What is a Signal Transduction Pathway?
- A signal transduction pathway refers to the series of molecular events that occur inside a cell in response to a chemical or physical signal. It is the mechanism by which a cell converts an external signal into a specific cellular response. The process begins when a ligand, which is a chemical signal, binds to its corresponding receptor on the cell surface or in the cytoplasm.
- Receptor proteins are specialized based on the type of cell they are attached to. Different cells receive various signals from the body or the environment, requiring specialized receptors to coordinate specific responses. Once a ligand binds to a receptor, it initiates a biochemical cascade, which is a chain of biochemical events known as a signaling pathway. These pathways can interact with each other, forming networks that allow for coordinated cellular responses.
- The signaling pathways involve various components or nodes, each playing a specific role in transmitting the signal. Ligands are the first messengers, while receptors act as signal transducers, converting the signal into a cellular response. Primary effectors, often proteins, are then activated by the receptors, which can further activate secondary effectors. This amplification of the signal, known as signal gain, allows a single signaling molecule to generate a response involving a large number of molecules.
- The transduction of biological signals is characterized by factors such as delay, noise, signal feedback and feedforward, and interference. Computational biology has become an essential tool in understanding signaling pathways and networks, aiding in the study of cellular functions and disease, including drug resistance.
- Signal transduction pathways play a crucial role in regulating cell communication in multicellular organisms. They control various processes such as cell growth, proliferation, metabolism, and many others. The pathways can lead to changes in gene transcription or translation, post-translational modifications, conformational changes in proteins, and alterations in protein localization.
- Overall, signal transduction pathways enable cells to receive and interpret signals from the environment or the body, allowing for coordinated cellular responses and the maintenance of homeostasis in the cell and the organism as a whole.
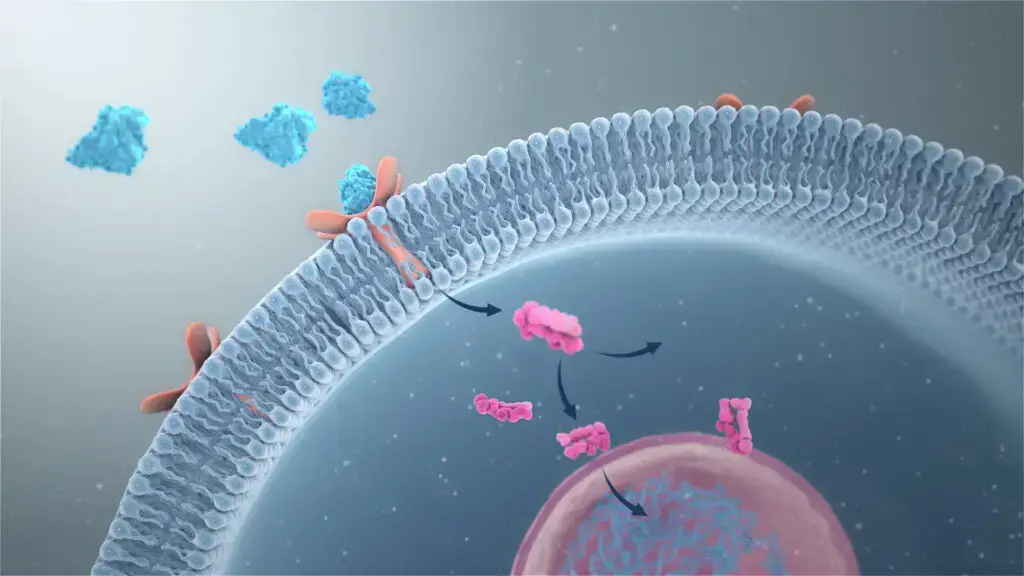
Definition of Signal Transduction Pathway
A signal transduction pathway is a series of molecular events inside a cell that converts an external signal into a specific cellular response.
Components of signal transduction
Signal transduction involves several key components that are common in both plants and animals. These components include:
- Signal molecules/Ligands/Extracellular messengers/Primary messengers: These are the molecules that carry the signal and initiate the process of signal transduction. Signal molecules can be various types of chemical messengers, such as hormones, neurotransmitters, growth factors, or environmental cues. They can be produced by the organism itself or received from the external environment.
- Receptors: Receptors are proteins located on the cell surface or inside the cell that specifically bind to the signal molecules. They act as molecular switches that recognize and bind to the ligands, triggering a series of events in the cell. Receptors are highly specialized and can differ depending on the type of signal they receive.
- Second messengers/Effector molecules: Once the signal molecule binds to its receptor, it often leads to the activation of second messengers or effector molecules. Second messengers are small molecules or ions that relay the signal from the receptor to downstream components in the signaling pathway. Common second messengers include cyclic AMP (cAMP), calcium ions (Ca2+), and inositol trisphosphate (IP3). They amplify and propagate the signal within the cell, activating or inhibiting various cellular processes.
- Downstream response elements: The activation of second messengers leads to the initiation of specific downstream response elements within the cell. These response elements can include activation or inhibition of enzymes, changes in gene expression, alterations in protein activity or localization, and ultimately, cellular responses. The nature of the response depends on the specific signaling pathway and the context of the cell or organism.
Overall, the components of signal transduction, including signal molecules, receptors, second messengers, and downstream response elements, work together to transmit and interpret signals, allowing cells and organisms to respond and adapt to changes in their environment or internal conditions.
Signal Transduction Pathway Steps
Signal transduction pathways are the processes by which cells convert external signals into intracellular responses. They involve a series of steps that allow cells to communicate and respond to various stimuli. Here are the general steps involved in a typical signal transduction pathway:
- Reception: The first step is the reception of the signal by a cell. External signals can be physical, chemical, or biological in nature and are detected by specific receptor molecules located on the cell surface or within the cell.
- Transduction: Once the signal is received, it needs to be transmitted across the cell membrane and converted into an intracellular signal. This step involves a series of biochemical reactions and often requires the involvement of signaling molecules or secondary messengers, such as cyclic AMP (cAMP), calcium ions (Ca2+), or inositol triphosphate (IP3).
- Amplification: In many cases, the signal needs to be amplified to ensure an adequate response within the cell. Amplification can occur through enzymatic cascades, where a single signaling molecule activates multiple downstream molecules, leading to an amplification of the signal.
- Transmission: After amplification, the intracellular signal is transmitted to its target destination within the cell. This can involve the activation or modulation of various proteins, enzymes, or transcription factors.
- Cellular Response: The ultimate goal of a signal transduction pathway is to initiate a specific cellular response. This response can be diverse and can include changes in gene expression, alteration of enzyme activity, cytoskeletal rearrangements, cell growth, proliferation, differentiation, or apoptosis (cell death).
- Termination: Once the signal has triggered the desired response, it is crucial for the pathway to be terminated to prevent continuous activation. Termination mechanisms can involve feedback loops, where the final response components inhibit earlier components of the pathway, or the removal of signaling molecules from the intracellular environment.
It is important to note that signal transduction pathways can vary significantly depending on the specific signaling molecule, receptor, and cellular context. Different pathways may involve different intermediates, proteins, and regulatory mechanisms to achieve their intended responses.
Signal Molecules
- Signal molecules, also known as signaling molecules or ligands, are chemical entities that carry information or messages within and between cells. These molecules serve as the initiators of signal transduction pathways, triggering specific cellular responses. Signal molecules can originate from the external environment, such as environmental cues, or they can be produced internally within the cell.
- In the context of plant cells, signal molecules play a crucial role in coordinating various physiological processes and responses to environmental stimuli. Plants employ specialized sensor proteins called receptors to perceive these signals. Receptors can be located on the cell surface or within the cell, depending on the type of signal they detect.
- When a signal molecule binds to its corresponding receptor, it initiates a series of biochemical steps known as signal transduction. This process involves the transmission of information from the site of signal perception to the site of cellular response. During signal transduction, the signal is relayed through a cascade of biochemical reactions, often involving protein kinases, phosphatases, second messengers, and other signaling molecules. These biochemical steps facilitate the amplification, integration, and modulation of the signal, ultimately leading to the activation or inhibition of specific cellular processes.
- Signal molecules can be diverse in nature. They can include hormones, neurotransmitters, growth factors, environmental cues (such as light, temperature, and nutrient availability), and other chemical messengers. Different signal molecules elicit specific responses in the cell or plant, regulating processes such as growth, development, metabolism, immune responses, and stress adaptation.
- In summary, signal molecules play a vital role in cellular communication and coordination. They are perceived by receptors, initiating signal transduction pathways that transmit the information to elicit specific cellular responses. The diverse range of signal molecules and their receptors enable cells and organisms to sense and respond to various environmental and intracellular cues, contributing to their survival and adaptation.
Stimuli
Stimuli are the various inputs or triggers that initiate signal transduction pathways and elicit responses in cells or organisms. These stimuli can be classified into different categories based on their nature and origin.
- Environmental cues: These stimuli originate from the external environment and include factors such as the presence of specific molecules or physical conditions. For example, the presence of epidermal growth factor (EGF) can serve as an extracellular cue that triggers a signal transduction pathway.
- Intracellular events: Some stimuli arise from events occurring within the cell itself. An example is DNA damage resulting from replicative telomere attrition, which can initiate signal transduction pathways involved in DNA repair or cell cycle regulation.
- Senses: Signals that reach the central nervous system and are transmitted between neurons are often classified as senses. This includes processes like synaptic transmission, which transfers signals from one neuron to another. Different senses, such as touch, vision, hearing, proprioception, and balance, rely on specialized mechanisms of signal transduction to convert external stimuli into cellular responses.
- Embryonic development: During embryogenesis, various intercellular signal relay mechanisms coordinate the development and patterning of tissues and organs. These signaling pathways play crucial roles in processes like cell differentiation, migration, and tissue morphogenesis.
Examples of Stimuli
- In signal transduction, ligands or signaling molecules are key components that interact with receptors to initiate the cascade of events leading to a cellular response. Ligands can be soluble molecules from the extracellular medium, including growth factors, cytokines, neurotransmitters, or components of the extracellular matrix. Some molecules, such as steroid hormones, are lipid-soluble and can cross the plasma membrane to interact with cytoplasmic or nuclear receptors.
- Mechanical forces can also act as stimuli and initiate signal transduction pathways. Cells have mechanosensitive proteins and complexes that detect changes in the stiffness of the substratum or mechanical stress and transmit these signals to downstream signaling molecules. Mechanotransduction pathways play essential roles in cell adhesion, migration, and tissue development.
- Osmotic stimuli, related to changes in osmolarity, can be detected by osmosensors or osmoreceptors. These proteins sense variations in macromolecular crowding, ionic strength, and properties of the plasma membrane or cytoskeleton. Changes in osmotic pressure are critical for cellular and systemic homeostasis.
- Temperature is another important stimulus that cells can detect. Thermoception, the sensing of temperature, is mediated by specific proteins called transient receptor potential channels. Cells also have mechanisms to protect against high temperatures, such as the heat-shock response, which is activated when temperatures cause the dissociation of inactive heat shock factor 1 (HSF1) from complexes with heat shock proteins.
- Light serves as a stimulus for visual perception and the regulation of the circadian clock in mammals. Photoreceptor cells in the retina detect light using light-sensitive proteins, such as rhodopsin in rod and cone cells for vision, or melanopsin in intrinsically photosensitive retinal ganglion cells for the circadian clock.
In summary, stimuli in signal transduction can be diverse, originating from the environment or internal cellular processes. They include environmental cues, intracellular events, sensory inputs, mechanical forces, osmolarity changes, temperature variations, and light. The specific nature of the stimulus determines the receptors, signaling molecules, and downstream responses involved in the signal transduction pathway.
Receptors of Signal Transduction
Receptors can be roughly divided into two major classes: intracellular and extracellular receptors.
1. Extracellular receptors
Extracellular receptors are a type of integral transmembrane proteins that play a crucial role in signal transduction. These receptors span the plasma membrane of the cell, with one part located on the outside of the cell and the other on the inside. When a ligand binds to the external region of the receptor, it induces a conformational change in the intracellular part of the receptor, which is referred to as “receptor activation.” This activation leads to the activation of an enzyme domain within the receptor or the exposure of a binding site for intracellular signaling proteins.
In eukaryotic cells, many intracellular proteins activated by ligand-receptor interactions possess enzymatic activities, such as tyrosine kinase and phosphatases. These enzymes are often covalently linked to the receptor and can generate second messengers like cyclic AMP and IP3. Additionally, activated proteins can interact with adaptor proteins, facilitating the coordination of signaling complexes necessary for responding to specific stimuli. Adaptor proteins and enzymes involved in signal transduction often possess specialized protein domains that bind to specific secondary messenger molecules. For example, calcium ions bind to the EF hand domains of calmodulin, allowing it to activate calmodulin-dependent kinase.
- G protein–coupled receptors: One prominent class of extracellular receptors is G protein-coupled receptors (GPCRs). GPCRs are integral transmembrane proteins with seven transmembrane domains and are linked to heterotrimeric G proteins. They are the largest family of membrane proteins and receptors in mammals. Upon ligand recognition, the conformation of the GPCR changes, leading to the activation of the associated G protein. The activated G protein subunits then detach from the receptor and initiate signaling through downstream effector proteins, including phospholipases and ion channels, resulting in the release of second messenger molecules.
- Tyrosine, Ser/Thr and Histidine-specific protein kinases: Receptor tyrosine kinases (RTKs) are another type of extracellular receptors. They possess an intracellular kinase domain and an extracellular ligand-binding domain. RTKs form dimers upon ligand binding, leading to autophosphorylation of tyrosine residues within the intracellular kinase domains. This phosphorylation initiates signaling cascades involving various cytoplasmic molecules, influencing cellular processes like cell differentiation and metabolism. Proteins that bind GTP, such as Ras, Rho, and Raf, play a significant role in transducing signals from activated RTKs into the cell.
- Integrins: Integrins, which play a role in cell adhesion and signal transduction, are another class of extracellular receptors. Ligand binding to integrins induces conformational changes, clustering the proteins at the cell membrane and initiating signal transduction. Integrins lack kinase activity, so their signaling relies on intracellular protein kinases and adaptor molecules, with integrin-linked kinase being a central coordinator. Integrins are essential for cell attachment to the extracellular matrix and mediate signals from matrix components, regulating cellular processes like survival, apoptosis, proliferation, and differentiation.
- Toll-like receptors: Toll-like receptors (TLRs) are involved in the detection of pathogens and activation of immune responses. When activated, TLRs recruit adapter molecules within the cell, leading to the induction or suppression of genes involved in immune responses. TLR signaling plays a crucial role in modulating gene expression and immune system activation.
- Ligand-gated ion channels: Ligand-gated ion channels are another type of extracellular receptors. These channels undergo conformational changes upon ligand binding, allowing ions to pass through the cell membrane. The influx of ions, such as calcium (Ca2+), can act as a second messenger, initiating signal transduction cascades and altering cellular physiology.
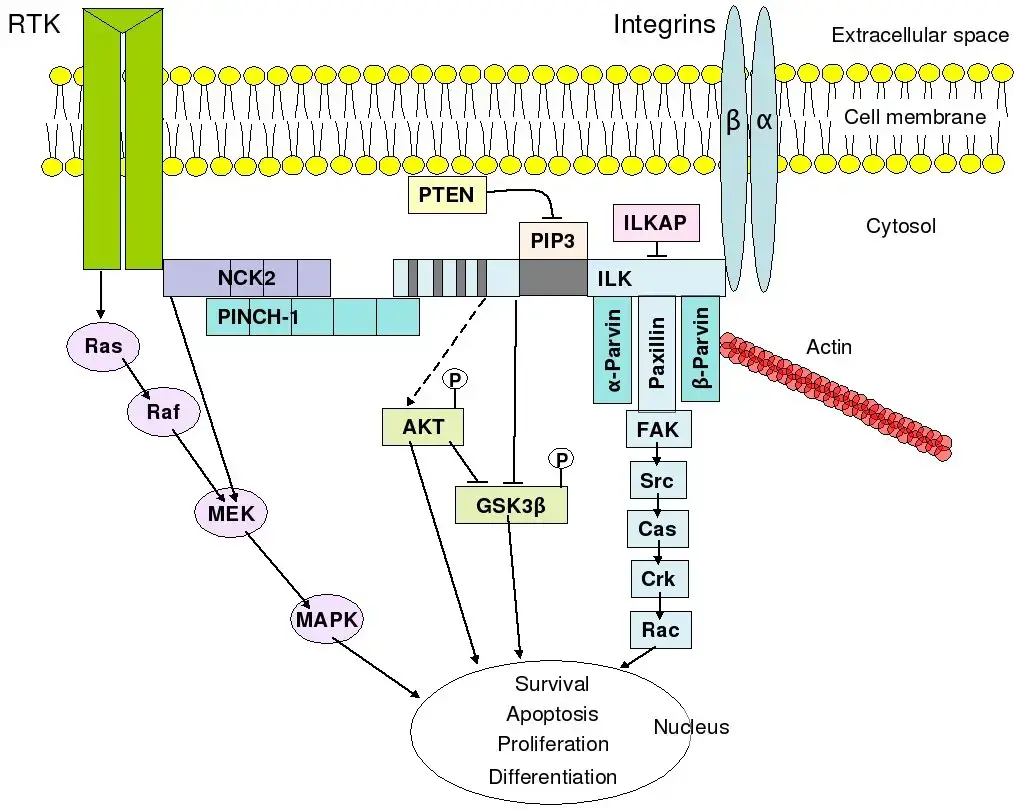
Overall, extracellular receptors are integral components of signal transduction pathways, converting extracellular signals into intracellular responses, and playing critical roles in various physiological processes.
2. Intracellular receptors
Intracellular receptors play a crucial role in signal transduction by responding to specific ligands within the cell. There are two main types of intracellular receptors: nuclear receptors and cytoplasmic receptors.
- Nuclear receptors are soluble proteins located within the nucleus and are activated by non-polar hormones such as steroid hormones, vitamin derivatives, and retinoic acid. The ligands for nuclear receptors must pass through the plasma membrane by passive diffusion to initiate signal transduction. Once bound to the receptor, the ligands enter the nucleus and alter gene expression. Activated nuclear receptors bind to specific DNA sequences called hormone-responsive elements (HREs) in the promoter region of target genes. This binding leads to the induction of gene expression, and the effects of hormone-receptor complex activation can persist even after the ligand concentration decreases. DNA-binding domains of nuclear receptors contain zinc fingers that stabilize DNA binding, while the ligand-binding domain is responsible for dimerization of receptors and transactivation of gene expression.
- Steroid receptors are a subclass of nuclear receptors primarily located in the cytosol. In the absence of steroids, they form a complex with chaperone or heat shock proteins (HSPs). HSPs assist in the proper folding of the receptor, enabling its translocation into the nucleus. Steroid receptors can also repress gene expression when their transactivation domain is hidden. Phosphorylation of serine residues in the N-terminal of the receptor can enhance its activity, a process known as crosstalk.
- Retinoic acid receptors are another type of nuclear receptor that can be activated by ligands that enter the cell through diffusion or are synthesized intracellularly. These receptors are found in the nucleus and do not require HSPs for activation. In the absence of ligands, they repress gene expression by binding to specific DNA sequences. Upon ligand binding, they undergo conformational changes that relieve the repression and allow gene expression to occur.
- Cytoplasmic receptors, such as NOD-like receptors (NLRs), are intracellular receptors of the immune system. NLRs are located in the cytoplasm of eukaryotic cells and interact with ligands using leucine-rich repeat (LRR) motifs. They play a role in innate immunity by interacting with various molecules and initiating signaling pathways. For example, NOD2 interacts with RIP2 kinase to activate NF-κB signaling, while NALP3 interacts with inflammatory caspases and triggers the processing of cytokines like interleukin-1β.
Overall, intracellular receptors, whether nuclear or cytoplasmic, are essential for transmitting signals within cells and regulating gene expression in response to specific ligands.
Second messengers
Second messengers play a crucial role in intracellular signal transduction, relaying information from the extracellular environment to trigger cellular responses. Unlike first messengers, which are signaling molecules that bind to specific cell surface receptors, second messengers are substances that enter the cytoplasm and act within the cell.
- Calcium is an important second messenger involved in various cellular processes. When released from the endoplasmic reticulum into the cytosol, calcium ions bind to signaling proteins, activating them and initiating a response. The transport of calcium is regulated by receptor/ion channel proteins such as the InsP3-receptor and the ryanodine receptor. Calcium is involved in muscle contraction, neurotransmitter release, cell migration, and regulation of proteins through direct binding or interaction with enzymes.
- Lipophilic second messengers are derived from lipids present in cellular membranes. When activated receptors stimulate specific enzymes, they modify these lipids, leading to the generation of second messengers. One example is diacylglycerol, which is necessary for the activation of protein kinase C.
- Nitric oxide (NO) acts as a second messenger due to its ability to diffuse through the plasma membrane and influence nearby cells. NO is synthesized from arginine and oxygen by the enzyme NO synthase. It activates soluble guanylyl cyclase, which produces another second messenger called cyclic guanosine monophosphate (cGMP). Additionally, NO can modify proteins or their metal co-factors through covalent interactions. It has diverse functions, including the relaxation of blood vessels, regulation of apoptosis, and involvement in penile erections. However, high concentrations of NO can be toxic and cause damage, particularly during strokes.
- Redox signaling is another form of second messenger signaling. In this process, electronically activated species, such as superoxide, hydrogen peroxide, carbon monoxide, and hydrogen sulfide, act as signal-transducing agents. Redox signaling also encompasses the modulation of electronic flows in semiconductive biological macromolecules.
Overall, second messengers play a critical role in transmitting signals from the extracellular environment to the intracellular space, facilitating cellular responses and coordinating various physiological processes.
Summery
- Calcium:
- Receptor/Ion Channel Proteins:
- InsP3-receptor: Transports calcium upon interaction with inositol triphosphate (InsP3) on its cytosolic side.
- Ryanodine receptor: Similar to the InsP3 receptor but has a feedback mechanism that releases more calcium upon binding with it.
- Receptor/Ion Channel Proteins:
- Lipid Messengers:
- Diacylglycerol: Required for the activation of protein kinase C.
- Nitric Oxide (NO):
- NO Synthase: Enzyme responsible for synthesizing NO from arginine and oxygen.
- Soluble Guanylyl Cyclase: Activated by NO and produces another second messenger, cyclic guanosine monophosphate (cGMP).
- Redox Signaling:
- Electronically Activated Species:
- Superoxide: Involved in redox signaling.
- Hydrogen Peroxide: Acts as a signal-transducing agent.
- Carbon Monoxide: Participates in redox signaling.
- Hydrogen Sulfide: Plays a role in redox signaling.
- Electronically Activated Species:
These receptors and molecules are part of the second messenger system, which facilitates intracellular signal transduction and coordinates various cellular responses.
Cellular responses
- Cellular responses refer to the reactions and changes that occur within cells in response to extracellular stimulation. These responses are essential for controlling cell behavior in both unicellular and multicellular organisms. Signal transduction pathways play a central role in mediating these cellular responses, and their dysregulation is associated with numerous diseases.
- One important cellular response is gene activation. When cells are stimulated by external signals, such as growth factors or hormones, a process known as signal transduction is initiated. This process involves a series of molecular events that transmit the signal from the cell surface to the nucleus, ultimately resulting in the activation of specific genes. The products of these responding genes can act as instigators of further gene activation, creating a cascade effect. Transcription factors, which are proteins produced as a result of signal transduction, play a crucial role in activating additional genes. As a result, a single initial stimulus can lead to the expression of a large number of genes, leading to various physiological events.
- The activation of specific genes in response to extracellular signals can have significant cellular effects. For example, the increased uptake of glucose from the bloodstream can be triggered by the expression of certain genes. This is important for regulating cellular metabolism and providing energy for various cellular processes. Additionally, the migration of neutrophils to sites of infection is another cellular response that involves the activation of specific genes. These examples highlight how cellular responses, driven by gene activation, contribute to physiological events and the overall behavior of cells.
- The specific set of genes and their activation order in response to certain stimuli is often referred to as a genetic program. This program dictates the sequence of gene expression and subsequent cellular responses. By understanding these genetic programs, researchers can gain insights into the mechanisms underlying cellular behavior and the complex interplay between extracellular signals and intracellular processes.
- Cell division and survival are crucial processes for mammalian cells. Stimulation in the form of growth factors is required to promote cell division and prevent apoptosis (programmed cell death). In the absence of these growth factors, cells undergo apoptosis. This highlights the importance of extracellular stimulation in regulating cell growth and survival.
- Cellular responses can be categorized into two types: transcription-dependent and transcription-independent responses. Transcription-dependent responses involve the activation of gene expression and subsequent protein synthesis. For example, steroids can act as transcription factors, directly binding to DNA and initiating gene transcription. However, this process is relatively slow as it requires DNA transcription, mRNA translation, and post-translational modifications of the resulting protein or peptide.
- In contrast, transcription-independent responses do not involve gene transcription and protein synthesis. Instead, these responses are mediated through intracellular signaling pathways. For instance, epidermal growth factor (EGF) binds to the epidermal growth factor receptor (EGFR), leading to the dimerization and autophosphorylation of the EGFR. This activates specific intracellular signaling pathways, triggering various cellular responses. Transcription-independent responses are generally faster compared to transcription-dependent responses.
- In addition to stimulatory signals, inhibitory signals and permissive signals also play a role in cellular responses. Inhibitory signals can arise from cell-cell contact, where certain molecules or interactions between cells inhibit cellular processes. On the other hand, permissive signals are derived from cell-matrix interactions, which provide the necessary conditions for cells to carry out specific functions.
- Overall, cellular responses involve the integration of multiple signals and subsequent alterations in cytoplasmic machinery, leading to changes in cell behavior. These responses are critical for maintaining cellular homeostasis, coordinating developmental processes, and adapting to the environment. Dysregulation of cellular responses and signal transduction pathways can contribute to the development of various diseases, underscoring the importance of understanding these processes for biomedical research and therapeutic interventions.
Signal transduction pathways that include a phosphorylation cascade
Signal transduction pathways involving phosphorylation cascades are common mechanisms for transmitting and amplifying signals within cells. Here are a few examples of signal transduction pathways that include phosphorylation cascades:
- MAPK/ERK Pathway: The MAPK/ERK pathway, mentioned earlier, involves a phosphorylation cascade. In this pathway, the activation of receptor tyrosine kinases (RTKs) or G-protein coupled receptors (GPCRs) leads to the activation of small GTPases and the subsequent activation of MAP kinase kinase kinases (MAPKKKs). MAPKKKs phosphorylate and activate MAP kinase kinases (MAPKKs), which, in turn, phosphorylate and activate mitogen-activated protein kinases (MAPKs) such as ERK1/2. The final activated ERK1/2 translocates to the nucleus and phosphorylates various transcription factors, thereby regulating gene expression and promoting cell growth, proliferation, and differentiation.
- JNK/SAPK Pathway: The c-Jun N-terminal kinase (JNK)/stress-activated protein kinase (SAPK) pathway is another phosphorylation cascade involved in cellular stress responses, apoptosis, and immune signaling. Various cellular stresses, such as UV radiation, inflammatory cytokines, or osmotic stress, activate upstream MAPKKKs, including MEKK1/2/3/4, which phosphorylate and activate MAPKKs called MKK4/7. These MAPKKs, in turn, phosphorylate and activate JNK/SAPKs. Activated JNK/SAPKs phosphorylate a range of downstream targets, including transcription factors, leading to the regulation of stress responses and cell fate decisions.
- Insulin Receptor Signaling Pathway: The insulin receptor signaling pathway, discussed previously, involves a phosphorylation cascade as well. Upon insulin binding to its receptor, the receptor undergoes autophosphorylation, leading to the recruitment and phosphorylation of insulin receptor substrates (IRS) proteins. Phosphorylated IRS proteins then serve as docking sites for phosphoinositide 3-kinase (PI3K), which phosphorylates phosphatidylinositol-4,5-bisphosphate (PIP2) to generate phosphatidylinositol-3,4,5-trisphosphate (PIP3). PIP3 recruits and activates protein kinase B (Akt), which phosphorylates various downstream targets involved in glucose metabolism and cell survival.
These examples demonstrate how phosphorylation cascades are utilized in signal transduction pathways to relay and amplify signals, leading to specific cellular responses. In each case, the sequential activation and phosphorylation of multiple kinases create a signaling cascade that culminates in the regulation of gene expression, metabolic processes, and cellular behaviors.
Types of signal transduction pathways
There are several types of signal transduction pathways that cells use to transmit and process signals. These pathways vary in their mechanisms and components. Here are some common types of signal transduction pathways:
- G-protein coupled receptor (GPCR) pathway: GPCRs are a large family of cell surface receptors that transmit signals from extracellular ligands such as hormones and neurotransmitters. Upon ligand binding, GPCRs undergo conformational changes that activate associated G-proteins. The activated G-proteins then modulate the activity of downstream effectors, such as enzymes or ion channels, initiating intracellular signaling cascades.
- Receptor tyrosine kinase (RTK) pathway: RTKs are transmembrane receptors with intrinsic kinase activity. Ligand binding induces receptor dimerization and autophosphorylation, leading to the activation of downstream signaling pathways. Phosphorylated tyrosine residues on the receptor serve as docking sites for various signaling molecules, triggering cascades of phosphorylation events and activation of downstream effectors.
- Ion channel signaling: Ion channels are proteins that regulate the flow of ions across cell membranes. Some ion channels can act as receptors, sensing extracellular signals such as neurotransmitters or changes in membrane voltage. Upon signal binding or voltage change, ion channels undergo conformational changes that allow ion flow, leading to changes in the cell’s electrical properties or triggering downstream events.
- Nuclear receptor signaling: Nuclear receptors are intracellular transcription factors that can directly bind to specific DNA sequences and regulate gene expression. These receptors are typically activated by lipophilic ligands, such as steroid hormones. Upon ligand binding, nuclear receptors translocate to the nucleus, interact with co-regulatory proteins, and modulate gene transcription.
- Notch signaling pathway: The Notch pathway regulates cell fate determination and development. It involves the interaction of transmembrane Notch receptors and their ligands. Ligand binding triggers proteolytic cleavage of the receptor, releasing the Notch intracellular domain (NICD). NICD translocates to the nucleus and interacts with transcription factors, regulating target gene expression.
- Wnt signaling pathway: The Wnt pathway plays crucial roles in embryonic development, tissue homeostasis, and stem cell regulation. Wnt ligands bind to cell surface receptors, leading to the stabilization and nuclear translocation of β-catenin. In the nucleus, β-catenin interacts with transcription factors, modulating gene expression and influencing cell fate determination.
These are just a few examples of the diverse types of signal transduction pathways that cells utilize to communicate and respond to extracellular signals. Each pathway is characterized by specific receptors, signaling molecules, and downstream effectors, resulting in unique cellular responses and physiological outcomes.
In signal transduction pathways water soluble hormones often
In signal transduction pathways, water-soluble hormones, such as peptides and proteins, often bind to cell surface receptors to initiate signaling cascades. Here are a few examples of water-soluble hormones and their associated signal transduction pathways:
- Epinephrine (Adrenaline) and the cAMP Pathway: Epinephrine, a water-soluble hormone released by the adrenal glands in response to stress, binds to G-protein coupled receptors (GPCRs) on the cell surface. The binding of epinephrine to its receptor activates the G-protein Gs, which stimulates adenylate cyclase. Adenylate cyclase converts ATP into cyclic adenosine monophosphate (cAMP), a secondary messenger. cAMP activates protein kinase A (PKA), which phosphorylates downstream target proteins, leading to various cellular responses such as increased heart rate and glycogen breakdown.
- Insulin and the PI3K/Akt Pathway: Insulin, a peptide hormone produced by the pancreas, binds to the insulin receptor on the cell surface. This binding activates the receptor’s intrinsic tyrosine kinase activity, leading to the phosphorylation of insulin receptor substrates (IRS). The phosphorylated IRS proteins recruit and activate phosphoinositide 3-kinase (PI3K), which generates phosphatidylinositol 3,4,5-trisphosphate (PIP3). PIP3 recruits and activates Akt (protein kinase B), which phosphorylates downstream targets involved in glucose metabolism, protein synthesis, and cell survival.
- Growth Factors (e.g., Epidermal Growth Factor – EGF) and the MAPK/ERK Pathway: Growth factors are water-soluble hormones that bind to specific receptors on the cell surface, initiating signaling cascades. For example, epidermal growth factor (EGF) binds to the EGF receptor (EGFR), a receptor tyrosine kinase. EGFR activation leads to the phosphorylation and recruitment of adaptor proteins such as Grb2, which in turn activates the small GTPase Ras. Ras activates the MAP kinase kinase kinase (MAPKKK) Raf, leading to the phosphorylation and activation of the MAP kinase kinase (MAPKK) MEK. Activated MEK phosphorylates and activates the mitogen-activated protein kinase (MAPK) ERK, which translocates to the nucleus and regulates gene expression, promoting cell proliferation and differentiation.
These examples illustrate how water-soluble hormones interact with cell surface receptors to initiate signal transduction pathways, leading to the activation of downstream effectors and the regulation of various cellular processes. Each hormone-receptor interaction triggers specific intracellular signaling events, ultimately resulting in diverse physiological responses.
Signal Transduction Pathway examples
There are numerous signal transduction pathways that play essential roles in cellular communication and coordination. Here are a few examples of well-known signal transduction pathways:
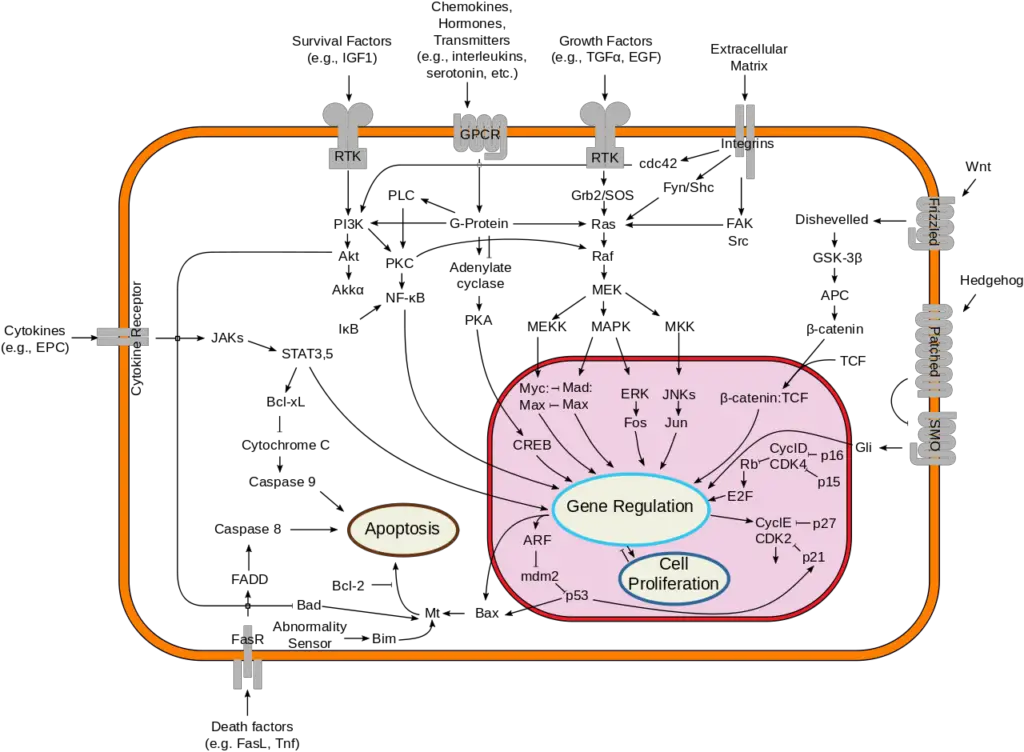
- MAPK/ERK Pathway: The mitogen-activated protein kinase (MAPK) pathway, also known as the extracellular signal-regulated kinase (ERK) pathway, is a highly conserved pathway involved in cell proliferation, differentiation, and survival. It is activated by growth factors, cytokines, and other extracellular signals. The pathway involves a series of protein kinases, including RAF, MEK, and ERK, which are sequentially activated by phosphorylation. Ultimately, ERK translocates to the nucleus, where it regulates gene expression and promotes various cellular responses.
- PI3K/Akt/mTOR Pathway: The phosphatidylinositol 3-kinase (PI3K)/Akt/mTOR pathway is critical for cell growth, survival, and metabolism. It is primarily activated by growth factors, insulin, and other stimuli. The pathway involves the activation of PI3K, leading to the generation of phosphatidylinositol 3,4,5-trisphosphate (PIP3). PIP3 recruits and activates Akt, which in turn regulates downstream targets involved in protein synthesis, cell growth, and glucose metabolism. The mTOR protein also plays a key role in this pathway, integrating signals and controlling cell growth and proliferation.
- cAMP-dependent pathway: The cAMP (cyclic adenosine monophosphate) pathway relies on the activation of protein kinase A (PKA), which is dependent on cAMP. Once activated, PKA can phosphorylate various target proteins, leading to diverse cellular effects. The specific outcomes of the cAMP-dependent pathway depend on the cell type involved.
- IP3/DAG pathway: The IP3 (inositol 1,4,5-triphosphate)/DAG pathway involves the cleavage of the phospholipid phosphatidylinositol 4,5-bisphosphate (PIP2) by phospholipase C (PLC). This cleavage generates DAG and IP3 as second messengers. DAG remains associated with the cell membrane, while IP3 is released into the cytosol. IP3 diffuses through the cytosol and binds to IP3 receptors located on the endoplasmic reticulum (ER), specifically calcium channels. This binding leads to an increase in cytosolic calcium concentration, initiating a cascade of intracellular changes. Additionally, calcium and DAG together activate protein kinase C (PKC), which phosphorylates other molecules, further modulating cellular activity. The end-effects of the IP3/DAG pathway can range from sensory perception, such as taste, to mood disorders like manic depression and even tumor promotion.
- Wnt/β-catenin Pathway: The Wnt/β-catenin pathway regulates embryonic development, tissue homeostasis, and stem cell renewal. Wnt ligands bind to specific receptors on the cell surface, leading to the stabilization and nuclear translocation of β-catenin. In the nucleus, β-catenin acts as a transcriptional co-activator, regulating the expression of genes involved in cell fate determination and proliferation.
- JAK/STAT Pathway: The Janus kinase (JAK)/signal transducer and activator of transcription (STAT) pathway is involved in cytokine signaling and immune responses. Cytokines bind to specific receptors, activating JAK kinases. JAKs then phosphorylate STAT proteins, which dimerize and translocate to the nucleus to regulate gene expression. This pathway plays a crucial role in immune cell activation, inflammation, and cellular responses to growth factors and hormones.
- Notch Pathway: The Notch signaling pathway is crucial for cell fate determination and development. Notch receptors and ligands are transmembrane proteins, and their interaction triggers proteolytic cleavage and release of the Notch intracellular domain (NICD). NICD translocates to the nucleus, where it interacts with transcription factors and regulates gene expression, influencing cell differentiation and tissue patterning.
These are just a few examples of the diverse and complex signal transduction pathways that exist in cells. Each pathway plays a specific role in transmitting and interpreting signals to orchestrate various cellular processes and ensure proper cell function and development.
Describe the Insulin signal transduction pathway
The insulin signal transduction pathway is an important signaling cascade that occurs in response to insulin binding to its receptor on the surface of target cells. It regulates various metabolic and growth-related processes in the body. Here is an overview of the insulin signal transduction pathway:
- Insulin Binding: Insulin, a hormone secreted by the pancreas, binds to the insulin receptor (INSR) located on the cell surface of target cells. The insulin receptor is a receptor tyrosine kinase, meaning it has an intrinsic kinase activity.
- Receptor Activation: Insulin binding triggers autophosphorylation of the insulin receptor. This autophosphorylation activates the kinase activity of the receptor, leading to the phosphorylation of specific tyrosine residues on the receptor itself.
- Insulin Receptor Substrate (IRS) Recruitment: Phosphorylated tyrosine residues on the activated insulin receptor provide docking sites for insulin receptor substrates (IRS). IRS proteins are adaptor molecules that bind to the phosphorylated receptor and serve as scaffolds for downstream signaling molecules.
- Activation of PI3K/Akt Pathway: IRS proteins recruit and activate phosphatidylinositol 3-kinase (PI3K). PI3K converts phosphatidylinositol 4,5-bisphosphate (PIP2) into phosphatidylinositol 3,4,5-trisphosphate (PIP3). PIP3 serves as a second messenger that recruits and activates protein kinase B (PKB/Akt) at the plasma membrane.
- Akt Activation: Once recruited to the plasma membrane, Akt is phosphorylated and activated by phosphoinositide-dependent kinase 1 (PDK1) and mammalian target of rapamycin complex 2 (mTORC2). Activated Akt then phosphorylates and modulates the activity of various downstream targets.
- Glucose Uptake: Akt promotes the translocation of glucose transporter 4 (GLUT4) vesicles to the plasma membrane in muscle and adipose cells. This facilitates glucose uptake from the bloodstream into the cells, leading to decreased blood glucose levels.
- Glycogen Synthesis: Akt activation also stimulates glycogen synthesis by promoting the activation and inhibition of key enzymes involved in glycogen metabolism. This results in the conversion of glucose into glycogen, which is stored in the liver and muscles.
- Protein Synthesis and Growth: Akt signaling also promotes protein synthesis by activating the mammalian target of rapamycin complex 1 (mTORC1) pathway. This pathway stimulates protein translation and cell growth by regulating various translational factors and ribosomal proteins.
Overall, the insulin signal transduction pathway plays a crucial role in regulating glucose metabolism, glycogen synthesis, and protein synthesis. Dysregulation of this pathway can lead to insulin resistance and contribute to the development of conditions such as type 2 diabetes.
What is epinephrine signal transduction pathway
The epinephrine (adrenaline) signal transduction pathway is an important pathway involved in the body’s response to stress and regulation of various physiological processes. Here is a simplified overview of the epinephrine signal transduction pathway:
- Ligand Binding: Epinephrine binds to and activates G-protein coupled receptors (GPCRs) called adrenergic receptors, specifically the beta-adrenergic receptors.
- Activation of G-proteins: Binding of epinephrine to the beta-adrenergic receptor leads to the activation of G-proteins. In this case, the G-protein involved is the Gs protein, which stimulates adenylate cyclase.
- cAMP Production: Activated adenylate cyclase converts adenosine triphosphate (ATP) into cyclic adenosine monophosphate (cAMP), a secondary messenger molecule.
- Activation of Protein Kinase A (PKA): The increased levels of cAMP lead to the activation of protein kinase A (PKA) by dissociating the regulatory subunits from the catalytic subunits of PKA.
- Phosphorylation of Targets: Activated PKA phosphorylates various target proteins, including ion channels, enzymes, and transcription factors, leading to downstream effects.
- Cellular Responses: The phosphorylation of target proteins by PKA regulates various cellular processes, such as glycogen breakdown, increased heart rate and contractility, smooth muscle relaxation, and enhanced lipolysis.
It’s important to note that the epinephrine signaling pathway is more complex and can have different effects depending on the cell type and receptor subtype involved. Additionally, there are feedback mechanisms and cross-talk with other signaling pathways that can modulate the overall response. Nevertheless, this simplified overview provides a general understanding of the key steps involved in the epinephrine signal transduction pathway and its impact on cellular responses.
How can signal transduction pathways be amplified?
Signal transduction pathways can be amplified through various mechanisms to enhance the cellular response to a given signal. Here are a few ways in which signal transduction pathways can be amplified:
- Enzymatic Amplification: Many signal transduction pathways involve enzymes that can catalyze multiple reactions, leading to signal amplification. For example, in the cyclic AMP (cAMP) pathway, a single activated adenylate cyclase enzyme can produce numerous cAMP molecules from ATP, amplifying the signal.
- Cascade Amplification: Some signaling pathways involve cascades of sequential events, where each step activates multiple downstream components. This cascade amplifies the signal because each activated molecule can trigger the activation of multiple molecules in the subsequent steps. The mitogen-activated protein kinase (MAPK) pathway is an example of a cascade amplification mechanism, where a series of kinases activate each other in a phosphorylation cascade, leading to signal amplification.
- Positive Feedback Loops: Positive feedback loops occur when a signaling event enhances its own occurrence. This mechanism can lead to signal amplification. For example, in certain signal transduction pathways, the activation of a downstream component can stimulate the activation of upstream components, reinforcing and amplifying the initial signal.
- Recruitment of Multiple Effectors: Some signaling pathways involve the recruitment of multiple effectors downstream of a receptor. Each effector can amplify the signal by independently generating second messengers or activating downstream signaling events, leading to a cumulative effect on the overall signal.
- Signal Integration: Cells can integrate signals from multiple pathways or multiple receptors to amplify the response. When different signaling pathways converge or interact, the combined effect can amplify the overall signal and enhance the cellular response.
- Receptor Upregulation: Cells can upregulate the expression or availability of receptors for a specific ligand, leading to an increased sensitivity and responsiveness to the signal. This upregulation can amplify the cellular response to a given stimulus.
It’s important to note that these mechanisms are not mutually exclusive and can act in combination to amplify signals in different contexts and cell types. The amplification of signal transduction pathways allows cells to generate robust and appropriate responses to external signals, ensuring efficient and effective cellular communication and adaptation.
How does a chemical signal trigger the signal transduction pathway
A chemical signal triggers a signal transduction pathway by binding to a specific receptor molecule on the cell surface or within the cell. This binding event initiates a series of intracellular events that transmit and amplify the signal, ultimately leading to a cellular response. Here’s a general overview of how a chemical signal triggers a signal transduction pathway:
- Receptor Binding: The chemical signal, which can be a hormone, neurotransmitter, growth factor, or other signaling molecule, encounters and binds to its specific receptor molecule. The receptor can be located on the cell surface or inside the cell, depending on the nature of the signal.
- Conformational Changes: Upon binding of the chemical signal, the receptor undergoes conformational changes. These changes can occur in the extracellular domain of cell surface receptors or the ligand-binding domain of intracellular receptors.
- Activation of Receptor: The conformational changes in the receptor lead to its activation, triggering a series of events that transmit the signal to the intracellular environment. This activation can involve dimerization or oligomerization of receptors, exposure of functional domains, or alteration in receptor phosphorylation state, among other mechanisms.
- Recruitment of Signaling Molecules: The activated receptor recruits and binds to specific signaling molecules or adaptor proteins, often through protein-protein interaction domains or phosphorylation sites. These signaling molecules serve as mediators or transducers, relaying the signal to downstream components.
- Activation of Downstream Pathways: The recruited signaling molecules transmit the signal to downstream components, often through enzymatic reactions or protein-protein interactions. This activation can involve the generation of second messengers, such as cyclic AMP (cAMP) or calcium ions, activation of protein kinases, or modulation of gene transcription.
- Amplification and Propagation: Downstream components of the pathway further amplify and propagate the signal by activating additional signaling molecules or participating in feedback loops. This amplification ensures that even weak or transient signals can trigger a robust cellular response.
- Cellular Response: The propagated signal ultimately reaches target molecules, such as enzymes, ion channels, transcription factors, or structural proteins, which undergo specific modifications or changes in activity. These modifications lead to the cellular response associated with the initial chemical signal, such as changes in gene expression, metabolism, cell proliferation, differentiation, or cell movement.
How does testosterone carry out signal transduction pathways
Testosterone, as a steroid hormone, carries out signal transduction pathways by binding to intracellular receptors known as androgen receptors (ARs). Here’s a general overview of how testosterone triggers signal transduction:
- Diffusion across Cell Membrane: Testosterone is a lipophilic hormone, allowing it to easily diffuse across the cell membrane without requiring a specific receptor on the cell surface.
- Binding to Androgen Receptor: Once inside the cell, testosterone binds to the androgen receptor (AR), which is typically located in the cytoplasm in an inactive state, complexed with heat shock proteins (HSPs).
- Dissociation of Heat Shock Proteins: Testosterone binding causes a conformational change in the AR, leading to the dissociation of heat shock proteins and exposure of the hormone-binding domain.
- Translocation to the Nucleus: The ligand-bound AR undergoes translocation from the cytoplasm to the nucleus, where it interacts with specific DNA sequences known as androgen response elements (AREs) located in the promoter regions of target genes.
- Transcriptional Regulation: Once bound to AREs, the activated AR acts as a transcription factor, recruiting co-regulatory proteins and initiating transcription of target genes. This process leads to changes in gene expression patterns and the synthesis of proteins that mediate the cellular response to testosterone.
- Co-regulatory Protein Interactions: The ligand-bound AR interacts with co-regulatory proteins, such as co-activators or co-repressors, to modulate the transcriptional activity of target genes. These interactions can enhance or suppress gene expression, depending on the specific context.
- Cellular Response: The altered gene expression profiles induced by the testosterone-AR complex regulate various cellular processes, including sexual development, reproductive function, muscle growth, bone density, and secondary sexual characteristics.
It’s important to note that the testosterone-AR signaling pathway is complex and can interact with other signaling pathways, such as growth factor signaling, to achieve integrated responses. Additionally, the AR is present in various cell types throughout the body, contributing to the diverse physiological effects of testosterone.
in eukaryotic cells signal transduction pathways involve
In eukaryotic cells, signal transduction pathways involve several key components and processes. Here are some common elements and processes involved in signal transduction pathways:
- Receptors: Eukaryotic cells have various types of receptors that recognize specific signaling molecules or ligands. These receptors can be located on the cell surface or within the cell, depending on the nature of the signal.
- Ligand Binding: When a signaling molecule or ligand binds to its specific receptor, it triggers a conformational change in the receptor, leading to its activation.
- Signal Amplification: Signal amplification mechanisms, such as enzymatic cascades or positive feedback loops, increase the strength or magnitude of the signal, ensuring an effective cellular response.
- Second Messengers: Second messengers are small molecules that act as intermediaries in signal transduction pathways. Examples of second messengers include cyclic AMP (cAMP), calcium ions (Ca2+), and inositol trisphosphate (IP3). Second messengers relay signals from the receptor to downstream components, often by activating or inhibiting specific proteins or enzymes.
- Protein Kinases: Protein kinases play a crucial role in signal transduction pathways. They are responsible for phosphorylating target proteins, usually by transferring a phosphate group from ATP to specific amino acid residues, such as serine, threonine, or tyrosine. Phosphorylation events can modify the activity, localization, or interactions of target proteins, leading to downstream signaling events.
- Phosphatases: Phosphatases are enzymes that catalyze the removal of phosphate groups from proteins. They act as negative regulators, counterbalancing the action of protein kinases and helping to terminate or attenuate signaling events.
- Transcription Factors: Signaling pathways often converge on transcription factors, which are proteins that regulate gene expression. Activated transcription factors translocate to the nucleus, where they bind to specific DNA sequences, known as response elements, and modulate the transcription of target genes.
- Cellular Responses: The activation of signal transduction pathways leads to specific cellular responses, which can include changes in gene expression, protein synthesis, enzyme activity, cell proliferation, differentiation, migration, or apoptosis. The exact response depends on the specific pathway, ligand, receptor, and cellular context.
It’s important to note that signal transduction pathways can be highly complex and interconnected, involving cross-talk between different pathways and feedback regulation to maintain cellular homeostasis. The specific components and processes involved in a signal transduction pathway vary depending on the particular signaling system and cellular context.
What is gpcr signal transduction pathway
The G-protein coupled receptor (GPCR) signal transduction pathway is a common and versatile mechanism used by cells to relay extracellular signals and initiate intracellular responses. Here is a simplified overview of the GPCR signal transduction pathway:
- Ligand Binding: The pathway is initiated when a specific ligand, such as a hormone or neurotransmitter, binds to the extracellular domain of a GPCR located on the cell surface.
- Conformational Change and G-protein Activation: Ligand binding induces a conformational change in the GPCR, leading to the activation of an associated heterotrimeric G-protein. The G-protein consists of three subunits: alpha (α), beta (β), and gamma (γ).
- G-protein Activation and Signal Transduction: Upon activation, the G-protein undergoes a conformational change that allows the exchange of GDP (guanosine diphosphate) bound to the alpha subunit with GTP (guanosine triphosphate). This GTP-bound alpha subunit dissociates from the beta-gamma subunits and becomes active.
- Effector Activation: The activated alpha subunit or beta-gamma subunits of the G-protein go on to modulate the activity of effector proteins, which can be enzymes or ion channels. The specific effector depends on the type of G-protein involved.
- Generation of Second Messengers: Activation of the effector can lead to the generation of second messengers such as cyclic adenosine monophosphate (cAMP), inositol trisphosphate (IP3), and diacylglycerol (DAG). These second messengers mediate downstream signaling events.
- Activation of Protein Kinases: Second messengers or other signaling molecules produced in the pathway can activate protein kinases, such as protein kinase A (PKA) or protein kinase C (PKC). These kinases phosphorylate target proteins, leading to cellular responses.
- Cellular Responses: The phosphorylation and activation of target proteins by protein kinases ultimately regulate various cellular processes, including gene expression, ion channel activity, enzyme activity, and cytoskeletal rearrangements, resulting in specific physiological responses.
It’s important to note that GPCR signal transduction pathways can vary depending on the specific GPCR, ligand, and downstream effectors involved. Additionally, there can be cross-talk with other signaling pathways and feedback mechanisms that modulate the overall response. Nevertheless, this simplified overview provides a general understanding of the key steps involved in the GPCR signal transduction pathway.
What is EGF signal transduction pathway
The epidermal growth factor (EGF) signal transduction pathway is a well-studied pathway that regulates cell growth, proliferation, and differentiation. Here is a simplified overview of the EGF signal transduction pathway:
- Ligand Binding: The pathway is initiated when the EGF ligand binds to the epidermal growth factor receptor (EGFR), a receptor tyrosine kinase located on the cell surface.
- Receptor Dimerization: Ligand binding induces conformational changes in EGFR, leading to the dimerization of two EGFR monomers.
- Autophosphorylation: Dimerized EGFR molecules phosphorylate specific tyrosine residues within their intracellular domains. This autophosphorylation step activates the tyrosine kinase activity of EGFR and creates docking sites for downstream signaling molecules.
- Recruitment of Adaptor Proteins: Phosphorylated tyrosine residues on EGFR attract adaptor proteins, such as GRB2 (growth factor receptor-bound protein 2), to the receptor complex.
- Activation of Ras: GRB2, together with the guanine nucleotide exchange factor SOS (son of sevenless), facilitates the activation of Ras, a small GTPase protein. This activation involves the exchange of GDP for GTP on Ras.
- Activation of MAPK Pathway: Active Ras triggers a phosphorylation cascade that activates the mitogen-activated protein kinase (MAPK) pathway. Sequentially, Ras activates Raf, which activates MEK (MAPK/ERK kinase), leading to the activation of ERK (extracellular signal-regulated kinase).
- Translocation to the Nucleus: Active ERK translocates to the nucleus, where it phosphorylates various transcription factors, such as Elk-1, c-Jun, and c-Myc. This phosphorylation modifies the activity of these transcription factors, leading to changes in gene expression.
- Cellular Responses: The altered gene expression profiles induced by the EGF signal transduction pathway regulate various cellular processes, including cell growth, proliferation, differentiation, and survival.
It’s important to note that the EGF signal transduction pathway is more complex than described here, involving additional feedback mechanisms and cross-talk with other signaling pathways. However, this simplified overview outlines the key steps in the EGF signaling cascade and its impact on cellular responses.
The most rapid signal transduction pathways cause
The most rapid signal transduction pathways in cells typically involve direct signaling through ion channels or G-protein coupled receptors (GPCRs). These pathways can elicit rapid responses within milliseconds to seconds. Here are two examples:
- Ion Channel Signaling: Ion channels are proteins that allow the passage of specific ions across the cell membrane. They can be rapidly activated or inhibited by various signals. For instance, neurotransmitters such as glutamate can bind to ionotropic receptors, which are ion channels themselves or closely associated with ion channels. This binding triggers a conformational change in the receptor, leading to the rapid opening or closing of ion channels and the subsequent flux of ions across the membrane. This rapid change in ion concentration can cause immediate changes in the cell’s membrane potential, leading to fast electrical signaling in neurons and muscle cells.
- G-Protein Coupled Receptor (GPCR) Signaling: GPCRs are a large family of cell surface receptors that transmit signals from a wide range of stimuli, including hormones, neurotransmitters, and sensory stimuli. When activated by their specific ligands, GPCRs interact with G-proteins, which are intracellular signaling molecules. This interaction leads to the rapid activation or inhibition of effector proteins such as enzymes or ion channels. For example, the binding of adrenaline (epinephrine) to the β-adrenergic receptor activates the G-protein Gs, which stimulates the enzyme adenylate cyclase. Adenylate cyclase rapidly converts ATP into cyclic AMP (cAMP), a second messenger that can rapidly initiate intracellular signaling events, such as the activation of protein kinase A (PKA). The whole process can occur within seconds, enabling a rapid cellular response.
It’s important to note that the speed of a signal transduction pathway depends on various factors, including the nature of the signal, the receptors involved, and the downstream components of the pathway. Different signaling pathways can operate at different time scales, ranging from milliseconds to minutes or even longer, depending on the complexity and intricacy of the cellular response.
What role do phosphatases play in signal transduction pathways?
Phosphatases play a critical role in signal transduction pathways by regulating the phosphorylation status of proteins. They are enzymes that catalyze the removal of phosphate groups from phosphorylated proteins or other molecules. This dephosphorylation process is essential for modulating the activity, localization, and duration of signaling events within the cell. Here are some key roles of phosphatases in signal transduction pathways:
- Signal termination: Phosphatases help in terminating the signaling cascade by dephosphorylating activated proteins. Once a signal has been transmitted and the desired response has been achieved, phosphatases remove the phosphate groups from key signaling molecules, effectively switching off the signaling pathway. This helps restore the system to its basal state and prevents prolonged activation that could be detrimental to the cell.
- Signal regulation and fine-tuning: Phosphatases play a role in regulating the amplitude and duration of signaling responses. By dephosphorylating proteins involved in signal transduction, phosphatases can attenuate or dampen the signal, ensuring a controlled and precise cellular response. This allows cells to modulate the intensity and duration of signaling events according to specific requirements.
- Cross-talk and integration: In complex signaling networks, different pathways often intersect and interact with each other. Phosphatases contribute to this cross-talk by dephosphorylating proteins that are involved in multiple signaling pathways. By removing phosphates from shared signaling molecules, phosphatases influence the integration of signals from different pathways, allowing cells to coordinate multiple signals and generate appropriate responses.
- Feedback regulation: Phosphatases can be part of negative feedback loops that regulate the activity of upstream signaling components. For example, a downstream signaling molecule can activate a phosphatase that dephosphorylates and inhibits an upstream signaling molecule. This feedback inhibition helps maintain a balance in the signaling network and prevents excessive activation or signaling flux.
Overall, phosphatases play a crucial role in regulating the phosphorylation state of proteins, which is a fundamental mechanism in signal transduction pathways. By dephosphorylating key signaling molecules, they modulate the strength, duration, and specificity of cellular responses, contributing to the fine-tuning and regulation of signal transduction events.
What are the functions of signal transduction pathways?
Signal transduction pathways serve several important functions in cellular processes. Here are some key functions of signal transduction pathways:
- Transmitting extracellular signals: Signal transduction pathways enable cells to receive and interpret signals from the extracellular environment. These signals can come from various sources, such as hormones, growth factors, neurotransmitters, or environmental cues. The pathways convert these signals into intracellular responses, allowing cells to adapt and respond to changes in their surroundings.
- Amplifying and integrating signals: Signal transduction pathways often involve amplification mechanisms, where a single extracellular signal can trigger a cascade of intracellular events, resulting in a robust response. This amplification step ensures that the signal is effectively transmitted and translated into appropriate cellular responses. Additionally, signal transduction pathways can integrate multiple signals, allowing cells to respond to complex stimuli and adjust their behavior accordingly.
- Regulating gene expression: Many signal transduction pathways regulate gene expression, influencing the transcription of specific genes. This regulation can lead to the synthesis of proteins that are critical for various cellular processes, including growth, differentiation, metabolism, and cell survival. By modulating gene expression, signal transduction pathways play a vital role in controlling cellular behavior and coordinating responses to extracellular cues.
- Controlling cellular processes: Signal transduction pathways are involved in regulating a wide range of cellular processes. These processes include cell growth, proliferation, differentiation, migration, metabolism, apoptosis (programmed cell death), and response to stress or injury. Through the activation or inhibition of specific proteins and enzymes, signal transduction pathways orchestrate these cellular processes and maintain cellular homeostasis.
- Modulating cell-cell communication: Signal transduction pathways contribute to cell-cell communication by regulating the release and response to signaling molecules. For example, neurotransmitters released from one neuron can activate signal transduction pathways in another neuron, allowing for the transmission of signals in the nervous system. Similarly, in multicellular organisms, signal transduction pathways mediate communication between different cell types, coordinating their activities and enabling proper tissue and organ function.
- Mediating developmental processes: Signal transduction pathways play a critical role in embryonic development, tissue morphogenesis, and organogenesis. They guide cell fate decisions, cell migration, and tissue patterning, ensuring the proper formation and organization of tissues and organs during development. By regulating the expression of specific genes and coordinating cellular behaviors, signal transduction pathways contribute to the complex processes of growth and differentiation.
Overall, signal transduction pathways are essential for relaying and processing extracellular signals, coordinating cellular responses, and maintaining cellular and organismal homeostasis. They provide a means for cells to sense and adapt to their environment, enabling them to function and respond appropriately to various physiological and pathological stimuli.
How to read signal transduction diagrams, what does normal arrow and flathead arrow means.
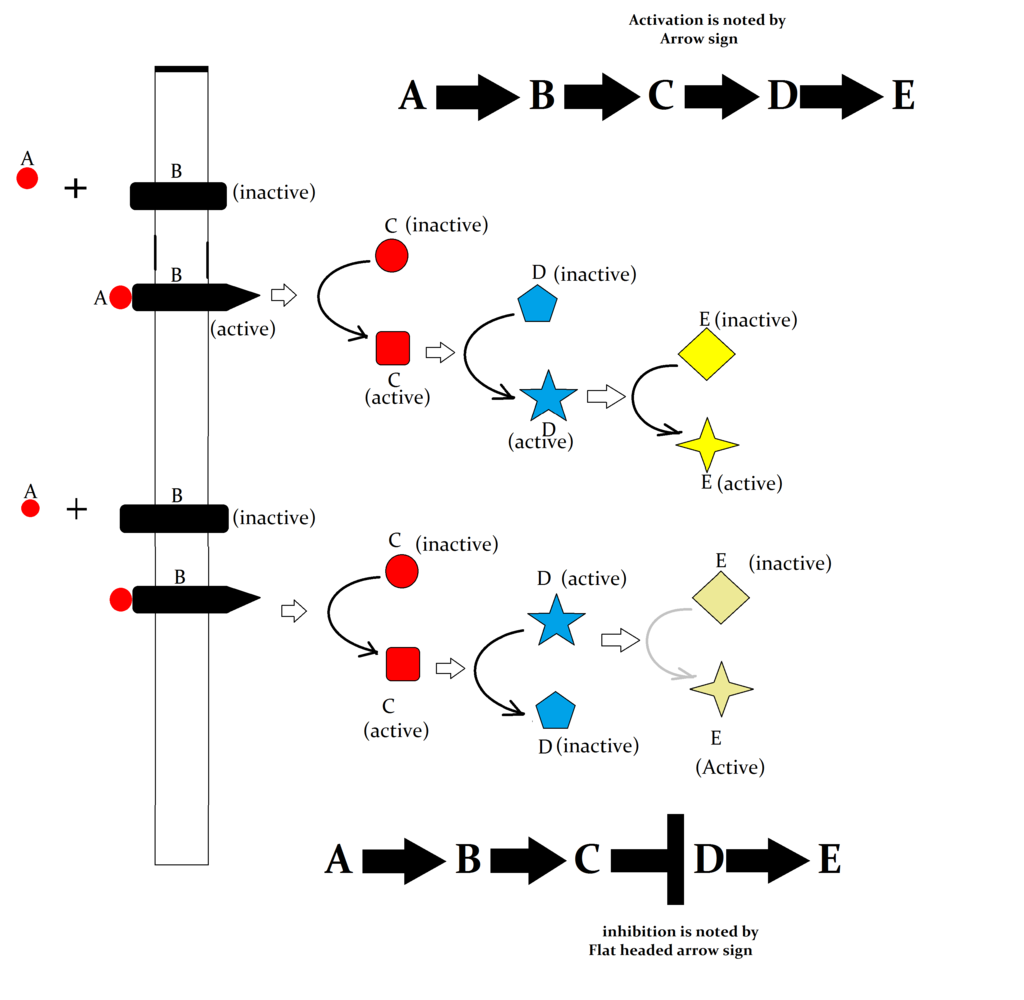
Elements of Signal transduction cascade networking
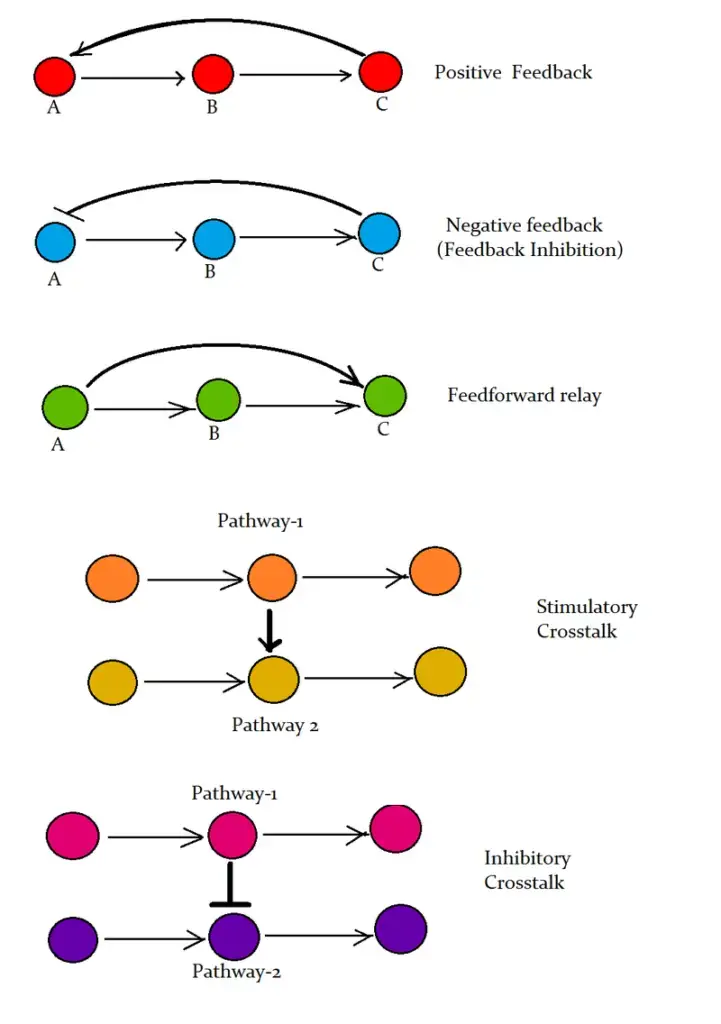
Signal Transduction Pathway Worksheet
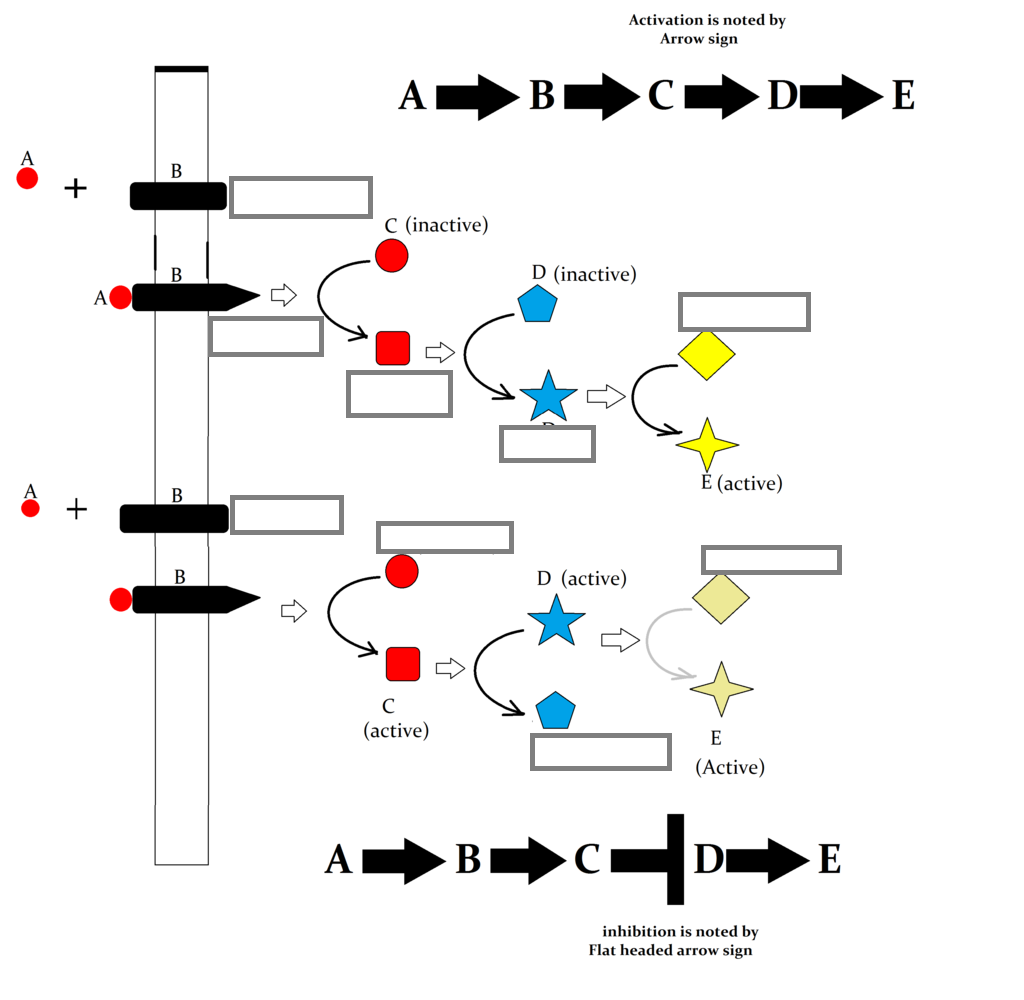
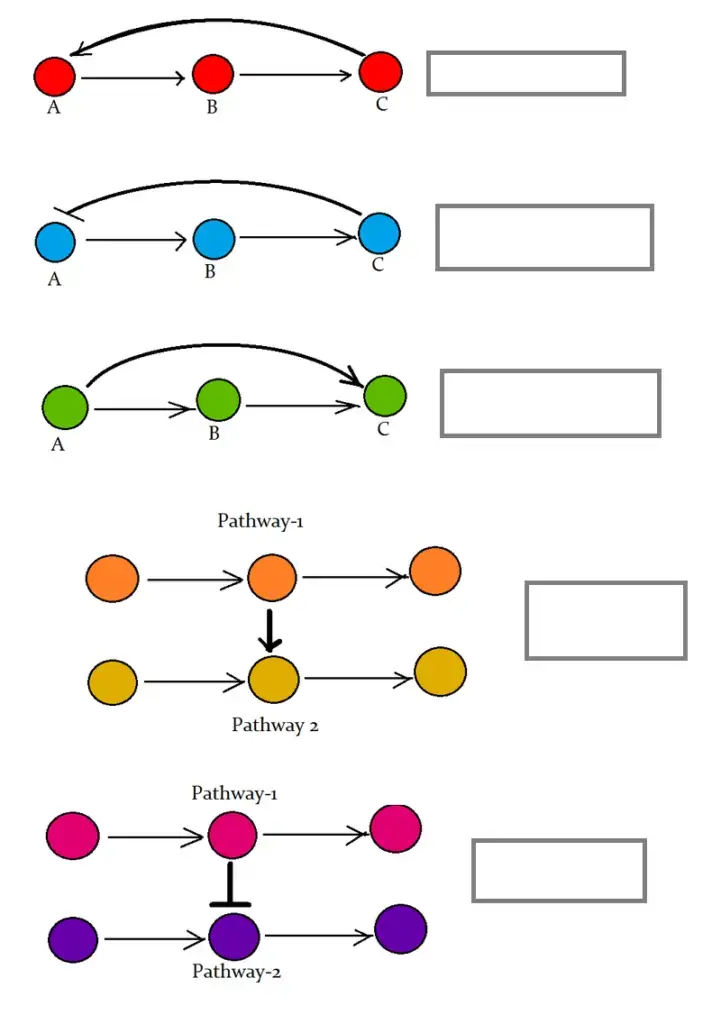
FAQ
What is a signal transduction pathway?
A signal transduction pathway is a series of biochemical events that occur within a cell in response to an external signal, such as a hormone, growth factor, or neurotransmitter. It involves the conversion of an extracellular signal into an intracellular response.
Why are signal transduction pathways important?
Signal transduction pathways allow cells to respond and adapt to changes in their environment. They regulate various cellular processes, including gene expression, metabolism, growth, differentiation, and cell survival. They play a crucial role in development, homeostasis, immune responses, and many other physiological processes.
How are signal transduction pathways initiated?
Signal transduction pathways are initiated when a signaling molecule or ligand binds to a specific receptor on the cell surface or within the cell. This binding event triggers a series of intracellular events that transmit and amplify the signal.
What are second messengers in signal transduction pathways?
Second messengers are small molecules that relay signals from the receptor to downstream components in a signal transduction pathway. Examples of second messengers include cyclic AMP (cAMP), calcium ions (Ca2+), inositol trisphosphate (IP3), and diacylglycerol (DAG). They help propagate the signal and regulate the activity of downstream effectors.
How do protein kinases and phosphatases regulate signal transduction pathways?
Protein kinases are enzymes that add phosphate groups to proteins, while phosphatases remove phosphate groups. Protein kinases often play a role in activating or propagating the signal by phosphorylating target proteins, which can lead to changes in their activity or function. Phosphatases act as negative regulators by dephosphorylating proteins, helping to terminate or attenuate signaling events.
How do cells ensure signal specificity in transduction pathways?
Cells employ multiple mechanisms to ensure signal specificity in transduction pathways. These include specific receptor-ligand interactions, compartmentalization of signaling components, selective activation of downstream effectors, and cross-talk between different signaling pathways. These mechanisms help cells respond appropriately to different signals and prevent unwanted activation or interference.
What is the role of feedback regulation in signal transduction pathways?
Feedback regulation mechanisms are essential for maintaining the balance and fine-tuning of signal transduction pathways. Negative feedback loops can dampen or terminate the signaling response once a desired cellular outcome is achieved. Positive feedback loops can amplify the signal or reinforce the response. Feedback regulation helps cells maintain stability, prevent excessive activation, and ensure precise control over signaling events.
How do signal transduction pathways interact with the cellular nucleus?
Signal transduction pathways can transmit signals to the cellular nucleus by activating transcription factors. These transcription factors can directly bind to specific DNA sequences and regulate gene expression, leading to long-term changes in cellular behavior and function. This nuclear signaling allows for the coordination of complex cellular responses.
What is cross-talk between signal transduction pathways?
Cross-talk refers to the communication and interaction between different signal transduction pathways. Pathways can converge, share common components, or influence each other’s activities. Cross-talk allows for integration of signals from multiple pathways, coordination of cellular responses, and modulation of signaling outcomes.
How can dysregulation of signal transduction pathways contribute to diseases?
Dysregulation of signal transduction pathways can contribute to various diseases. Aberrant activation or inhibition of signaling pathways can disrupt normal cellular processes, leading to conditions such as cancer, metabolic disorders, immune disorders, neurological disorders, and cardiovascular diseases. Understanding and targeting these dysregulated pathways are important for developing therapeutic interventions.
References
- http://bcs.whfreeman.com/webpub/biology/sadavalife9e/animated%20tutorials/life9e_0701_script.html
- https://www.ck12.org/biology/signal-transduction/lesson/signal-transduction-advanced-bio-adv/
- https://aklectures.com/lecture/signal-transduction-pathways/common-properties-of-signal-pathways
- https://www.cshlpress.com/default.tpl?action=full&–eqskudatarq=1101
- https://www.deshbandhucollege.ac.in/pdf/resources/1587027260_BT(H)-VI-L-4.pdf
- https://www.sciencedirect.com/topics/medicine-and-dentistry/signal-transduction-pathway
- https://biologydictionary.net/signal-transduction/
- https://www.studysmarter.co.uk/explanations/biology/cell-communication/signal-transduction-pathways/
- https://www.rndsystems.com/pathways/signal-transduction