What is X-Ray Crystallography?
- X-ray crystallography is a method of atomic structural analysis of a crystal using X-rays.
- X-rays striking a crystal cause the atoms to scatter forming a diffraction pattern with information about atom configuration.
- Fourier analysis converts the observed diffraction data mathematically into an electron density map.
- Scientists may create a three-dimensional representation of atom locations inside the crystal by use of this electron density map.
- Understanding the structure of molecules ranging from basic salts to sophisticated proteins and viruses depends on this approach.
- For design of novel pharmaceuticals and materials, it has become an indispensable tool in chemistry, biology, and materials science.
- X‑ray crystallography started with Wilhelm Röntgen’s 1895 discovery of X‑rays.
- Max von Laue proved in 1912 that crystals had an ordered, periodic structure by showing they could diffract X-rays.
- Bragg’s law was developed in 1913 by William Henry Bragg and his son William Lawrence Bragg, therefore defining the basic link among X-ray wavelength, crystal plane spacing, and diffraction angles.
- Their groundbreaking efforts won them Nobel Prizes in Physics and set the groundwork for crystal structure determination.
- X‑ray crystallography’s power and resolution have been much improved over the years by developments like the creation of cryocrystallography, synchrotron radiation utilization, and advanced computational approaches.
- Major scientific discoveries made possible by this progression include the identification of the structures of DNA, proteins, and viruses, therefore changing our knowledge of molecular biology and inspiring developments in technology and medicine.
Principles and Workings of X-Ray Crystallography
- X-ray crystallography is a technique for atom location in a material revealed by X-ray diffraction by crystals.
- An X-ray beam striking a well-ordered crystal causes the regular arrangement of atoms to scatter the X-rays and produce a diffraction pattern of spots.
- Bragg’s law relates the wavelength, the angle of incidence, and the distance between crystal planes to define quantitatively the locations and intensities of these spots under the structure of the crystal.
- Fourier translates the acquired diffraction data into an electron density map that indicates where electrons—and thus atoms—are found in the crystal.
- Researchers put atomic models into the electron density map derived from the diffraction data to create a three-dimensional representation of the molecule.
- The size and quality of the crystal are very important as a well-formed crystal generates a more exact and detailed diffraction pattern.
- Synchrotron radiation and other X-ray generators give extremely strong and focussed X-ray beams that enable quicker and higher-resolution data collecting.
- Cryocrystallography methods freeze the crystal to extremely low temperatures, therefore lowering radiation damage during data collecting and hence retaining crystal quality.
- Modern computer algorithms and automated software process the diffraction data, improve the molecular model, and confirm the ultimate structure.
- Determining the structures of tiny molecules, proteins, DNA, viruses, and other complicated materials has made X-ray crystallography indispensable, thereby considerably improving disciplines including medication design and materials research.
What is Diffraction?
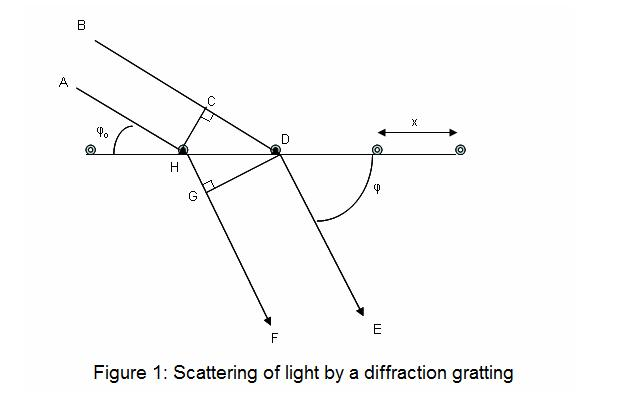
Diffraction is a process that happens when light strikes an obstruction. Light waves can bend around the obstacle or, when a slit is present may pass between the slits. The resulting pattern of diffraction will reveal the areas that are subject to constructive interference when two waves interact in a phase relationship, and destructive interference, in which two waves interact outside of the phase. The process of calculating the phase differs can be explained by looking at the figure below.
In the diagram below, two waves, BD and AH are creating a gradient at an angle . This incidental wave BD travels further than AH by an amount of CD before it reaches the gradient. A scattered wave (depicted beneath the gradient) HF, is farther than the scattering wave DE by an amount of HG. Thus, the total distance between the paths AHGF BCDE and path AHGF BCDE will be CD – HG. To be able to observe a wave of high intensity (one caused by constructive interference) and the differences CD – HG must be equal to an integer amount of wavelengths that can be observed at an angle of that is psi. CD-HG = nl , which represents the frequency of light. Utilizing the basic trigonometric properties The following two equations are able to be drawn on the lines:

What is Bragg’s Law?
The x-ray beam is diffraction. beam happens when light is in contact in the cloud of electrons around the atoms in the crystal solid. Because of the periodic crystalline structure of a solid you can think of it as a sequence of planes that have an interplaner distance equal to. If an x-ray’s light hits the crystal’s surface at an angle ? certain portions of the light will be diffracted at the same distance from the solid (Figure 2.). The remaining light will penetrate the crystal, and certain portions of it will be absorbed by two atoms in the same plane. A portion of the light will be diffracted in an angle that is theta, while the rest will travel further inside the crystal. The process repeats across the various planes of the crystal. The beams of x-rays travel in various lengths before striking the different crystal planes and, after diffraction beams will be constructively interacting only if their path lengths differ by equivalent to an integer amount of wavelengths (just like the normal difffraction scenario earlier). In the diagram below, the difference between lengths of beams striking one plane as well as that hitting the second plane are similar to BG plus. Thus the two diffracted beams are likely to constructively interfer (be at a synchronized angle) only when both beams are BG+GF=nl . The basics of trigonometry tell us that the two beams are equivalent to each other using the interplaner distance multiplied by that of the angle’s sine of . So we get:

The equation is referred to as Bragg’s Law. The name comes from in honor of W. H. Bragg and his son W. L. Bragg who first discovered this geometric relation in 1912. The law of Bragg’s connects to the space between planes of crystals, and also angles of reflection to wavelength of the x-ray. The x-rays diffracted by the crystal must be in phase to transmit a signal. Only angles that meet the following conditions will be registered:
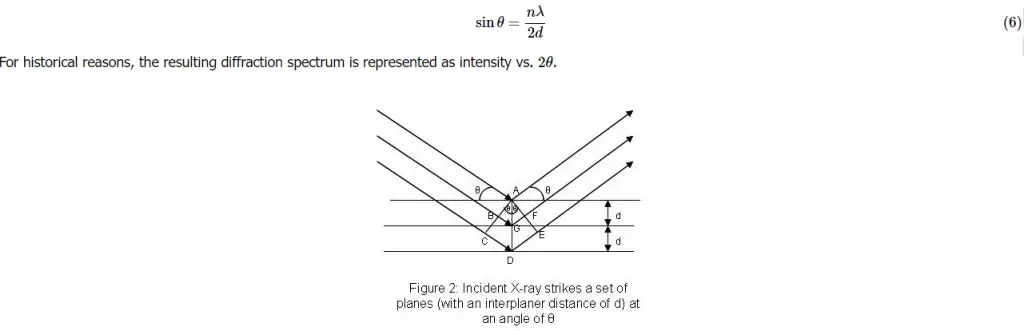
Components of X-Ray Crystallography
X-ray crystallography is a powerful technique used to determine the atomic and molecular structure of a crystal. The main components of X-ray crystallography include:
- X-ray Source:
- X-ray Tube: Produces X-rays by accelerating electrons onto a metal target.
- Synchrotron: Provides highly intense and collimated X-rays, often used for high-resolution studies.
- Crystal Sample:
- Crystal: A solid material whose atoms, molecules, or ions are arranged in an ordered, repeating pattern extending in all three spatial dimensions. The quality and size of the crystal are crucial for obtaining high-resolution data.
- Goniometer:
- A device that holds and precisely rotates the crystal in the path of the X-ray beam. This allows for the collection of diffraction data from multiple angles.
- Detector:
- Image Plate: A photographic film that captures the diffraction pattern.
- CCD Detector: A charge-coupled device that digitally records the diffraction pattern.
- Pixel Array Detector: Advanced detectors that provide high-speed and high-resolution data collection.
- Beam Collimator:
- A device that narrows the X-ray beam to a fine, focused beam, ensuring that it hits the crystal precisely.
- Cryostat (optional):
- A device used to cool the crystal to very low temperatures (often using liquid nitrogen) to reduce radiation damage and improve data quality.
- Data Collection Software:
- Software used to control the goniometer, detector, and X-ray source, and to collect and process the diffraction data.
- Data Processing Software:
- Software used to analyze the diffraction patterns, determine the crystal structure, and refine the atomic model. Examples include:
- HKL-2000: For data integration and scaling.
- CCP4: A suite of programs for crystallographic computations.
- PHENIX: For structure solution and refinement.
- COOT: For model building and visualization.
- Software used to analyze the diffraction patterns, determine the crystal structure, and refine the atomic model. Examples include:
- Computational Resources:
- High-performance computers and algorithms are used to solve the phase problem, refine the atomic model, and simulate the electron density.
- Safety Equipment:
- Lead Shielding: To protect researchers from X-ray radiation.
- Radiation Monitors: To ensure safe levels of radiation exposure.
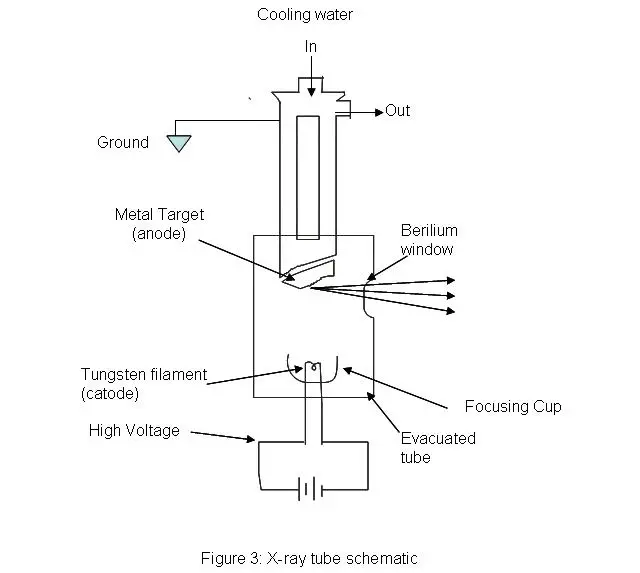
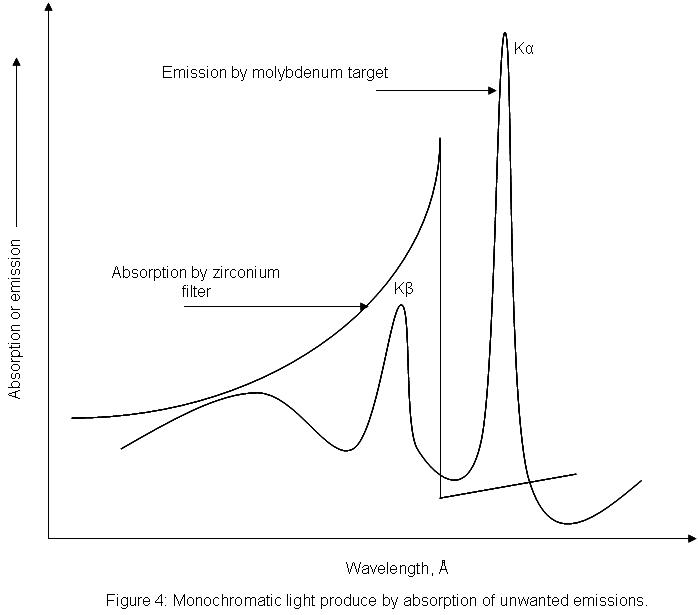
Step by Step procedure of X-Ray Crystallography
- Grow premium crystals from the sample using techniques like microbatch or vapor diffusion.
- Mount the crystal exactly in the course of a monochromatic X-ray beam by use of a sample holder fastened to a goniometer.
- Expose the crystal to the X-ray beam and rotate it; then, record the resultant diffraction pictures with a detector such as imaging plate or CCD.
- Index the reflections, integrate the intensities, and scale the data to create a whole dataset from the gathered diffraction pictures.
- Using methods such molecular replacement, isomorphous replacement, or anomalous dispersion, solve the phase issue to get phase information for every reflection.
- Utilizing the observed amplitudes and the computed phase angles, generate an electron density map by Fourier transformation.
- Fit known sequences and chemical restrictions into the measured density to build an initial atomic model into the electron density map.
- Iteratively refine the atomic model by changing atomic locations and thermal parameters to reduce observed to computed diffraction pattern differences.
- Verify the last structure using statistical tools like the R-factor, then publish the improved model into a public database like the Protein Data Bank.
What is Fourier Transform?
In math in mathematics, the term “fourier transform” is used to describe Fourier transform refers to an action that transforms one real function to another. In the instance of FTIR it is the Fourier transform applies to a function within the time domain in order to transform it to the frequency domain. One method of thinking about this is drawing the illustration of music by recording it on a piece of paper. Each note is placed in the”sheet” domain “sheet” area. Notes of the same kind can be played. The act of playing notes is considered as the process of converting notes of their “sheet” domain to”sound” “sound” area. Each note played represents what’s on the paper but in a different manner. This is exactly that is what the Fourier transform process does to the recorded data of an Diffraction of x-rays. This is done to determine the electron density of crystal atoms in real space. These equations are able to be utilized in determining electron’s location:

In this case, p(xyz) represents the electron density formula where p(xyz) is the electron density function, and F(hkl) corresponds to the electron density value in the real world. Equation 1 describes what is known as the Fourier extension of the electron density functions. To find the formula F(hkl) the equation 1 has to be evaluated across all possible values of h, K, and l leading to equation 2. The resultant formula F(hkl) is typically expressed as a complex figure (as shown at equation 3.) with the F(q)| which is the size of the function, and ph being the component of the function.
What is Crystallization?
To run an experiment using x-rays it is necessary to first create crystals. In organometallic chemical chemistry, a reaction could be successful, but if there is no formation of crystals it’s impossible to identify the components. Crystals form by cooling slowly a supersaturated solution. This solution is created by heating a solution in order to reduce how much solvent is present, and to enhance the solubility of the compound of choice within the solvent. After it is prepared, the solution needs to be slowly cooled.
A rapid temperature drop will result in the compound crashing into the solution capturing impurities and solvent within the newly created matrix. The process continues to cool until the formation of a seed crystal. The crystal is in which the solute will be released out of the solution to the solid phase. Solutions are usually placed in freezers (-78 oC) to ensure that all the substance has crystallized. One method to ensure the gradual cooling process in a -78 freezing is to put the container in which the compound is stored in a beaker of alcohol.
The ethanol serves as an insulator of temperature, which will ensure an even diminution in the temperature difference between the flask as well as the freezer. After the crystals have formed it is crucial that they remain cool, because any energy addition could cause a disruption in the crystal’s lattice. This could result in poor diffraction data. The results of crystallization of an organometallic chromium compound can be seen in the following figure.
How to Mount the Crystal?
Due to the air-sensitivity in the majority of organometallic compounds, crystals are transported using the form of a highly viscous organic substance known as paratone oil (Figure 7 ). Crystals are removed from their Schlenks by squirting the tip of a spatula using paratone oil before gluing the crystal on the oil. While there is a possibility of exposure of the substances to water and air crystals are able to withstand greater exposure than solutions (of preserved protein) before being destroyed. Apart from helping to protect the crystalfrom damage, the paratone oil serves as a glue that binds it to needle.
What is Rotating Crystal Method?
To explain the periodic, three-dimensional nature of crystals The Laue equations are used:
a(cosθo–cosθ)=hλ——(10)
b(cosθo–cosθ)=kλ——-(11)
c(cosθo–cosθ)=lλ——–(12)
where a, b, and c represent the three main axes in the unit cell. however, o,?o represent the angle of radiation that is incident and ?,? represent the angles of diffracted radiation. Diffraction signals (constructive interference) occurs from the fact that h and l are integers. This method of rotating uses these equations. The X-ray radiation is projected onto the crystal as it spins around one cell’s axis. The beam hits on the surface of the crystal with a 90-degree angle. By using equation 1 we can see that if the angle is 90 degrees and costho is 0 degrees, then costho=0 . In order for the equation to be as true, we must set h=0assuming it is 90o . The three equations above are satisfied at different locations as the crystal rotates. This creates the diffractive design (shown in the picture below as multiple different h values). The cylindrical film is removed from the wrapper and then created. The following equation could be utilized to identify the length-axis on which the crystal rotated

In this case, a defines the length and length of the axis it is the distance from zero to the h of concern where an estimate of the size and radius the company has and? represents the frequency of radiation used to generate x-rays. The first wavelength is easily determined However, the two other lengths require more effort and include changing the mounting of the crystal to ensure that it is rotated around the specific direction.
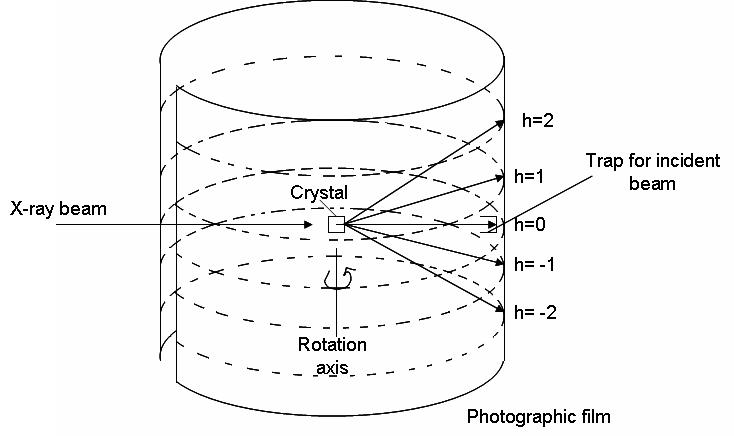
X-ray Crystallography of Proteins
These crystals are thawed in liquid nitrogen before being transferred to the synchrotron, which is a high-powered variable sources of x-rays. They are positioned on a goniometer before being bombarded with beams of radiation. The data is collected when the crystal rotates an array of angles. The angle is determined by the crystal’s symmetry.
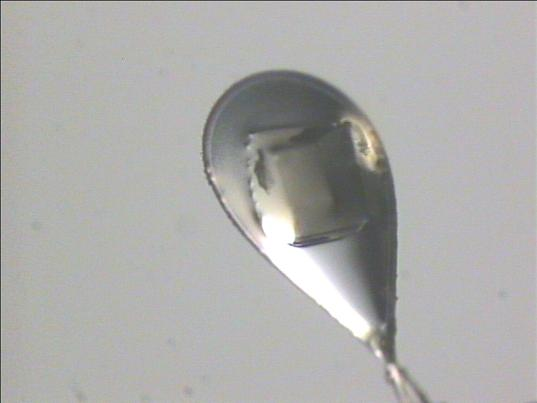
Proteins are one of the numerous biological molecules that are utilized in an x-ray Crystallography research. They are involved in numerous ways in biology, typically catalysts of reactions, increasing the rate at which reactions occur. The majority of scientists employ x-ray crystallography to unravel the structures of proteins as well as to identify the what the functions of proteins, interactions with substrates and relationships with the other proteins and nucleic acid. Proteins may be co crystallized in these substrates or they could be absorbed in the crystal following the process of crystallization.
Protein Crystallization
Proteins can become crystals under certain conditions. These conditions are typically composed of buffers, salts, as well as precipitating agents. This is usually the most difficult part of the process of x-ray crystallography. A multitude of conditions, including pH, salts, buffer and other precipitating substances are incorporated with the protein to make the protein crystallize in the proper conditions. This is achieved with 96 well plates, each one containing a distinct condition and crystalsthat are formed over days weeks, days, or months. The images below show crystals of the APS Kinase D63N from Penicillium chrysogenum . They were taken in the Chemistry building at UC Davis after crystals formed over the course of about a week.
Applications of X-Ray Crystallography
- In biology, X-ray crystallography is used to establish the three-dimensional structures of proteins, nucleic acids, and viruses thereby offering a comprehensive knowledge of molecular function and biological systems.
- of chemistry, it shows the exact atomic configurations of molecules thereby enabling researchers to investigate chemical interactions, reaction processes, and the characteristics of novel compounds.
- X-ray crystallography is used by materials scientists to examine the crystal structures of minerals, metals, and semiconductors, thereby clarifying material characteristics and guiding the design of new materials.
- Structure-based drug development depends on atomic-level understanding of the interaction between drug candidates and their target proteins, which this method provides in pharmacological drug design.
- By examining their distinct crystal structures, forensic experts identify and describe drugs using X-ray crystallography, therefore supporting criminal investigations.
- By means of the technique to probe the atomic structure and flaws in nanomaterials, nanotechnologists promote the creation of creative nanoscale gadgets.
- X-ray crystallography is used by geologists and mineralogists to establish the structures of rocks and minerals, therefore supporting the discovery and categorization of natural resources.
- Applying the method to investigate electron density distributions and lattice dynamics in crystals, physicists advance our basic knowledge of material behavior.
- X-ray crystallography is used in industry quality control and failure analysis by means of crystal phase identification and structural fault detection in produced materials.
- Using the technique to non-destructively examine cultural heritage objects, conservation scientists ascertain their structural integrity and composition for preservation needs.
Advantages of X-Ray Crystallography
- It provides atomic-level resolution of structures, allowing precise determination of atom positions and bonding details.
- It generates detailed electron density maps that are crucial for understanding molecular function.
- It is a non-destructive analytical method, preserving the sample for further analysis.
- It is applicable to a wide range of substances, from small molecules and minerals to large biological macromolecules.
- It supports structure-based drug design by revealing how drugs interact with their molecular targets.
- It benefits from advanced instrumentation, such as synchrotron radiation and free-electron lasers, which enhance data quality and speed.
- It has a well-established framework with robust computational tools for data processing and structure refinement.
- It aids in identifying and characterizing material defects, contributing to materials science and quality control.
Limitations of X-Ray Crystallography
- X-ray crystallography depends on the development of high-quality crystals, hence many compounds—particularly flexible proteins or membrane proteins—are either rather difficult or even impossible to crystallize.
- It produces a stationary, time-averaged shape that might not fully represent the dynamic conformational and mobility range seen in solution.
- Particularly hydrogen, the method has limited sensitivity to light atoms as their low electron density generates somewhat weak scattering signals.
- Since only the amplitudes of the diffracted beams are measured, the phase problem calls for more typically difficult, usually sophisticated techniques (such as molecular replacement or anomalous dispersion) to recover the phase information.
- Extended exposure to strong X-ray beams can cause radiation damage in crystals, therefore degrading the diffraction data quality.
- X‑ray crystallography is essentially confined to crystalline or highly organized materials, rendering it unsuitable for researching amorphous or disordered compounds
- Grown under non-physiological environments, crystals may develop conformations that deviate from their native state, hence producing artifacts in the resultant structures.
- The approach sometimes calls for specialized, costly tools (like synchrotron sources or free-electron lasers) and large computer resources for data processing and structural refinement.
- https://www.news-medical.net/life-sciences/What-is-X-ray-Crystallography.aspx
- https://wiki.anton-paar.com/us-en/x-ray-crystallography/
- https://en.wikipedia.org/wiki/Synchrotron_light_source