What is Hemoglobin?
- Hemoglobin, also known as haemoglobin or Hb, is a type of protein found in red blood cells (RBCs) that plays a crucial role in transporting oxygen (O2) throughout the body. It is a tetrameric protein, meaning it is made up of four subunits, and each subunit contains a heme prosthetic group. Hemoglobin is considered a respiratory pigment because it carries oxygen in the form of oxyhemoglobin from the lungs to various tissues and organs in the body.
- In addition to oxygen transport, hemoglobin also assists in the transportation of hydrogen ions and carbon dioxide (CO2) back to the lungs. It acts as carbaminohemoglobin to carry a portion of the body’s respiratory carbon dioxide. Moreover, hemoglobin binds to nitric oxide, a significant regulatory molecule, releasing it simultaneously with oxygen.
- The level of hemoglobin in the blood can be measured in grams per deciliter (g/dL). The normal range for hemoglobin levels is typically between 12 and 20 g/dL. In males, the levels are usually higher than in females, with a normal range of 13.5 to 17 g/dL for males and 12 to 15.5 g/dL for females.
- Hemoglobin is an essential component of red blood cells and accounts for a significant portion of their weight. In mammals, it makes up approximately 96% of a red blood cell’s weight, excluding water, and around 35% of the total weight, including water. Its oxygen-binding capacity is about 1.34 mL of O2 per gram of hemoglobin, significantly increasing the blood’s oxygen-carrying capacity compared to dissolved oxygen in the plasma alone. A single hemoglobin molecule can bind and transport up to four oxygen molecules.
- While hemoglobin is primarily found in red blood cells, it is also present in other cells and tissues throughout the body. These include A9 dopaminergic neurons, macrophages, alveolar cells, retinal pigment epithelium, hepatocytes, and various other cell types. In these tissues, hemoglobin functions as an antioxidant, absorbing excess oxygen and regulating iron metabolism.
- Hemoglobin is not exclusive to vertebrates; it is also found in many invertebrates, fungi, and plants. In these organisms, hemoglobin may serve different functions, such as carrying oxygen or regulating other molecules and ions like carbon dioxide, nitric oxide, hydrogen sulfide, and sulfide.
- In summary, hemoglobin is a vital protein involved in oxygen transport throughout the body. Its structure, with heme prosthetic groups and globin subunits, allows it to bind and release oxygen efficiently. Additionally, hemoglobin aids in the transport of carbon dioxide and plays a role in regulating various molecules in different tissues.
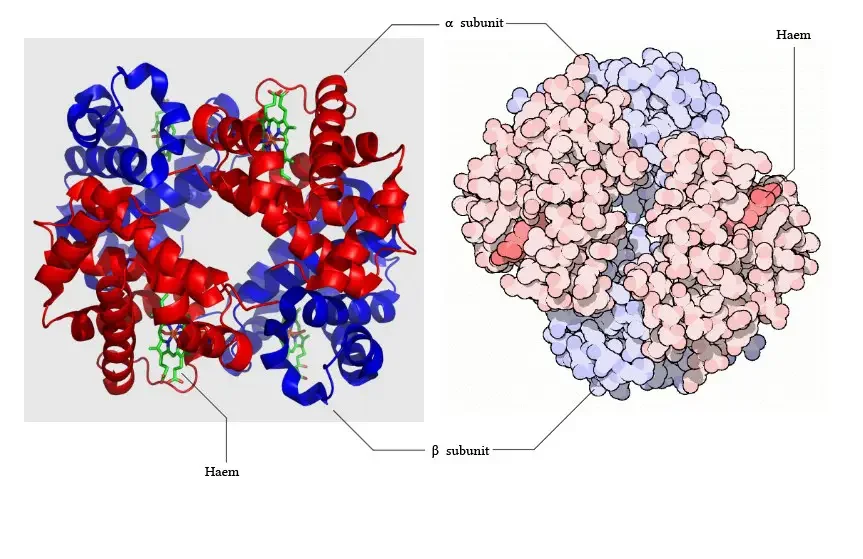
What is Globin Chain?
The globin chain refers to the specific combination of protein subunits that make up different types of hemoglobin. Hemoglobin is composed of four globin chains, which can vary depending on the specific type of hemoglobin.
The different types of hemoglobin and their corresponding globin chains are as follows:
- Hemoglobin A: Hemoglobin A is the most common type of hemoglobin found in adults. It consists of two alpha globin chains and two beta globin chains. The specific combination is referred to as Alpha2, beta2.
- Hemoglobin A2: Hemoglobin A2 is a minor component of adult hemoglobin. It contains two alpha globin chains and two delta globin chains. The combination is known as Alpha2, delta2.
- Hemoglobin F: Hemoglobin F, also called fetal hemoglobin, is predominant in newborns but decreases as an individual grows older. It is composed of two alpha globin chains and two gamma globin chains. The globin chain combination is referred to as Alpha2, gamma2.
- Hemoglobin H: Hemoglobin H is an abnormal type of hemoglobin that forms when there are deficiencies or mutations in the alpha globin genes. It consists of four beta globin chains and is denoted as Beta4.
Each type of hemoglobin with its specific globin chain composition has different properties and functions. Hemoglobin A is responsible for oxygen transport in adults, Hemoglobin A2 helps maintain the stability and integrity of red blood cells, Hemoglobin F aids in oxygen transfer in the fetus, and Hemoglobin H is associated with certain genetic disorders affecting alpha globin production.
Location of Hemoglobin
- Hemoglobin is primarily located within the cells of the bone marrow, where it is synthesized. These cells then develop into red blood cells or erythrocytes, which contain hemoglobin. Hemoglobin is a crucial component of red blood cells and is responsible for their oxygen-carrying capacity.
- When red blood cells reach the end of their lifespan and die, hemoglobin is broken down. During this process, iron is removed from hemoglobin and transported back to the bone marrow through proteins called transferrins. The iron is then utilized in the production of new red blood cells.
- Within red blood cells, hemoglobin occupies approximately one-third of the cell’s volume. In fact, hemoglobin accounts for around 90 to 95% of the dry weight of red blood cells. It is an essential component for their functioning.
- However, hemoglobin can also be found outside of red blood cells and their precursor lines. For example, certain cells such as macrophages, specific neurons in the substantia nigra, alveolar cells, and mesangial cells in the kidney show the presence of hemoglobin. In these cells or tissues, the role of hemoglobin may vary in comparison to its role in normal red blood cells.
- Additionally, after the breakdown of hemoglobin, a byproduct called bilirubin is formed. Bilirubin is a chemical that is excreted into the bile and contributes to the characteristic yellow-brown color of feces.
- Overall, while the primary location of hemoglobin is within red blood cells in the bone marrow, it can also be observed in other cells and tissues with varying functions and implications.
What is the normal haemoglobin level?
The normal hemoglobin level in individuals can vary depending on factors such as age and sex. Here are the typical hemoglobin ranges per deciliter (dl) of blood:
- Newborns: The normal hemoglobin level for newborns is usually between 17 to 22 g/dl. This higher range is necessary to support oxygen transport in the early stages of life.
- Children: For children, the normal hemoglobin level is generally in the range of 11 to 13 g/dl. As children grow, their hemoglobin levels gradually increase but remain lower than those of adults.
- Adult Males: Adult males typically have a normal hemoglobin level ranging from 14 to 18 g/dl. This higher range is attributed to factors such as increased muscle mass and higher oxygen demands.
- Adult Females: Adult females tend to have slightly lower hemoglobin levels compared to males, with a normal range of 12 to 16 g/dl. The hormonal and physiological differences between males and females contribute to this variation.
- Elderly Males: As males age, their hemoglobin levels may decrease slightly. The normal range for older males is around 12.4 to 14.9 g/dl. This decline is often considered a normal part of the aging process.
- Elderly Females: Similarly, elderly females also experience a gradual decline in hemoglobin levels. The normal range for older females is approximately 11.7 to 13.8 g/dl.
It’s important to note that these ranges are general guidelines and may vary slightly between different laboratories and healthcare providers. Additionally, individual variations and specific health conditions can affect hemoglobin levels. If there are concerns about an individual’s hemoglobin level, it is best to consult a healthcare professional for a comprehensive evaluation and interpretation of the results.
Haemoglobin Synthesis/Formation
Haemoglobin, or Hb, is formed through a series of complex steps involving the synthesis of the heme component and the globin protein chains.
The process of haemoglobin formation begins in the proerythroblasts, which are precursor cells found in the bone marrow. These cells eventually progress to the reticulocyte phase of red blood cells (RBCs). Even as reticulocytes leave the bone marrow and enter the bloodstream, they continue to synthesize small amounts of haemoglobin until they mature into erythrocytes.
The formation of haemoglobin involves several steps:
- SuccinylCoA, a product of the Krebs metabolic cycle, binds with glycine to form a pyrrole molecule.
- Four pyrroles come together to form protoporphyrin IX.
- Protoporphyrin IX combines with iron to create a heme molecule.
- Each heme molecule then binds with a long polypeptide chain to form a subunit of haemoglobin known as a haemoglobin chain.
- Four haemoglobin chains loosely bind together to form the complete haemoglobin molecule.
The composition of amino acids in the polypeptide chains determines the specific subunit haemoglobin chains. There are different types of chains, including alpha, beta, gamma, and delta chains, which give rise to variations in haemoglobin structure.
The synthesis of haemoglobin involves a collaboration between different cellular compartments. The heme component is synthesized in the mitochondria and cytosol of immature red blood cells, while the globin protein parts are synthesized by ribosomes in the cytosol. This production of haemoglobin continues as the cells progress from the proerythroblast stage to reticulocytes in the bone marrow. In mammals, the loss of the nucleus occurs in mature red blood cells, but residual ribosomal RNA allows for further haemoglobin synthesis until the reticulocyte loses its RNA upon entering the bloodstream.
The intricate process of haemoglobin formation ensures the production of functional red blood cells capable of transporting oxygen throughout the body.
Structure of Hemoglobin
The structure of hemoglobin is composed of several key components:
- Subunits: Hemoglobin consists of four subunits, each containing one polypeptide chain and one heme group. Each subunit has a molecular weight of approximately 16,000 daltons, resulting in a total molecular weight of the hemoglobin tetramer of about 64,000 daltons.
- Protein and Heme Complex: Hemoglobin is made up of a protein component called globin and the iron complex of a porphyrin derivative known as haemochromogen (Fe (II) complex). The combination of globin and haemochromogen forms the hemoglobin molecule.
- Heme Groups: There are four heme groups in hemoglobin, with each heme group attached to one globin chain. These heme groups contain an iron atom at the center and are responsible for binding and carrying oxygen. Therefore, a single hemoglobin molecule can bind and transport up to four oxygen molecules.
- Prosthetic Heme Group: All hemoglobins share the same prosthetic heme group, which is an iron protoporphyrin IX complex. This heme group makes up approximately 4% of the hemoglobin molecule, while the remaining 96% is comprised of the globin component, which is a combination of alpha and beta polypeptide chains. The alpha chain consists of 141 amino acid residues, while the beta chain consists of 146 amino acid residues.
- Heme-Polypeptide Interactions: The ferrous ion (Fe2+) of the heme group is linked to the imidazole nitrogen (N) of two histidine residues within the polypeptide chain. Additionally, the porphyrin ring of the heme group is wedged into its pocket by a phenylalanine residue of the polypeptide chain, providing stability to the heme structure within the globin protein.
The intricate arrangement of the heme groups and polypeptide chains in hemoglobin allows for its oxygen-binding capacity and efficient transport of oxygen throughout the body.
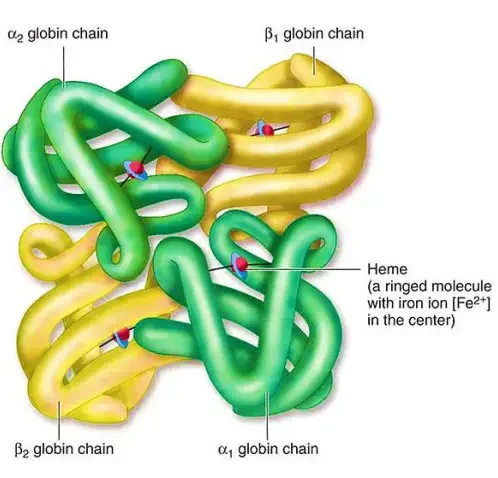
A. Globin Chains
The globin chains are the polypeptide chains that make up the structure of hemoglobin. Here are some key points about globin chains:
- Alpha and Beta Chains: The polypeptide chains of adult hemoglobin are classified into two types: alpha chains and beta chains. These chains have similar lengths but differ in their amino acid sequences.
- Consistency of Alpha Chains: The alpha chain is consistent in all human hemoglobins, whether they are embryonic or adult. This means that the amino acid sequence of the alpha chain remains the same across different types of hemoglobin.
- Non-Alpha Chains: Apart from the alpha chain, there are other non-alpha chains that contribute to different types of hemoglobin. These include:
- Beta Chain: The beta chain is part of normal adult hemoglobin, known as HbA (α2β2). It is one of the main globin chains in adult hemoglobin.
- Gamma Chain: The gamma chain is found in fetal hemoglobin, known as HbF (α2γ2). Fetal hemoglobin has a higher affinity for oxygen compared to adult hemoglobin.
- Delta Chain: The delta chain is associated with minor adult hemoglobin, called HbA2 (α2δ2). HbA2 makes up a small proportion of adult hemoglobin.
- Sickle Cell Hemoglobin: Sickle cell hemoglobin, which is associated with the genetic disorder sickle cell anemia, is denoted as α2S2 (HbS). It results from a mutation in the beta chain of hemoglobin.
- Histidine Residues: The alpha chain of hemoglobin contains 38 histidine residues. Histidine has buffering properties and plays a role in maintaining the stability and function of hemoglobin. The 58th histidine residue is located distal to the ferrous ion, while the 87th histidine residue is proximal to the ferrous ion.
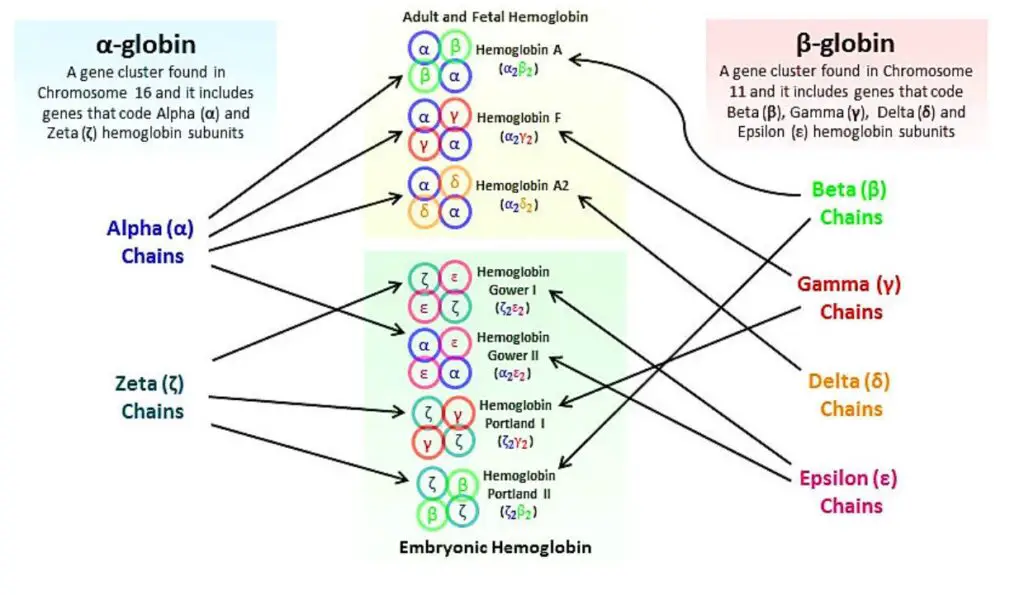
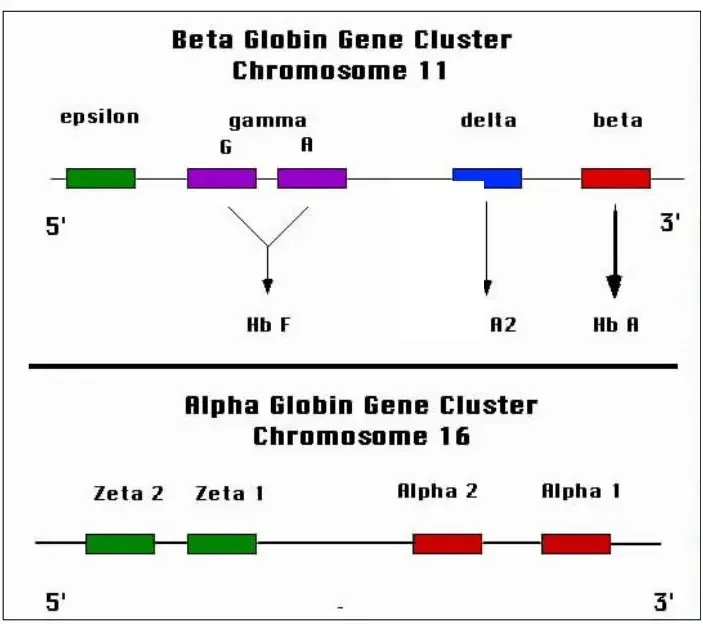
The different combinations of alpha and non-alpha chains in hemoglobin contribute to its functional diversity and determine its properties, such as oxygen affinity and stability.
B. Protoporphyrin IX ring
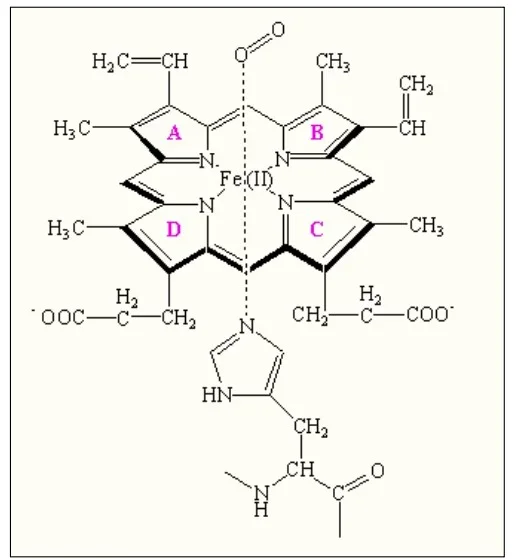
The protoporphyrin IX ring is an essential component of the prosthetic group in hemoglobin. Here are some key points about the protoporphyrin IX ring:
- Composition of the Prosthetic Group: The prosthetic group in hemoglobin consists of a ferrous ion (Fe2+) and a protoporphyrin IX ring. The protoporphyrin IX ring is a cyclic structure that is responsible for binding oxygen and giving hemoglobin its characteristic red color.
- Tetrapyrroles: Protoporphyrin IX is composed of tetrapyrroles. Tetrapyrroles are a class of organic compounds that contain four pyrrole rings, which are five-membered rings composed of four carbon atoms and one nitrogen atom.
- Conjugated Double Bond System: The pyrrole rings in protoporphyrin IX are linked together by methane (=CH-H) bridges, creating a conjugated double bond system. This conjugation gives the protoporphyrin IX ring its planar structure and allows for efficient electron delocalization.
- Ferrous Ion Binding: The ferrous ion (Fe2+) is coordinated to the protoporphyrin IX ring through the nitrogen atoms of the pyrrole rings. Specifically, the ferrous ion is linked to four nitrogen atoms, one from each of the four pyrrole rings in the protoporphyrin IX structure.
- Side Chains of the Pyrrole Rings: The pyrrole rings in protoporphyrin IX are numbered I (A), II (B), III (C), and IV (D). Pyrrole I and II have methyl and vinyl side chains, while pyrrole III and IV have methyl and propionate side chains. These side chains contribute to the overall structure and stability of the protoporphyrin IX ring.
- Oxygen Binding Site: The heme portion of hemoglobin, which includes the protoporphyrin IX ring and the coordinated ferrous ion, is the site where oxygen molecules reversibly bind. When oxygen attaches to the heme group, it forms oxyhemoglobin, allowing for oxygen transport in the bloodstream.
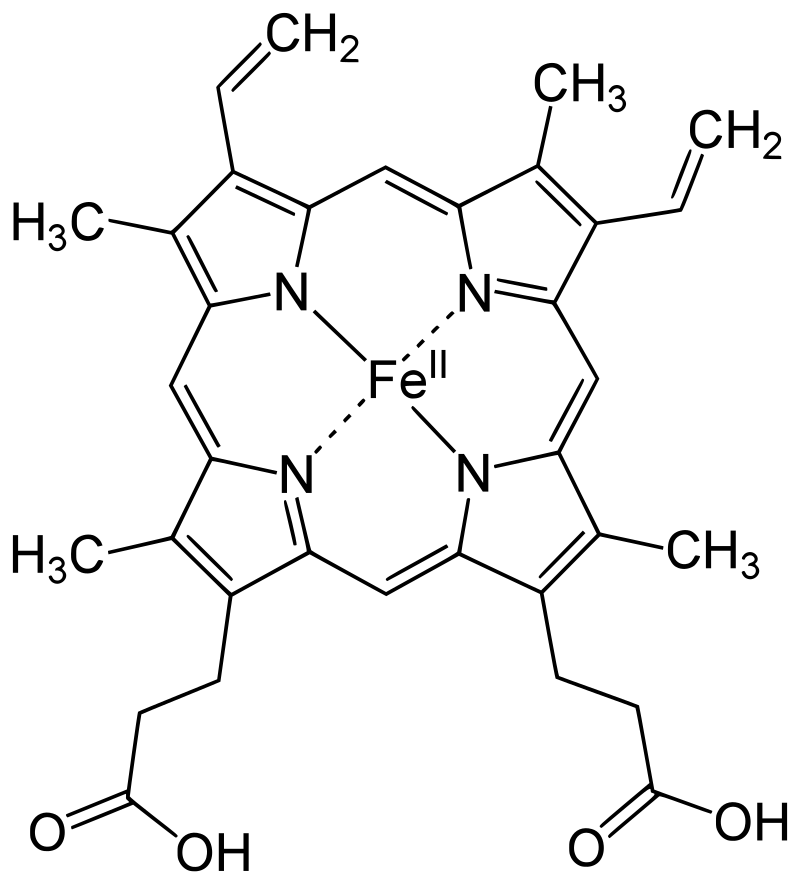
The protoporphyrin IX ring plays a crucial role in the functionality of hemoglobin by providing a site for oxygen binding and facilitating oxygen transport throughout the body.
Four levels structure of Hemoglobin
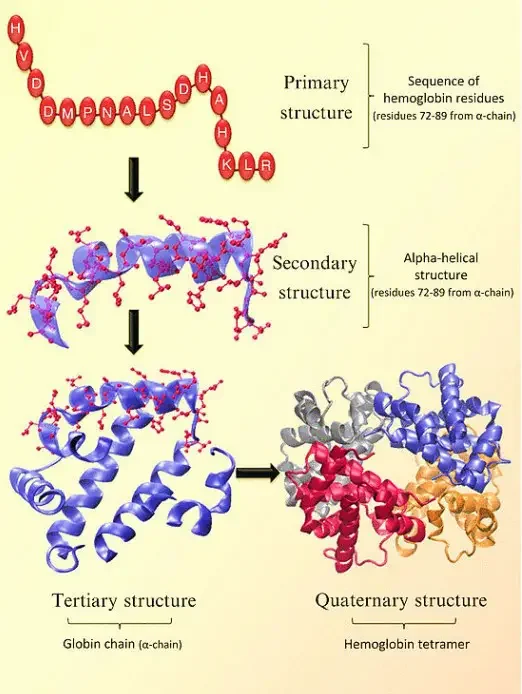
The structure of hemoglobin can be described at four levels:
- Primary Structure: At the primary level, hemoglobin is composed of amino acids arranged in chains. These chains are known as polypeptides or globins. There are four individual globin chains in hemoglobin, each associated with a heme molecule. The primary structure of hemoglobin determines the sequence of amino acids in each globin chain.
- Secondary Structure: The secondary structure of hemoglobin refers to the local folding patterns within each globin chain. It consists of seven alpha helices (coiled structures) and short non-helical segments. These secondary structures contribute to the overall stability and shape of the globin chains.
- Tertiary Structure: The tertiary structure of hemoglobin is influenced by the presence of heme molecules. The heme molecule plays a crucial role in the bending and twisting of the globin chains. It connects to specific histidine residues within the chains, helping to shape the protein. Non-polar residues are located deep inside the protein’s structure, while polar residues are found on the outer surface. The heme group is buried in a hydrophobic pocket, shielded from the surrounding aqueous environment.
- Quaternary Structure: Hemoglobin exhibits a quaternary structure, which refers to the arrangement and interaction of multiple globin chains. Hemoglobin consists of four globin chains: two identical alpha chains and two identical beta chains. These chains come together in a tightly packed arrangement to form a spherical structure. The quaternary structure is stabilized by hydrophobic interactions, hydrogen bonds, and salt bridges between the globin chains.
The four levels of structure in hemoglobin—primary, secondary, tertiary, and quaternary—work together to create a functional protein capable of binding and transporting oxygen throughout the body. The precise arrangement and folding of the globin chains, as well as the interaction with heme molecules, are crucial for hemoglobin’s role in oxygen transport.
R and T state of Hemoglobin
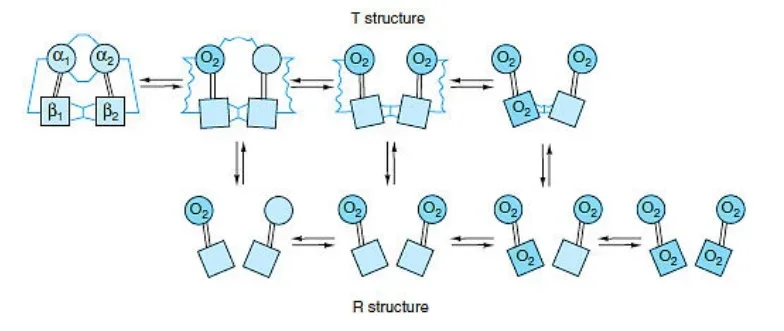
- Hemoglobin exists in two distinct states: the T state (tense state) and the R state (relaxed state). These states represent different conformations of the hemoglobin molecule and are associated with varying affinities for oxygen.
- The T state is characterized by a lower affinity for oxygen compared to the R state. In the concerted mode of cooperativity, hemoglobin can exist in either the T state or the R state. The transition between these states is critical for hemoglobin’s oxygen-binding and release properties.
- In the T state, there are salt bridges that link the subunits of hemoglobin. As oxygen molecules are added, these salt bridges progressively break or weaken, represented by thin and wavy lines. The transition from the T state to the R state does not occur after a fixed number of oxygen molecules have bound to hemoglobin but rather becomes more likely with each successive oxygen binding event.
- The transition between the T and R states is influenced by various factors, including protons (H+ ions), carbon dioxide (CO2), chloride ions (Cl-), and 2,3-bisphosphoglycerate (BPG). Higher concentrations of these molecules promote the transition from the T state to the R state, thereby facilitating the release of oxygen to tissues.
- It is important to note that fully oxygenated hemoglobin in the T state and fully deoxygenated hemoglobin in the R state are not shown because they are unstable intermediates in the oxygen-binding process.
- The ability of hemoglobin to switch between the T and R states allows it to effectively transport oxygen from the lungs to tissues and facilitate oxygen release based on the surrounding conditions and oxygen demands of different body tissues.
Sickle cell hemoglobin
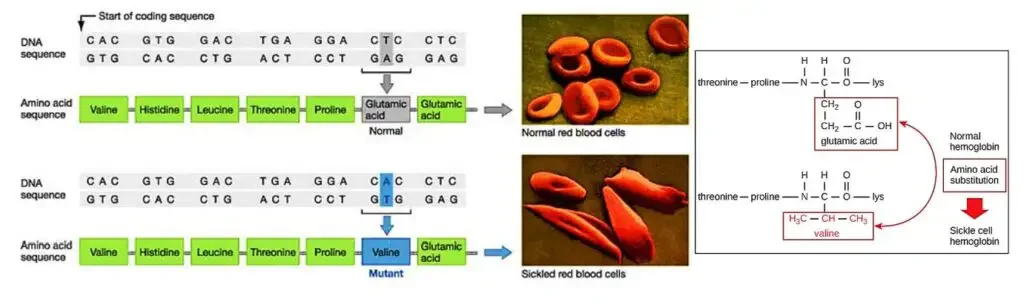
- Sickle cell hemoglobin, also known as hemoglobin S (HbS), is a variant of hemoglobin that is responsible for the development of sickle cell anemia, a blood disease affecting red blood cells.
- The primary difference between sickle hemoglobin and normal hemoglobin is a single amino acid substitution. In sickle hemoglobin, valine replaces glutamate at position 6 on the surface of the beta chain. This alteration in the protein structure leads to significant changes in the behavior and shape of red blood cells.
- In individuals with sickle cell anemia, the defective hemoglobin S replaces the normal hemoglobin A. As a result, the red blood cells gradually change their shape and become rigid and crescent-shaped, resembling sickles or crescent moons. These abnormal red blood cells have several detrimental effects on the body.
- Firstly, the sickle-shaped red blood cells can clog blood vessels, leading to episodes of pain and reducing the supply of oxygen to tissues and organs. This lack of oxygen can cause tissue damage and severe pain known as sickle cell crisis.
- Secondly, the sickle cells have a tendency to get trapped in the spleen, which is responsible for removing old or damaged red blood cells from circulation. The trapped sickle cells are destroyed in the spleen, resulting in a rapid loss of red blood cells. The body is unable to replace the lost cells quickly enough, leading to a condition called anemia, characterized by a deficiency of red blood cells.
- The combination of blood vessel blockages, tissue damage, and anemia contributes to the various complications associated with sickle cell anemia, including pain crises, organ damage, increased susceptibility to infections, and chronic fatigue.
- Sickle cell hemoglobin and the resulting sickle cell anemia are genetic conditions inherited from both parents. Treatment for sickle cell anemia aims to manage symptoms, prevent complications, and improve the overall quality of life for individuals living with this condition. This may involve pain management, blood transfusions, medications, and other supportive measures. Research is ongoing to develop more effective treatments and potential cures for sickle cell disease.
Oxygen saturation
- Oxygen saturation refers to the amount of oxygen that is bound to hemoglobin in red blood cells. Hemoglobin can exist in two forms: oxyhemoglobin, which is saturated with oxygen, and deoxyhemoglobin, which lacks bound oxygen.
- During respiration, oxygen molecules bind to the heme component of hemoglobin in the pulmonary capillaries near the lungs’ alveoli. This process forms oxyhemoglobin. The oxygenated blood then travels through the bloodstream, delivering oxygen to cells where it is used in the production of ATP through oxidative phosphorylation.
- The affinity of hemoglobin for oxygen is influenced by various factors. A taut (tense) form (T) and a relaxed form (R) of hemoglobin exist. The taut form has low oxygen affinity and is favored in conditions such as low pH, high carbon dioxide (CO2), and high 2,3 BPG levels in the tissues. On the other hand, the relaxed form has higher oxygen affinity and is favored by conditions such as high pH, low CO2, or low 2,3 BPG levels. The partial pressure of oxygen in the system also affects the oxygen affinity of hemoglobin. In high partial pressures of oxygen, the relaxed state is favored, while in low partial pressures, the taut state is favored.
- Deoxygenated hemoglobin refers to the form of hemoglobin that lacks bound oxygen. Oxyhemoglobin and deoxyhemoglobin have different absorption spectra. Deoxyhemoglobin has lower absorption of the 660 nm wavelength and slightly higher absorption at 940 nm compared to oxyhemoglobin. This difference is utilized by a medical instrument called a pulse oximeter to measure the oxygen levels in a patient’s blood. It is also responsible for the cyanosis, the bluish or purplish coloration of tissues during hypoxia.
- Furthermore, deoxygenated hemoglobin exhibits paramagnetic properties, meaning it is weakly attracted to magnetic fields. In contrast, oxygenated hemoglobin demonstrates diamagnetism, which causes a weak repulsion from a magnetic field.
- Measuring oxygen saturation is crucial in assessing the oxygen-carrying capacity of the blood and monitoring respiratory function. It helps healthcare professionals determine if a patient is receiving adequate oxygenation and can aid in the diagnosis and management of respiratory conditions.
Iron’s oxidation state in oxyhemoglobin
The oxidation state of iron in oxyhemoglobin (Hb-O2) is a topic of scientific interest and has been the subject of extensive research. Determining the exact oxidation state is challenging because experimental measurements have shown that oxyhemoglobin is diamagnetic, meaning it has no net unpaired electrons, despite both oxygen and iron having paramagnetic ground-state electron configurations.
The lowest-energy form of molecular oxygen is triplet oxygen, which has two unpaired electrons in antibonding π* molecular orbitals. Iron(II) tends to exist in a high-spin 3d6 configuration with four unpaired electrons. Iron(III) has an odd number of electrons (3d5) and must have one or more unpaired electrons in any energy state. Both of these configurations are paramagnetic and have unpaired electrons, whereas oxyhemoglobin is diamagnetic and lacks unpaired electrons.
To explain the observed diamagnetism and absence of unpaired electrons in oxyhemoglobin, a non-intuitive distribution of electrons in the combination of iron and oxygen is necessary. There are two logical possibilities for producing diamagnetic Hb-O2:
- Low-spin Fe2+ binds to singlet oxygen: In this case, both low-spin iron and singlet oxygen are diamagnetic. However, singlet oxygen is the higher-energy form of the molecule.
- Low-spin Fe3+ binds to O2•− (superoxide ion): The two unpaired electrons of the superoxide ion couple antiferromagnetically, resulting in observed diamagnetic properties. In this scenario, the iron is oxidized (loses one electron), and the oxygen is reduced (gains one electron).
The possibility of low-spin Fe4+ binding to peroxide, O22−, can be ruled out because the iron is paramagnetic, even though the peroxide ion is diamagnetic. In this case, the iron is oxidized by two electrons, and the oxygen is reduced by two electrons.
Experimental data provides further insights into the oxidation state of iron in oxyhemoglobin:
- X-ray photoelectron spectroscopy suggests an oxidation state of iron around 3.2.
- Infrared vibrational frequencies of the O-O bond align with those of superoxide (bond order of approximately 1.6, with superoxide being 1.5).
- X-ray Absorption Near Edge Structures at the iron K-edge indicate an energy shift of 5 eV between deoxyhemoglobin and oxyhemoglobin, suggesting a local charge closer to Fe3+ than Fe2+.
Based on these experimental observations, the most likely formal oxidation state of iron in Hb-O2 is +3, with oxygen in the -1 state as superoxide .O2−. The diamagnetism in this configuration arises from the antiferromagnetic alignment of the single unpaired electron on superoxide with the single unpaired electron on low-spin iron (d5 state), resulting in no net spin for the entire configuration.
The second logical possibility mentioned above for diamagnetic oxyhemoglobin, involving singlet oxygen (possibility #1), is unlikely due to its high energy state. Model 3, where low-spin Fe4+ binds to peroxide, is also inconsistent with the magnetic data and involves an unfavorable charge separation. The shift to a higher oxidation state of iron in Hb-O2, along with the decreased size of the iron atom and its interaction with the coordinated histidine residue, initiates the allosteric changes observed in globulins.
Previous models by bio-inorganic chemists proposed the presence of Fe(II) in oxyhemoglobin, assuming that iron remained in the same oxidation state when binding oxygen in the lungs. However, these models failed to explain the presence of diamagnetic singlet oxygen. The revised understanding suggests that iron is oxidized in Hb-O2, and the presence of superoxide .O2− is necessary to “hold” the oxidation electron. The change in iron’s oxidation state and size, as well as the weaker bond of oxygen, are explained by the extraction of an additional electron from iron by oxygen.
It is important to note that assigning a whole-number oxidation state is a formalism, as covalent bonds do not necessarily involve perfect bond orders with complete electron transfer. While all three models for paramagnetic Hb-O2 may contribute to the actual electronic configuration to some extent (via resonance), the model of Fe(III) in Hb-O2 is more accurate than the classical notion of Fe(II) remaining unchanged.
Other oxygen-binding proteins
There are several other oxygen-binding proteins found in various organisms, each with unique characteristics and functions:
- Myoglobin: Found in the muscle tissue of vertebrates, including humans, myoglobin gives muscle tissue a distinct red or dark gray color. It has a similar structure and sequence to hemoglobin but exists as a monomer instead of a tetramer. Unlike hemoglobin, myoglobin does not exhibit cooperative binding and is primarily involved in the storage of oxygen rather than its transport.
- Hemocyanin: This oxygen-transporting protein is the second most common in nature and is found in the blood of arthropods and mollusks. Hemocyanin uses copper prosthetic groups instead of iron heme groups found in hemoglobin. When oxygenated, it gives a blue coloration to the organism.
- Hemerythrin: Certain marine invertebrates and a few species of annelids utilize hemerythrin, an iron-containing non-heme protein, to carry oxygen in their blood. Hemerythrin appears pink or violet when oxygenated and becomes clear when deoxygenated.
- Chlorocruorin: Found in many annelids, chlorocruorin is structurally similar to erythrocruorin, but its heme group differs significantly. It displays a green color when deoxygenated and turns red when oxygenated.
- Vanabins: These proteins, also known as vanadium chromagens, are present in the blood of sea squirts. Although they were initially believed to use vanadium as an oxygen-binding prosthetic group, they bind little oxygen and likely serve another unknown function. Vanabins may have toxic properties in addition to their unclear role.
- Erythrocruorin: Annelids, including earthworms, possess erythrocruorin, a large and complex oxygen-binding protein. Erythrocruorin is a massive protein complex composed of numerous iron- and heme-bearing protein subunits, with a molecular mass exceeding 3.5 million daltons.
- Pinnaglobin: This protein is unique to the mollusc Pinna nobilis and is a brown manganese-based porphyrin protein.
- Leghemoglobin: Leguminous plants like alfalfa or soybeans produce leghemoglobin to protect nitrogen-fixing bacteria in their roots from oxygen. Leghemoglobin, containing an iron heme group, shields the specific enzyme nitrogenase, which is unable to function in the presence of free oxygen.
- Coboglobin: Coboglobin is a synthetic oxygen-binding protein based on cobalt-containing porphyrin. When oxygenated, coboglobin appears colorless, but it turns yellow when deoxygenated.
These diverse oxygen-binding proteins illustrate the adaptations and variations in oxygen transport and storage mechanisms across different organisms and serve as examples of the remarkable biochemistry and diversity found in nature.
Functions of Hemoglobin
Hemoglobin, the protein found in red blood cells, plays several important functions in the body:
- Oxygen Carrier: Hemoglobin is responsible for carrying and transporting oxygen from the lungs to various tissues and organs throughout the body. Each hemoglobin molecule can bind with up to four oxygen molecules, forming oxyhemoglobin. This oxygen binding is crucial for aerobic respiration and the production of ATP.
- Carbon Dioxide Carrier: Hemoglobin also acts as a carrier for carbon dioxide, a waste product of cellular metabolism. Around 20% of carbon dioxide is transported in the bloodstream by binding to hemoglobin, forming carbaminohemoglobin. This helps in the removal of carbon dioxide from the tissues and its transport to the lungs for elimination.
- Imparts Red Color to Blood: Hemoglobin is responsible for the characteristic red color of blood. The iron ions (Fe2+) present in the heme groups of hemoglobin contribute to the red hue.
- Maintains Shape of Red Blood Cells: Hemoglobin helps maintain the shape and flexibility of red blood cells, which are biconcave discs. The interaction between hemoglobin molecules and the cell membrane contributes to the unique shape and structure of red blood cells.
- Buffering Action: Hemoglobin acts as a buffer, helping to maintain the pH balance in the blood. It can bind with excess hydrogen ions (H+) or release them as needed, helping to regulate the acidity or alkalinity of the blood.
- Interaction with Ligands: Hemoglobin can interact with various ligands, such as cyanide, carbon monoxide, and nitric oxide. These interactions have significant implications for gas exchange and can also affect the transport of other substances, such as drugs, to their target sites.
- Production of Physiologically Active Catabolites: When red blood cells reach the end of their lifespan, hemoglobin is broken down, leading to the production of various catabolites. One important catabolite is bilirubin, which is responsible for the characteristic yellow color of feces. Iron released from hemoglobin is stored as ferritin for later use.
Overall, hemoglobin plays a vital role in oxygen transport, carbon dioxide removal, pH regulation, and the maintenance of red blood cell structure and function. Its multifunctional nature makes it a crucial protein for maintaining homeostasis in the body.
Types of Hemoglobin
There are several types of hemoglobin found in the human body:
- Hemoglobin A (HbA): Hemoglobin A is the most common form of hemoglobin found in adult humans. It is composed of two alpha chains and two beta chains. Hemoglobin A is responsible for the majority of oxygen transport in the bloodstream.
- Hemoglobin A2 (HbA2): Hemoglobin A2 is present in small amounts, comprising approximately 2-3% of the total hemoglobin in adult humans. It is formed by the combination of two alpha chains and two delta chains. The function of HbA2 is not fully understood, but it is believed to assist in the regulation of oxygen affinity in red blood cells.
- Hemoglobin F (HbF): Hemoglobin F is predominantly found in newborns and gradually declines after birth. It makes up around 1% of the total hemoglobin in newborns. Hemoglobin F is composed of two alpha chains and two gamma chains. Its higher affinity for oxygen allows efficient oxygen transfer from the mother’s blood to the fetus during development.
These different types of hemoglobin molecules have slight structural and functional variations, allowing for specific adaptations at different stages of life. The relative proportions of these hemoglobin types can change in certain conditions or disorders, such as thalassemia or sickle cell disease, resulting in altered oxygen-carrying capacity and physiological effects.
How does oxygen bind to Haemoglobin?
The process of oxygen binding to hemoglobin involves the following steps:
- In the lungs, oxygen enters the alveoli and diffuses into the blood capillaries. It then binds to the hemoglobin molecules present in red blood cells.
- Each hemoglobin molecule has four binding sites located on the iron atoms within the four heme groups. These binding sites have a high affinity for oxygen.
- As oxygen binds to the heme groups, the shape of the hemoglobin molecule changes. This conformational change allows for easier binding of subsequent oxygen molecules, exhibiting cooperative binding.
- The oxygen binding capacity of hemoglobin is influenced by the partial pressure of oxygen (PO2) in the surrounding environment. In the lungs, where the PO2 is high, hemoglobin readily binds oxygen.
- The relationship between the PO2 and the percentage of oxygen saturation of hemoglobin is depicted by the oxygen dissociation curve or oxygen saturation curve. This curve shows how the binding of oxygen to hemoglobin changes with varying PO2 levels.
- The oxygen dissociation curve exhibits a sigmoid shape due to the cooperative binding nature of oxygen to hemoglobin. It means that the binding of the first oxygen molecule enhances the affinity of hemoglobin for subsequent oxygen molecules.
- As the partial pressure of oxygen increases, the hemoglobin becomes increasingly saturated with oxygen, leading to a higher oxygen-carrying capacity.
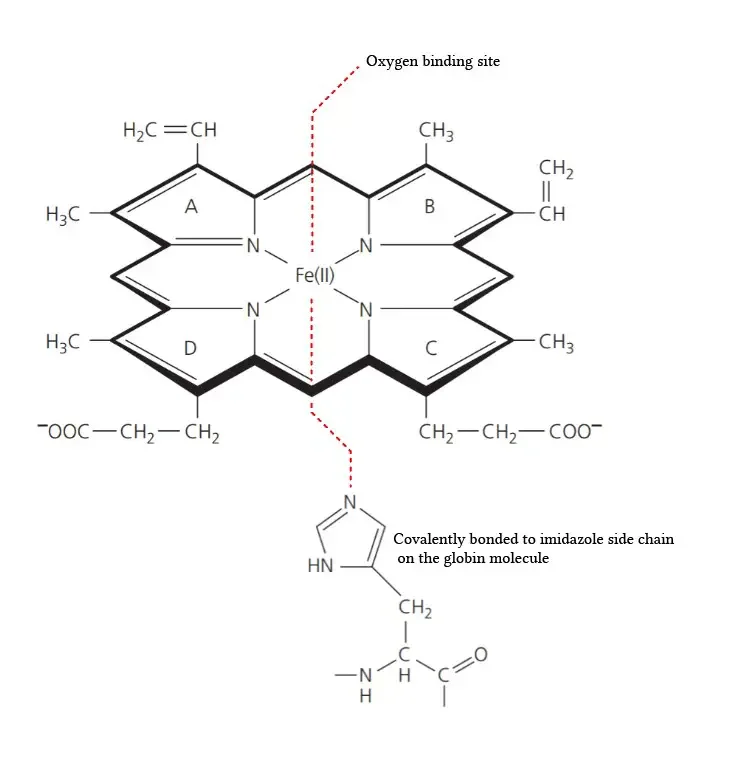
This mechanism ensures efficient oxygen uptake in the lungs and release of oxygen in tissues where the oxygen partial pressure is lower. The ability of hemoglobin to bind and release oxygen is crucial for oxygen transport throughout the body to meet the oxygen demands of various tissues and organs.
Nitric oxide scavenger role of haemoglobin
The role of haemoglobin as a nitric oxide (NO) scavenger is a significant aspect of its function. Nitric oxide is a free radical mediator known for its vasodilatory properties and is used medically as an inhaled pulmonary vasodilator. However, it has a high affinity for haemoglobin, rapidly binding to the ferrous (Fe2+) iron in haemoglobin and forming nitrosylhaemoglobin.
The affinity between nitric oxide and haemoglobin is so strong that the reaction between deoxygenated haemoglobin and nitric oxide is limited only by the diffusion of nitric oxide into the haem pocket. In the presence of sufficient haemoglobin, all nitric oxide can be removed from a solution within moments. This means that the half-life of nitric oxide in solution is significantly reduced when haemoglobin is present.
The normal half-life of nitric oxide in acellular plasma is around 1 minute. However, calculations by Helms & Kim-Shapiro (2014) showed that with a haemoglobin concentration of 10 mmol/L (approximately 64 g/L), the half-life of nitric oxide in solution would be reduced to about 1 µs. This extremely short half-life means that nitric oxide would not have the opportunity to act as an endothelium-derived relaxation factor. Even if a certain amount of nitric oxide is produced at the endothelial surface, it would only be able to diffuse about 0.1 µm within that 1 µs half-life, making it unable to reach the vascular smooth muscle or have any significant effect.
This nitric oxide scavenger role of free acellular haemoglobin has made it unattractive as a resuscitation fluid, to the point that there have been no reported human trials using free haemoglobin solutions. Removing nitric oxide from the endothelium on a whole-body scale would result in widespread vasoconstriction, which would interfere with regional blood flow autoregulation. Studies in animals, such as the experiments conducted by De Figueiredo et al (1997) involving severely shocked pigs, have shown that administering free haemoglobin solutions led to improved blood pressure but no increase in cardiac output, while peripheral and pulmonary arterial pressure increased significantly.
The presence of haemoglobin packaged inside red blood cells is what allows nitric oxide to function as a vasodilator. This packaging increases the diffusion distance for nitric oxide molecules and extends their half-life. Haemoglobin acts as a regulator of regional nitric oxide content in the blood, contributing to the autoregulation of regional blood flow. It ensures that the vasodilatory effects of nitric oxide remain in check. Other mechanisms, such as the conversion and reverse-conversion of nitric oxide to and from nitrite (NO2-), are also controlled by haemoglobin. The direction of these reactions depends on the oxygenation state of haemoglobin, resulting in increased nitric oxide availability in the presence of deoxyhaemoglobin or decreased availability in the presence of oxyhaemoglobin. These mechanisms help explain hypoxia-mediated regional vasodilation in the peripheral circulation and hypoxic pulmonary vasoconstriction in the pulmonary circulation.
In summary, haemoglobin’s role as a nitric oxide scavenger is crucial for regulating the availability and effects of nitric oxide in the body. Its interaction with nitric oxide influences regional blood flow and plays a significant role in vascular regulation.
Haemoglobin Diseases
Haemoglobin diseases are characterized by abnormalities or deficiencies in the structure or production of haemoglobin, leading to various health problems. Some common haemoglobin diseases include:
- Sickle Cell Anaemia: This is an inherited disorder caused by a mutation in the haemoglobin gene. In sickle cell anaemia, a single nucleotide mutation results in the substitution of glutamic acid with valine at the 6th position of the beta-globin chain. This leads to the formation of abnormal haemoglobin called haemoglobin S (HbS), which causes red blood cells to become sickle-shaped. These abnormal cells can block blood vessels, leading to pain episodes and reduced oxygen delivery to tissues and organs.
- Thalassemia: Thalassemia is a group of inherited blood disorders characterized by reduced or absent production of one or more of the globin chains that make up haemoglobin. There are two main types: alpha-thalassemia and beta-thalassemia. The severity of thalassemia depends on the number of genes that are affected or missing. Individuals with thalassemia may experience anemia, fatigue, bone deformities, and other complications.
- Haemoglobinopathies: Haemoglobinopathies are a group of genetic disorders that involve abnormalities in the structure or synthesis of haemoglobin. Examples include HbC disease, HbE disease, and HbD disease. These conditions can lead to varying degrees of anaemia and other symptoms depending on the specific mutation and its impact on haemoglobin function.
- Methemoglobinemia: Methemoglobinemia is a rare condition characterized by the presence of methemoglobin, a form of haemoglobin that cannot bind oxygen efficiently. It can be inherited or acquired and is caused by defects in the enzymes responsible for converting methemoglobin back to its oxygen-binding form. Symptoms may include bluish skin coloration, shortness of breath, and fatigue.
Significance of Haemoglobin in Blood
Haemoglobin is a crucial component of the blood and plays a vital role in the human body. Here are some of the significant roles and functions of haemoglobin:
- Oxygen Transport: The primary function of haemoglobin is to carry oxygen from the lungs to various tissues and organs throughout the body. Oxygen molecules bind to the iron atoms in the haemoglobin protein, forming oxyhaemoglobin. This complex is responsible for transporting oxygen to the body’s cells, ensuring their proper functioning and energy production.
- Carbon Dioxide Transport: Haemoglobin also aids in the removal of carbon dioxide, which is a waste product produced by cells. As blood passes through tissues, haemoglobin binds to carbon dioxide, forming a compound called carbaminohaemoglobin. It transports carbon dioxide back to the lungs, where it is expelled from the body during exhalation.
- Acid-Base Balance: Haemoglobin acts as a buffer and helps maintain the acid-base balance in the blood. It can bind and release hydrogen ions (H+) to regulate pH levels, preventing drastic changes in blood acidity that can disrupt normal bodily functions.
- Red Blood Cell Structure: Haemoglobin is a major constituent of red blood cells (erythrocytes). Its presence gives red blood cells their characteristic red color. The unique structure of haemoglobin enables red blood cells to be flexible and deformable, allowing them to squeeze through narrow blood vessels and transport oxygen efficiently.
- Blood Testing and Health Assessment: Haemoglobin levels are routinely measured as part of a complete blood count (CBC) to assess overall health. Abnormalities in haemoglobin levels can indicate various conditions, such as anaemia (low haemoglobin), polycythaemia (high haemoglobin), or certain blood disorders.
- Oxygen Saturation Measurement: Haemoglobin’s ability to bind oxygen is utilized in pulse oximetry, a non-invasive method to measure oxygen saturation in the blood. A device called a pulse oximeter measures the absorption of light by oxygenated and deoxygenated haemoglobin, providing an estimate of oxygen levels in the blood.
What is Haemopoiesis?
Haemopoiesis, also known as hematopoiesis, is the process by which new blood cells are formed in the body. It takes place primarily in the bone marrow, although some blood cells are also produced in other organs, such as the liver and spleen, during fetal development.
The word “haemopoiesis” is derived from the Greek words “haima,” meaning blood, and “poiesis,” meaning to make. The process involves the proliferation, differentiation, and maturation of blood cells from hematopoietic stem cells, which are multipotent cells found in the bone marrow.
There are three main types of blood cells that are produced through haemopoiesis: red blood cells (erythrocytes), white blood cells (leukocytes), and platelets (thrombocytes). Each type of blood cell has a specific function:
- Red blood cells: These cells are responsible for carrying oxygen from the lungs to the body’s tissues and removing carbon dioxide. They contain a protein called hemoglobin, which binds to oxygen and gives red blood cells their characteristic color.
- White blood cells: White blood cells are crucial components of the immune system and are involved in defending the body against infections and diseases. There are several types of white blood cells, including neutrophils, lymphocytes, monocytes, eosinophils, and basophils, each with its own specialized functions.
- Platelets: Platelets play a vital role in blood clotting. When a blood vessel is damaged, platelets adhere to the site of injury, aggregate together, and form a clot to prevent excessive bleeding.
The process of haemopoiesis is tightly regulated by various growth factors, hormones, and cytokines that influence the production and differentiation of blood cells. Disruptions in haemopoiesis can lead to various disorders, such as anemia, leukopenia (low white blood cell count), and thrombocytopenia (low platelet count).
What is Haemostasis?
Haemostasis refers to the physiological process by which bleeding is stopped or controlled after injury to a blood vessel. It involves a series of intricate mechanisms that work together to maintain blood vessel integrity and prevent excessive bleeding, while also promoting the formation of a clot to aid in wound healing.
The process of haemostasis can be divided into three main stages:
- Vasoconstriction: When a blood vessel is damaged, the smooth muscles in its walls contract, causing the vessel to narrow. This vasoconstriction helps reduce blood flow to the injured area, minimizing blood loss. It is initiated by local reflexes and the release of vasoconstrictor substances.
- Platelet plug formation: Platelets, small cell fragments found in the blood, play a crucial role in haemostasis. When a blood vessel is injured, platelets adhere to the site of injury and aggregate together, forming a platelet plug. This plug temporarily seals the damaged vessel and prevents further bleeding. Platelets release various substances, such as ADP and thromboxane A2, which promote further platelet activation and aggregation.
- Blood clot formation (coagulation): The final stage of haemostasis involves the formation of a blood clot, which reinforces the platelet plug and stabilizes the injured blood vessel. Coagulation, or blood clotting, is a complex process that involves a cascade of reactions. It relies on clotting factors, which are proteins in the blood, and calcium ions. The cascade results in the conversion of soluble fibrinogen into insoluble fibrin threads, which form a mesh that traps blood cells and platelets, leading to the formation of a stable blood clot.
Once the damaged blood vessel is repaired and healing occurs, the clot is gradually dissolved through a process called fibrinolysis, allowing the blood flow to be restored.
Disorders of haemostasis can manifest as bleeding disorders or thrombotic disorders. Examples of bleeding disorders include hemophilia and von Willebrand disease, where the blood’s ability to clot is impaired. Thrombotic disorders, on the other hand, involve an excessive or abnormal formation of blood clots, such as deep vein thrombosis (DVT) or pulmonary embolism (PE).
FAQ
What is hemoglobin?
Hemoglobin is a protein found in red blood cells that is responsible for carrying oxygen from the lungs to the body’s tissues and transporting carbon dioxide back to the lungs.
What is the function of hemoglobin?
The primary function of hemoglobin is to bind with oxygen in the lungs and release it to the body’s cells, while also picking up carbon dioxide and transporting it back to the lungs for removal.
How is hemoglobin measured?
Hemoglobin levels are typically measured through a blood test that provides the concentration of hemoglobin in grams per deciliter (g/dl) of blood.
What factors can affect hemoglobin levels?
Various factors can influence hemoglobin levels, including age, sex, altitude, certain medical conditions (e.g., anemia, kidney disease), nutritional deficiencies (e.g., iron, vitamin B12, folate), and certain medications or treatments.
What is considered a normal hemoglobin level?
Normal hemoglobin levels can vary depending on factors such as age and sex. Generally, for adult males, the range is 14-18 g/dl, while for adult females, it is 12-16 g/dl. However, specific reference ranges may vary between laboratories and healthcare providers.
What does low hemoglobin indicate?
Low hemoglobin levels, known as anemia, can indicate various underlying health conditions such as iron deficiency, vitamin deficiencies, chronic diseases, bleeding disorders, or bone marrow problems.
What does high hemoglobin indicate?
High hemoglobin levels, known as polycythemia, can indicate conditions such as dehydration, lung or heart diseases, bone marrow disorders, or living at high altitudes. In some cases, it may be a sign of an underlying health issue that requires further evaluation.
How can hemoglobin levels be increased naturally?
To increase hemoglobin levels naturally, it is important to maintain a balanced diet rich in iron, folate, and vitamin B12. Foods such as lean meats, leafy greens, legumes, nuts, and fortified cereals can help promote healthy hemoglobin levels. In certain cases, supplements may be recommended by a healthcare professional.
Can exercise affect hemoglobin levels?
Regular exercise can indirectly influence hemoglobin levels by improving cardiovascular health, increasing oxygen utilization, and promoting overall well-being. However, exercise alone typically does not cause significant changes in hemoglobin levels.
What should I do if I have abnormal hemoglobin levels?
If you have abnormal hemoglobin levels, it is important to consult a healthcare professional for a proper evaluation and diagnosis. They can help determine the underlying cause and recommend appropriate treatment or further testing, if necessary. Treatment options may include lifestyle modifications, dietary changes, supplements, medications, or addressing any underlying health conditions.
References
- Marengo-Rowe AJ. Structure-function relations of human hemoglobins. Proc (Bayl Univ Med Cent). 2006 Jul;19(3):239-45. doi: 10.1080/08998280.2006.11928171. PMID: 17252042; PMCID: PMC1484532.
- Ahmed MH, Ghatge MS, Safo MK. Hemoglobin: Structure, Function and Allostery. Subcell Biochem. 2020;94:345-382. doi: 10.1007/978-3-030-41769-7_14. PMID: 32189307; PMCID: PMC7370311.
- https://biochemden.com/hemoglobin/
- https://surendranathcollege.ac.in/new/upload/SUBHADIPA%20MAJUMDER2022-07-22Hemoglobin%20structure.pdf
- https://www.vedantu.com/biology/haemoglobin
- https://www.getbodysmart.com/respiratory-gases-and-their-transport/hemoglobin-structure/
- https://www.uptodate.com/contents/structure-and-function-of-normal-hemoglobins
- https://alevelbiology.co.uk/notes/haemoglobin-structure-and-function/
- https://derangedphysiology.com/main/cicm-primary-exam/required-reading/haematological-system/Chapter%20011/structure-and-function-haemoglobin
- https://derangedphysiology.com/main/cicm-primary-exam/required-reading/haematological-system/Chapter%20011/structure-and-function-haemoglobin
- https://royalsocietypublishing.org/doi/10.1098/rspa.1952.0136
- https://gpnotebook.com/simplepage.cfm?ID=-241565653
- https://thesciencenotes.com/hemoglobin-structure-confirmation-binding-and-transportation-of-oxygen/
- https://labpedia.net/haemoglobin-part-1-hemoglobin-hb-structure-and-functions/
- https://www.sciencelearn.org.nz/images/2144-haemoglobin-structure
- https://collegedunia.com/exams/haemoglobin-biology-articleid-7482
- https://www.geeksforgeeks.org/hemoglobin-definition-structure-and-function/