What is a Spectrophotometer?
- An analytical tool used to find the composition and concentration of a sample by measuring its absorbed light intensity over a spectrum of wavelengths is a spectrophotometer.
- It works by passing light from a steady source through a cuvette, then using a monochromator to distribute the light thereby separating particular wavelengths before detection.
- The observed absorbance is correlated with the concentration of chemical species in the sample using ideas of absorption spectroscopy and the Beer–Lambert law.
- Early models were produced in the 1930s and 1940s thanks in large part to Arnold Beckman and his research team, producing the generally known Beckman DU spectrophotometer.
- Important parts are a sample holder, a dispersing element (prism or diffraction grating), a light source—such as tungsten or deuterium lamps—that turns light intensity into an electrical signal.
- Essential in many disciplines including chemistry, biochemistry, environmental research, and clinical diagnostics, spectrophotometers provide quantitative examination of enzymes, nucleic acids, and other molecules.
- From single-beam devices to more sophisticated double-beam instruments that concurrently measure reference and sample signals, the design has progressed in complexity and precision.
- Modern spectrophotometers may use diode arrays to quickly scan several wavelengths, therefore enhancing throughput and repeatability in applications including research and quality control. They also include computer-controlled data acquisition.
Definition of Spectrophotometer
A spectrophotometer is a laboratory instrument that measures the amount of light absorbed by a sample and is used to determine properties such as concentration or absorption patterns of substances.
Principle of Spectrophotometer
- The Beer-Lambert law describes how a spectrophotometer works: the intensity of light absorbed is exactly proportional to both the concentration of the absorbing species and the path length across the sample when a monochromatic light beam passes through a medium.
- The device generates a continuous spectrum from a steady light source—such as a tungsten or deuterium lamp—then filters or distributes it using a monochromator to extract particular wavelengths for use in analysis.
- The chosen light is focused through a cuvette containing a sample where molecules absorb specific wavelengths depending on their individual electronic transitions, therefore producing a spectral “fingerprint” for the substance.
- Measuring the light intensity passing through the sample, a photodetector transforms the unabsorbed light into an electrical signal commensurate with it.
- Through a logarithmic function, the instrument computes the transmittance by comparing the incident light intensity with the transmitted intensity, so directly relates to the concentration of the analyte by the Beer-Lambert equation.
- Whereas in a double-beam design simultaneous measurements across a reference and a sample allow for correction of source variations and increased accuracy, in a single-beam configuration the instrument measures the light before and after the sample sequentially.
- Usually in the ultraviolet, visible, and near-infrared ranges, modern spectrophotometers combine digital processing and calibration methods to provide great repeatability and exact quantitative analysis over several wavelengths.
- In several scientific disciplines, including chemistry, biochemistry, environmental science, and clinical diagnostics, the basic operational concept helps quick, non-destructive analysis to be conducted.
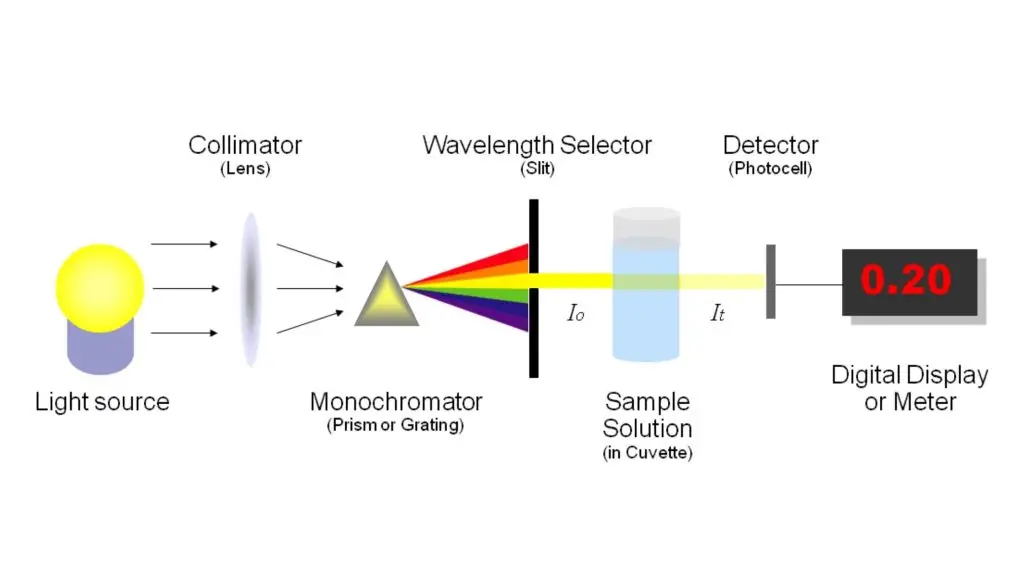
Types of Spectrophotometer
Spectrophotometers are analytical devices for measuring, at designated wavelengths, the intensity of light absorbed or transferred by a sample. Their operating concepts and the areas of the electromagnetic spectrum they study help to classify them. There are mostly two kinds:
- UV-Visible (UV-Vis) Spectrophotometers– Measurement of light absorption in the ultraviolet (185–400 nm) and visible (400–700 nm) ranges using UV-Visible (UV-Vis) Spectrophotometers In analytical chemistry and biology, they are extensively applied in order to calculate concentrations in solutions and quantify colored substances.
- Infrared (IR) Spectrophotometers – Often used in the identification of chemical bonds and functional groups in organic compounds, infrared (IR) spectrophotometers examine light absorption in the infrared range (higher than 760 nm.). They may examine samples in several states—gas, liquid, and solid among others.
- Atomic Absorption Spectrophotometers (AAS) – Atomic Absorption Spectrophotometers (AAS) find the amounts of metals and metalloids in samples by means of the absorption of light by vaporized atoms. In disciplines including clinical diagnosis and environmental analysis, they are absolutely vital.
- Fluorescence Spectrophotometers– Fluorescent illumination Fluorescent spectrophotometers track the intensity of fluorescent light produced from excitable substances. They enable extremely sensitive sample fluorescence either naturally occurring or generated.
- Colorimeters – Simpler types of spectrophotometers, colorimeters are used in colorimetric assays and tests to quantify light absorption, therefore guaranteeing color constancy in many materials like plastics, paper, liquids, metals, and fabrics.
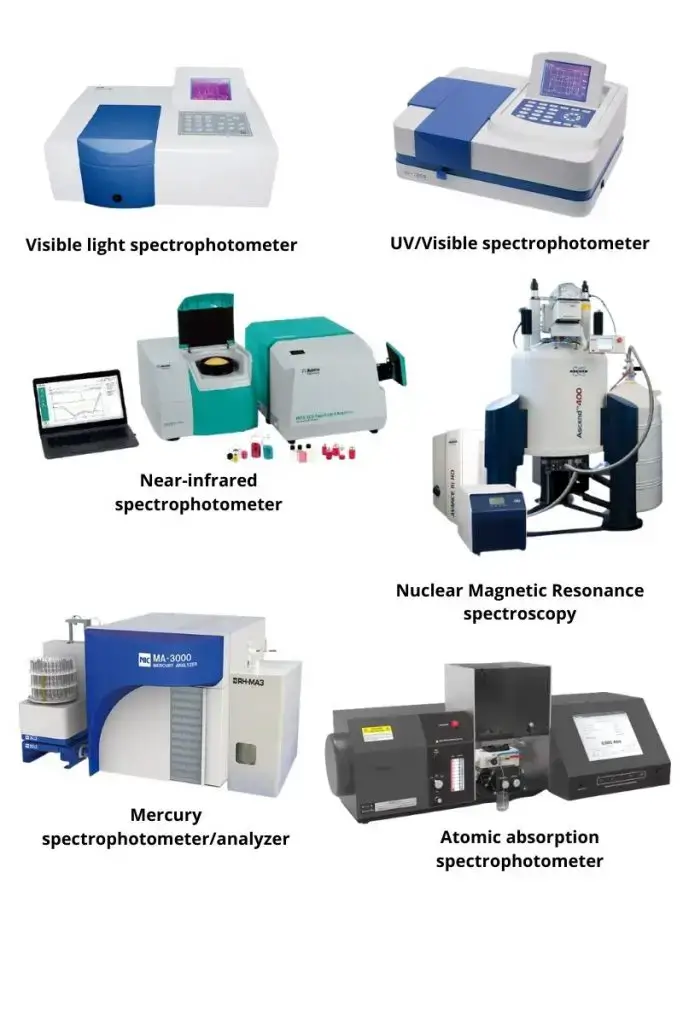
Instrumentation of Spectrophotometer/Parts of Spectrophotometer
Light Source: Provides the initial radiation necessary for measurement. Common sources include tungsten lamps for visible light and deuterium lamps for ultraviolet light.
Collimator: Transforms divergent light from the source into a parallel beam, ensuring consistent illumination of the monochromator.
Monochromator: Separates the light into its component wavelengths using prisms or diffraction gratings, allowing selection of a specific wavelength for analysis.
Wavelength Selector (Slit): Isolates the desired wavelength from the monochromator output, enhancing the instrument’s spectral resolution.
Sample Holder (Cuvette): Holds the sample solution. Typically made from materials like quartz or glass, depending on the wavelength range of interest.
Detector: Measures the intensity of transmitted or absorbed light, converting it into an electrical signal. Common detectors include photomultiplier tubes and photodiodes.
Display System: Processes the detector’s electrical signal to provide a readable output, often as absorbance or transmittance values.
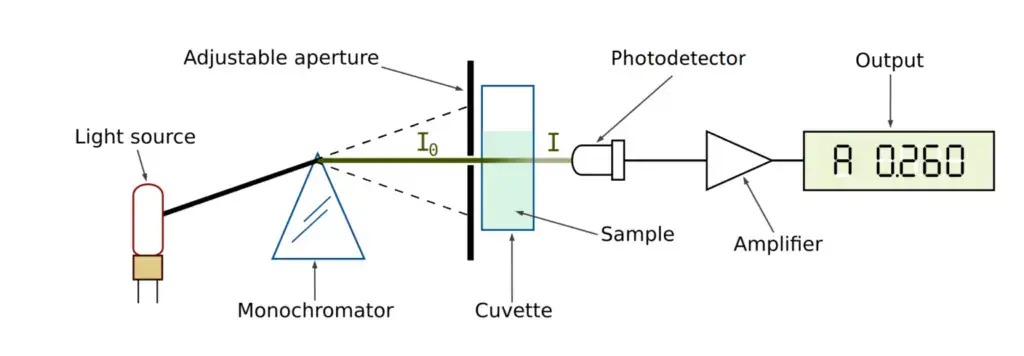
Optical system (monochromator)
The spectrophotometer’s optical system, known as the monochromator, is integral to the precise analysis and manipulation of light. This system is meticulously designed to select and isolate specific wavelengths for accurate measurement. Below is an in-depth exploration of the monochromator’s components and their respective roles:
Component | Function and Description |
---|---|
Lenses | These components gather the radiation from the light source and channel it towards the entrance slit. Their primary role is to focus and align the light accurately. |
Entrance Slit | Serving as a spatial filter, the entrance slit narrows down the image of the collected radiation, permitting only a specific segment of light to progress. |
Collimator Lens | Positioned post the entrance slit, this lens converts the incoming light into a parallel beam. Ensuring that all light rays are parallel is pivotal for subsequent dispersion and analysis. |
Exit Slit | Situated after the dispersive element, the exit slit acts as a wavelength selector. It restricts the passage of light, allowing only a designated spectrum to proceed for detection. |
Dispersive Device | This is the heart of the optical system, tasked with decomposing the incident light into its foundational wavelengths. The two predominant types of dispersive devices are prisms and diffraction gratings. |
Prism | Operating on the principle of refraction, prisms segregate polychromatic light by refracting distinct wavelengths differently. For spectra below 350 nm in the ultraviolet range, prisms made of quartz or fused silica are typically employed. |
Diffraction Grating | Comprising numerous closely spaced parallel lines on a polished surface, usually aluminum, diffraction gratings disperse light by diffracting it into its constituent wavelengths. The grating’s dispersion capability is determined by its ruling density, with higher densities (e.g., 3600 grooves/mm or more) being favored for visible and ultraviolet wavelengths. Gratings are often regarded as having superior dispersion properties compared to prisms. |
How does a spectrophotometer work? – Working Mechanism of Spectrophotometer
- Usually covering visible and UV areas using tungsten or deuterium lamps, the light source produces a wide spectrum of wavelengths.
- The collimator guarantees homogeneous illumination by turning the divergent light from the source into a parallel beam.
- By means of prisms or diffraction gratings, the monochromator divides the light into its component wavelengths and chooses the required wavelength.
- Often a small slit, the wavelength selector further separates the particular wavelength for exact measurement.
- The sample kept in a cuvette passes the collimated light through where portion of the molecular structure absorbs it.
- The detector transforms the transmitted or absorbed light into an electrical signal thereby measuring its intensity.
- The electronics of the instrument process and calibrate the electrical signal so transforming it into an absorbance or transmittance value.
- The last data is shown on a computer interface that lets quantitative analysis and interpretation be possible.
Operating Procedure of spectrophotometer
- Turn on the spectrophotometer and let it warm up till the light source steadies.
- Prepare and clean the cuvettes such that they are free of fingerprints and residue.
- Usually the solvent used in the experiment, a cuvette should be filled with a blank solution.
- Using the monochromator, change the wavelength settings and slide the blank cuvette into the device.
- Use the blank sample to set the baseline absorbance to zero, so calibrating the instrument.
- Eliminate the blank and replace it with the sample solution to guarantee few air bubbles in a new cuvette.
- Record the absorbance or transmittance value by setting the sample cuvette in the sample chamber.
- As required to confirm the accuracy and repeatability of the findings, do repeat observations.
- Track the gathered information and do any necessary computations or analysis.
- Following normal laboratory techniques, clean the cuvettes and turn off the equipment.

Absorbance Wavelengths
- Calculated as A = –log₁₀(I/I₀), absorbance measures the proportion of incoming light absorbed by a sample and is computed from I to I₀.
- By means of absorbance at particular wavelengths, one can get understanding of the electronic transitions of molecules, usually from the highest occupied molecular orbital (HOMO) to the lowest unoccupied molecular orbital (LUMO).
- Although specific ranges may vary depending on instrument design, in ultraviolet–visible (UV-Vis) spectroscopy the UV region typically spans 200–400 nm and the visible region covers around 400–700 nm.
- Chromophores are particular molecular substructures in charge of light absorption; their absorption wavelengths depend on the kind of electronic transitions, such π→π* and n→π* transitions.
- Usually, increased conjugation inside a molecule lowers the energy gap between HOMO and LUMO, therefore extending the absorption maximum (λₐₓ) to longer wavelengths—a phenomena known as a bathochromic shift.
- By considering elements such the amount of double bonds, substituent effects, and solvent influences, empirical rules—like the Woodward–Fieser rules—allow one to estimate absorbance maxima in conjugated systems.
- By linking absorbance to concentration, the Beer-Lambert law—expressed as A = εcl—with ε as the molar absorptivity, c the concentration, and l the route length—underpins quantitative analysis.
- Different materials have different absorption wavelengths; for instance, DNA usually shows maximal absorbance close to 260 nm, whereas aromatic amino acids in proteins absorb almost 280 nm.
- By changing the electronic environment of chromophores, solvent polarity, pH, and temperature can modify the absorption spectra, therefore influencing the λₘₐₓ and the absorption intensity.
- To show the λₘₐₓ of the analyte, modern UV-Vis spectrophotometers scan across a variety of wavelengths to produce an absorption spectrum displaying absorbance as a function of wavelength.
- Key metric determining how strongly a substance absorbs light at a given wavelength is molar absorptivity, sometimes known as extinction coefficient, which helps one to estimate analyte concentrations in solution.
- Apart from helping to identify substances and evaluate purity, the thorough investigation of absorbance wavelengths assists kinetic research and structural change evaluation in chemical and biological samples.
Single vs Double Beam Spectrophotometers
Feature | Single Beam Spectrophotometer | Double Beam Spectrophotometer |
---|---|---|
Design and Light Path | Uses a single light path where light passes through the sample. Calibration requires manual measurement of a reference. | Splits light into two paths — one for the sample and one for the reference — allowing simultaneous comparison. |
Accuracy and Precision | More prone to fluctuations in light source intensity, affecting measurement accuracy. | Compensates for variations by comparing sample and reference beams, providing higher accuracy and precision. |
Stability and Baseline Drift | More susceptible to baseline drift, requiring frequent recalibration. | More stable due to continuous sample-reference comparison, reducing baseline drift. |
Speed and Efficiency | Faster measurements but requires manual switching between sample and reference. | Simultaneously measures sample and reference, improving efficiency, especially for high-volume work. |
Cost and Complexity | Simpler design and more affordable — ideal for routine or educational use. | More complex and expensive — suitable for research requiring advanced features. |
Applications | Suitable for routine analysis, teaching labs, and basic quality control. | Ideal for research, quantitative analysis, and kinetic studies requiring high precision. |
Advantages of Spectrophotometer
- Rapid, non-destructive examination made possible by spectrophotometers helps to preserve samples for next tests or additional study.
- Simplifying quantitative calculations, the method depends on the Beer-Lambert law to offer a direct, linear relationship between absorbance and analyte concentration.
- Usually requiring little sample preparation, instruments let aqueous solutions be effectively processed and help to simplify procedures.
- Operating throughout a wide spectrum of wavelengths—from UV to visible and near-infrared—they are flexible to suit different kinds of molecules.
- Appropriate calibration and equipment settings will help to obtain low detection limits and high sensitivity, thereby enabling exact measurements.
- Routine laboratory use as well as educational environments might benefit from the rather affordable and sturdy equipment.
- By tracking dynamic changes in real time and reaction kinetics, spectrophotometers offer important new perspectives on chemical and biological processes.
- Reliable comparisons between studies and measurement repeatability are enhanced by standardized calibration with known benchmarks.
Limitations of Spectrophotometer
- Spectrophotometers limit analysis to chromophoric or derivatized analytes by not being able to measure compounds that do not absorb in the UV–visible range.
- Stray light limits instrument precision and can cause measurement accuracy to be affected as well as absorbance at high optical densities to be underestimated.
- A non-monochromatic probe beam can smooth or shift spectral characteristics, hence deviating from perfect Beer-Lambert behavior; spectral bandwidth and wavelength accuracy are inherent limits.
- While low-concentration measurements might be affected by noise and detector limits, low sensitivity at high absorbance levels drives sample dilution.
- Interferences including fluorescence, overlapping absorption bands from several species, and light scattering can cause artifacts and lower quantitative accuracy.
- Limited resolution and feature rounding and measurement uncertainty arise from limiting performance of instrument components such as diffraction gratings and detectors.
- Further limit the accuracy and repeatability of spectrophotometric data are calibration issues resulting from environmental conditions (e.g., temperature variations) and instrument drift.
Applications of Spectrophotometer
- Using the Beer-Lambert law, spectrophotometers are routinely applied in quantitative chemical analysis to ascertain analyte concentrations in solution.
- Through record of absorbance changes, they provide real-time monitoring of reaction kinetics, hence clarifying reaction processes.
- Spectrophotometers are crucial for testing proteins and nucleic acids in biochemistry so that one may evaluate concentration and purity.
- Environmental monitoring uses them to assess water quality and identify contaminants by use of spectral absorbance feature analysis.
- Spectrophotometers guarantee batch consistency by exact spectrum measurements, check drug composition, and monitor stability in pharmaceutical quality control.
- Using spectrophotometers, industrial colorimetric analysis evaluates dyes and pigments, therefore helping quality assurance in the food, textile, printing, and textile industries.
- In laboratory environments, they are valuable teaching aids since they give analytical chemistry and instrumental calibration practical experience.
- Spectrophotometric methods help clinical diagnostics evaluate metabolic markers for efficient patient monitoring, blood components, and enzyme activity.
Preventive Measures
- Use certified reference standards to routinely calibrate the spectrophotometer so that over time both wavelength accuracy and photometric linearity are kept constant.
- Using manufacturer-recommended solvents and methods, clean optical components—including cuvettes, lenses, and mirrors—to prevent contamination and degradation of light transmission.
- Plan regular light source and detector maintenance, replacing bulbs or filters as advised to prevent drift in performance and guarantee steady signal output.
- Operating the equipment in a controlled laboratory setting helps to shield it from environmental variations such temperature and humidity, hence reducing calibration mistakes and measurement drift.
- Regular monochromator inspection and alignment will help to reduce stray light interference by confirming optical seal and baffle integrity.
- Store optical accessories and cuvettes in temperature-regulated, dust-free environments to avoid chemical degradation that can compromise measurements or scratches and residue accumulation.
- Track instrument performance trends by documenting all maintenance actions and calibration records, then quickly fix any indications of degradation.
- Rigorously follow manufacturer recommendations and preventative maintenance schedule to guarantee data accuracy and extend the operating lifetime of the instrument.
What does a spectrophotometer measure?
- Usually spanning the ultraviolet (UV), visible, and near-infrared (NIR), a spectrophotometer gauges the strength of electromagnetic radiation over a given wavelength range.
- By use of a comparison between the incident light intensity and the transmitted light intensity, it determines the absorbance spectrum reflecting the chemical and physical characteristics of a material.
- The device calculates transmittance values, which when used to the Beer-Lambert law allow analyte concentrations in solution to be computed.
- By means of absorption, scattering, or emission, it gauges variations in light intensity, therefore providing information on molecular structure, reaction kinetics, and compound identification.
- Apart from solution analysis, spectrophotometers can be set to quantify reflectance from surfaces or thin films, hence increasing their relevance in materials research and quality control.
Difference between a spectrometer and a spectrophotometer
Aspect | Spectrometer | Spectrophotometer |
---|---|---|
Purpose | Analyzes light emitted or absorbed by substances | Measures light intensity at different wavelengths |
Application Areas | Astronomy, chemistry, physics, biomedical research | Chemistry, materials science, quality control |
Temperature and Speed Measurement | Yes | No |
Composition Analysis | Yes | No |
Biomedical Applications | Yes | No |
Absorbance Measurement | No | Yes |
Transparency and Reflectance Analysis | No | Yes |
Types | Various types for different applications | Mainly double-beam and basic types |
Key Principle | Analyzes emitted or absorbed light spectra | Measures light absorption, transmission, or reflection |
Primary Function | Qualitative and quantitative analysis | Concentration determination and material characterization |
Common Wavelength Range | Visible, ultraviolet, infrared | Ultraviolet, visible, near-infrared |
Differences between spectrometer and spectrophotometer – spectrometer vs spectrophotometer
Aspect | Spectrometer | Spectrophotometer |
---|---|---|
Components and Configuration | Component of a spectrophotometer | Complete system with a light source and spectrometer |
Usage | Requires initial warm-up; subject loaded and calibrated before measurements | Used for quantitative analysis of light interactions with samples; requires careful handling and calibration |
Measurement Process | Measures reflected or emitted colors and spectral information | Measures intensity before and after sample interaction to quantify absorbance, transmittance, or reflectance |
Calibration | Primarily establishes the baseline for wavelength measurements | Critical step involving instrument adjustment and setting a reference point (zero) with an empty cuvette |
Difference between UV (ultraviolet spectroscopy) and visible spectrophotometry
Aspect | UV (Ultraviolet) Spectroscopy | Visible Spectrophotometry |
---|---|---|
Spectral Range | 190 nm to 400 nm | Approximately 400 nm to 750 nm |
Absorption Mechanism | Excitation of non-bonding electrons or π-electron systems | Primarily involves transitions in π-electron systems |
Molecular States | Excitation to singlet excited states following quantum mechanical selection rules | Transitions to singlet excited states, often involving π-electron systems |
Applications | Analyzing compounds with non-bonding electrons, aromatic compounds, nucleic acids, proteins | Determining the concentration of colored solutions, studying transition metal complexes |
Fluorescence Spectroscopy | Forms the basis for fluorescence spectroscopy | Also serves as the foundation for fluorescence spectroscopy |
Difference between a double-beam spectrophotometer and a split beam spectrophotometer
Aspect | Double-Beam Spectrophotometer | Split-Beam Spectrophotometer |
---|---|---|
Real-Time Referencing | Utilizes separate reference position for simultaneous reference and sample measurements | Employs a beam splitter to divide incoming light into two beams, allowing simultaneous measurements |
Optical Path | Sample and reference beams follow separate optical paths | Sample and reference measurements follow distinct but simultaneous optical paths |
Applications | Suitable for high-accuracy and stable measurements, often used in research and quality control | Versatile and suitable for rapid data acquisition, making it ideal for kinetic measurements and routine analysis |
Key Advantages | Precise baseline correction, high accuracy, and stability | Rapid data acquisition, versatility, and suitability for kinetic measurements |
Considerations for Selection | Instrument features, performance validation options, service contracts, warranty, and application-specific accessories | Similar considerations as double-beam spectrophotometer, with a focus on the need for rapid data acquisition and parallel measurements |
Difference between a colorimeter and spectrophotometer
Aspect | Colorimeter | Spectrophotometer |
---|---|---|
Functionality | Measures absorption of a specific color | Measures transmittance/reflectance across a range of wavelengths |
Spectral Range | Limited to the visible spectrum (400 nm to 750 nm) | Covers UV, visible, and sometimes IR regions of the spectrum |
Cost | Generally less expensive due to limited functionality | Often more expensive due to extended capabilities and wider spectral range |
Primary Applications | Routine color analysis within the visible spectrum | Quantitative analysis and comprehensive spectral characterization in various scientific and industrial applications |
Quiz
What is the primary function of a spectrophotometer?
a) To measure the pH of a solution
b) To measure light intensity as a function of wavelength
c) To separate mixtures based on density
d) To determine the boiling point of a liquid
Which component of a spectrophotometer disperses polychromatic light into its constituent wavelengths?
a) Collimator lens
b) Detector
c) Prism or diffraction grating
d) Cuvette
Beer’s Law relates which of the following?
a) Absorbance to concentration
b) Absorbance to path length
c) Concentration to path length
d) Refractive index to concentration
Which light source is commonly used in a spectrophotometer for the visible spectrum?
a) Hydrogen lamp
b) Deuterium lamp
c) Tungsten lamp
d) LED light
What is the purpose of the entrance slit in a spectrophotometer?
a) To focus the light beam
b) To disperse light into different wavelengths
c) To provide a narrow image of the radiation
d) To detect the intensity of light
Which law states that the amount of light absorbed is directly proportional to the concentration of the solute in the solution?
a) Lambert’s Law
b) Newton’s Law
c) Beer’s Law
d) Boyle’s Law
In which region of the electromagnetic spectrum does a UV-Vis spectrophotometer operate?
a) Infrared and visible
b) Ultraviolet and infrared
c) Ultraviolet and visible
d) Microwave and visible
What material are cuvettes meant for the visible region typically made of?
a) Aluminum
b) Quartz
c) Plastic
d) Steel
Which component of a spectrophotometer ensures that light rays are parallel to each other?
a) Collimator lens
b) Prism
c) Detector
d) Entrance slit
In a double-beam spectrophotometer, what is the purpose of the beam splitter?
a) To focus the light beam
b) To split the single beam of light into two beams
c) To detect the intensity of light
d) To disperse light into different wavelengths
FAQ
What is a spectrophotometer?
A spectrophotometer is a scientific instrument used to measure the intensity of light at different wavelengths. It is commonly used in analytical chemistry and biochemistry for quantitative analysis of substances based on their absorption or transmission of light.
How does a spectrophotometer work?
A spectrophotometer works by passing a beam of light through a sample and measuring the intensity of light before and after it interacts with the sample. The instrument then calculates the absorbance or transmittance of the sample, which can be correlated to the concentration of a substance or used for qualitative analysis.
What are the main components of a spectrophotometer?
The main components of a spectrophotometer include a light source, a monochromator or wavelength selector, a sample holder (cuvette), a detector, and a display or readout system. These components work together to generate and analyze the light transmitted through the sample.
What is the purpose of calibration in a spectrophotometer?
Calibration is essential in a spectrophotometer to ensure accurate and reliable measurements. It involves using standard solutions of known concentration or absorbance to establish a reference point for the instrument. By calibrating the spectrophotometer, you can account for any variations or deviations in the system and obtain accurate results.
Can a spectrophotometer measure color?
Yes, a spectrophotometer can measure color by analyzing the absorption or reflection of light at different wavelengths. Colorimetry, a technique based on spectrophotometry, is commonly used to quantify the color properties of samples and materials.
What is the difference between absorbance and transmittance?
Absorbance and transmittance are two measurements obtained from a spectrophotometer. Absorbance (A) represents the amount of light absorbed by a sample and is calculated using the Beer-Lambert Law. Transmittance (T) indicates the percentage of light that passes through the sample and is calculated as the ratio of the transmitted light intensity to the incident light intensity.
Can a spectrophotometer analyze multiple components in a mixture?
Yes, a spectrophotometer can analyze multiple components in a mixture by employing various techniques such as multicomponent analysis or spectral deconvolution. These methods use mathematical algorithms to separate and quantify the contributions of different substances in a complex sample based on their unique absorption characteristics.
What is the range of wavelengths that a spectrophotometer can measure?
The range of wavelengths that a spectrophotometer can measure depends on its design and type. UV-visible spectrophotometers typically cover the ultraviolet (UV) range of 185 to 400 nm and the visible (VIS) range of 400 to 700 nm. Infrared (IR) spectrophotometers can measure wavelengths ranging from 700 nm to as high as 15,000 nm.
Can a spectrophotometer be used for qualitative analysis?
Yes, a spectrophotometer can be used for qualitative analysis. By analyzing the absorption spectrum of a sample, which shows the pattern of light absorbed at different wavelengths, it is possible to identify the presence of specific substances based on their characteristic absorption peaks or bands.
What are some common applications of spectrophotometers?
Spectrophotometers have diverse applications in various fields. Some common applications include quantitative analysis of chemicals in pharmaceuticals, environmental monitoring, food and beverage quality control, DNA and protein analysis, color measurement in textiles and paints, and research in physics, chemistry, and biology.
References
- BATRA, S. (2022). SPECTROPHOTOMETER – Principle, Components, Working & Application | BIOCHEMISTRY PRACTICALS. Paramedics World. Retrieved 6 June 2022, from https://paramedicsworld.com/biochemistry-practicals/demonstration-of-spectrophotometer-principle-components-working-applications/
- Manandhar, S., & Sharma, S. (2017). Practical Approach to Microbiology (3rd ed., pp. 18-20). National Book Centre.
- Phillips, K. (2022). What Is a Spectrophotometer and How Does It Work? – HunterLab Horizons Blog. HunterLab Horizons Blog. Retrieved 6 June 2022, from https://blog.hunterlab.com/blog/color-measurement/what-is-spectrophotometer/
- https://www.xrite.com/learning-color-education/other-resources/what-is-a-spectrophotometer
- https://chem.libretexts.org/Bookshelves/Physical_and_Theoretical_Chemistry_Textbook_Maps/Supplemental_Modules_(Physical_and_Theoretical_Chemistry)/Kinetics/02_Reaction_Rates/2.01_Experimental_Determination_of_Kinetics/2.1.05_Spectrophotometry#:~:text=Aspectrophotometerisaninstrument,theintensityoflightdetected.
- https://www.labcompare.com/Spectroscopy/106-Spectrophotometer/
- https://blog.hunterlab.com/blog/color-measurement/what-is-spectrophotometer/
- https://tipbiosystems.com/blog/spectrophotometry/
- https://www.linquip.com/blog/types-of-spectrophotometers/
- https://laboratoryinfo.com/spectrophotometer/
- https://microbenotes.com/spectrophotometer-principle-instrumentation-applications/