What is a vector in biology?
- A vector, in the context of genetic engineering, is a vital tool used to transfer genetic material from one cell to another. It acts as a carrier, introducing a specific DNA sequence or other genetic material into a new cell. Vectors play a crucial role in various molecular cloning procedures, facilitating the insertion of desired DNA fragments into host cells.
- Vectors are typically DNA sequences that consist of different components serving different functions. They contain an insert, also known as a transgene, which carries the recombinant DNA to be transferred. Additionally, vectors possess a larger sequence called the backbone, which provides structural support to the vector.
- Classification of vectors depends on specific characteristics, and the choice of vector depends on the intended purpose of the genetic engineering process. Vectors are fundamental in genetic engineering as they form the basis for transferring DNA fragments between cells. They possess unique features that allow the gene sequences to survive within the host cell.
- The process of gene transfer can vary depending on the type of vector used. Some vectors integrate into the host DNA after entering the cell, while others solely pass the genetic material to the host cell without integrating themselves. Additionally, besides DNA sequences, viruses and other particles can also serve as vectors in processes such as transduction.
- Vectors can be reused in multiple processes as they can be recovered at the end of each procedure. Cloning vectors, specifically designed for cloning purposes, are a specific category of vectors. These vectors contain sequences that enable them to initiate replication within host cells and propagate themselves effectively.
- In summary, vectors are essential tools in genetic engineering that enable the transfer of genetic material between cells. They play a vital role in molecular cloning procedures, facilitating the insertion of desired DNA fragments. Understanding the characteristics and types of vectors is crucial for effective genetic engineering processes.
Vector Definition
A vector is a DNA molecule or genetic material used to transfer specific genetic information between cells in molecular biology and genetic engineering.
Characteristics Features of vectors
The following outlines the key features and functions of vectors, providing insights into their design and effectiveness.
- Autonomous Replication: Vectors must possess specific sequences that facilitate autonomous replication within a host cell. This ability ensures that the vector, along with its inserted DNA, can multiply independently, promoting efficient propagation and expression of the desired genes.
- Optimal Size: An ideal vector should be sufficiently small to facilitate its incorporation into the host genome. Smaller vectors not only enhance the likelihood of successful integration but also allow for the accommodation of larger inserts of genetic material, which is critical for various applications.
- Isolation and Purification: Effective vectors should be easily isolatable and purifiable. This characteristic is important for recovering vectors after they have served their purpose in gene transfer experiments, enabling their reuse in subsequent applications.
- Selectable Marker Genes: To determine whether the host cell has successfully incorporated the vector, it is essential to include marker genes. These genes typically confer resistance to antibiotics or produce specific proteins, providing a straightforward method to identify transformed cells.
- Restriction Enzyme Sites: Many vectors are engineered with unique restriction enzyme recognition sites. These sites facilitate the insertion of foreign DNA by enabling precise cleavage at specific locations. Additionally, vectors often contain multiple cloning sites (MCS), which offer a range of restriction sites to accommodate various enzymes, thereby increasing versatility in genetic manipulation.
- Ease of Introduction: The methodology used to introduce vectors into host cells should be straightforward and efficient. The effectiveness of this process can depend on multiple factors, including the type of vector, the host organism, and the chosen delivery method, such as electroporation, microinjection, or viral transduction.
- Integration Capability: For vectors employed in gene transfer processes, it is crucial that they can integrate their recombinant DNA into the host genome. Successful integration allows for stable expression of the transgene, which is vital for long-term studies or applications in gene therapy.
- Preservation of Vector Functionality: It is imperative that the introduction of recombinant DNA into a vector does not disrupt its replication cycle. The integrity of the vector’s replication mechanism must be maintained to ensure consistent performance and reliability during genetic experiments.
- Adaptability: Vectors should exhibit flexibility in their design to accommodate various types of genetic material. This adaptability allows researchers to modify vectors to meet the specific requirements of different experimental conditions or target organisms.
Types of vectors
Vectors are classified into these following types;
- Cloning Vectors: Cloning vectors are specialized vectors used in molecular cloning procedures. These vectors have specific features that allow for the replication and propagation of DNA fragments within host cells. They typically contain essential elements such as an origin of replication, selectable markers, and cloning sites to facilitate the cloning and manipulation of DNA fragments.
- Viral Vectors: Viral vectors are vectors derived from viruses and are widely used in gene therapy and gene delivery applications. These vectors are engineered to carry and deliver therapeutic genes or desired genetic material into target cells. Viral vectors utilize the natural ability of viruses to infect cells, making them efficient delivery vehicles for introducing genetic material into host cells.
- Expression Vector: Expression vectors are designed to facilitate the expression of genes in host cells. These vectors typically contain regulatory elements such as promoters, enhancers, and terminators that control gene expression. Expression vectors are widely used in molecular biology research to produce proteins of interest or study gene function by inducing the expression of specific genes in host cells.
- Shuttle Vector: Shuttle vectors are versatile vectors that can replicate in multiple host organisms. These vectors possess sequences allowing them to be maintained in different host species, such as bacteria and eukaryotic cells. Shuttle vectors enable the cloning and manipulation of DNA fragments in one host system and subsequent transfer into another host system for further analysis or expression.
- Secretion Vector: Secretion vectors are designed to facilitate the secretion of proteins or peptides of interest into the extracellular space. These vectors contain signal sequences that direct the protein to be synthesized and secreted from the host cell. Secretion vectors are commonly used in biotechnology and protein production applications, as they allow for easy recovery and purification of the secreted proteins.
1. Cloning vectors
Cloning vectors are essential tools in molecular biology, specifically designed to facilitate the replication and maintenance of recombinant DNA within host organisms. They serve as vehicles for inserting foreign DNA into host cells, thereby enabling the study and manipulation of genetic material. Understanding the various types of cloning vectors and their unique features is critical for applications in genetic engineering, gene therapy, and genomics.
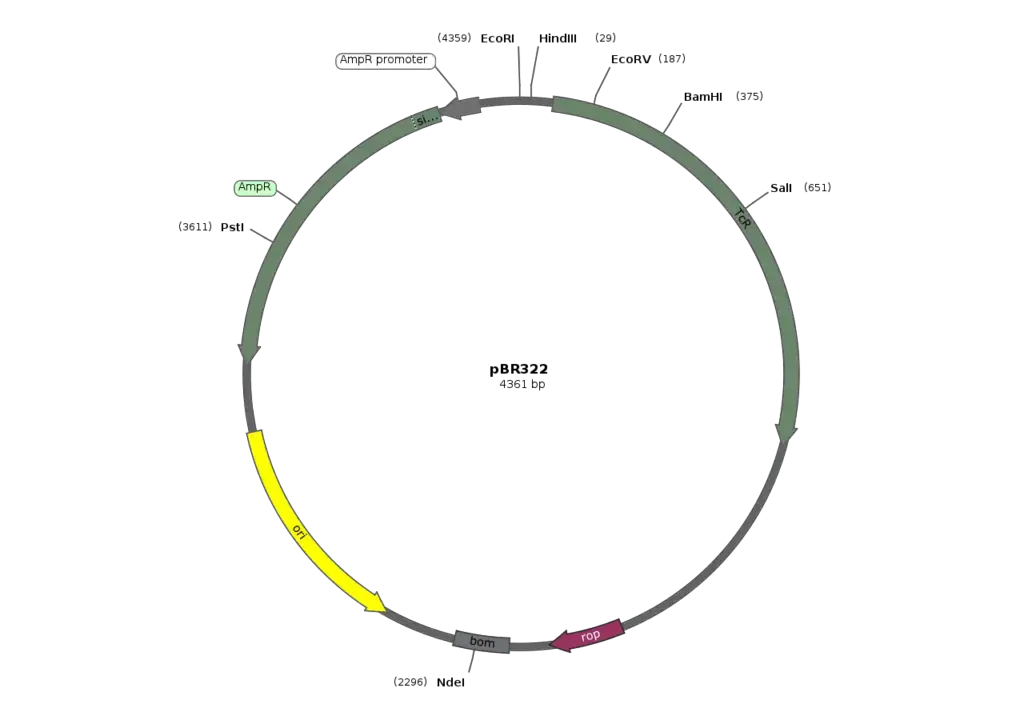
- Definition and Purpose: Cloning vectors are specialized plasmids or other DNA constructs capable of autonomous replication. Their primary purpose is to enable the insertion, propagation, and manipulation of specific DNA sequences within host cells, thereby aiding in the study of gene function and the production of proteins.
- Types of Cloning Vectors: Cloning vectors can be classified into several categories, each defined by unique characteristics and capabilities.
- Plasmid Vectors:
- Plasmids are circular, extrachromosomal DNA molecules that replicate independently within bacterial cells, primarily E. coli.
- These vectors typically range from a few thousand base pairs to over 100 kilobases in size. They can efficiently carry insert DNA up to approximately 20 kb, although larger inserts may reduce cloning efficiency.
- The autonomous replication of plasmids is facilitated by origin of replication (ori) sequences, allowing for stable maintenance and propagation of the vector.
- Common plasmid vectors include pBR322, pUC, and pBluescript, which often feature selectable markers such as antibiotic resistance genes.
- Cosmid Vectors:
- Cosmids are hybrid vectors that combine features of plasmids and bacteriophage λ, enabling the incorporation of larger DNA fragments, typically up to 42 kb.
- These vectors are constructed by integrating the cos region of a phage vector into a plasmid backbone, allowing for efficient replication within host cells.
- Cosmids are particularly advantageous for cloning larger DNA sequences that exceed the capacity of standard plasmids, making them valuable in genomic library construction.
- Bacteriophage Vectors:
- Bacteriophage vectors, such as λ phage, are viral vectors specifically designed to infect bacterial cells and facilitate the transfer of large DNA inserts.
- Phages exhibit high transformation efficiency, which enhances the recovery of clones containing the desired recombinant DNA.
- The packaging system of phage vectors allows the incorporation of substantial eukaryotic genes (up to about 50 kb) along with their regulatory elements, providing an effective means for gene cloning and analysis.
- Bacterial Artificial Chromosomes (BACs):
- BACs are engineered vectors derived from the F-factor of bacteria, capable of cloning large DNA fragments up to 300 kb in size.
- These vectors maintain a supercoiled circular form, promoting stability and reducing insert chimerism during propagation.
- BACs are commonly used in genomic mapping, positional cloning, and sequencing projects due to their capacity for maintaining large inserts with minimal rearrangement.
- Yeast Artificial Chromosomes (YACs):
- YACs are eukaryotic vectors that can clone extensive DNA segments, up to 500 kb, within yeast cells such as Saccharomyces cerevisiae.
- They are particularly useful for cloning large genomic sequences and studying gene function in a eukaryotic context.
- Despite their advantages, YACs may introduce high levels of chimerism and insert rearrangements, which can complicate downstream applications.
- Human Artificial Chromosomes (HACs):
- HACs are designed to function as new chromosomes within human cells, enabling the stable maintenance of large genomic sequences without integration into the host genome.
- These vectors can incorporate virtually any size of DNA insert, facilitating studies of gene expression and regulation.
- While HACs hold promise for gene therapy and research, they face technical challenges in gene loading and structural characterization.
- Plasmid Vectors:
2. Viral vectors
Viral vectors represent a powerful and versatile approach for gene transfer, enabling the manipulation of host cells and tissues to express specific genes. By harnessing the natural ability of viruses to infect host cells, researchers can modify these pathogens to deliver therapeutic genes effectively.
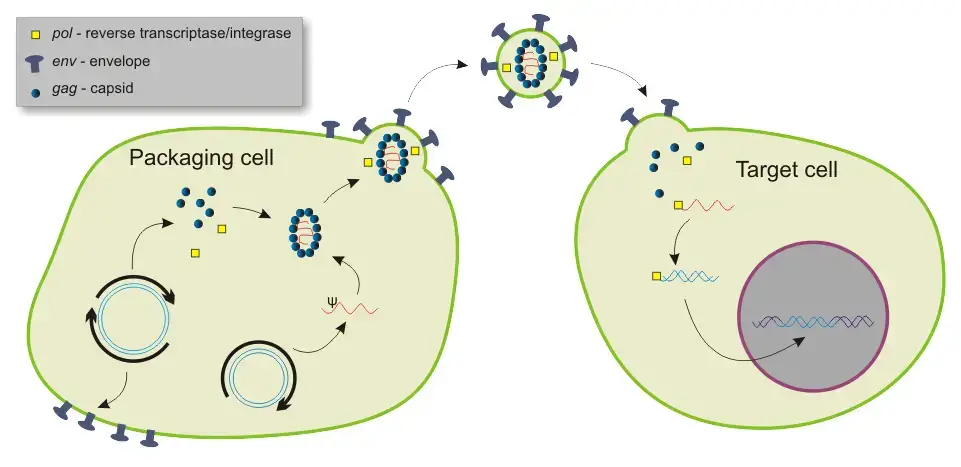
- Definition and Mechanism: Viral vectors are engineered viruses that have been modified to replace non-essential viral genes with foreign DNA sequences of therapeutic interest. This modification results in recombinant viral vectors that can efficiently transduce target cells with the desired genetic material.
- Types of Viral Vectors: Various groups of viruses have been studied extensively for their potential application as viral vectors, each offering unique advantages and characteristics.
- Adenoviral Vectors:
- Adenoviruses are known for their ability to infect a broad range of cell types, including both dividing and non-dividing cells.
- They have been widely utilized in gene therapy due to their high transduction efficiency and capacity to deliver large DNA inserts.
- While adenoviral vectors do not integrate into the host genome, they provide transient expression of therapeutic genes, making them suitable for applications requiring short-term gene activity.
- Retroviral Vectors:
- Derived from retroviruses, these vectors are capable of integrating therapeutic genes into the host genome, leading to stable and long-term gene expression.
- Retroviral vectors are particularly advantageous for applications in gene therapy aimed at treating genetic disorders or in the context of tissue engineering.
- They are effective in transducing dividing cells, which is essential for applications requiring sustained gene expression over time.
- Lentiviral Vectors:
- Lentiviral vectors, originating from the human immunodeficiency virus (HIV), can transduce both dividing and non-dividing cells, thus broadening their application range.
- They are especially useful for stable gene transfer, making them ideal candidates for research and therapeutic applications involving various cell types, including stem cells.
- Adeno-Associated Viral Vectors (AAV):
- AAVs are small viruses that can integrate into the host genome, allowing for long-term expression of the inserted gene.
- They exhibit low immunogenicity and a favorable safety profile, making them suitable for use in clinical gene therapy applications.
- Poxviral Vectors:
- Poxviruses, such as vaccinia virus, have been explored for their ability to carry large inserts of foreign DNA.
- These vectors can elicit strong immune responses, which may be beneficial in certain therapeutic contexts, such as cancer treatment or vaccine development.
- Adenoviral Vectors:
- Factors Influencing Vector Selection: The choice of a specific viral vector for a given application depends on several critical factors:
- Transgenic Expression Efficiency: The ability of the vector to effectively deliver and express the therapeutic gene in target cells.
- Ease of Production: The feasibility of producing large quantities of the viral vector for research or clinical use.
- Safety: The potential for adverse effects, including immunogenic responses or pathogenicity, which must be carefully evaluated.
- Stability: The vector’s ability to maintain its structure and function over time, particularly for long-term gene expression applications.
- Clinical Applications: Numerous clinical trials have been initiated to explore the utility of various viral vectors in therapeutic contexts. For example:
- Adenoviruses have been investigated for the delivery of tumor suppressor genes in cancer treatment, aiming to inhibit tumor growth and promote apoptosis in cancerous cells.
- Retroviruses are being studied for their potential in tissue repair and engineering, particularly in the context of hematopoietic stem cell therapies.
3. Shuttle vector
Shuttle vectors are important tools in molecular biology, designed to facilitate the transfer of genetic material between different host organisms. Their unique ability to replicate in both bacterial and mammalian cells allows researchers to manipulate genes in diverse experimental contexts.
- Definition and Structure: Shuttle vectors are DNA plasmids that possess origins of replication from two distinct host organisms. This design enables the vectors to “shuttle” between these hosts, making them versatile for various applications in genetic engineering and cloning.
- Hybrid Nature: These vectors are considered hybrid because they incorporate DNA sequences from both bacterial plasmids and mammalian viruses. This combination allows them to leverage the strengths of both types of genetic systems for efficient gene transfer.
- Functional Components: Shuttle vectors typically include three critical functional DNA sequences:
- Viral Replication Origin: This sequence enables replication of the vector within mammalian cells.
- Bacterial Replication Origin: This component allows the vector to replicate in bacterial cells, facilitating the cloning and amplification of the vector.
- Drug Resistance Gene: This gene provides a selection mechanism, allowing researchers to identify and isolate cells that have successfully incorporated the shuttle vector.
- Types of Shuttle Vectors: There are three primary types of shuttle vectors, each defined by its replication system:
- Transiently Replicating Shuttle Vectors: These vectors require recognition by large T antigen for replication in human cells. They are commonly used for short-term experiments, such as transient expression assays.
- Episomal Shuttle Vectors: Designed to establish stable cell lines, these vectors replicate as plasmid DNA containing the desired insert. They allow for long-term expression of genes, making them suitable for applications in gene therapy and protein production.
- Integrated Shuttle Vectors: Unlike the previous types, integrated shuttle vectors undergo replication only after fusing with specific cell types. This property allows for targeted gene expression, making them useful in contexts where precise control over gene activity is required.
- Applications in Research: The versatility of shuttle vectors has revolutionized genetic engineering by enabling:
- The study of gene function through the expression of specific genes in various host organisms.
- The development of gene therapies, where the goal is to treat genetic disorders by delivering corrective genes.
- The production of recombinant proteins, which are essential for research, pharmaceuticals, and therapeutic interventions.
- Examples of Shuttle Vectors:
- pUC18: A widely utilized shuttle vector that can replicate in Escherichia coli and other Gram-negative bacteria, commonly used for cloning purposes.
- pRS416: A shuttle vector specifically designed for use in Saccharomyces cerevisiae (baker’s yeast), facilitating the cloning and expression of genes in a eukaryotic system.
- pCMV6-AC: This shuttle vector is tailored for mammalian cell expression and contains a cytomegalovirus (CMV) promoter, which enables high-level gene expression.
4. Expression vector
Expression vectors are specialized tools in molecular biology designed to facilitate the expression of cloned genes in various host organisms. These vectors play a critical role in determining the success of cloning processes by enabling the production of specific proteins encoded by the inserted genes.
- Purpose and Function: Unlike standard cloning vectors, which primarily allow the insertion and maintenance of DNA, expression vectors are engineered to enable the transcription and translation of the cloned gene into a functional protein. This characteristic is essential for applications in research, biotechnology, and therapeutic development.
- Key Components: Expression vectors are meticulously designed with several essential elements that contribute to their function:
- Promoter Sequence: This regulatory region initiates transcription of the gene. It can be either constitutive, resulting in continuous expression, or inducible, requiring specific conditions (e.g., the presence of an inducer like IPTG) for activation.
- Transcription Termination Sequence: This sequence signals the end of transcription, ensuring proper processing of the mRNA.
- Translation Initiation Sequence: Often includes a ribosomal binding site and a start codon, facilitating the initiation of protein synthesis.
- Origin of Replication: This region allows the vector to replicate within the host cell.
- Selectable Marker: Typically a gene that confers resistance to antibiotics, enabling the identification of successfully transformed cells.
- Multiple Cloning Site (MCS): A region containing several unique restriction sites where the gene of interest can be inserted.
- Types of Expression Vectors: Depending on the host organism, expression vectors can vary in complexity:
- Prokaryotic Expression Vectors: Designed for use in bacteria such as Escherichia coli. They often include specific sequences like the Shine-Dalgarno sequence to facilitate ribosome binding during translation.
- Eukaryotic Expression Vectors: These vectors are tailored for expression in eukaryotic cells, incorporating sequences such as the Kozak consensus sequence to ensure proper translation in the eukaryotic machinery.
- Gene Transfer Process: The cloning of a gene into an expression vector typically involves:
- Insertion of the Gene: The gene of interest is inserted into the vector using restriction enzymes and ligases.
- Transformation: The expression vector is introduced into the host cells through methods such as heat shock or electroporation.
- Protein Production: Once inside the host, the expression vector uses the cell’s machinery to produce the encoded protein.
- Applications: Expression vectors are widely used in various fields, including:
- Protein Production: They enable the synthesis of proteins for research, therapeutic, or industrial purposes. For example, insulin production for diabetes treatment utilizes expression vectors.
- Functional Studies: Researchers can express proteins to study their functions and interactions within cellular environments.
- Therapeutic Development: Expression vectors are critical in gene therapy approaches, where therapeutic proteins are produced to treat diseases.
- Protein Tags and Purification: To facilitate the purification of expressed proteins, many expression vectors include protein tags, such as:
- His Tags: Allow for easy purification through nickel affinity chromatography.
- Fusion Proteins: Tags like glutathione S-transferase (GST) or maltose-binding protein (MBP) can enhance solubility and simplify the purification process. Reporter genes, such as green fluorescent protein (GFP), can also be incorporated to visualize protein expression.
- Examples of Expression Vectors:
- pET Vectors: Commonly used for protein expression in E. coli, featuring inducible promoters and fusion tags for purification.
- pDEST Vectors: Designed for Gateway cloning, these vectors can express proteins in multiple systems, including bacteria, yeast, and mammalian cells.
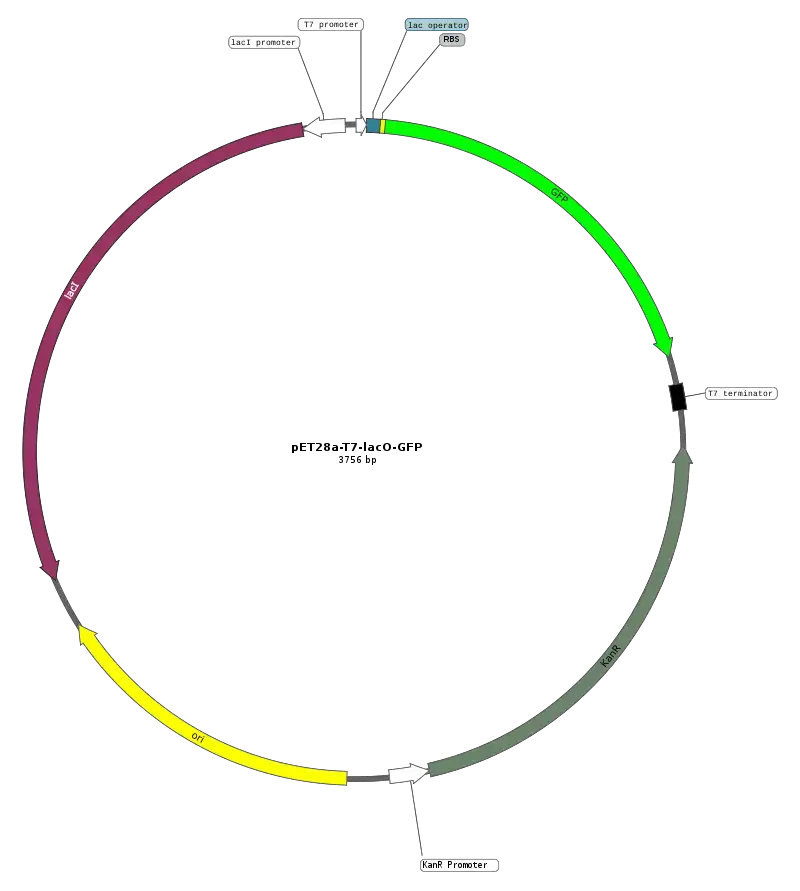
5. Secretion vector
Secretion vectors are specialized types of expression vectors specifically engineered to facilitate the production and transport of proteins outside the cytoplasm of host cells. This capability allows for the effective secretion of proteins into the extracellular environment, enhancing their yield and simplifying subsequent purification processes.
- Functionality: Secretion vectors function by fusing the gene of interest with a nucleotide sequence that encodes a signal peptide or other secretion signals. This modification directs the newly synthesized protein toward the secretory pathway, ensuring its transport out of the cell.
- Advantages of Secretion Vectors:
- Increased Protein Yield: By enabling the secretion of proteins, these vectors often yield higher quantities compared to conventional cytoplasmic expression systems. This advantage is particularly significant in large-scale industrial applications where high protein output is crucial.
- Simplified Purification: Since the target proteins are secreted into the culture medium, they can be purified directly from the extracellular space. This approach avoids the complexities associated with extracting proteins from the cellular interior, which may contain numerous contaminants.
- Enhanced Protein Stability: The extracellular environment typically offers conditions that are more conducive to proper protein folding and stability, which reduces the likelihood of aggregation or degradation. This stability often results in higher-quality proteins that maintain their functionality.
- Design Flexibility: Secretion vectors can be tailored for use in various host systems, including both prokaryotic and eukaryotic cells. This versatility allows researchers to optimize expression systems according to specific experimental needs.
- Addressing Challenges with Eukaryotic Proteins: A common issue in expressing eukaryotic proteins within prokaryotic systems is the formation of inclusion bodies—aggregates of misfolded proteins. Secretion vectors mitigate this problem by directing proteins for secretion, thereby minimizing the risk of aggregation and increasing the chances of obtaining soluble, functional proteins.
- Applications: Secretion vectors have become critical tools in biotechnology, particularly in the context of producing recombinant proteins for a variety of applications, including:
- Biopharmaceuticals: They are used to produce therapeutic proteins and antibodies.
- Industrial Enzymes: Secretion vectors facilitate the production of enzymes used in various industrial processes.
- Research Tools: These vectors are employed to study protein functions and interactions in a more natural context.
- Examples of Secretion Vectors:
- pPICZ: Utilized in the yeast Pichia pastoris system, this vector is designed for the production and secretion of recombinant proteins, capitalizing on the yeast’s robust secretory pathway.
- pSecTag: A mammalian secretion vector that enables the efficient secretion of recombinant proteins into the culture medium, making it suitable for mammalian cell systems.
- pBAD/His: This vector allows for the inducible expression and secretion of recombinant proteins in Escherichia coli using an arabinose promoter, providing flexibility in protein production.
Examples of Vectors
Vectors are essential tools in genetic engineering, serving as vehicles to introduce foreign DNA into host cells for various applications in molecular biology. This section highlights several commonly used vectors, outlining their structures, functions, and specific applications in research and biotechnology.
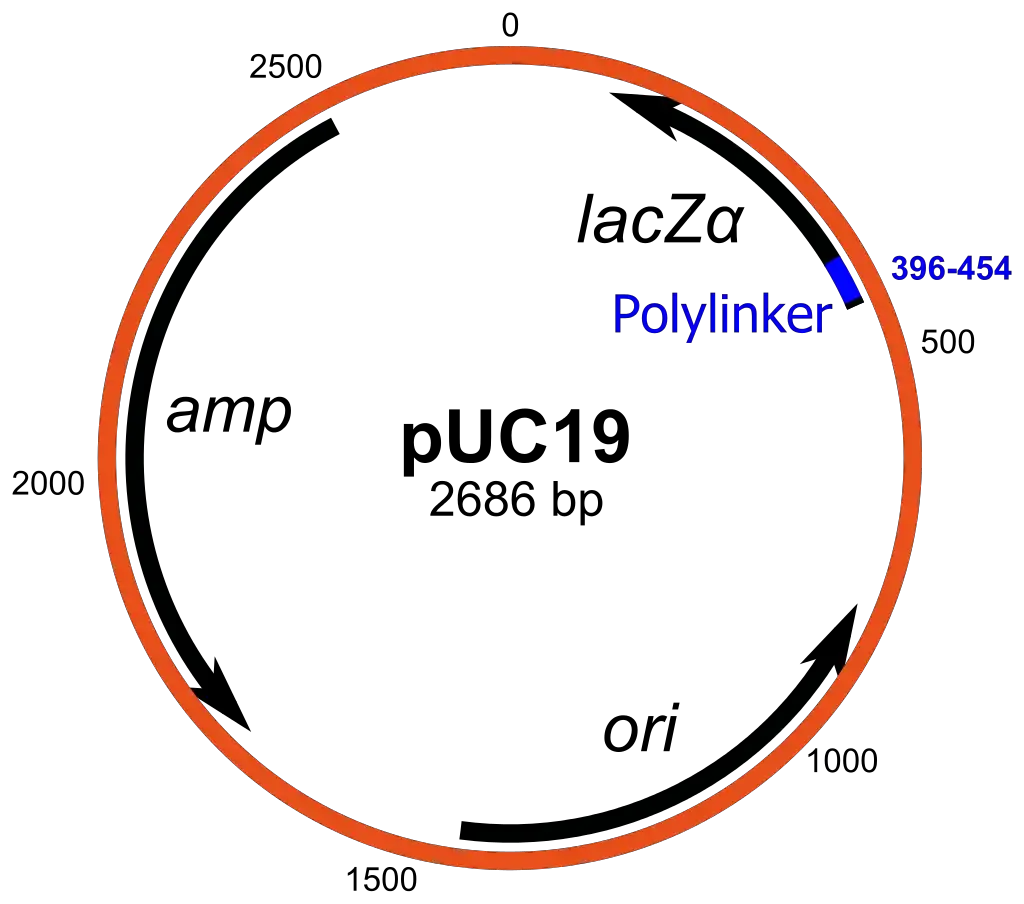
- pBR322:
- pBR322 is a widely recognized plasmid cloning vector primarily utilized in Escherichia coli (E. coli) and similar prokaryotes.
- The vector comprises an origin of replication derived from a ColE1-like plasmid (pMB1), an ampicillin resistance gene (ApR) from transposon Tn3, and a tetracycline resistance gene (TcR) from pSC101.
- Its design minimizes unnecessary DNA sequences while maximizing restriction enzyme cleavage sites, incorporating a total of twenty-one unique sites, with eleven located within the antibiotic resistance genes.
- A distinctive EcoRI cleavage site enhances its efficiency for cloning applications.
- While pBR322 has proven effective for decades, it has limitations, such as potential loss during continuous culture without selective pressure, which can hinder large-scale fermentation processes.
- pUC19:
- pUC19 is another prominent plasmid cloning vector, constructed at the University of California, hence the name “pUC.”
- The vector consists of a circular double-stranded DNA structure with 2,686 base pairs, featuring a high copy number due to the absence of the rop gene and specific mutations in its origin of replication.
- A notable characteristic of pUC19 is its multiple cloning site (MCS), which contains a 54 base-pair region that allows the insertion of foreign DNA at various hexanucleotide-specific restriction endonuclease sites.
- The presence of the N-terminal fragment of the β-galactosidase gene (lacZ) serves as a selective marker, enabling the differentiation of recombinant from non-recombinant cells through colony color screening on media containing IPTG and X-gal. Recombinant cells appear white, while non-recombinant cells display blue colonies due to the hydrolysis of X-gal.
- This vector facilitates efficient transformation into bacterial cells, allowing for replication and expression of inserted DNA fragments.
- λ Phage:
- λ-phage is a bacteriophage specifically designed to infect E. coli, offering distinct advantages over plasmid vectors due to its efficiency in entering bacterial cells and integrating recombinant DNA into the host genome.
- This double-stranded DNA bacteriophage includes an origin of replication and various DNA sequences that encode proteins essential for replication and regulation.
- The replication of λ-phage employs both theta and rolling circle mechanisms, producing a linear double-stranded DNA molecule, followed by the circularization facilitated by the cos sequence post-infection.
- The vector contains non-essential DNA sequences between its two arms, which can be replaced with recombinant DNA during cloning, enabling the efficient delivery of genetic material into the bacterial genome.
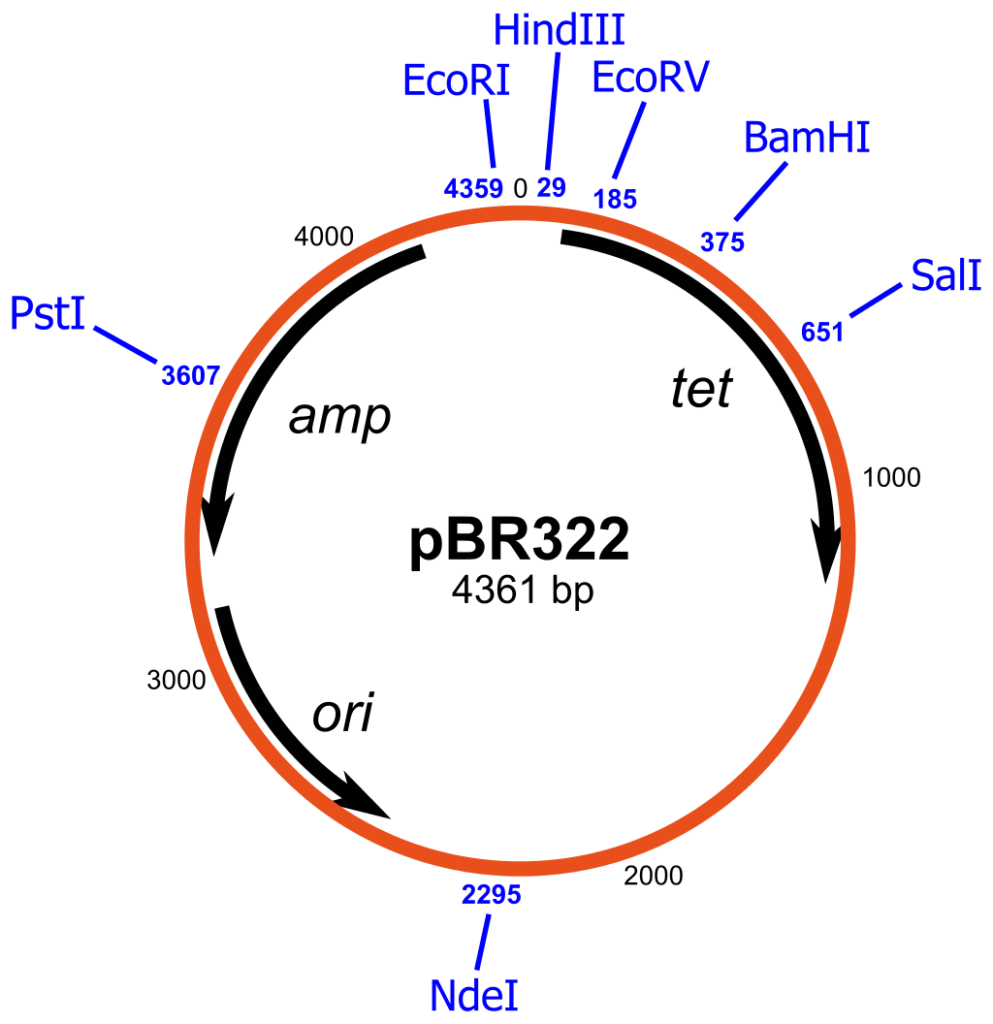
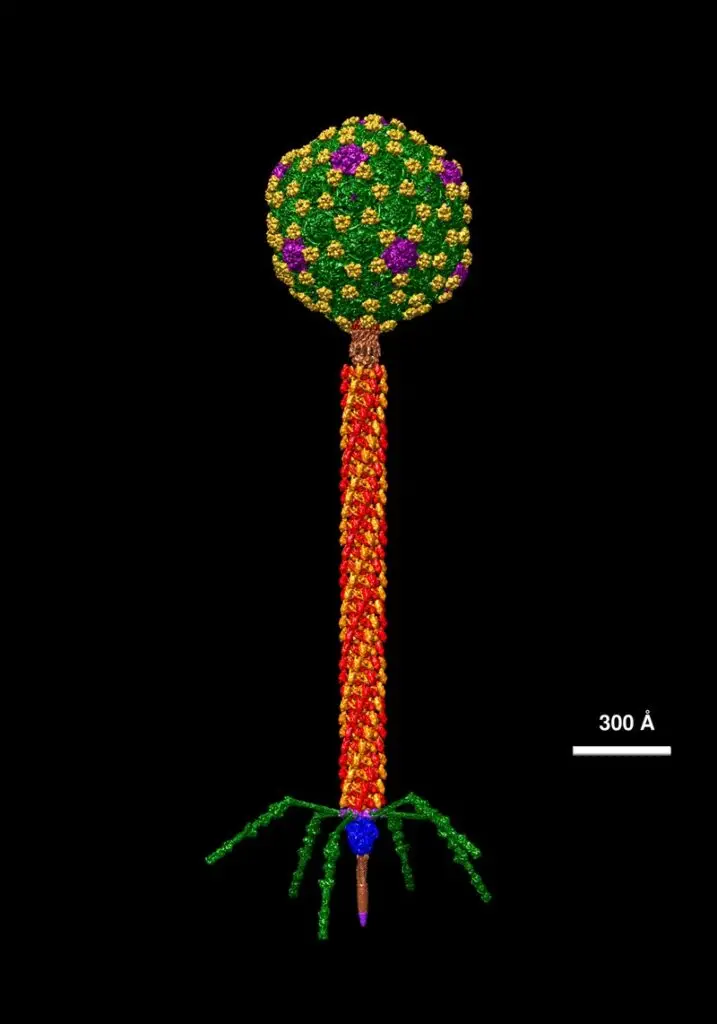
Features of Vectors
Characteristics of vectors play a crucial role in molecular biology and genetic engineering processes. These features ensure the efficient replication, expression, and manipulation of genetic material. Here are some important characteristics of vectors:
- Origin of replication: Vectors should possess an origin of replication, allowing them to replicate autonomously within host cells. This feature ensures that the vector can be propagated and maintained in the host cell.
- Promoter: Vectors used for gene expression require a promoter sequence. Promoters drive the transcription of the transgene and other genes within the vector. This enables the production of the desired protein or RNA molecule.
- Cloning site: Vectors contain a cloning site, also known as a multiple cloning site or polylinker. This region contains multiple unique restriction enzyme recognition sites, allowing for the insertion of foreign DNA into the vector through ligation. The cloning site is essential for incorporating the desired DNA fragment into the vector.
- Genetic markers: Vectors often carry genetic markers that facilitate the identification and selection of host cells containing the vector. Common markers include antibiotic resistance genes, which allow for the survival and growth of cells that have taken up the vector in the presence of specific antibiotics.
- Epitope tags: Some vectors may include specific epitope tags that can be fused to the expressed protein. These tags enable the identification and purification of the protein using antibodies against the epitope. Examples of epitope tags include polyhistidine-tag, glutathione-S-transferase (GST) tag, and maltose binding protein (MBP) tag.
- Reporter genes: Vectors may incorporate reporter genes that produce easily detectable proteins or enzymes, indicating the successful insertion of the desired DNA fragment. Common reporter genes include lacZ-α, which generates a fragment of β-galactosidase that can be detected using a colorimetric assay, and genes encoding green fluorescent protein (GFP) or luciferase, which emit light for visualization.
- Targeting sequences: Expression vectors may include targeting sequences that direct the expressed protein to specific organelles or cellular locations. These sequences ensure proper localization and function of the protein within the cell.
- Protein purification tags: Some vectors contain tags or fusion partners that facilitate the purification of the expressed protein. These tags, such as polyhistidine-tag or GST tag, can be used for affinity chromatography purification, simplifying the downstream purification process.
- Integration capability: Certain vectors, such as viral vectors, have the ability to integrate the transgene into the host genome. This feature allows for stable and long-term expression of the inserted DNA.
- Size and isolation: Vectors should have an optimal size for efficient delivery and incorporation into host cells. Additionally, vectors should be easy to isolate and purify for subsequent experiments.
Applications of Vectors
Vectors play a crucial role in various applications within molecular biology and genetic engineering, thanks to their simplicity, cost-effectiveness, and efficiency. These applications leverage the versatility of vectors to facilitate a range of processes and studies. Here are some significant applications of vectors in the field:
- Cloning: Vectors, particularly cloning vectors, are extensively used to transfer foreign DNA into host cells. This process allows for the replication and expression of the inserted DNA, enabling the production of specific proteins or the study of gene function.
- Genetic Engineering: Vectors are instrumental in engineering organisms for specific functions. For instance, they can be used to modify E. coli bacteria to produce insulin, a critical hormone used in diabetes treatment.
- Gene Isolation and Sequencing: Vectors aid in isolating specific gene sequences within a genome, enabling their subsequent analysis and determination of nucleotide sequences through DNA sequencing techniques.
- Study of Control and Regulatory Sequences: Vectors help researchers study and analyze control sequences and regulatory elements within genomes. These sequences govern gene expression and play crucial roles in cellular processes.
- Protein Structure and Function Studies: Cloning vectors are utilized to investigate the structure, function, and production of proteins in various organisms. This research provides valuable insights into protein properties, interactions, and potential applications.
- Phage Therapy: Bacteriophage vectors are employed in phage therapy, a promising treatment approach for bacterial infections in humans and animals. Phages specifically target and infect bacteria, offering a potential alternative to conventional antibiotics.
- Mutation Identification and Disease Diagnosis: Vectors can be utilized to identify mutations within DNA sequences, aiding in the diagnosis of gene defects associated with specific diseases. This application plays a crucial role in genetic screening and personalized medicine.
- Clinical Microbiology: Recombinant DNA technology, facilitated by vectors, has been instrumental in clinical microbiology. It has enabled the development of recombinant antigens, vaccines, and diagnostic probes for screening diseases such as HIV, HCV (Hepatitis C virus), and CMV (Cytomegalovirus).
Advantages of Vectors
Vectors offer several advantages in various fields of molecular biology and genetic engineering. Here are some key advantages of using vectors:
- Efficient DNA Transfer: Vectors serve as vehicles for the transfer of foreign DNA into host cells. They facilitate the efficient introduction and incorporation of the desired DNA sequences into the host genome, allowing for the expression and study of the inserted genes.
- Replication and Amplification: Vectors often contain origins of replication that enable them to replicate within host cells. This feature allows for the production of multiple copies of the vector and the inserted DNA, increasing the yield of the desired DNA sequences.
- Selectable Markers: Vectors frequently include selectable markers, such as antibiotic resistance genes, which enable the identification and selection of host cells that have successfully taken up the vector. This ensures that only cells containing the desired DNA are selected and cultured, simplifying the isolation and purification of the target DNA.
- Versatility: Vectors come in various types, each designed for specific applications. This versatility allows researchers to choose the most suitable vector for their specific experimental needs, whether it’s for cloning, protein expression, gene editing, or other purposes.
- Molecular Tags and Fusion Proteins: Vectors can be engineered to include molecular tags or fusion protein tags. These tags facilitate the detection, purification, and localization of the expressed proteins, aiding in their study and characterization.
- Modular Design: Many vectors have modular structures with multiple cloning sites, also known as polylinkers or multiple cloning regions. These regions contain multiple restriction enzyme recognition sites, allowing for easy insertion of the desired DNA sequences. This flexibility and modularity simplify the cloning process and enable the rapid generation of recombinant DNA constructs.
- Genetic Manipulation: Vectors provide a means for genetic manipulation, such as gene knockout, gene silencing, or gene overexpression. They enable researchers to introduce specific genetic modifications into host cells or organisms, allowing the study of gene function and the investigation of molecular mechanisms.
- Compatibility with Various Hosts: Vectors can be designed to be compatible with different host organisms, including bacteria, yeast, plants, and mammalian cells. This adaptability enables researchers to work with a wide range of organisms and systems, expanding the scope of their experiments and applications.
- Standardization and Well-Characterized Vectors: Over time, certain vectors have become widely adopted and well-characterized within the scientific community. This standardization facilitates reproducibility and enables researchers to build upon existing knowledge and methodologies.
Limitations of Vectors
While vectors have revolutionized molecular biology and genetic engineering, they do have certain limitations that researchers need to consider. Here are some key limitations associated with the use of vectors:
- Stability: Vectors may exhibit instability within host cells due to various factors, such as changes in metabolic energy, pH, and temperature. Different host genotypes can also impact vector stability. These factors can lead to the loss or rearrangement of the inserted DNA sequences, affecting the reliability and reproducibility of experiments.
- Gene Overexpression: The use of vectors for gene expression can sometimes result in the overexpression of the target gene in the host cell. This excessive expression can have adverse effects, such as cellular toxicity, altered cellular physiology, or misregulation of endogenous genes. Careful regulation and control of gene expression levels are necessary to avoid such issues.
- Complexity and Compatibility: For certain applications, a single type of vector may not be sufficient to achieve the desired outcome. Researchers may need to use multiple vectors, each with different characteristics or functionalities. This can introduce complexities and challenges during experimental design, vector construction, and analysis.
- Time and Cost: Developing more efficient vectors or optimizing existing ones requires significant time, effort, and resources. Research in vector design and improvement involves extensive experimentation, testing, and validation. Additionally, the production and purification of vectors can be costly, particularly for large-scale applications.
- Host-Specific Limitations: Some vectors are designed for specific host organisms, such as bacteria, yeast, plants, or mammalian cells. Each host system may have its own limitations, including differences in gene expression machinery, post-translational modifications, or immune responses. Researchers must carefully select the appropriate vector-host system to ensure compatibility and maximize the success of their experiments.
- Insert Size Limitations: Vectors have a limited capacity to accommodate large DNA inserts. The size of the insert that can be effectively cloned and maintained within a vector can vary depending on the vector type and the host organism. Large or complex DNA sequences may exceed the capacity of certain vectors, necessitating the use of alternative cloning strategies.
- Cell-Type Specificity: Some vectors may exhibit preferential efficiency or compatibility with specific cell types or tissues. This can restrict their applicability in certain experimental systems or limit the generalizability of findings across different cell types or organisms.
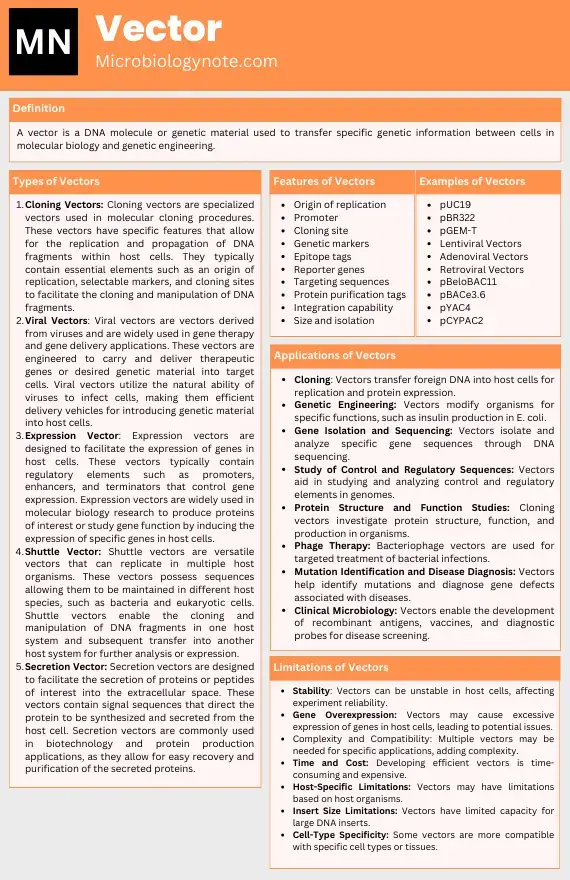
FAQ
What is a vector in molecular biology?
A vector in molecular biology refers to a DNA molecule used to carry and transfer foreign DNA sequences into host cells. It acts as a vehicle for the replication and expression of the inserted DNA.
What are the types of vectors commonly used in molecular biology?
Commonly used vectors include plasmids, viral vectors (e.g., retroviruses, adenoviruses), and artificial chromosomes (e.g., yeast artificial chromosomes, bacterial artificial chromosomes).
What is the purpose of using vectors in molecular biology?
Vectors are used to introduce foreign DNA into host cells for various purposes, such as gene cloning, gene expression, protein production, and genetic engineering.
How are vectors constructed?
Vectors are constructed by incorporating specific DNA elements, such as an origin of replication, promoter, cloning sites, and selectable markers, into a backbone DNA molecule. These elements facilitate replication, gene expression, and selection of transformed cells.
What is the role of selectable markers in vectors?
Selectable markers, often antibiotic resistance genes, allow for the identification and selection of host cells that have taken up the vector. Cells without the vector or those with failed transformations are eliminated through antibiotic selection.
How are vectors introduced into host cells?
Vectors can be introduced into host cells through various methods, including transformation (for bacteria), transfection (for mammalian cells), viral transduction (using viral vectors), or physical methods like electroporation or microinjection.
Can vectors integrate into the host cell’s genome?
Yes, some vectors, particularly viral vectors like retroviruses, have the ability to integrate into the host cell’s genome. This integration allows long-term expression of the inserted DNA in the host cell and its progeny.
What is the importance of multiple cloning sites (MCS) in vectors?
Multiple cloning sites, also known as polylinkers, are regions within a vector that contain multiple unique restriction enzyme recognition sites. These sites allow for the insertion of DNA fragments at specific locations, making it easier to clone different genes or DNA sequences into the vector.
Can vectors be used for gene therapy?
Yes, vectors, especially viral vectors, have been extensively used in gene therapy to deliver therapeutic genes into target cells to treat genetic disorders, cancer, and other diseases.
Are there any safety considerations when working with vectors?
Yes, safety precautions must be followed when working with vectors, particularly those derived from pathogenic sources or containing potentially harmful genes. Adherence to biosafety guidelines and proper containment measures is crucial to minimize the risk of accidental release or exposure.
- Gibson, D. G. (2011). Enzymatic assembly of overlapping DNA fragments. Methods in enzymology, 498, 349-361.
- Sambrook, J., Fritsch, E. F., & Maniatis, T. (1989). Molecular cloning: a laboratory manual (2nd ed.). Cold Spring Harbor Laboratory Press.
- Galloway, D. R. (2014). Introduction to cloning vectors. In Methods in molecular biology (Vol. 1116, pp. 1-12). Humana Press.
- Botstein, D., & Shortle, D. (1985). Strategies and applications of in vitro mutagenesis. Science, 229(4719), 1193-1201.
- Wirth, D., & Gama-Norton, L. (2016). Genome integration and gene targeting by homologous recombination. In Gene editing (pp. 37-61). Humana Press.
- Zinder, N. D., & Lederberg, J. (1952). Genetic exchange in Salmonella. Journal of bacteriology, 64(5), 679-699.
- Brantl, S. (2014). Plasmid replication control: replication initiator complexes and beyond. Nucleic acids research, 42(7), 3884-3893.
- Ellis, H. M., Yu, D., DiTizio, T., & Court, D. L. (2001). High efficiency mutagenesis, repair, and engineering of chromosomal DNA using single-stranded oligonucleotides. Proceedings of the National Academy of Sciences, 98(12), 6742-6746.
- Muzyczka, N. (1992). Use of adeno-associated virus as a general transduction vector for mammalian cells. Current topics in microbiology and immunology, 158, 97-129.
- Weber, W., & Fussenegger, M. (2006). Molecular diversity—the toolbox for synthetic gene switches and networks. Current opinion in chemical biology, 10(6), 638-646.
- https://www.genome.gov/genetics-glossary/Vector#:~:text=A%20vector%2C%20as%20related%20to,cloning%20or%20recombinant%20DNA%20technique.
- https://byjus.com/biology/cloning-vector/
- https://kvmwai.edu.in/upload/StudyMaterial/clonning_vectors.pdf
- https://www.ncbi.nlm.nih.gov/pmc/articles/PMC6302727/
- https://www.excedr.com/resources/genetic-vectors
- https://www.ladykeanecollege.edu.in/files/userfiles/file/VECTORS%20IN%20GENETIC%20ENGINEERINg.pdf
- https://en.wikipedia.org/wiki/Vector_(molecular_biology)
- https://www.nature.com/articles/s41417-021-00331-7
- https://www.slideshare.net/slideshow/vectors-in-biotechnolgy/45007522