional groups present in the sample.
NIR spectra are typically characterized by the vibrations of bonds involving C-O, C=O, N-O, and other functional groups. The NIR region is particularly useful for analyzing the structure and composition of materials in the food, pharmaceutical, and chemical industries, as many functional groups absorb NIR light strongly.
NIR spectroscopy is widely used in a variety of fields, and is often used in conjunction with other analytical techniques, such as Raman spectroscopy, to provide a more complete understanding of the sample. It is a non-destructive technique, meaning that the sample is not altered during analysis, making it suitable for analyzing fragile or valuable samples.
Why do Molecules Only Absorb IR Frequency Resonant to Their Internal Vibrational Frequencies?
Molecules only absorb infrared (IR) frequencies that are resonant with their internal vibrational frequencies because the energy of the absorbed IR light must be equal to the energy difference between two vibrational energy levels of the molecule. This is known as the selection rule for vibrational transitions.
When a molecule absorbs an IR photon, it absorbs energy that is equal to the energy difference between two vibrational energy levels. The vibrational energy levels of a molecule are determined by the bond lengths, bond angles, and other structural parameters of the molecule. Each vibrational mode corresponds to a specific vibrational energy level, and the energy difference between two vibrational energy levels is equal to the energy of the absorbed IR photon.
Therefore, a molecule will only absorb an IR photon if the energy of the IR photon is equal to the energy difference between two vibrational energy levels of the molecule. This means that the IR frequency must be resonant with the internal vibrational frequencies of the molecule.
For example, if the vibrational energy levels of a molecule are separated by an energy of 1000 cm-1, then the molecule will only absorb IR photons with a frequency of 1000 cm-1. This frequency will be absorbed by the molecule, while other frequencies will not be absorbed.
This is why molecules only absorb IR frequencies that are resonant with their internal vibrational frequencies. It is also why the IR spectrum of a molecule is a fingerprint of its vibrational modes and can be used to identify the specific bonds and functional groups present in the molecule.
Types of vibrations
The type of vibrations or vibrational modes refers to the positional variations of the atoms constituting the link. Stretching and bending are the two primary types of vibrational modes.
1. Stretching
This entails modifications to the bond length between atoms. Either the atoms can move closer together, so lengthening the connection, or farther apart, thereby weakening it.
Stretching can be categorised as;
- Symmetric stretching: When two atoms simultaneously move toward or away from a centre atom, this is symmetric stretching.
- Asymmetric stretching: Asymmetric stretching occurs when two atoms attached to a core atom move in opposite directions.
2. Bending
This sort of vibration refers to changes in the ‘angle’ between two bonds and is subdivided as follows:
- Rocking: when two atoms move in opposite directions on the same plane.
- Scissoring: When two atoms move towards or away from one another in the same plane, they are scissoring.
- Twisting: Twisting occurs when two atoms travel out of the plane in opposite directions.
- Wagging: an out-of-plane vibration in which the atoms simultaneously move away from and toward one another in a v-shape.
What are the units of spectroscopy?
Spectroscopy is the study of the interaction of electromagnetic radiation with matter. In spectroscopy, the intensity or intensity ratio of the radiation is typically measured as a function of wavelength, frequency, or energy. The units used to express these measurements depend on the specific type of spectroscopy being used and the nature of the radiation being measured.
In many cases, spectroscopy measurements are expressed in terms of the energy or frequency of the radiation. The energy of electromagnetic radiation is typically expressed in units of electron volts (eV), while the frequency is typically expressed in units of Hertz (Hz).
In other cases, spectroscopy measurements are expressed in terms of the wavelength of the radiation. The wavelength is the distance between two adjacent peaks or troughs of a wave, and it is typically expressed in units of meters (m), nanometers (nm), or angstroms (Å).
In infrared (IR) spectroscopy, the absorption or transmittance of infrared light is often expressed in units of absorbance (A) or transmittance (%T). Absorbance is defined as the logarithm of the ratio of the intensity of the incident light to the intensity of the absorbed light, and it is typically expressed on a scale from 0 to 10. Transmittance is defined as the ratio of the intensity of the transmitted light to the intensity of the incident light, and it is typically expressed as a percentage.
In ultraviolet-visible (UV-Vis) spectroscopy, the absorption or transmittance of ultraviolet or visible light is often expressed in units of absorbance or transmittance, as in IR spectroscopy. In addition, the absorption or transmittance may also be expressed in terms of the molar absorbance coefficient (ε) or the molar extinction coefficient (ε), which are related to the concentration and molar mass of the sample.
In nuclear magnetic resonance (NMR) spectroscopy, the intensity of the magnetic resonance signal is typically expressed in units of parts per million (ppm). The chemical shift of the signal is often expressed in units of ppm relative to a reference compound.
In mass spectrometry, the intensity of the ionized species is typically expressed in units of counts per second (cps) or relative abundance. The mass-to-charge ratio (m/z) of the ions is often expressed in units of atomic mass units (amu).
Infrared vs Raman spectroscopy
Infrared (IR) spectroscopy and Raman spectroscopy are two techniques that are used to identify and analyze the molecular structure of a substance. Both techniques involve the interaction of light with a sample, but they differ in the way that the light is used to probe the sample.
Infrared spectroscopy involves the absorption of infrared light by a sample. Infrared light has a longer wavelength than visible light, and it is absorbed by certain vibrational modes of the bonds between atoms in a molecule. By measuring the absorption of infrared light at different wavelengths, it is possible to determine the vibrational modes of the bonds in the molecule, which can be used to identify the molecular structure of the sample.
Raman spectroscopy, on the other hand, involves the scattering of light by a sample. When light is scattered by a sample, some of the scattered light is shifted to a higher or lower energy level. This energy shift is called the Raman shift, and it is caused by the vibrational and rotational modes of the bonds in the molecule. By measuring the Raman shift of the scattered light at different wavelengths, it is possible to determine the vibrational and rotational modes of the bonds in the molecule, which can also be used to identify the molecular structure of the sample.
Both IR and Raman spectroscopy are widely used in a variety of fields, including chemistry, materials science, and biology. They are powerful tools for identifying and analyzing the molecular structure of a wide range of materials, including organic and inorganic compounds, polymers, and biomolecules.
What is necessary condition for IR spectroscopy?
There are several conditions that are necessary for infrared (IR) spectroscopy to be successful. These include:
- The presence of bonds that absorb infrared light: IR spectroscopy relies on the absorption of infrared light by certain vibrational modes of the bonds between atoms in a molecule. In order for IR spectroscopy to be useful, the sample must contain bonds that absorb infrared light.
- A transparent or translucent sample: IR spectroscopy involves the measurement of the absorption of infrared light by a sample. In order for the measurement to be accurate, the sample must be transparent or translucent, so that the infrared light can pass through it.
- A stable sample: IR spectroscopy involves the measurement of the absorption of infrared light by a sample over a certain period of time. In order for the measurement to be accurate, the sample must be stable and not undergo any changes during the measurement.
- A representative sample: In order for IR spectroscopy to be useful, the sample must be representative of the material being analyzed. This means that the sample must be representative of the average molecular structure of the material, and it must not be contaminated with any other materials.
- A suitable solvent: In many cases, it is necessary to dissolve the sample in a solvent in order to prepare it for IR spectroscopy. The choice of solvent can affect the absorption spectrum of the sample, and it is important to choose a solvent that does not interfere with the measurement of the absorption of the sample.
- Suitable instrumentation: IR spectroscopy requires specialized instrumentation, such as an infrared spectrometer, to measure the absorption of infrared light by the sample. The sensitivity and resolution of the instrumentation can affect the accuracy and precision of the measurement.
Applications of Infrared (IR) Spectroscopy
Infrared (IR) spectroscopy is a useful analytical method that has applications in a wide range of scientific domains. Here are some prominent IR spectroscopy applications:
- Protein characterization: Infrared spectroscopy is commonly used to examine protein structure and conformational changes. It reveals information about protein secondary structures such alpha helices, beta sheets, and random coils. The method aids researchers in their understanding of protein folding, stability, and interactions.
- Nanoscale semiconductor analysis: IR spectroscopy is essential for analyzing nanoscale semiconductor materials. It describes their composition, surface chemistry, and structural features. This is critical for enhancing semiconductor device performance and understanding their behavior at the nanoscale level.
- Space exploration: Space exploration uses IR spectroscopy to detect and evaluate the composition of planetary surfaces and atmospheres. Scientists can learn about the geological and atmospheric conditions of celestial bodies by identifying the distinctive spectral fingerprints of diverse substances.
- Analysis of gaseous, liquid, or solid samples: Because IR spectroscopy is so adaptable, it may be used to evaluate a broad variety of materials. It is used to identify and characterize chemicals in gases, liquids, and solids. Organic and inorganic substances, polymers, medicines, and environmental materials are all analyzed.
- Quantitative analysis: Infrared spectroscopy may be used to quantify chemicals in a sample. The concentration of a certain substance can be estimated by measuring the intensity of various absorption bands.
- Functional group and molecular structure determination: IR spectroscopy gives vital information on the functional groups present in a molecule. Different functional groups display distinct absorption bands in the IR spectra, allowing them to be distinguished. Furthermore, the pattern of absorption bands might reveal information about a compound’s molecular structure and bonding.
- Study of molecular interactions: Infrared spectroscopy is used to analyze intermolecular interactions such as hydrogen bonding and van der Waals forces. Scientists can learn about the nature and intensity of these interactions by observing changes in absorption bands or the emergence of new bands.
These are only a handful of the many uses of infrared spectroscopy. Because it is non-destructive and adaptable, it is useful in chemical analysis, material science, biochemistry, environmental research, and many other domains.
Infrared Spectrophotometry Advantages
- Non-destructive: IR spectrophotometry is a non-destructive technique, meaning that the sample is not altered during analysis. This makes it suitable for analyzing fragile or valuable samples.
- High sensitivity: IR spectrophotometry is highly sensitive and can detect trace amounts of certain functional groups.
- Wide range of applications: IR spectrophotometry has a wide range of applications and can be used to analyze a variety of materials, including liquids, gases, solids, and complex mixtures.
- Versatility: IR spectrophotometry can be used in conjunction with other analytical techniques, such as Raman spectroscopy, to provide a more complete understanding of the sample.
- You can receive both qualitative (structure, presence of functional groups) and quantitative (number of chemicals in the sample) information.
- Different IR regions can be optimised for particular applications, such as the far IR region for the study of inorganic chemicals, the mid-IR region for organic species, and the near IR region for routine quantitative analysis.
- Data interpretation of the regularly employed mid-IF area is straightforward; peak intensities, peak positions, peak widths, and peak shapes can be read readily, and data may be extracted rapidly.
- The FTIR spectrometer can acquire data quickly and with a higher signal-to-noise ratio.
Infrared Spectrophotometry Disadvantages
- Requires sample preparation: Some types of IR spectrophotometry, such as transmission spectrophotometry, require the sample to be prepared in a specific way (e.g., placed in a cell). This can be time-consuming and may require specialized equipment.
- Limited to certain functional groups: IR spectrophotometry is limited to the vibrational modes of certain functional groups, and may not be suitable for analyzing all types of molecules.
- Interference from water: Water absorbs IR light strongly and can interfere with the analysis of certain samples. This can be mitigated by using a technique such as attenuated total reflectance (ATR) spectrophotometry, which does not require the sample to be prepared in a specific way.
- Limited to certain sample types: Some types of IR spectrophotometry, such as reflectance spectrophotometry, are limited to solid samples and may not be suitable for analyzing liquids or gases.
- IR spectrophotometry provides no information regarding molecular mass or the relative positions of functional groups.
- A single IR absorption spectra cannot determine if an unidentified material is pure or a combination of components.
- Materials that absorb IR, such as plastic and glass, cannot be utilised in an IR spectrophotometer.
- The dispersive spectrophotometer has low sensitivity and is relatively sluggish.
FAQ
What is the basic principle of infrared spectroscopy?
The basic principle of infrared (IR) spectroscopy is the absorption of infrared light by a sample. Infrared light is a type of electromagnetic radiation with a longer wavelength than visible light. It is absorbed by certain vibrational modes of the bonds between atoms in a molecule.
When infrared light is absorbed by a sample, it causes the bonds in the molecule to vibrate at the same frequency as the absorbed light. The absorbed light is then absorbed as energy by the molecule, and the molecule transitions to an excited state. The molecule will eventually return to its ground state, releasing the absorbed energy as heat or light.
By measuring the absorption of infrared light at different wavelengths, it is possible to determine the vibrational modes of the bonds in the molecule. Each bond in a molecule has a characteristic vibrational frequency, and the absorption of infrared light at these frequencies can be used to identify the molecular structure of the sample.
Infrared spectroscopy is a powerful tool for identifying and analyzing the molecular structure of a wide range of materials, including organic and inorganic compounds, polymers, and biomolecules. It is widely used in a variety of fields, including chemistry, materials science, and biology.
What is infrared spectroscopy used for?
Infrared (IR) spectroscopy is a technique used to identify and analyze the molecular structure of a substance. It involves the absorption of infrared light by a sample, and the measurement of the absorption at different wavelengths. By analyzing the absorption spectrum, it is possible to determine the vibrational modes of the bonds in the molecule, which can be used to identify the molecular structure of the sample.
Infrared spectroscopy is used in a variety of fields, including chemistry, materials science, and biology. It is particularly useful for identifying and analyzing the molecular structure of organic and inorganic compounds, polymers, and biomolecules. It is also used in quality control and research applications, as well as for the analysis of environmental samples and forensic evidence.
What is range of IR spectroscopy?
The range of infrared (IR) spectroscopy is typically defined by the range of wavelengths that can be measured using the instrumentation available. The range of IR spectroscopy is typically between 400 and 4000 cm^-1 (4000 and 400 nm), although some instruments are capable of measuring even lower or higher wavelengths.
The specific range of IR spectroscopy that is used depends on the nature of the sample being analyzed and the vibrational modes of the bonds in the molecule. Different bonds absorb infrared light at different wavelengths, and the absorption spectrum of a molecule can be used to identify the molecular structure of the sample.
For example, the absorption of infrared light by stretching and bending vibrations of bonds in organic molecules is typically in the range of 4000-400 cm^-1 (400-4000 nm). The absorption of infrared light by vibrational modes of inorganic compounds and polymers is typically in the range of 1000-100 cm^-1 (10000-100000 nm). The absorption of infrared light by rotational modes of molecules is typically in the range of 100-10 cm^-1 (100000-1000000 nm).
What instrument is used in IR spectroscopy?
Infrared (IR) spectroscopy is typically performed using a specialized instrument called an infrared spectrometer. An infrared spectrometer consists of three main components: a light source, a sample holder, and a detector.
The light source in an infrared spectrometer is typically a tungsten halogen lamp or a deuterium lamp, which produces a broad spectrum of infrared light. The sample holder is a device that holds the sample and allows infrared light to pass through it. The detector is a device that measures the intensity of the infrared light that is transmitted or absorbed by the sample.
There are several different types of infrared spectrometers, including dispersive spectrometers and Fourier transform spectrometers. Dispersive spectrometers use a prism or grating to separate the different wavelengths of light, while Fourier transform spectrometers use interferometry to measure the spectrum of the sample.
Infrared spectrometers are widely used in a variety of fields, including chemistry, materials science, and biology, to identify and analyze the molecular structure of a wide range of materials, including organic and inorganic compounds, polymers, and biomolecules.
Which type of solvent is used in IR?
Infrared (IR) spectroscopy is a technique that is used to identify and analyze the molecular structure of a substance. In order to prepare a sample for IR spectroscopy, it is often necessary to dissolve the sample in a solvent. The choice of solvent depends on the nature of the sample and the vibrational modes of the bonds in the molecule that are being studied.
In general, it is best to use a solvent that does not absorb infrared light at the wavelengths of interest. This is because the absorption of the solvent can interfere with the measurement of the absorption of the sample.
Common solvents that are used for IR spectroscopy include liquid hydrocarbons (such as hexane, cyclohexane, and toluene), alcohols (such as methanol, ethanol, and isopropanol), and water. In some cases, it may be necessary to use a more specialized solvent, such as dimethylformamide (DMF) or dimethylsulfoxide (DMSO), depending on the nature of the sample.
It is important to note that the choice of solvent can affect the absorption spectrum of the sample. In some cases, the presence of a solvent can alter the vibrational modes of the bonds in the molecule, which can affect the interpretation of the IR spectrum. Therefore, it is important to carefully consider the choice of solvent when preparing a sample for IR spectroscopy.
What is UV and IR range?
The electromagnetic spectrum is the range of all types of electromagnetic radiation, including radio waves, microwaves, infrared (IR) radiation, visible light, ultraviolet (UV) radiation, X-rays, and gamma rays. Each type of electromagnetic radiation has a specific range of wavelengths and frequencies, and is characterized by its unique properties and uses.
The ultraviolet (UV) range is the portion of the electromagnetic spectrum with wavelengths shorter than visible light. UV radiation has wavelengths between 100 and 400 nm, and it is characterized by its ability to cause chemical reactions and damage to living tissues. The UV range is further divided into three regions: UVA (400-315 nm), UVB (315-280 nm), and UVC (280-100 nm).
The infrared (IR) range is the portion of the electromagnetic spectrum with wavelengths longer than visible light. IR radiation has wavelengths between 750 nm and 1 mm, and it is characterized by its ability to be absorbed by certain vibrational modes of the bonds between atoms in a molecule. The IR range is further divided into three regions: near-infrared (NIR, 750-1400 nm), mid-infrared (MIR, 1400-3000 nm), and far-infrared (FIR, 3000-1 mm).
UV and IR radiation have a variety of practical applications, including UV disinfection, UV curing, and IR spectroscopy. They are also used in a variety of scientific and medical instruments, such as UV spectrophotometers and IR cameras.
Can we use water in IR spectroscopy?
Yes, water can be used as a solvent in infrared (IR) spectroscopy. Water is a common solvent for IR spectroscopy because it is widely available, inexpensive, and has a low absorption in the IR region.
However, it is important to note that water can absorb infrared light at certain wavelengths, particularly in the mid-infrared (MIR) region (1400-3000 cm^-1 or 4000-1000 nm). This means that the absorption spectrum of the sample may be affected by the presence of water, particularly if the sample absorbs at the same wavelengths as water.
To minimize the impact of water absorption on the IR spectrum of the sample, it is often necessary to use a deuterated solvent (such as deuterated methanol or deuterated water) or to dry the sample prior to analysis. Deuterated solvents have a lower absorption in the IR region than their non-deuterated counterparts, and they are often used to reduce interference from the solvent in IR spectra. Drying the sample can also help to remove any water that may be present, which can reduce interference from water absorption.
It is also important to carefully consider the nature of the sample when selecting a solvent for IR spectroscopy. In some cases, it may be necessary to use a more specialized solvent, such as dimethylformamide (DMF) or dimethylsulfoxide (DMSO), depending on the nature of the sample. The choice of solvent can affect the absorption spectrum of the sample, and it is important to choose a solvent that does not interfere with the measurement of the absorption of the sample.
How sensitive is IR spectroscopy?
The sensitivity of infrared (IR) spectroscopy depends on a variety of factors, including the nature of the sample, the instrumentation used, and the experimental conditions. In general, IR spectroscopy is sensitive enough to detect small changes in the molecular structure of a sample, and it is often used to identify and quantify trace amounts of compounds in complex mixtures.
One of the main factors that determines the sensitivity of IR spectroscopy is the strength of the vibrational absorption bands in the spectrum of the sample. Strong absorption bands are more likely to be detected than weak absorption bands, and they are generally easier to quantify.
The sensitivity of IR spectroscopy can also be affected by the choice of solvent, the sample preparation techniques, and the instrumentation used. For example, using a deuterated solvent or drying the sample can reduce interference from the solvent, which can improve the sensitivity of the measurement. Using a high-resolution spectrometer or a Fourier transform spectrometer (FTIR) can also improve the sensitivity of the measurement, as these instruments have a higher resolution and can detect smaller changes in the absorption spectrum.
In general, IR spectroscopy is a sensitive and powerful tool for identifying and analyzing the molecular structure of a wide range of materials, including organic and inorganic compounds, polymers, and biomolecules. It is widely used in a variety of fields, including chemistry, materials science, and biology, for a wide range of applications.
Which lamp is used in IR spectroscopy?
Infrared (IR) spectroscopy is typically performed using a specialized instrument called an infrared spectrometer. An infrared spectrometer consists of three main components: a light source, a sample holder, and a detector.
The light source in an infrared spectrometer is typically a tungsten halogen lamp or a deuterium lamp, which produces a broad spectrum of infrared light. The tungsten halogen lamp is a type of incandescent lamp that uses a tungsten filament and a halogen gas to produce light. It has a broad emission spectrum and is capable of producing a wide range of wavelengths in the infrared region.
The deuterium lamp is a specialized type of lamp that uses deuterium, a stable isotope of hydrogen, as the light-emitting element. It produces a spectrum that is rich in ultraviolet and infrared radiation, and it is often used in spectroscopy applications that require a broad spectrum of light.
Both tungsten halogen lamps and deuterium lamps are widely used in IR spectrometry because they produce a broad spectrum of infrared light that is suitable for a wide range of applications. The specific type of lamp used depends on the specific requirements of the measurement, including the wavelength range of interest and the sensitivity of the instrument.
References
- Kamariotis, A.; Boyarkin, O. V.; Mercier, S. R.; Beck, R. D.; Bush, M. F.; Williams, E. R.; Rizzo, T. R. J. Am. Chem. Soc. 2006, 128, 905
- Peter J. Larkin. “Infrared and Raman Spectroscopy. Principles and Spectral Interpretation” Chapters 1 to 6. Radarweg 29, PO Box 211, 1000 AE Amsterdam, The Netherlands. Elsevier 2011
- Marwa El-Azazy. Introductory Chapter: Infrared Spectroscopy – A Synopsis of the Fundamentals and Applications, Infrared Spectroscopy – Principles, Advances, and Applications. 2018. IntechOpen, DOI: 10.5772/intechopen.82210
- Donald L. Pavia, Gary M. Lampman and George S. Kriz. “Introduction to Spectroscopy. A Guide for Students of Organic Chemistry” Chapter 2. Thompson Learning. United States of America 2001.