What are Viruses?
- Viruses are submicroscopic infectious particles that depend on living cells to replicate and survive. They are found in every type of life form, including animals, plants, and even microorganisms like bacteria and archaea. Unlike other microorganisms, viruses are not considered alive in the conventional sense, as they lack cellular structure and cannot independently carry out life processes. Instead, they exist as a borderline between the living and the non-living, a unique position in the biological world.
- Structurally, viruses are composed of genetic material, either DNA or RNA, which encodes the information for viral reproduction. This genetic core is encased in a protective protein shell known as the capsid, and some viruses possess an additional lipid envelope surrounding the capsid. These viral particles, or virions, come in various shapes and sizes, ranging from simple helical forms to complex structures, but they are generally too small to be seen with standard microscopes.
- The origins of viruses remain unclear, but scientists have proposed several hypotheses. The regressive hypothesis suggests that viruses may have originated from small parasitic cells that lost the ability to survive independently. The cellular origin hypothesis posits that viruses evolved from fragments of DNA or RNA that escaped from living cells. The co-evolution hypothesis, also known as the virus-first hypothesis, proposes that viruses originated alongside the earliest cells. Although none of these hypotheses has been definitively proven, it is widely believed that viruses predate most known life forms and may have contributed significantly to the evolution of life through mechanisms like horizontal gene transfer.
- Viruses can infect a wide range of hosts, although each virus type generally has a specific host range it can infect. Transmission methods vary; for instance, respiratory viruses like influenza and SARS-CoV-2 spread through airborne particles, while gastrointestinal viruses like norovirus are transmitted via the fecal-oral route. Some viruses are transmitted by vectors such as insects, which carry the virus between hosts. The infection process involves a virus entering a host cell and taking control of the cellular machinery to produce thousands of copies of itself, ultimately leading to the release of new virions that can infect other cells.
- In response to viral infections, the immune system activates to eliminate the virus. Vaccines, which introduce weakened or inactive viral particles, help the immune system build a defense against specific viruses. Some viruses, however, such as HIV, can evade immune responses, leading to chronic infections. Antiviral drugs are also used to manage certain viral infections, though their development is often complex due to the rapid mutation rates of many viruses.
- The study of viruses, or virology, has revealed that viruses are ancient entities, likely existing since the earliest forms of cellular life emerged. Because viruses do not leave fossils, researchers rely on molecular techniques to trace their evolutionary history, sometimes using remnants of viral genetic material embedded in host genomes. This genetic evidence helps scientists understand the complex relationship between viruses and their hosts, shedding light on how viruses may have influenced evolutionary processes over millions of years.
- Ultimately, viruses are remarkable for their simplicity yet profound influence on life. While they cannot independently conduct the processes necessary for life, they are integral to the genetic diversity of life on Earth, acting as agents of genetic transfer and evolution in ecosystems worldwide.
Origins of Viruses
Viruses have likely existed since the earliest life forms and have evolved alongside cellular organisms. Due to their lack of fossil records, the origins of viruses remain unclear, and scientists use molecular biology techniques to explore their evolutionary history. Three primary hypotheses attempt to explain how viruses may have arisen:
- Regressive Hypothesis
- Suggests that viruses may have originated from small parasitic cells that once had independent functions.
- These parasitic cells possibly lost essential genes over time as they adapted to a reliance on host cells for survival.
- Evidence supporting this theory includes bacteria like rickettsia and chlamydia, which, like viruses, can only reproduce within host cells due to their dependence on parasitism.
- Also referred to as the “degeneracy” or “reduction” hypothesis, this idea suggests viruses emerged from cells that streamlined their genetic material to maximize their parasitic efficiency.
- Cellular Origin Hypothesis
- Proposes that viruses may have evolved from fragments of genetic material, such as DNA or RNA, that “escaped” from larger cells.
- These genetic fragments might have originated from plasmids (DNA pieces that can move between cells) or transposons, known as “jumping genes,” which replicate and move within the genome.
- Discovered in maize by Barbara McClintock, transposons are a type of mobile genetic element, and the theory posits that these elements might have evolved into viruses.
- Also known as the “vagrancy” or “escape” hypothesis, this perspective suggests viruses emerged from genetic material that became independently mobile.
- Co-evolution Hypothesis
- Known as the “virus-first” hypothesis, it proposes that viruses may have evolved from complex molecules at the same time as the earliest cells on Earth.
- Viruses, according to this theory, may have coexisted and co-evolved with cellular organisms from the beginning.
- Viroids, simple RNA molecules without protein coats that cause plant diseases, resemble subviral agents and support the idea of early viral forms.
- Examples include the hepatitis delta virus, which lacks its protein coat and depends on the hepatitis B virus to replicate, acting similarly to viroids.
- Viruses reliant on other viruses, like the sputnik virophage that needs mimivirus for reproduction, are also known as “satellite” viruses and represent possible evolutionary intermediates between viroids and viruses.
- Challenges with Hypotheses and Re-evaluation
- Each hypothesis has limitations. The regressive hypothesis fails to account for why some cellular parasites do not resemble viruses, while the escape hypothesis cannot explain the complexity of viral capsids and structures.
- The virus-first hypothesis conflicts with the idea that viruses need a host cell to reproduce, making it difficult to consider viruses as originating independently of cellular life.
- Current research reveals that viruses are ancient, potentially predating the divergence of life into distinct domains, which encourages re-evaluation of these hypotheses by modern virologists.
- Molecular Evidence and Evolutionary Analysis
- Studies on RNA cells and comparative DNA analysis provide insights into the evolutionary connections between viruses and their hosts.
- Although none of the hypotheses is definitively proven, molecular studies suggest that viruses likely arose multiple times throughout evolutionary history through a combination of these mechanisms.
Are viruses alive or dead?
The classification of viruses as living or non-living remains debated, as viruses exhibit both characteristics of living organisms and traits typically associated with non-living entities. Viruses are distinct in their acellular, parasitic nature and need a host to perform biological functions. They possess features shared with life forms but lack other essential components, making their classification challenging.
- Characteristics Supporting Viruses as Living Entities
- Reproduction
- Although viruses lack the machinery for independent reproduction, they can replicate within a host. By utilizing the host cell’s nucleic acids and ribosomes, they multiply and spread, which is a basic feature of living organisms.
- Presence of Nucleic Acid
- Viruses contain either DNA or RNA as their genetic material, which allows them to transfer genetic information and undergo mutation, adapting to new conditions. The ability to transmit and mutate genetic material aligns with traits observed in living entities.
- Reproduction
- Characteristics Supporting Viruses as Non-Living Entities
- Lack of Cellular Organization
- Viruses lack cell structure, containing only genetic material enclosed within a protein coat, without protoplasm or cellular organelles. Even their envelope, when present, originates from the host cell membrane rather than a virus-specific structure.
- Absence of Metabolic Machinery
- Viruses lack metabolic systems, such as those required for energy production or respiration. They depend entirely on the host for all energy needs related to replication and do not perform anabolic or catabolic reactions within themselves.
- Inability for Autonomous Reproduction
- Viruses are incapable of self-replication. They rely entirely on the host’s nucleic acids and proteins to replicate their genome, assemble viral particles, and propagate, making them parasitic entities rather than self-sustaining life forms.
- Lack of Growth
- Unlike living organisms, viruses do not grow in size or complexity; they remain static in structure until they infect a host cell, where they replicate without increasing in individual size.
- Non-responsiveness to Stimuli
- Viruses do not respond to environmental stimuli, unlike living organisms that adapt or react to changes like light or sound. While they can be physically altered by environmental conditions, viruses do not show any reflexive response.
- Ability to Be Crystallized
- Viruses can be crystallized, forming solid, organized structures under certain laboratory conditions. This crystalline nature is atypical of living organisms, which cannot be reduced to an inert, crystalline state.
- Lack of Cellular Organization
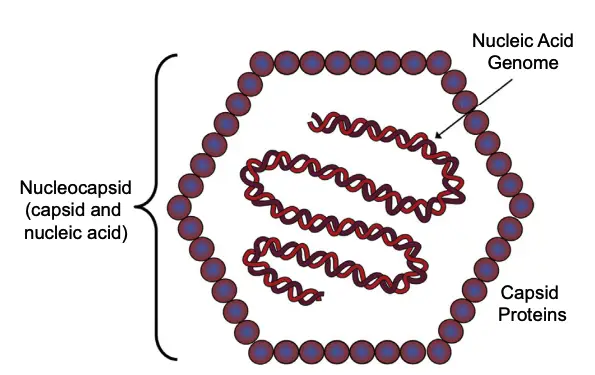
Characteristics of Virus
Viruses represent a unique category of entities that straddle the line between living and non-living. They are non-cellular and obligate intracellular parasites that exhibit distinct structural and functional characteristics. Understanding these traits is essential for comprehending how viruses operate and interact with their hosts.
- Acellular Structure
- Viruses lack cellular organization, which distinguishes them from prokaryotic and eukaryotic cells. They do not possess cell membranes, cytoplasm, or organelles such as mitochondria and ribosomes. Instead, they consist solely of a nucleic acid core surrounded by a protective protein coat known as the capsid.
- Genetic Material
- Each virus contains either DNA or RNA, which serves as its genetic material. This nucleic acid is vital for the virus’s ability to replicate and evolve. The genetic material is encased within the capsid, which is composed of protein subunits that protect the viral genome.
- Size and Microscopy
- Viruses are significantly smaller than prokaryotic cells, with sizes ranging from 20 to 300 nanometers (nm), whereas prokaryotic cells typically measure between 0.5 and 5.0 micrometers (µm). This diminutive size renders viruses ultramicroscopic and capable of passing through bacterial-proof filters, allowing for their classification as filterable agents.
- Reproduction and Activity
- Viruses are unique in that they cannot reproduce independently; they rely entirely on the host cell’s replication machinery. Outside of a host, viruses remain inactive and do not exhibit metabolic processes such as respiration or growth. However, once inside a host cell, they become active and utilize the cell’s enzymes and raw materials to replicate and produce new viral particles.
- Host Specificity and Infection
- Viruses exhibit host specificity, meaning that each type typically infects a particular range of organisms, including animals, plants, and bacteria. This specificity is facilitated by surface spikes, which are viral-encoded proteins that assist in attaching the virus to the host cell receptors. These spike projections play a critical role in receptor recognition and determine the viral tropism, or the range of host cells the virus can infect.
- Resistance and Environmental Adaptation
- Viruses demonstrate remarkable resistance to various environmental conditions, including chemicals and alcohols, which often disrupt cellular structures. This resilience allows them to persist in changing environments and contributes to their survival outside of host cells.
- Crystallization
- Due to their simple structure, viruses can be crystallized, a property that is not typical of living organisms. This crystallization reflects their non-cellular nature and aids in their study through various scientific techniques.
- Lack of Growth
- Unlike living cells, viruses do not grow or increase in size. They remain consistent in size and shape, adopting various forms including helical, icosahedral, envelope, and complex structures based on their classification.
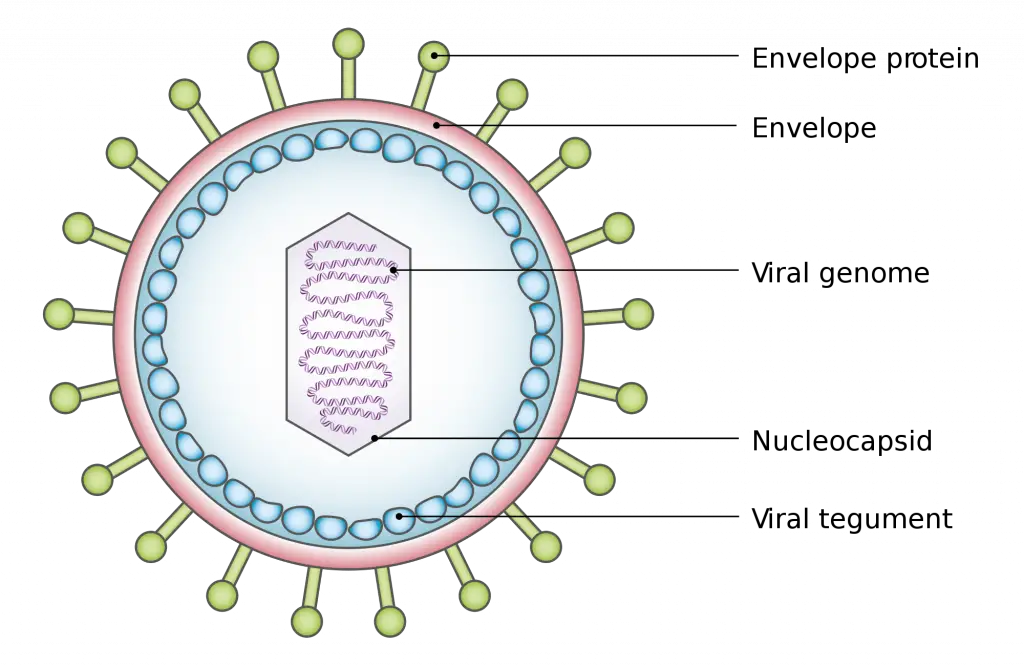
Shape and Size of Viruses
Viruses are notable for their extraordinary diversity in both shape and size, factors that significantly influence their functionality and interaction with host cells. Understanding the variations in viral morphology aids in the classification and study of viruses, as their structural characteristics can reveal much about their biology and behavior.
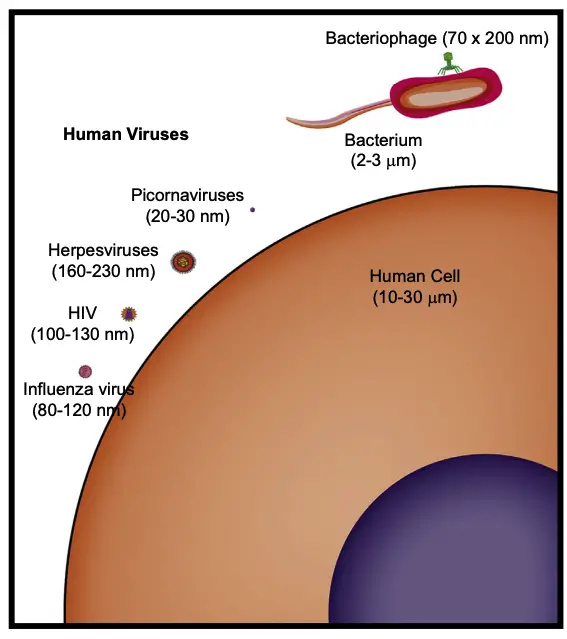
- Size of Viruses
- Viruses can exhibit a remarkable range in size. Some are as small as 20 nanometers in diameter, while others can reach up to 1,400 nanometers in length and 80 nanometers in diameter.
- Giant viruses, which have garnered considerable attention, can measure up to 400 nanometers in diameter, highlighting the wide spectrum of viral dimensions.
- Basic Structural Symmetry
- The viral capsid, which encases the viral genome, generally features two predominant structural symmetries: helical and icosahedral.
- The majority of viruses can be classified as either helical or icosahedral, though a smaller subset exhibits complex structures.
- Icosahedral Structure
- An icosahedron is a geometric form characterized by 20 equilateral triangular faces. While they may appear spherical, advanced imaging techniques such as electron microscopy reveal their true icosahedral nature.
- This structure, also referred to as a cubical form, is prevalent among animal viruses, many of which are enveloped. In contrast, most plant viruses tend to be naked.
- Each triangular face comprises at least three capsomers, necessitating a minimum of 60 capsomers to form a complete icosahedron. The viral genome is entirely encapsulated within this structure.
- Examples of icosahedral viruses include the hepatitis B virus, dengue virus, parvovirus, rhinovirus, human papillomavirus, and herpes virus.
- Helical Structure
- Helical viruses possess a spiral shape, wherein the capsid wraps cylindrically around a central axis that houses the nucleic acid core. The viral genome coils in a helical manner, with capsid proteins winding around it, resulting in an elongated, tube-like nucleocapsid.
- The length of the capsid and the number of capsomers are directly proportional to the length of the viral genome. Helical viruses generally feature shorter genomes, as only a single type of capsomer is needed, thus requiring only one type of gene for capsid production.
- This structure is energetically efficient, requiring less free energy to assemble than icosahedral or complex viruses. Enveloped helical viruses predominantly infect animals, while many well-characterized helical viruses are plant viruses, most of which are naked.
- Notable examples of helical viruses include the Tobacco mosaic virus, influenza virus, measles virus, mumps virus, rabies virus, and Ebola virus.
- Complex Structure
- A small number of viruses display unique architectures that do not conform to either helical or icosahedral shapes; these are classified as complex structures.
- Bacteriophages and poxviruses exemplify this complexity. Poxviruses, for instance, are brick-shaped, enveloped viruses featuring a dumbbell-shaped capsid surrounded by lateral bodies whose functions remain largely unidentified.
- Many bacteriophages exhibit a combination of structures, possessing icosahedral heads connected to cylindrical tail sheaths.
- Similarly, geminiviruses are characterized by two interconnected icosahedral heads, further showcasing the diversity in viral morphology.
The Structure of Virus (Morphology of Virus)
Viruses are complex entities characterized by specific structural components that facilitate their survival and ability to infect host cells. Their structure consists primarily of nucleic acid genomes, protein capsids, and, in some cases, lipid envelopes. Understanding the intricate design of viruses is essential for comprehending their mechanisms of infection and replication.
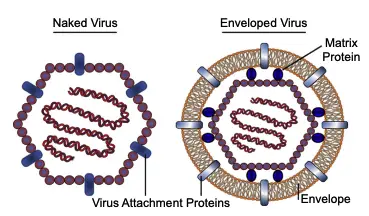
- Nucleic Acid Genome:
- The core of a virus is its nucleic acid, which can be classified as double-stranded DNA (dsDNA), single-stranded DNA (ssDNA), double-stranded RNA (dsRNA), or single-stranded RNA (ssRNA).
- This genome must be shielded effectively to prevent damage from environmental factors such as enzymes, physical stresses, ultraviolet radiation, and radioactivity.
- If the nucleic acid becomes compromised, the virus will fail to produce new virions.
- Capsid:
- The nucleic acid is encapsulated in a protein shell known as the capsid, derived from the Latin word “capsa,” meaning “box.”
- The capsid is constructed from one or more types of proteins that repeat to form a resilient yet flexible structure. This design is analogous to how bricks fit together to create a wall, providing strength and protection for the fragile nucleic acid.
- The combination of the nucleic acid and the capsid is referred to as the nucleocapsid.
- Most viral genomes are relatively small, limiting the number of proteins they can encode. Consequently, the capsid is typically formed from only a few types of proteins repeated numerous times, allowing it to maintain a compact structure.
- Self-Assembly of Capsid Proteins:
- Capsid proteins have the capability to self-assemble, a phenomenon first demonstrated by researchers H. Fraenkel-Conrat and Robley Williams in 1955.
- In their experiments with the tobacco mosaic virus, they separated the RNA genome from the protein subunits. Upon reassembly in a test tube, infectious virions formed spontaneously.
- This self-assembly indicates that no external instructions are required for virus formation; the physical properties of the components are sufficient to facilitate assembly, driven primarily by electrostatic and hydrophobic interactions.
- Envelope:
- Many viruses possess an envelope, which is a lipid membrane derived from the host cell’s membranes, such as the plasma membrane, endoplasmic reticulum, Golgi complex, or nuclear membrane.
- Within the envelope, matrix proteins may be present, serving to anchor the envelope to the capsid.
- Viruses that lack an envelope are referred to as nonenveloped or naked viruses.
- Virus Attachment Protein:
- Embedded in the outer layer of the virus is a protein known as the virus attachment protein.
- In naked viruses, this protein is located within the capsid, while in enveloped viruses, it is found within the envelope.
- This protein plays a crucial role in the virus’s ability to attach to the plasma membrane of the host cell, initiating the process of viral entry.
- Envelope Proteins (Spikes)
- The glycoprotein spikes that extend from the envelope are essential for the virus’s ability to attach to and enter host cells.
- These spikes are often found on viruses such as influenza and HIV, facilitating specific interactions with host cell receptors.
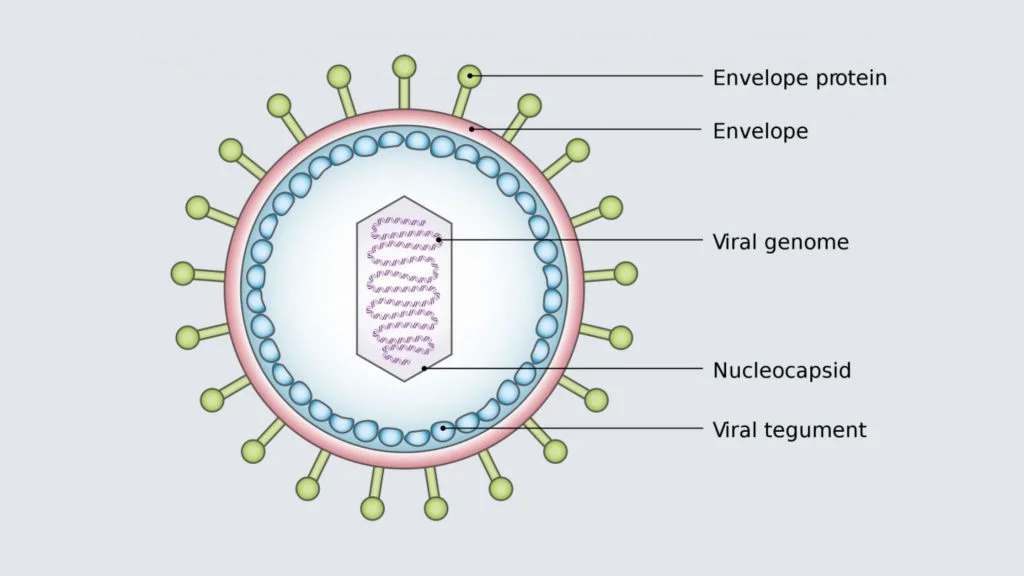
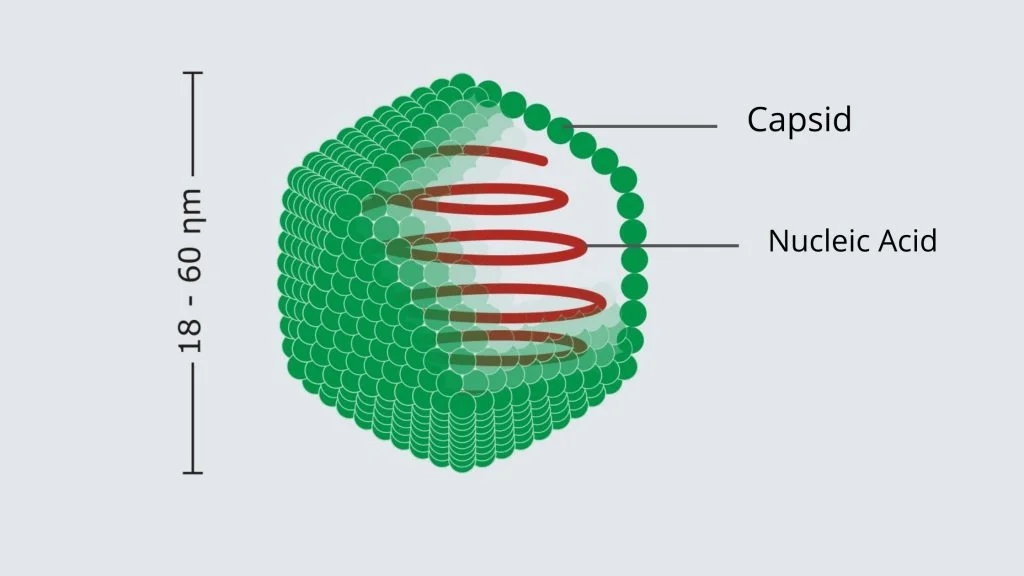
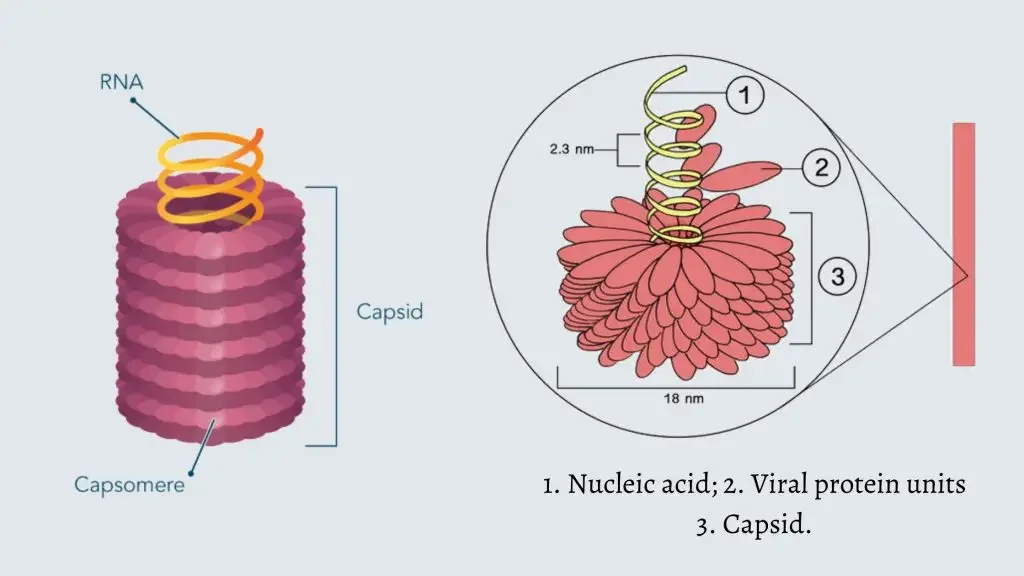
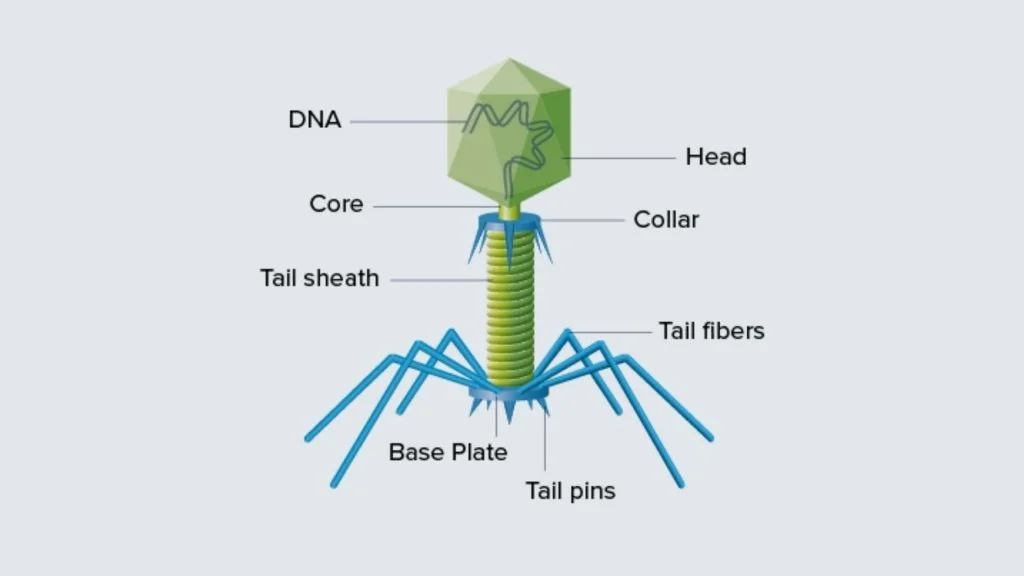
Helical Capsid Structure of Viruses
The structure of viruses is crucial for their survival and ability to infect host cells, with the capsid serving as a protective layer for the viral nucleic acid genome. Among the various capsid forms, the helical structure is particularly significant due to its unique arrangement and functional advantages.
- Definition of Helical Structure:
- A helical capsid is characterized by a spiral configuration that wraps around the viral nucleic acid.
- This spiral shape, known as a helix, curves cylindrically along a central axis, resembling a long tube or rod.
- Many biological structures exhibit this helical form, including certain proteins and the double helix of DNA.
- Formation of the Nucleocapsid:
- In helical viruses, the nucleic acid coils into a helical configuration, with capsid proteins either winding around the inside or outside of the nucleic acid.
- Together, the nucleic acid and the protein shell form the nucleocapsid, with the protein often referred to as the nucleocapsid protein.
- Upon entering a host cell, the helical nucleocapsid uncoils, rendering the nucleic acid accessible for replication and transcription.
- Advantages of Helical Capsid Formation:
- Simplicity in Composition: Only one type of capsid protein is required, which simplifies the structure. This repetitive protein subunit forms the entire capsid, resulting in a structure that demands less free energy for assembly compared to more complex capsids made of multiple proteins.
- Reduced Genetic Requirement: The use of a single nucleocapsid protein translates to the necessity of only one gene instead of several, thereby minimizing the nucleic acid length required for encoding the capsid.
- Flexible Capacity for Nucleic Acid Packaging: The helical design allows for indefinite extension, meaning that there are no constraints on the amount of nucleic acid that can be enclosed within the virion. The capsid’s length can adapt to match the size of the coiled nucleic acid.
- Types of Helical Viruses:
- Helical viruses can be categorized as either enveloped or naked.
- The tobacco mosaic virus, which was the first virus to be described, is an example of a naked helical virus.
- Most plant viruses exhibit helical structures and are predominantly nonenveloped.
- Conversely, all helical viruses that infect animals possess an envelope.
- Notable examples of enveloped helical viruses include the influenza virus, measles virus, mumps virus, rabies virus, and Ebola virus.
Icosahedral Capsid Structure
The icosahedral capsid structure is one of the two predominant forms of viral capsids, characterized by its geometric complexity and efficiency. Unlike helical viruses, where capsid proteins coil around the nucleic acid, icosahedral viruses encapsulate their genomes entirely within a protein shell that exhibits a distinctive icosahedral shape.
- Geometric Configuration:
- An icosahedron consists of 20 equilateral triangular faces, making it a highly symmetrical structure.
- It possesses 2–3–5 symmetry, indicating the different ways it can rotate around its axes: twofold, threefold, and fivefold.
- This symmetry is essential for identifying specific locations on the virus where interactions with host cells occur. For example, if a virus binds to a host receptor at a threefold axis, the interaction takes place on one of the triangular faces, while proteins at the fivefold axis are located at the vertices of the icosahedron.
- Structural Composition:
- Each face of the icosahedral capsid is formed by the assembly of at least three viral protein subunits, which together create what is termed a structural unit.
- These structural units are repeated to construct the entire capsid, with each unit potentially being the same protein or different proteins.
- This repetition allows the formation of larger icosahedral capsids without the need for additional unique proteins, thus increasing the size of the virion efficiently.
- Triangulation Number (T):
- The size of the icosahedral capsid can vary based on the triangulation number (T), which indicates the number of structural units that comprise each triangular face.
- For example, in a T = 1 virus, one structural unit forms each face, while a T = 4 virus consists of four structural units per face.
- In more complex cases, such as T = 3 and T = 7 viruses, structural units may overlap between adjacent faces, allowing for more intricate designs and larger capsids.
- Capsomeres:
- The proteins that make up the structural units can aggregate into three-dimensional formations known as capsomeres, which are visible under an electron microscope.
- In icosahedral viruses, these capsomeres typically take the form of pentons (comprising five units) and hexons (comprising six units), which create a discernible pattern on the surface of the capsid.
- Energy Efficiency and Evolutionary Advantage:
- The prevalence of icosahedral structures among viruses can be attributed to the reduced energy requirements for assembling these capsids compared to other structural forms.
- This efficiency offers an evolutionary advantage, contributing to the widespread occurrence of icosahedral viruses in nature.
- Examples of Icosahedral Viruses:
- Numerous viruses that infect animals exhibit the icosahedral structure, including human papillomavirus, rhinovirus, hepatitis B virus, and herpesviruses.
- Similar to helical viruses, icosahedral viruses can also be either naked or enveloped, and the type of viral nucleic acid—be it double-stranded DNA (dsDNA), single-stranded DNA (ssDNA), double-stranded RNA (dsRNA), or single-stranded RNA (ssRNA)—does not dictate the capsid structure.
Complex Viral Structures
While most viruses exhibit either helical or icosahedral capsid structures, a select group possesses complex architectures that defy these classifications. Such complexity enhances their ability to infect hosts and adapt to various environments. Notable examples of viruses with complex structures include poxviruses, geminiviruses, and many bacteriophages.
- Poxviruses:
- Poxviruses are characterized by their large, oval, or brick-shaped particles, measuring between 200 to 400 nanometers in length.
- Within these complex virions lies a dumbbell-shaped core that houses the viral DNA.
- Surrounding this core are two lateral bodies, whose precise functions remain a subject of ongoing research, indicating that there are still aspects of poxvirus biology that are not fully understood.
- Geminiviruses:
- The name “geminivirus” stems from their unique structure, which comprises two icosahedral heads connected together.
- These plant-infecting viruses exemplify how structural complexity can facilitate specific interactions with host cells, thereby enhancing their infectivity and adaptability.
- Bacteriophages:
- Bacteriophages, or bacterial viruses, are designed to infect and replicate within bacterial cells.
- Many bacteriophages exhibit a complex structure; for instance, bacteriophage P2 features an icosahedral head that contains the viral nucleic acid, attached to a cylindrical tail sheath.
- This tail sheath is crucial for the bacteriophage’s binding to bacterial cells, highlighting the functional significance of structural complexity in viral infection mechanisms.
Classification of Viruses
The classification of viruses is essential for understanding their biology, epidemiology, and impact on health. Various systems have been developed to categorize viruses based on their unique characteristics, which can aid in their study and management. Two of the most widely recognized classification systems are the Baltimore classification and the International Committee on Taxonomy of Viruses (ICTV) classification.
- Baltimore Classification System
- Developed by David Baltimore in the 1970s, this system classifies viruses based on the type of nucleic acid they contain and their method of replication.
- The Baltimore classification divides viruses into seven classes:
- Class I: Double-stranded DNA (dsDNA) viruses
- Class II: Single-stranded DNA (ssDNA) viruses
- Class III: Double-stranded RNA (dsRNA) viruses
- Class IV: Positive-sense single-stranded RNA (+ssRNA) viruses
- Class V: Negative-sense single-stranded RNA (-ssRNA) viruses
- Class VI: RNA viruses that reverse transcribe (Retroviruses)
- Class VII: DNA viruses that reverse transcribe
- ICTV Classification System
- As of April 2023, the ICTV has established a hierarchical taxonomy for viral classification, consisting of 15 ranks based on various properties, including genome type, number of proteins encoded, nucleic acid sequence, virion shape, and capsid structure.
- The most commonly utilized taxonomic ranks in this system are order, family, genus, and species. Further divisions within species can include strains, variants, biotypes, serotypes, or isolates.
- A brief overview of the taxonomic ranks includes:
- Realm: 6 taxa (suffix: –viria)
- Kingdom: 10 taxa (suffix: –virae)
- Phylum: 17 taxa (suffix: –viricota)
- Class: 40 taxa (suffix: –viricetes)
- Order: 72 taxa (suffix: –virales)
- Family: 264 taxa (suffix: –viridae)
- Genus: 2,818 taxa (suffix: –virus)
- Species: 11,273 (no suffix applicable)
- Classification Based on Nucleic Acid
- Viruses can be primarily classified by the type of nucleic acid they possess:
- DNA Viruses: These contain DNA as their genetic material. They can be further divided into:
- Single-stranded DNA (ssDNA) viruses: Examples include Picornaviruses and Parvovirus.
- Double-stranded DNA (dsDNA) viruses: Examples include Adenoviruses and Herpesviruses.
- RNA Viruses: These contain RNA as their genetic material. They can be categorized into:
- Double-stranded RNA (dsRNA) viruses: An example is Reovirus.
- Single-stranded RNA (ssRNA) viruses: These are divided into positive-sense and negative-sense categories. Examples include Poliovirus and Hepatitis A virus for positive-sense, and Rabies and Influenza viruses for negative-sense.
- DNA Viruses: These contain DNA as their genetic material. They can be further divided into:
- Viruses can be primarily classified by the type of nucleic acid they possess:
- Classification Based on Structure and Symmetry
- Viruses can also be classified according to their shape and symmetry, including:
- Helical viruses: These exhibit a spiral shape. Examples include Paramyxovirus and Orthomyxovirus.
- Icosahedral viruses: These display a cubical or radial symmetry. Examples include Reovirus and Picornavirus.
- Complex viruses: Such as Poxvirus, which have more intricate structures.
- Bacteriophages: Characterized by their distinct head-tail structures.
- Viruses can also be classified according to their shape and symmetry, including:
- Classification Based on Replication Properties
- Viruses can be grouped based on their replication properties and sites of replication within host cells. Examples include:
- Replication in the cytoplasm: Most RNA viruses replicate here, with the exception of Influenza virus.
- Replication in the nucleus and cytoplasm: Examples include Influenza virus and Poxvirus.
- Replication in the nucleus: This is the case for all DNA viruses except Poxvirus.
- Viruses can be grouped based on their replication properties and sites of replication within host cells. Examples include:
- Classification Based on Host Range
- The host range of viruses can further delineate their classification, including:
- Animal viruses: Such as Influenza virus and Rabies virus, which infect animal cells.
- Plant viruses: Examples include Tobacco mosaic virus and Potato virus, which exclusively infect plant cells.
- Bacteriophages: Viruses that specifically infect bacterial cells, such as λ phage.
- Insect viruses: These viruses infect insect cells and can serve as biocontrol agents in agriculture.
- The host range of viruses can further delineate their classification, including:
- Classification Based on Mode of Transmission
- The transmission mode of viruses can also provide a basis for classification, including:
- Airborne infections: Such as Swine flu and Rhinovirus.
- Fecal-oral route: Examples include Hepatitis A and Poliovirus.
- Sexually transmitted diseases: Such as Retroviruses and Human papillomavirus.
- Transfusion-transmitted infections: Including Hepatitis B and HIV.
- Zoonoses: Viruses transmitted from animals to humans, such as Rabies and Ebola viruses.
- The transmission mode of viruses can also provide a basis for classification, including:
Virus Genome
The structure of a virus genome is characterized by its diversity in nucleic acid composition and arrangement, which distinguishes it from cellular organisms. Understanding these variations is crucial for comprehending how viruses replicate, infect host cells, and adapt to different environments.
- Nucleic Acid Types: Unlike cellular genomes, which predominantly consist of double-stranded DNA (dsDNA), viral genomes exhibit a broader range of structures:
- Double-Stranded DNA (dsDNA): Some viruses possess a dsDNA genome, which resembles that of cellular organisms.
- Single-Stranded DNA (ssDNA): This form is less common but is significant in certain viral species.
- Double-Stranded RNA (dsRNA): These viruses contain a genome made of dsRNA, playing critical roles in various viral life cycles.
- Single-Stranded RNA (ssRNA): This category is particularly diverse and includes two subtypes:
- Positive-Sense ssRNA (+ssRNA): This form acts similarly to messenger RNA (mRNA), allowing for direct translation into proteins upon entering a host cell.
- Negative-Sense ssRNA (-ssRNA): This form is complementary to mRNA and requires conversion into a positive-sense strand before protein synthesis can occur.
- Genome Variability: Some viruses exhibit unique genomic dynamics, where they may start with one nucleic acid form and convert it to another during their replication process. This ability enhances their adaptability and can influence how they interact with host cellular machinery.
- Implications of Genome Structure: The structural characteristics of viral genomes dictate their replication strategies and influence the development of antiviral therapies. Understanding whether a virus has dsDNA, ssDNA, dsRNA, or ssRNA allows researchers to determine the appropriate methods for targeting these viruses in clinical settings.
- Genomic Organization: Viral genomes can also vary in size and organization. They may contain essential genes for viral replication, protein synthesis, and other functions critical for infectivity. The compact nature of viral genomes often leads to overlapping genes and multifunctional proteins, allowing viruses to maximize their limited genetic material.
Replication of Virus
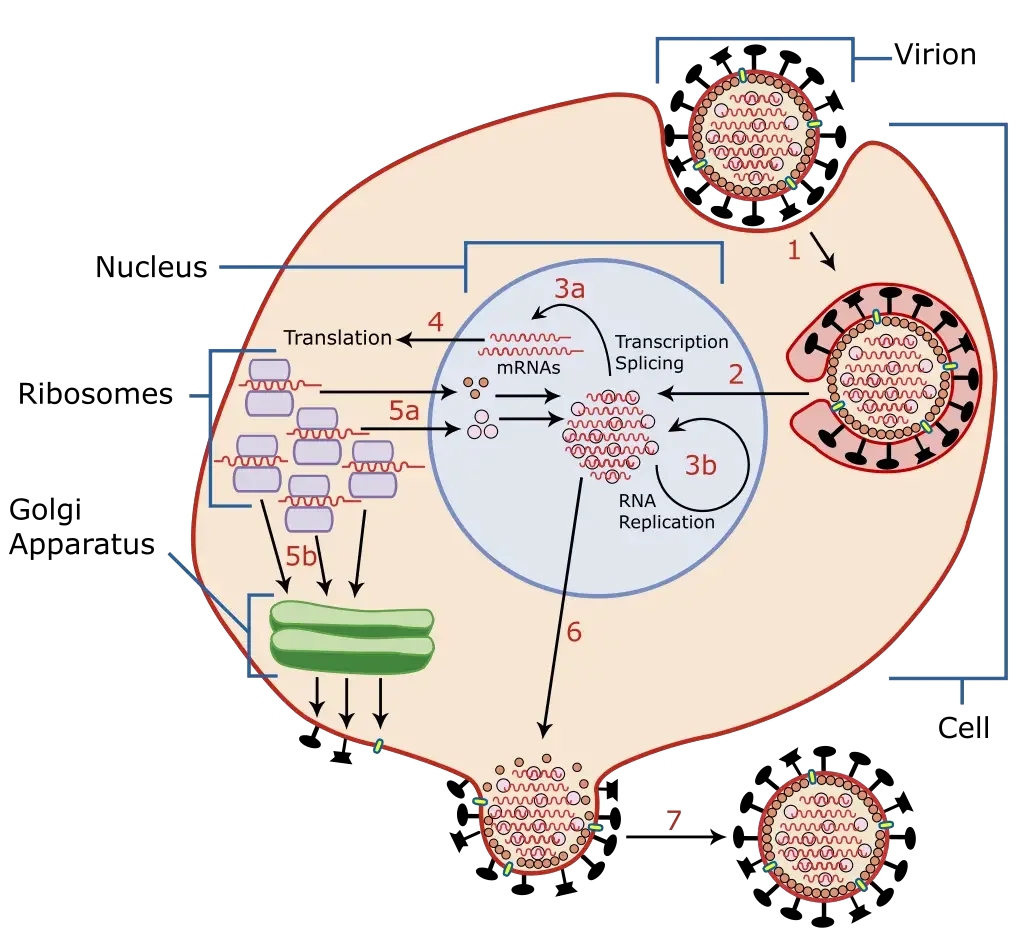
Replication in viruses, while unique due to their acellular nature, is a complex process that allows them to multiply within host organisms. Viruses depend entirely on the metabolic and protein-synthesizing machinery of their host cells to replicate, utilizing various cellular components such as nucleic acids, ribosomes, and enzymes. The replication process can be categorized into seven fundamental stages, each critical for the successful propagation of the virus.
- Attachment
- The initial stage of viral replication begins with the attachment of the virus to the host cell. This is facilitated by specific interactions between viral attachment proteins (VAP) located on the virus’s capsid or envelope and receptor molecules on the host’s plasma membrane, which are often glycoproteins or glycolipids.
- Each virus has a unique receptor it targets; for example, HIV-1 binds to CD4 receptors, while influenza viruses attach to sialic acid residues. This specificity is crucial for determining host range and susceptibility.
- Penetration
- Following successful attachment, the virus must penetrate the host cell membrane to enter the cytoplasm. This process typically requires metabolic energy and can occur through three main mechanisms:
- Receptor-mediated endocytosis: This is the most common method, which includes clathrin-mediated and caveolin-mediated endocytosis, among others.
- Fusion: Enveloped viruses often use fusion, where the viral envelope merges with the host cell membrane, enabling the viral nucleocapsid to enter.
- Translocation: Although less common and not well understood, some viruses may translocate entirely into the host cell.
- Following successful attachment, the virus must penetrate the host cell membrane to enter the cytoplasm. This process typically requires metabolic energy and can occur through three main mechanisms:
- Uncoating
- Uncoating involves the disassembly of the viral capsid, releasing the viral genome into the host’s cytoplasm. This process can occur simultaneously with or shortly after penetration. In bacteriophages, uncoating is equivalent to penetration, as they inject their genome directly into bacterial cells while leaving the capsid outside.
- Replication
- The replication stage is where the viral genome is duplicated, and viral proteins are synthesized to form new virions. The method of replication varies according to the virus’s genome type, with seven distinct strategies corresponding to the Baltimore classification system.
- Generally, most DNA viruses replicate within the host’s nucleus, while RNA viruses typically do so in the cytoplasm. Viral proteins are synthesized first, followed by the replication or transcription of the viral genome to produce numerous copies.
- Assembly
- After replication, the newly synthesized viral proteins and genomes gather at specific sites within the host cell to form immature viral particles. The assembly location depends on the virus’s replication strategy and release mechanism, with most occurring in the cytoplasm.
- Enveloped viruses often assemble at the inner side of the host membrane, embedding their envelope proteins into the host’s lipid bilayer, which later contributes to the viral envelope during the budding process.
- Maturation
- Maturation is characterized by structural and conformational changes in the assembled viral proteins and capsomers, leading to the formation of infectious virus particles. This step is critical for ensuring the stability and infectivity of the virion.
- Release
- The final stage of viral replication is the release of newly formed virions from the host cell. Enveloped viruses typically exit through exocytosis or budding, while nonenveloped (naked) viruses may cause lysis of the host cell, releasing virions in large numbers. The mode of release is significant, as it impacts the host’s cellular integrity and the potential spread of the virus.
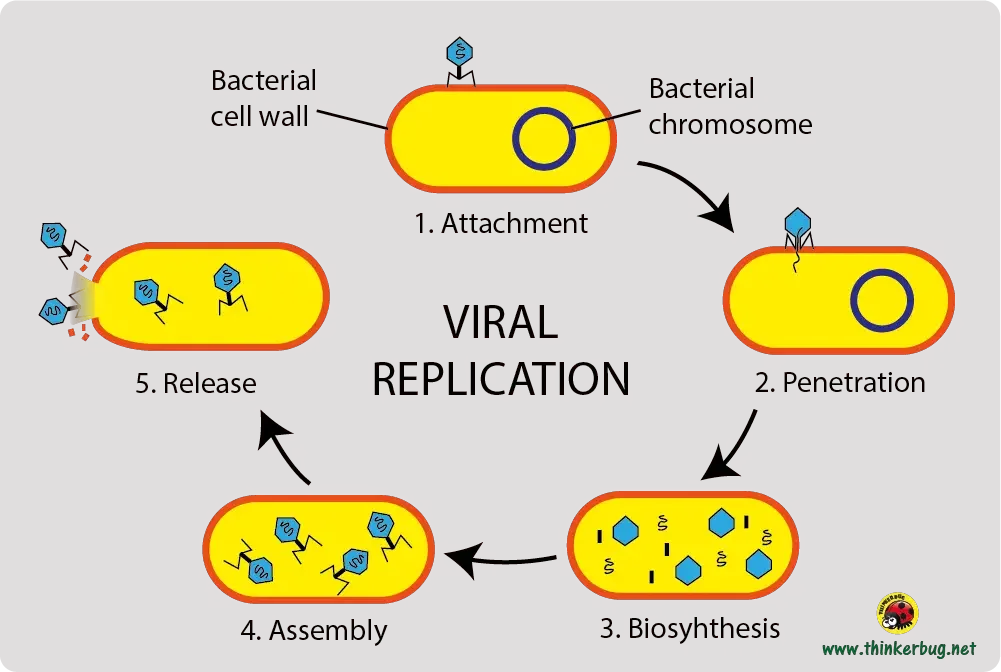
Different Diagnosis Methods of Virus
There are various methods used to diagnose viruses. Here are some of them:
- Molecular tests: These tests detect the genetic material of the virus, such as RNA or DNA, using a technique called polymerase chain reaction (PCR). Molecular tests are highly accurate and can detect viruses even in low concentrations.
- Serological tests: These tests detect the presence of antibodies produced by the body in response to a viral infection. Serological tests can determine if a person has been infected with a virus in the past, but they may not be able to detect a current infection.
- Antigen tests: These tests detect proteins produced by the virus in the body. Antigen tests are faster than molecular tests, but they may not be as accurate and can miss some infections.
- Viral culture: This method involves growing the virus in a laboratory from a sample taken from the patient. Viral culture is a slow process and requires special equipment and expertise.
- Imaging tests: Imaging tests, such as X-rays or CT scans, can help identify the presence of viral infections in certain parts of the body, such as the lungs.
- Clinical presentation: In some cases, a doctor may be able to diagnose a viral infection based on the patient’s symptoms and physical examination.
It’s important to note that different viruses may require different diagnostic methods, and the choice of method may depend on the severity of the illness, the stage of the infection, and other factors.
Significance of Viruses
The significance of viruses extends far beyond their role as pathogens. While they are well-known for causing diseases across various life forms, including humans, their impact on ecosystems, medicine, and biotechnology is profound and multifaceted.
- Disease Causers
- Viruses are recognized as major agents of disease, affecting all life forms. They are responsible for a wide range of illnesses, from mild infections to severe diseases, and are also implicated in various cancers. The ability of certain viruses to induce oncogenesis highlights their potential impact on health and disease dynamics.
- Ecosystem Dynamics
- Viruses play a critical role in maintaining ecological balance, particularly in aquatic environments. They are the most abundant biological entities in these ecosystems and significantly influence biogeochemical cycles. By regulating microbial populations, viruses help sustain the aquatic microbiome, which is essential for nutrient cycling and ecosystem health.
- Evolutionary Impact
- The evolutionary significance of viruses cannot be overlooked. They are believed to contribute to species extinction events and the emergence of new species. Through mechanisms such as horizontal gene transfer, viruses can influence genetic diversity, thus shaping evolutionary trajectories.
- Therapeutic Applications
- Beyond their pathogenic nature, viruses are being explored as therapeutic agents in modern medicine. For example, oncolytic viruses are engineered to target and kill cancer cells specifically, presenting a novel treatment approach. Furthermore, viruses are being investigated for their potential to correct genetic disorders through gene therapy techniques.
- Phage Therapy
- The use of bacteriophages, viruses that infect bacteria, as therapeutic agents represents an innovative strategy to combat antibiotic-resistant infections. Phage therapy harnesses the specificity of bacteriophages to target pathogenic microorganisms without harming beneficial bacteria, thereby offering a promising alternative to traditional antibiotics.
- Biotechnology and Genetic Engineering
- In the field of biotechnology, viruses serve as valuable vectors for gene delivery. They can introduce desirable genetic traits into recipient cells, facilitating advancements in agricultural and medical applications. This gene transfer capability enhances the potential for creating genetically modified organisms (GMOs) with beneficial characteristics.
- Natural Pest Control
- Viruses can also function as natural pesticides and insecticides. Certain viral formulations are used to manage pest populations in agriculture, minimizing reliance on chemical pesticides and promoting sustainable farming practices. This aspect emphasizes the potential of viruses in integrated pest management strategies.
List of Viral Diseases
Viral diseases in humans represent a significant public health challenge, affecting millions globally and leading to considerable morbidity and mortality. Various viruses are responsible for a range of illnesses, from mild infections to severe diseases. Understanding the causative agents and the diseases they provoke is essential for prevention and treatment strategies.
- Common Cold
- The common cold is primarily caused by several viral agents, including rhinovirus, coronaviruses, respiratory syncytial virus (RSV), and parainfluenza virus. These viruses predominantly lead to upper respiratory tract infections, presenting with symptoms such as a runny nose, sore throat, and cough.
- Coronaviruses and Severe Acute Respiratory Syndromes
- Rabies
- The rabies virus (Lyssavirus) is a fatal viral disease primarily transmitted through animal bites. Once symptoms appear, rabies is almost universally fatal, making timely post-exposure prophylaxis critical for survival.
- Pox Viruses
- Diseases such as chickenpox and smallpox are attributed to pox viruses. Chickenpox, typically a childhood illness, can lead to serious complications in adults, while smallpox has been eradicated through successful vaccination campaigns.
- Hepatitis
- Hepatitis viruses are responsible for liver inflammation, leading to chronic health issues. Different types of hepatitis viruses (A, B, C, D, and E) vary in transmission routes and severity, with hepatitis B and C potentially causing chronic liver disease.
- Dengue and Chikungunya
- Dengue viruses cause dengue fever, characterized by high fever, severe headaches, and joint pain. The chikungunya virus leads to similar symptoms but is distinguished by debilitating joint pain that can persist for months.
- Influenza
- Influenza viruses (types A and B) are significant contributors to seasonal epidemics and pandemics. These viruses cause acute respiratory infections and can lead to serious complications, especially in vulnerable populations.
- Poliomyelitis
- Poliovirus is the causative agent of poliomyelitis, a disease that can cause paralysis and permanent disability. Vaccination efforts have significantly reduced its prevalence worldwide.
- Encephalitis and Meningitis
- Various viruses, including Japanese encephalitis virus (JEV) and human polyomavirus 2, can cause encephalitis and meningitis. These conditions represent serious neurological diseases that require prompt medical attention.
- Viral Conjunctivitis
- Adenoviruses and herpes simplex virus (HSV) can lead to viral conjunctivitis, resulting in red, itchy eyes. This condition is often contagious and can spread easily in communal settings.
- Pneumonia
- Viral pneumonia can be caused by RSV, influenza viruses, coronaviruses, and adenoviruses. This type of pneumonia can range from mild to life-threatening, particularly in infants and the elderly.
- HIV-AIDS
- The human immunodeficiency virus (HIV) leads to acquired immunodeficiency syndrome (AIDS), a condition characterized by severe immune system impairment. This virus is primarily transmitted through sexual contact, blood transfusions, and from mother to child.
- Gastroenteritis
- Rotavirus, adenovirus, and noroviruses are major causes of viral gastroenteritis, leading to symptoms like diarrhea, vomiting, and abdominal pain. These infections are particularly concerning in young children and can result in severe dehydration.
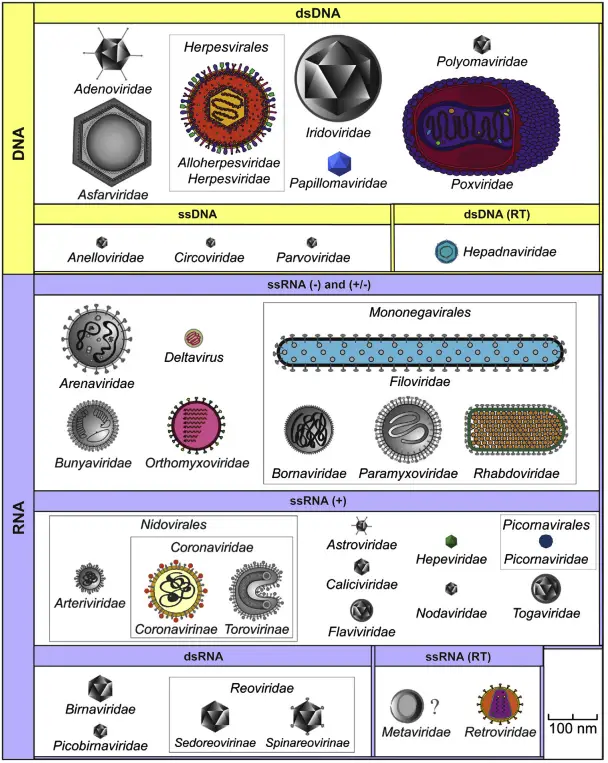
Economic Importance of Virus
The economic importance of viruses extends across various sectors, influencing biotechnology, agriculture, medicine, and environmental management. While often perceived primarily as agents of disease, viruses possess numerous beneficial applications that can significantly impact economies and societal health.
- Biotechnology and Research
- Viruses are pivotal in biotechnology research due to their unique characteristics that straddle the line between living and non-living entities. Their ability to infect specific host cells makes them valuable tools in genetic engineering and molecular biology. In laboratory settings, viruses serve as models for studying genetic functions and processes, thereby facilitating advancements in genetic research.
- Vaccination and Disease Control
- The development of vaccines relies heavily on the use of inactivated or weakened viruses to stimulate immune responses in humans. Vaccines for diseases such as polio, mumps, and hepatitis are created by introducing dead or attenuated viruses into the body, prompting the immune system to develop protection against these pathogens. This process not only protects individual health but also reduces healthcare costs associated with treating viral diseases, leading to significant economic benefits for societies.
- Virotherapy and Gene Therapy
- Virotherapy employs specific viruses to target and destroy harmful bacteria, such as E. coli, thereby managing diseases like dysentery. The T2 bacteriophage virus is an example of this therapeutic approach. Moreover, viruses are being explored as vehicles for gene therapy, wherein they deliver therapeutic genes directly to cells. This technique holds promise for treating genetic disorders and certain types of cancer, potentially reducing long-term healthcare costs and improving patient outcomes.
- Agricultural Applications
- Certain viruses can effectively control pest populations in agriculture. By targeting specific insects and animals that pose threats to crops, viruses can be employed as natural biocontrol agents, reducing the need for chemical pesticides. This not only minimizes chemical usage but also promotes sustainable agricultural practices, contributing to environmental health and economic savings.
- Environmental Management
- Viruses play a crucial role in aquatic ecosystems, where they are the most abundant biological entities. For instance, a spoonful of seawater can contain approximately one million viruses, which help regulate microbial populations and nutrient cycles. Additionally, viruses contribute to carbon cycling by enhancing photosynthesis in marine environments, ultimately aiding in the reduction of atmospheric carbon dioxide by approximately three gigatonnes annually. Such processes can have far-reaching implications for climate change mitigation and the health of marine ecosystems.
- Nanotechnology
- In the field of nanotechnology, viruses are considered organic nanoparticles. Their structural properties and ability to self-assemble make them suitable models for arranging materials on the nanoscale. This application opens avenues for innovative product development and enhanced material properties, further contributing to economic growth through technological advancement.
Examples of a Virus
Here are several examples of different types of viruses, categorized based on their characteristics and the diseases they cause:
- Common Cold Viruses
- Rhinovirus: A primary cause of the common cold.
- Coronavirus: Includes various strains, some of which cause respiratory infections.
- Respiratory Syncytial Virus (RSV): Commonly affects infants and young children.
- Parainfluenza Virus: Causes respiratory infections in children.
- Coronaviruses
- SARS-CoV: Responsible for Severe Acute Respiratory Syndrome (SARS).
- SARS-CoV-2: The virus responsible for COVID-19.
- MERS-CoV: Causes Middle East Respiratory Syndrome (MERS).
- Poxviruses
- Variola Virus: Causes smallpox, which has been eradicated.
- Varicella Zoster Virus: Causes chickenpox and shingles.
- Hepatitis Viruses
- Hepatitis A Virus: Causes viral hepatitis transmitted through contaminated food and water.
- Hepatitis B Virus: Transmitted through bodily fluids; can cause chronic liver disease.
- Hepatitis C Virus: Primarily transmitted through blood; can lead to chronic liver disease.
- Dengue Virus
- Dengue Virus: Transmitted by mosquitoes, causing dengue fever.
- Chikungunya Virus
- Chikungunya Virus: Also transmitted by mosquitoes, leading to fever and severe joint pain.
- Influenza Viruses
- Influenza A: Responsible for seasonal flu and potential pandemics.
- Influenza B: Causes seasonal flu, primarily affecting humans.
- Poliovirus
- Poliovirus: Causes poliomyelitis, which can lead to paralysis.
- Encephalitis and Meningitis Viruses
- Japanese Encephalitis Virus (JEV): Causes encephalitis, transmitted by mosquitoes.
- Human Polyomavirus 2: Associated with certain neurological diseases.
- Arboviruses: A group of viruses transmitted by arthropods, including those causing encephalitis.
- Conjunctivitis Viruses
- Adenovirus: Common cause of viral conjunctivitis (pink eye).
- Herpes Simplex Virus (HSV): Can also cause conjunctivitis.
- Pneumonia Viruses
- Respiratory Syncytial Virus (RSV): Causes pneumonia, especially in young children.
- Influenza Viruses: Both types A and B can lead to pneumonia.
- Adenovirus: Can cause pneumonia in various age groups.
- HIV/AIDS
- Human Immunodeficiency Virus (HIV): Leads to acquired immunodeficiency syndrome (AIDS).
- Gastroenteritis Viruses
- Rotavirus: A leading cause of severe diarrhea in infants and young children.
- Adenovirus: Can cause gastroenteritis, especially in children.
- Noroviruses: Known for causing outbreaks of gastroenteritis.
Difference between Virus and Bacteria
The distinction between viruses and bacteria is critical for understanding their respective roles in health and disease. While both are associated with infections, they are fundamentally different in several key aspects.
- Type:
- Virus: Viruses are classified as acellular entities, meaning they lack cellular structure and cannot carry out metabolic processes independently.
- Bacteria: Bacteria, on the other hand, are unicellular organisms that possess a complete cellular structure, allowing them to perform essential life functions.
- Size:
- Virus: The size of viruses typically ranges from 20 to 400 nanometers (nm), making them significantly smaller than bacteria.
- Bacteria: Bacteria generally measure between 0.2 and 10 micrometers (μm) in diameter, which is considerably larger than viruses.
- Structure:
- Virus: A virus consists of a protein capsid that encases its genetic material, which can be either DNA or RNA. Some viruses also possess an outer lipid envelope.
- Bacteria: Bacteria are characterized by a more complex structure, including a rigid cell wall, a cell membrane, cytoplasm, and DNA, which is typically organized in a single circular chromosome.
- Replication:
- Virus: Viruses are incapable of self-replication and require host cells to reproduce. They hijack the host’s cellular machinery to produce new viral particles.
- Bacteria: Bacteria can replicate independently through a process called binary fission, wherein a single bacterial cell divides into two identical daughter cells.
- Treatment:
- Virus: Treatment for viral infections typically involves antiviral drugs that inhibit viral replication or assembly, as antibiotics are ineffective against viruses.
- Bacteria: Bacterial infections can often be treated with antibiotics, which target specific bacterial functions and structures, leading to cell death or inhibition of growth.
- Diseases Caused:
- Virus: Viruses are responsible for a variety of diseases, including COVID-19, influenza, human immunodeficiency virus (HIV) infections, and smallpox.
- Bacteria: Bacterial infections can lead to conditions such as tuberculosis, pneumonia, and tetanus, among others.
- Kaur, Ramandeep & Taheam, Nisha & Sharma, Anil Kumar & Kharb, Rajeev. (2013). Important Advances on Antiviral Profile of Chromone Derivatives. Research Journal of Pharmaceutical, Biological and Chemical Sciences. 4. 79-96
- Gelderblom HR. Structure and Classification of Viruses. In: Baron S, editor. Medical Microbiology. 4th edition. Galveston (TX): University of Texas Medical Branch at Galveston; 1996. Chapter 41. Available from: https://www.ncbi.nlm.nih.gov/books/NBK8174/
- Louten J. Virus Structure and Classification. Essential Human Virology. 2016:19–29. doi: 10.1016/B978-0-12-800947-5.00002-8. Epub 2016 May 6. PMCID: PMC7150055.
- Speir, J. A., & Johnson, J. E. (2008). Virus Particle Structure: Nonenveloped Viruses. Encyclopedia of Virology, 380–393. doi:10.1016/b978-012374410-4.00600-2
- https://courses.lumenlearning.com/wm-nmbiology1/chapter/viral-morphology/
- https://www.ndvsu.org/images/StudyMaterials/Micro/Structure–of-Virus.pdf
- https://study.com/academy/lesson/video/what-are-viruses-definition-structure-function.html
- https://www.sparknotes.com/biology/microorganisms/viruses/section1/
- https://www.immunology.org/public-information/bitesized-immunology/pathogens-and-disease/viruses-introduction
- https://www.britannica.com/science/virus#ref32740
- https://biologydictionary.net/virus/#examples-of-a-virus
- https://micro.magnet.fsu.edu/cells/virus.html
- https://courses.lumenlearning.com/boundless-microbiology/chapter/structure-of-viruses/
- https://en.wikipedia.org/wiki/Virus
- https://www.khanacademy.org/test-prep/mcat/cells/viruses/a/are-viruses-dead-or-alive
- https://morgridge.org/outreach/teaching-resources/virology-immunology/virus-structure/
- https://www.biologydiscussion.com/viruses/components-viruses/components-of-viruses-4-components/44608
- https://www.britannica.com/science/virus/Size-and-shape
- https://micro.magnet.fsu.edu/cells/virus.html
- https://www.sciencedirect.com/topics/medicine-and-dentistry/viral-structure
- https://open.oregonstate.education/generalmicrobiology/chapter/introduction-to-viruses/
- https://vajiramandravi.com/quest-upsc-notes/virus/
- https://www.biophysics.org/blog/coronavirus-structure-vaccine-and-therapy-development
- https://www.osmosis.org/learn/Viral_structure_and_functions
- https://app.jove.com/science-education/10822/viral-structure