What are Prions?
- Prions are distinct, misfolded proteins that induce other proteins of the same type to adopt an abnormal structure, ultimately leading to cellular damage and death. Unlike traditional infectious agents such as bacteria, viruses, or fungi, prions lack nucleic acids, which are typically required for replication. The term “prion” is derived from “proteinaceous infectious particle,” highlighting their unique nature.
- Prions are essentially misfolded forms of a normal protein known as the prion protein (PrP). The normal variant, PrPc (cellular prion protein), is believed to have a role in cell signaling and neuroprotection, although its precise function remains uncertain. In contrast, the pathogenic variant, PrPsc (scrapie prion protein), has a misfolded, aggregated structure that can convert normal PrPc into the disease-associated form. This misfolded state leads to the formation of amyloid fibrils, which accumulate and disrupt normal cellular processes.
- Prions are implicated in a range of fatal neurodegenerative diseases collectively referred to as transmissible spongiform encephalopathies (TSEs). These include scrapie in sheep, chronic wasting disease (CWD) in deer, bovine spongiform encephalopathy (BSE) in cattle, and Creutzfeldt-Jakob disease (CJD) in humans. The diseases caused by prions are characterized by progressive neurological deterioration and are invariably fatal, with no effective treatments currently available.
- The structural stability of prions, coupled with their resistance to conventional sterilization methods, presents significant challenges for containment and disposal. This resilience raises concerns about potential iatrogenic transmission, where prions could be spread through contaminated medical instruments or tissues.
- Besides the classic prion diseases, prions or prion-like mechanisms have been linked to other neurodegenerative disorders, such as Alzheimer’s disease, Parkinson’s disease, and amyotrophic lateral sclerosis (ALS). These conditions, while not caused by prions per se, exhibit similar pathological features related to protein misfolding and aggregation.
- The harmful conformations of prions, particularly PrPsc, differ from their normal counterparts primarily in their secondary and tertiary structures. While no chemical differences between PrPc and PrPsc have been identified, their interactions with cellular components, such as plasminogen, reveal functional distinctions. Plasminogen, which is involved in synaptic remodeling and memory processes, is converted into plasmin by specialized cell membrane factors associated with normal PrPc. The abnormal prion structure disrupts this process, contributing to neuronal damage and disease progression.
Definition of Prion
A prion is an infectious, misfolded protein that causes neurodegenerative diseases by inducing abnormal folding of normal cellular proteins, leading to the accumulation of these misfolded proteins in the brain. Unlike other infectious agents, prions lack nucleic acids and propagate their abnormal structure through direct protein-protein interactions.
Characteristics of Prion Protein
- Structural Variants: Prions exist in two main forms: the normal prion protein (PrPc) and the abnormal, disease-causing prion protein (PrPsc). PrPc consists predominantly of alpha helices, whereas PrPsc features a high content of beta-sheets, leading to its pathogenic properties.
- Resistance to Degradation: PrPsc is notably resistant to proteolytic enzymes, heat, ionizing radiation, and standard chemical disinfectants like formaldehyde. This resistance complicates the inactivation and containment of prions.
- Lack of Nucleic Acids: Unlike other infectious agents, prions do not contain nucleic acids (DNA or RNA). This absence makes them impervious to treatments aimed at destroying nucleic acids, such as those used for sterilizing conventional pathogens.
- Immunological Characteristics: Prions do not provoke a conventional immune response because they are aberrant versions of a normal protein. This property allows them to evade detection and clearance by the host’s immune system.
- Aggregation and Detection: Prions are typically visible under an electron microscope only when they have aggregated into larger clusters. These aggregates are critical to their pathogenicity.
- Transmission and Contamination: Prions can be transmitted through various routes, including airborne particles such as aerosols. They are also resistant to conventional sterilization methods but can be decontaminated through specific procedures such as protein hydrolysis, the use of sodium hypochlorite, sodium hydroxide, and strongly acidic detergents. Ozone sterilization is also being explored as a potential method for prion inactivation.
- Genetic and Environmental Factors: Prion diseases can arise from genetic mutations in the PrP gene, leading to inherited forms of the disease. Additionally, prions can spread through environmental exposure, such as contaminated food or medical procedures.
- Differential Enzyme Susceptibility: While PrPc and PrPsc share no chemical differences, their interaction with cellular components, such as plasminogen, varies significantly. PrPsc disrupts normal plasminogen processing, contributing to the pathological effects of prion diseases.
- Species-Specific Impact: Prion diseases affect various species differently. For instance, BSE in cattle and scrapie in sheep both involve prions but exhibit distinct pathological and clinical features. Transmission can occur through contaminated feed or direct contact, with different species showing varying susceptibility and symptomatology.
Structure of Prion Protein
- Normal Prion Protein (PrPc)
- Signal Peptide: PrPc begins with a signal peptide (amino acids 1–22), which is essential for the protein’s translocation into the endoplasmic reticulum.
- Octapeptide Repeats: The protein contains five octapeptide repeats (amino acids 51–91) with the sequence PHGGGWGQ. These repeats are notable for their ability to bind copper ions, which may influence cellular processes such as endocytosis.
- Hydrophobic Domain: A highly conserved hydrophobic domain (amino acids 106–126) anchors PrPc to the cell membrane.
- Secondary Structure: PrPc predominantly features alpha-helices (α1–α3), contributing to its normal, soluble conformation.
- Glycosylphosphatidylinositol (GPI) Anchor: At the C-terminus (amino acids 231–253), PrPc includes a GPI anchor that attaches the protein to the cell membrane.
- Glycosylation: Two consensus sequences (T181 and T197) are present for N-linked glycosylation, resulting in various glycosylated forms of PrPc.
- Disulfide Bond: A critical disulfide bond between cysteine residues at positions 179 and 214 is vital for the correct folding and stability of PrPc.
- Abnormal Prion Protein (PrPSc)
- Structural Differences: PrPSc, the pathogenic form, differs from PrPc by having a higher proportion of beta-sheets compared to the normal alpha-helical content. This structural alteration leads to the formation of amyloid fibrils.
- Aggregation and Amyloid Formation: PrPSc aggregates into amyloid fibers, which consist of stacked PrP molecules linked via intermolecular beta-sheets. These fibrils can form plaques outside cells and are associated with prion diseases.
- Resistance to Proteases: Unlike PrPc, PrPSc is resistant to proteolytic enzymes like proteinase K, contributing to its persistence and pathogenicity.
- Interaction with Cellular Membranes: PrPSc may bind to cellular membranes via its glycolipid anchors or accumulate as extracellular plaques. This interaction involves a complex array of glycans and lipid components.
- Recombinant Proteins
- Doppel: The recombinant Doppel protein has a tertiary structure similar to the C-terminal domain of PrPc but lacks the N-terminal hydrophobic and octapeptide regions. It is involved in neuroprotection against toxic prion forms.
- Shadoo: Shadoo, another related protein, is expressed in the central nervous system and provides protection against neurotoxic effects of prions and truncated PrPc forms.
- PrPres (Protease-Resistant PrP)
- Definition: PrPres refers to any isoform of PrPc that has been converted into a misfolded, protease-resistant form. It may or may not be infectious.
- Conversion and Amplification: The conversion of PrPc to PrPres can be modeled in vitro and involves cyclic amplification techniques that sustain prion infectivity.
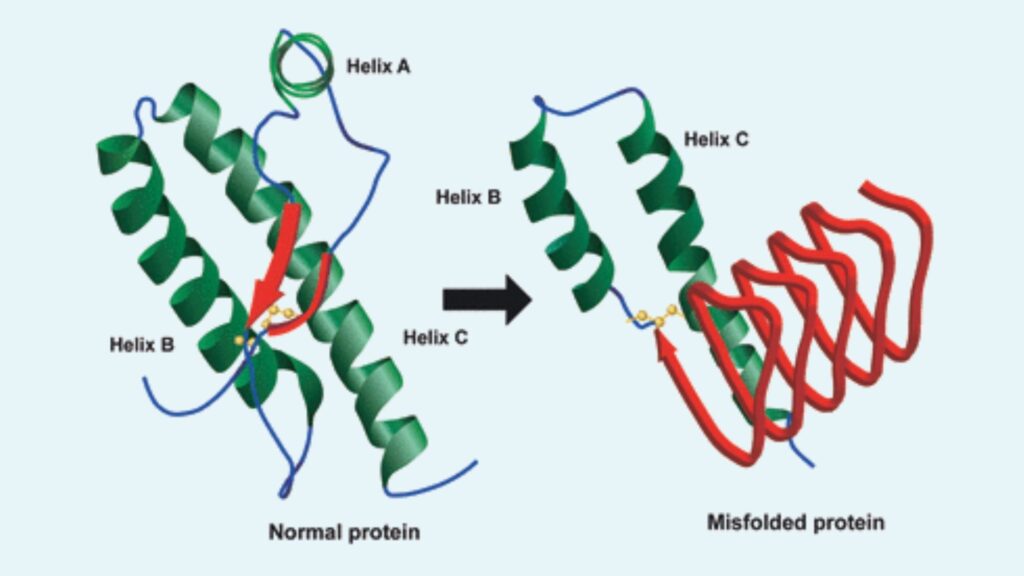
Replication of Prions
The replication of prions has intrigued scientists for decades, primarily because it does not involve nucleic acids. Below is an outline of the mechanisms proposed to explain prion replication, focusing on the conversion of normal prion protein (PrPC) into its pathogenic form (PrPSc).
1. Heterodimer Model
- Description: One of the earliest hypotheses to explain prion replication was the heterodimer model. This model proposes that a single PrPSc molecule binds to a single PrPC molecule.
- Mechanism: When PrPSc binds to PrPC, it catalyzes the conversion of PrPC into PrPSc. The two PrPSc molecules then dissociate, allowing them to interact with additional PrPC molecules.
- Limitation: The heterodimer model falls short in explaining the efficiency of prion propagation. For this model to work effectively, PrPSc would need to act as an extraordinarily potent catalyst, increasing the reaction rate by a factor of 10^15. This degree of catalytic activity is rarely observed. Additionally, infectious monomeric PrPSc has never been isolated, which weakens the validity of this model.
2. Fibril Model
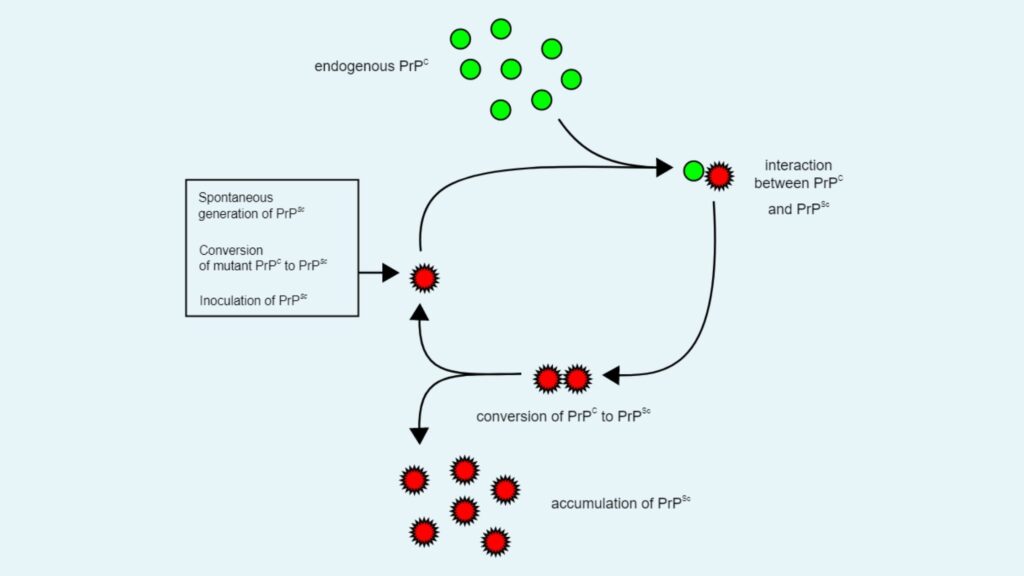
- Description: Another model suggests that PrPSc exists as fibrils, with the ends of these fibrils binding to PrPC and converting it into PrPSc.
- Fibril Growth: If prions replicated solely by this mechanism, the prion quantity would increase linearly. However, exponential growth of PrPSc is observed during prion diseases, which this model alone cannot explain.
- Fibril Breakage: The exponential growth can be explained by the breakage of fibrils. When fibrils break, they create more ends, allowing more PrPC to bind and convert into PrPSc, thus facilitating faster replication. The growth rate of prions is largely dependent on the concentration of PrPC, and it follows a square root dependency.
- Mathematical Model: The combination of fibril growth and breakage explains the observed exponential growth rates, which align with in vivo data from studies on transgenic mice. This same mechanism has been validated in vitro with various amyloid proteins, further supporting the fibril model.
3. Implications for Drug Design
- Targeting Fibril Ends: Understanding the mechanism of prion replication opens the door for potential therapeutic strategies. Since prion diseases have long incubation periods, it is not necessary to eliminate all PrPSc molecules to stop disease progression. A drug that targets fibril ends, preventing their growth, could significantly slow the exponential growth of prions.
- Optimal Drug Strategy: The most effective drugs would likely bind to fibril ends, preventing PrPC from converting into PrPSc, thereby inhibiting further fibril elongation. This approach would require a lower dosage of the drug, making it more efficient and less toxic.
4. Role of Host Cofactors
- Cofactors in Prion Formation: Research has shown that the presence of host cofactors, such as phospholipids and polyanions, is essential for prions to achieve high levels of infectivity. For example, phosphatidylethanolamine, a type of phospholipid, and single-stranded RNA molecules serve as cofactors in prion replication.
- In Vitro vs. In Vivo Infectivity: Protein-only PrPSc molecules, which lack these cofactors, exhibit significantly lower infectivity compared to those that form in the presence of these endogenous molecules. This indicates that the replication of infectious prions is not solely a protein-based process but also involves molecular interactions with specific cofactors.
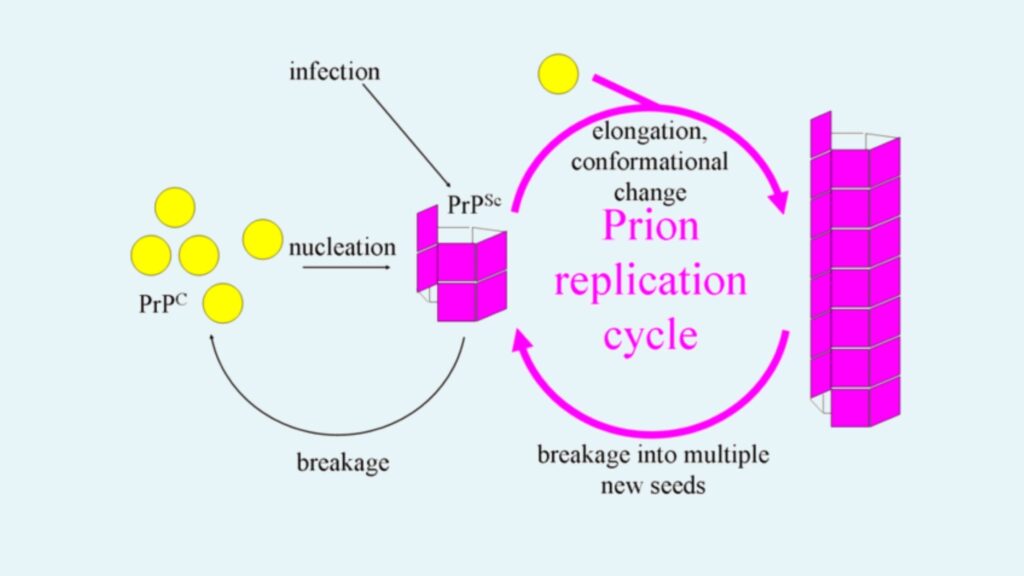
Structural Features of PrPC and PrPSc
Below is an analysis of the structural features that define each of these prion forms.
1. PrPC Structure
- General Features: PrPC has been extensively studied through methods like Nuclear Magnetic Resonance (NMR) and crystallography. Its structure has been characterized in various species, showing remarkable consistency across different organisms.
- Alpha-Helix Bundle: The primary structure of PrPC includes a well-defined three alpha-helix bundle, which constitutes the carboxyl-terminal region of the protein. This bundle gives PrPC its stable, soluble, and non-pathogenic form.
- Beta-Strands: PrPC also features two short antiparallel β-strands that contribute to its overall structural integrity. These strands are found near the core of the protein, within the folded regions.
- Amino-Terminal Domain: The amino-terminal region of PrPC is structurally distinct from the rest of the protein, as it is highly flexible and lacks identifiable secondary structure under typical experimental conditions. This region is generally disordered and more dynamic.
- Crystallographic Data: Recent crystallographic studies of PrPC have confirmed the structural observations from NMR, reinforcing the importance of the alpha-helical regions in maintaining the protein’s stability and function.
2. PrPSc Structure
- Challenges in Structural Study: Unlike PrPC, PrPSc is insoluble and tends to form aggregates. These properties make it difficult to study using traditional methods like NMR or X-ray crystallography. Nevertheless, researchers have made significant progress through alternative techniques.
- Solid-State NMR: Though limited by the difficulties in labeling PrPSc and handling its large molecular size, solid-state NMR has provided some structural insights, particularly about the aggregated state of PrPSc.
- Electron Crystallography: The most substantial structural information about PrPSc comes from electron crystallography and computational modeling. Negative-stain electron microscopy has allowed researchers to generate 2D crystals, which, in turn, have provided a resolution of approximately 7 Å.
- Beta-Sheet Content: PrPSc is characterized by an increased β-sheet content compared to PrPC. Fourier-transform infrared (FTIR) spectroscopy has confirmed that PrPSc contains a significantly higher proportion of β-sheets, which is a hallmark of its pathogenic and aggregated nature.
- Parallel β-Helices: Computational models suggest that the key structural component of PrPSc is a trimeric, left-handed parallel β-helix. This β-helix structure is thought to be responsible for the protein’s ability to form fibrils and propagate in its infectious state.
- X-Ray Diffraction: X-ray diffraction patterns from PrPSc fibers have provided further evidence supporting the β-helix model, confirming that PrPSc’s structure allows it to form the stable, yet pathogenic, amyloid aggregates seen in prion diseases.
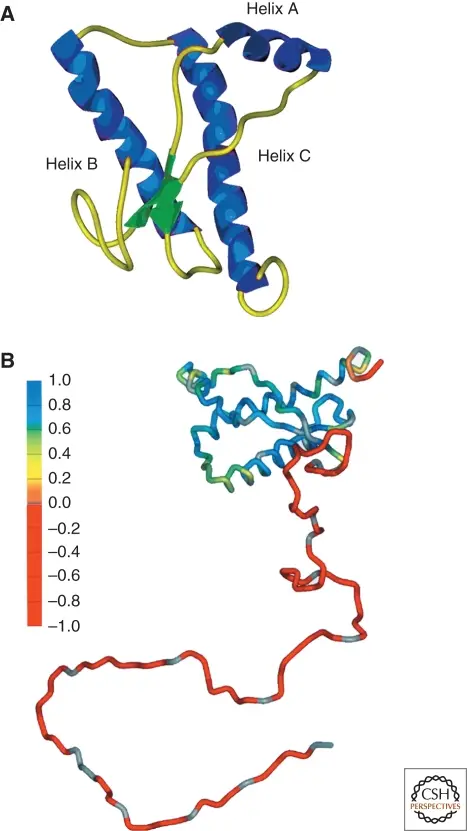
3. Conformational Flexibility and Prion Strains
- Prion Strain Variability: One of the more intriguing aspects of PrPSc is that different conformations of the same protein can result in distinct prion strains. This conformational flexibility means that prions can exist in multiple structural forms, each with its own pathogenic properties.
- Multiple Prion Structures: Given the variability in prion strains, it may be more appropriate to speak of prion “structures” rather than a single PrPSc structure. It remains unclear whether the differences between these structures are subtle or more substantial, but they play a key role in determining the biological behavior of prion diseases.
PrPC secondary, tertiary, and quaternary structure
The prion protein (PrPc) has been extensively studied to elucidate its structural characteristics. Understanding the secondary, tertiary, and quaternary structures of PrPc is crucial for deciphering its physiological roles and how it undergoes pathological transformations.
- Secondary Structure:
- Alpha-Helices and Beta-Strands:
- PrPc’s secondary structure is characterized by three alpha-helices and two beta-strands. The alpha-helices are arranged in a bundle, with helices 2 and 3 linked by a disulfide bond, providing structural stability.
- The beta-strands flank helix 1 and contribute to the overall stability of the globular domain. These secondary structures form the basis of PrPc’s globular core, spanning residues 125 to 230.
- Flexible Regions:
- The N-terminal region of PrPc, preceding the globular domain, is a hydrophobic stretch of approximately 20 amino acids. This region, initially predicted to form an additional alpha-helix, is actually quite flexible. It plays a role in anchoring PrPc to the endoplasmic reticulum (ER) membrane.
- Alpha-Helices and Beta-Strands:
- Tertiary Structure:
- Globular Domain:
- The tertiary structure of PrPc is defined by its globular domain, which consists of a well-defined three-helix bundle. The helices are arranged in a specific configuration that is stabilized by a disulfide bond.
- This domain is compact and characterized by short loops linking the secondary structural elements. The globular domain is crucial for PrPc’s normal function and stability.
- Intrinsically Disordered N-Terminal Region:
- N-terminal to the globular domain, PrPc contains an intrinsically disordered region of about 80 amino acids. This region is rich in glycine and proline, contributing to its flexibility.
- This disordered section includes octapeptide repeats that have been shown to bind metal ions, potentially influencing PrPc’s conformation and interactions.
- Poly-L-Proline II Structure:
- Some segments of the N-terminal region may adopt a poly-L-proline II-like conformation, which is reminiscent of collagen structures. This conformational feature may affect PrPc’s interactions and stability.
- Globular Domain:
- Quaternary Structure:
- Potential Dimerization:
- There is evidence suggesting that PrPc might exist as dimers, although this is still under investigation. Initial studies, including those using crystallization techniques, revealed a domain-swapped dimeric structure.
- However, it is uncertain whether these dimeric forms are physiological or artifacts of the experimental conditions. More research is needed to confirm whether PrPc dimers play a role in prion disease pathogenesis or in normal physiological processes.
- Potential Dimerization:
Molecular Mechanisms of Conversion of PrPC to PrP^TSE
The conversion of the normal prion protein (PrPC) to its pathogenic form, PrP^TSE, is a fundamental aspect of prion diseases. This process involves a transformation where PrPC adopts an abnormal, disease-associated conformation. Understanding the molecular mechanisms behind this conversion provides insight into prion disease pathology.
- Prion Hypothesis and Initial Observations:
- Prion Hypothesis:
- The prion hypothesis, first proposed by Stanley Prusiner, posits that PrP^TSE acts as a template inducing PrPC to misfold into the pathogenic PrP^TSE form (Prusiner, 1998). This hypothesis suggests that PrP^TSE facilitates the conversion of PrPC by acting as a structural template.
- Cell-Free Conversion Assay:
- Seminal experiments demonstrated that PrPC, when mixed with PrP^TSE in a suitable buffer, could adopt characteristics of PrP^TSE, evidenced by resistance to protease digestion (Kocisko et al., 1994). This cell-free conversion assay replicated key features of prion diseases, such as species barriers and the impact of sequence variations.
- Prion Hypothesis:
- Mechanistic Insights:
- Direct or Indirect Interaction:
- The conversion process likely involves direct or indirect interactions between PrPC and PrP^TSE. Evidence supports that physical interaction is crucial for conformational conversion (Horiuchi and Caughey, 1999; Baron et al., 2002).
- Induction of Misfolding:
- It is hypothesized that PrPC binds to PrP^TSE aggregates, leading to its misfolding. This process might involve a partial unfolding of PrPC into an activated conformation (PrP*) before it associates with PrP^TSE (Gill et al., 2010).
- Fragmentation and Seeding:
- Fragmentation of PrP^TSE aggregates into smaller “seeds” is considered an important part of the misfolding cascade. These seeds can initiate further conversions of PrPC (Knowles et al., 2009).
- Direct or Indirect Interaction:
- Structural Motifs Involved:
- Helix 1:
- Helix 1, loosely associated with the rest of the PrPC structure, may be primed for unfolding. The unfolding of this helix could lead to an extension of the beta-sheet structure, initiating the misfolding process (Speare et al., 2003; Norstrom and Mastrianni, 2006).
- Beta2–Alpha2 Loop:
- The beta2–alpha2 loop is another critical region for initiating the unfolding of PrPC. Amino acid substitutions in this region can affect misfolding, and it may bind to molecular effectors of misfolding (Sigurdson et al., 2011; Kurt et al., 2014a, b).
- Hydrophobic Core:
- The hydrophobic core, composed of aromatic amino acids, contributes to the stability of PrPC. Disruption of this core is a key driver of PrPC unfolding prior to its conversion to PrP^TSE. The exposure of hydrophobic residues may facilitate intermolecular interactions (Cheng and Daggett, 2014).
- Helix 1:
- Role of Cofactors:
- Inefficiency of Conversion:
- The cell-free conversion assay is inefficient, reflecting the low conversion rates observed in vivo and the prolonged incubation periods of prion diseases. Improved in vitro conversion methods have been developed to enhance conversion efficiency.
- Chaotropes and Mechanical Energy:
- Techniques using chaotropes and vigorous shaking can induce partial unfolding of PrPC and increase conversion rates. These methods produce amyloid fibrils but may not model all aspects of prion disease (Colby et al., 2007; Breydo et al., 2008).
- Sonication and Physiological Buffers:
- Methods incorporating high-powered sonication and physiological buffers, including brain homogenates, enhance conversion efficiency. These techniques utilize cofactors from brain tissue that may aid in the PrPC to PrP^TSE conversion (Saborio et al., 2001).
- Inefficiency of Conversion:
- Potential Cofactors:
- Polyanionic Compounds and Lipids:
- Polyanionic compounds such as glycosaminoglycans and nucleic acids, coupled with lipid environments, are believed to promote the PrPC to PrP^TSE conversion. These cofactors appear necessary for disease-specific misfolding (Deleault et al., 2003, 2005; Wang et al., 2007).
- Identification of Cofactors:
- The precise identity of cofactors involved in vivo remains elusive. Studies have detected potential cofactors like polysaccharides and sphingolipids in PrP^TSE preparations, but their roles require further characterization (Appel et al., 1999; Klein et al., 1998).
- Polyanionic Compounds and Lipids:
Normal function PrP
Below, the primary functions of PrP are detailed based on current scientific understanding:
- Peripheral Nerve Myelin Repair:
- Role in Schwann Cells: PrP is involved in the regulation of myelin repair in Schwann cells, which are crucial for peripheral nerve function. Experimental studies have shown that the cleavage of PrP in these cells triggers repair mechanisms that maintain myelin integrity.
- PrP Knockout Observations: Mice genetically modified to lack PrP exhibit demyelination, highlighting its importance in the maintenance and repair of peripheral nerve myelin.
- Regulated Cell Death:
- Comparison with Prion-like Proteins: Proteins such as MAVS, RIP1, and RIP3, which share similarities with PrP, form amyloid-like fibers that play a role in initiating regulated cell death during viral infections. This mechanism prevents the spread of pathogens to adjacent cells.
- Long-Term Memory Maintenance:
- Evidence from Studies: Research from 2005 suggested that PrP contributes to the maintenance of long-term memory. This is supported by findings that mice deficient in cellular PrP display altered long-term potentiation in the hippocampus.
- Mechanistic Insights: Recent studies indicate that neuronal protein CPEB, which shares genetic similarities with yeast prion proteins, is involved in sustaining long-term synaptic changes essential for memory formation.
- Stem Cell Renewal:
- Bone Marrow Self-Renewal: According to research from the Whitehead Institute for Biomedical Research, PrP expression is crucial for the self-renewal of hematopoietic stem cells in the bone marrow. Long-term hematopoietic stem cells express PrP on their surfaces, and the absence of PrP results in heightened sensitivity to cell depletion in hematopoietic tissues.
- Innate Immunity:
- Antiviral Properties: PrP appears to play a role in innate immune responses. The expression of the PRNP gene is upregulated during viral infections, and PrP exhibits antiviral properties against various viruses, including HIV.
Types of Prion Diseases
The types of prion diseases are categorized based on their host species and clinical manifestations. Below is an overview of the various prion diseases observed in humans and animals:
Prion Diseases in Humans
- Creutzfeldt-Jakob Disease (CJD):
- Description: CJD is a fatal degenerative brain disorder first identified in 1920. It is characterized by rapid cognitive decline and neurological symptoms.
- Symptoms: Memory loss, behavioral changes, poor coordination, visual disturbances, dementia, involuntary movements, blindness, weakness, and coma.
- Types:
- Sporadic CJD (sCJD): Occurs spontaneously due to the misfolding of prion proteins.
- Familial CJD (fCJD): Results from inherited mutations in the PRNP gene.
- Acquired CJD: Contracted through exposure to contaminated human tissues or products, such as corneal grafts or human growth hormone.
- Diagnosis and Treatment: CJD is diagnosed through clinical examination, cerebrospinal fluid analysis, and brain imaging. There is currently no effective treatment.
- Gerstmann–Sträussler–Scheinker Syndrome:
- Description: A rare and usually familial neurodegenerative disorder first described in 1936.
- Symptoms: Progressive ataxia, pyramidal signs, and adult-onset dementia.
- Affected Age: Typically manifests between 20 and 60 years of age.
- Diagnosis and Treatment: Diagnosis is based on clinical symptoms and genetic testing. There is no cure.
- Fatal Insomnia:
- Description: A rare disorder characterized by progressive insomnia leading to severe health issues.
- Symptoms: Increasing difficulty with sleep, speech problems, coordination difficulties, dementia, and eventually death.
- Forms:
- Fatal Familial Insomnia (FFI): An autosomal dominant form due to mutations in the PRNP gene.
- Sporadic Fatal Insomnia (sFI): Caused by inherited mutations.
- Diagnosis and Treatment: Diagnosed through sleep studies and PET scans. No known cure exists.
- Kuru:
- Description: A fatal neurodegenerative disease historically found among the Fore people of Papua New Guinea.
- Symptoms: Progressive cerebellar ataxia, which involves loss of muscle coordination. Also known as “laughing sickness.”
- Diagnosis and Treatment: Kuru was associated with ritualistic cannibalism; it has become rare due to changes in cultural practices. There is no treatment.
- Variably Protease-Sensitive Prionopathy (VPSPr):
- Description: A rare prion disease affecting approximately 2-3 out of every 100 million people.
- Symptoms: Includes speech deficits (aphasia, dysarthria), cognitive and motor decline (dementia, ataxia, parkinsonism, psychosis).
- Affected Age: Typically occurs in individuals over 70 years of age.
Prion Diseases in Animals
- Scrapie:
- Description: A fatal degenerative disease affecting sheep and goats, classified as a TSE.
- Symptoms: Lip smacking, altered gait, convulsive collapse, and compulsive fleece scraping.
- Transmission: Not transmissible to humans. Management involves quarantine and culling affected animals.
- Bovine Spongiform Encephalopathy (BSE):
- Description: Commonly known as “mad cow disease,” it affects cattle and is a TSE.
- Symptoms: Abnormal behavior, trouble walking, and weight loss. The incubation period is approximately 4-5 years.
- Transmission to Humans: Can lead to variant Creutzfeldt–Jakob disease (vCJD) if contaminated beef is consumed.
- Camel Spongiform Encephalopathy:
- Description: Also referred to as “mad camel disease,” this condition affects camels.
- Symptoms: Similar to BSE, with symptoms including abnormal behavior and coordination issues.
- Chronic Wasting Disease (CWD):
- Description: Known as “Zombie deer disease,” it affects deer species including mule deer, white-tailed deer, and moose.
- Symptoms: Chronic weight loss, behavioral changes, and brain lesions.
- Transmission: Spread through consumption of infected tissues.
- Other Notable Diseases:
- Spongiform Encephalopathy in Ostriches: Unknown if transmissible.
- Exotic Ungulate Encephalopathy (EUE): Affects Nyala, Oryx, Greater Kudu.
- Feline Spongiform Encephalopathy (FSE): Affects domestic cats.
Cellular Localization and Trafficking of PrPc
The key aspects of PrPc’s cellular localization and trafficking are as follows:
- Biogenesis and Localization:
- Endoplasmic Reticulum (ER) and Golgi Apparatus:
- Nascent Chain Import: The synthesis of PrPc begins in the rough endoplasmic reticulum, where the nascent protein chain is imported.
- Post-Translational Modifications: In the ER, PrPc undergoes critical modifications including the attachment of N-linked core glycans, formation of disulfide bonds, and addition of a glycosylphosphatidylinositol (GPI) anchor.
- Golgi Processing: The protein is then transported to the Golgi apparatus, where glycan structures are further processed into complex forms.
- Plasma Membrane:
- Targeting and Topology: Following processing, PrPc is transported to the outer leaflet of the plasma membrane. It can adopt various topologies including fully translocated (SecPrP), two distinct transmembrane forms (NtmPrP and CtmPrP), and a soluble cytosolic form (CytPrP).
- Endoplasmic Reticulum (ER) and Golgi Apparatus:
- Cleavage and Processing:
- Normal Cleavage:
- Alpha-Cleavage: PrPc undergoes constitutive cleavage between residues 110 and 111. This cleavage produces a 9-kDa soluble N-terminal fragment (N1) and a 17 kDa C-terminal fragment (C1 or PrP-II) still attached to the membrane via the GPI anchor. This process is stimulated by protein kinase C pathway agonists and is mediated by ADAM 10 and ADAM 17 metalloproteases.
- Beta-Cleavage:
- Beta-Cleavage: Occurs within or adjacent to the octapeptide repeats, generating a 19-kDa GPI-anchored C-terminal fragment (C2) and a 7-kDa N-terminal fragment (N2). This cleavage is induced by reactive oxygen species (ROS).
- Normal Cleavage:
- Isoforms and Localization:
- Cytosolic and Nuclear Localization:
- Cytosolic PrPc (CytPrP): Found in the cytoplasm of certain neurons and tissues, this isoform may be associated with cellular dysfunction, although its exact role remains controversial. CytPrP can be translated from a downstream AUG codon and might accumulate in the nucleus or form insoluble cytosolic aggregates under conditions of proteasome inhibition.
- Nuclear Localization: PrPc constructs lacking the N-terminal signal sequence and GPI anchor (e.g., 23–230 PrPc) are predominantly nuclear. This nuclear localization is associated with chromatin binding and may contribute to the formation of multinucleated cells in certain contexts.
- Cytosolic and Nuclear Localization:
- Endocytosis and Internalization:
- Internalization Mechanisms:
- Caveolae and Clathrin-Mediated Endocytosis: PrPc can be internalized via caveolae/raft and clathrin-dependent pathways. The interaction with caveolin-1 and the involvement of Fyn kinase are noted in some internalization processes. Additionally, copper and zinc binding can influence PrPc endocytosis through clathrin-coated pits.
- Receptor-Mediated Endocytosis: PrPc interacts with cell surface receptors, including the 37-kDa/67-kDa laminin receptor and low-density lipoprotein receptor-related protein 1 (LRP1), which play roles in its internalization and trafficking.
- Internalization Mechanisms:
Physiological Functions of PrPc
The cellular prion protein (PrPc) exhibits a range of physiological functions across different systems in the body. While the precise roles of PrPc are still under investigation, substantial evidence from various studies highlights its involvement in several key biological processes:
- Functions in the Nervous System:
- Neurite Outgrowth:
- Axonal and Dendritic Growth: PrPc influences neurite outgrowth, as observed in neurons lacking PrPc, which show reduced axon and dendrite growth.
- Synaptic Function: PrPc’s role in synaptic function is suggested by impaired spatial learning and altered neurotransmission in PrP-null mice. PrPc may affect synaptic transmission and neuroprotection by modulating N-methyl-D-aspartate receptors (NMDARs) containing NR2D subunits, thereby attenuating glutamate excitotoxicity.
- Neuroprotection:
- Myelination: PrPc contributes to the maintenance of myelinated axons in white matter, suggesting a neuroprotective role.
- Neurodegeneration: Abnormal levels of PrPc have been linked to progressive neurodegeneration, underscoring its protective functions in the nervous system.
- Olfactory Behavior:
- Sensory Processing: PrPc is important for normal olfactory function. Studies using Prnp-deficient and PrPc neuronal-specific expression transgenic mice have demonstrated that PrPc impacts odor perception by influencing local circuit functions in the olfactory system.
- Other Functions:
- Circadian Rhythms and Cognitive Functions: PrPc is involved in regulating circadian rhythms, memory formation, and cognitive functions.
- Neurite Outgrowth:
- Functions in the Immune System:
- Hematopoietic Stem Cells:
- Self-Renewal: PrPc is required for the self-renewal of hematopoietic stem cells. Despite this, PrP-null mice do not show a higher incidence of tumors or infections compared to wild-type mice.
- Lymphoid and Myeloid Cells:
- T-Cell Activation: PrPc is expressed in various immune cells, including T-cells, B-cells, natural killer (NK) cells, and dendritic cells (DCs). It modulates T-cell activation and proliferation, potentially by influencing lipid raft constituents and signaling pathways.
- Macrophage Function: PrPc is involved in macrophage phagocytosis by activating ERK1/2 and Akt kinases, which are crucial for immune responses.
- Antigen Presentation:
- DC-T Cell Interaction: PrPc plays a role in antigen presentation and T-cell activation by dendritic cells. An absence of PrPc on DCs results in a diminished allogenic T-cell response.
- Hematopoietic Stem Cells:
- Functions in Cell Adhesion:
- Cell-Cell Adhesion:
- Neuronal Differentiation: PrPc promotes cell adhesion and neuritogenesis by interacting with various cell adhesion molecules such as laminin, LRP, heparan sulfate proteoglycans, and neural cell adhesion molecules (N-CAMs).
- Junctional Protein: PrPc accumulates at cell–cell junctions in brain endothelial cells, contributing to the integrity of the blood-brain barrier and influencing monocyte transmigration.
- Embryonic Development:
- Cell Adhesion in Zebrafish: PrPc mediates both Ca2+-independent and Ca2+-dependent cell adhesion in zebrafish embryos. It regulates E-cadherin delivery to the plasma membrane, affecting embryonic cell adhesion and morphogenetic movements during gastrulation.
- Cell-Cell Adhesion:
Transmission of Prion Disease
The primary methods of prion disease transmission include:
- Acquired Transmission:
- Description: This form of transmission occurs when an individual comes into direct contact with prion-contaminated materials or tissues.
- Mechanisms:
- Contaminated Food: Consuming food products infected with prions, such as beef contaminated with bovine spongiform encephalopathy (BSE), can transmit the disease. This pathway is notably implicated in variant Creutzfeldt-Jakob disease (vCJD).
- Medical Equipment: Use of contaminated medical instruments, such as those employed in brain surgery or during organ transplants, can lead to prion infection. The risk is associated with procedures involving human tissues that may harbor prions.
- Body Fluids: Exposure to infected body fluids, such as urine or saliva, from prion-infected animals may also pose a risk, although this method is less common in human disease transmission.
- Inherited Transmission:
- Description: Inherited prion diseases result from genetic mutations that predispose individuals to produce abnormal prion proteins.
- Mechanism:
- Genetic Mutations: Specific mutations in the PRNP gene, which encodes the prion protein, lead to the production of misfolded PrP. This inherited abnormal protein then accumulates, causing neurodegenerative conditions such as familial Creutzfeldt-Jakob disease (fCJD) and Gerstmann–Sträussler–Scheinker syndrome (GSS).
- Sporadic Transmission:
- Description: Sporadic prion diseases arise spontaneously without a known external cause or identifiable genetic mutation.
- Mechanism:
- Spontaneous Misfolding: In sporadic cases, prion proteins misfold and aggregate in the brain without any preceding genetic or environmental trigger. This type of transmission is exemplified by sporadic Creutzfeldt-Jakob disease (sCJD), where the misfolded PrP appears without an apparent reason.
Symptoms of Prion Disease
The symptoms of prion diseases vary depending on the specific type of prion disease and the stage of progression. Below are the common symptoms associated with these diseases:
- Rapidly Developing Dementia:
- Description: One of the hallmark features of prion diseases is the swift onset of cognitive decline. Patients experience a significant deterioration in memory, reasoning, and other cognitive functions over a relatively short period.
- Difficulty Walking and Changes in Gait:
- Description: Patients may exhibit a range of motor impairments, including difficulty walking and abnormal gait patterns. These issues arise due to the progressive degeneration of motor control regions in the brain.
- Jerking Movements of the Muscles:
- Description: Involuntary muscle jerks, known as myoclonus, are common. These movements can be sporadic and affect various muscle groups, contributing to overall motor dysfunction.
- Hallucinations:
- Description: Visual or auditory hallucinations may occur, where patients perceive things that are not present. This symptom contributes to the overall confusion and cognitive impairment experienced by individuals.
- Muscle Stiffness:
- Description: The rigidity or stiffness of muscles is often observed, which can impair movement and coordination. This symptom reflects the involvement of motor pathways affected by prion protein accumulation.
- Confusion:
- Description: Patients frequently experience severe confusion and disorientation. This symptom is related to the overall cognitive decline and affects the ability to understand or respond to the environment.
- Fatigue:
- Description: Persistent fatigue or a general feeling of exhaustion is common. As the disease progresses, the cumulative effects of neurological damage and impaired motor function contribute to this symptom.
- Difficulty Speaking:
- Description: Problems with speech, including difficulties in articulating words and forming coherent sentences, are prevalent. This symptom is linked to the degeneration of brain areas responsible for language and communication.
Diagnosis of Prion Disease
- Prion disease is difficult to diagnose because their symptoms are similar to other neurodegenerative disorders. Brain biopsy is the only way to confirm Prion Disease, but this process only can be performed after death.
- Currently, there are several tests which can be used to diagnose prion disease such as Magnetic resonance imaging (MRI), Cerebrospinal fluid (CSF) testing, Electroencephalography (EEG).
FAQ
Prions are a type of infectious agent composed solely of protein. They are abnormal, misfolded versions of a normal protein called prion protein (PrP). Unlike other pathogens, prions do not contain nucleic acids (DNA or RNA). They cause a range of neurodegenerative diseases by inducing misfolding of normal prion proteins, leading to the accumulation of abnormal proteins in the brain, which disrupts normal cellular function and leads to brain damage. Diseases caused by prions include Creutzfeldt-Jakob disease, bovine spongiform encephalopathy (mad cow disease), and kuru.
Prions can be transmitted through various routes. Ingesting contaminated food products, such as beef infected with bovine spongiform encephalopathy (BSE), is one common method of transmission. Medical procedures involving contaminated surgical instruments or tissue grafts can also spread prions. Additionally, certain prion diseases can be inherited due to genetic mutations in the prion protein gene. Transmission through blood transfusions or organ transplants from infected donors has also been reported. The prions are resistant to conventional sterilization processes, making them particularly challenging to manage.
Prions replicate through a unique mechanism that does not involve nucleic acids. Instead, they propagate by inducing conformational changes in normal prion proteins (PrP^C) to transform them into the abnormal, disease-causing form (PrP^Sc). This misfolding process creates a chain reaction, where the newly formed prions cause further misfolding of normal proteins. As more normal proteins are converted into the prion form, the number of infectious prions increases, leading to a progressive accumulation of prion proteins in the brain and associated neurodegeneration.
The term “prions” is derived from the phrase “proteinaceous infectious particles.” It was coined by Stanley Prusiner in 1982 to describe these unique infectious agents that are composed solely of protein and are capable of causing disease. The term emphasizes the protein-based nature of prions and their role in transmitting infectious disease without the involvement of nucleic acids.
Prions spread through several mechanisms depending on the type of prion disease. Ingesting contaminated food, such as beef infected with BSE, can lead to the spread of prions. The prions can then migrate from the digestive tract to the central nervous system. Prions can also spread through contaminated medical instruments, such as those used in surgeries or tissue transplants. In some cases, prions can be transmitted through exposure to infected bodily fluids or through inherited genetic mutations. The spread of prions is facilitated by their resistance to standard disinfection and sterilization procedures.
Stanley Prusiner discovered prions in 1982. His groundbreaking research identified these proteinaceous infectious particles as the causative agents of several neurodegenerative diseases, leading to a new understanding of how infectious diseases can be transmitted without nucleic acids. Prusiner's work challenged existing paradigms in microbiology and earned him the Nobel Prize in Physiology or Medicine in 1997 for his discovery.
Prions differ from viruses in several fundamental ways. Unlike viruses, which are composed of nucleic acids (either DNA or RNA) encased in a protein coat, prions consist solely of abnormal proteins without any genetic material. Viruses replicate by hijacking the host cell's machinery to produce new viral particles, while prions propagate by inducing conformational changes in normal proteins, leading to the accumulation of abnormal prion proteins. Additionally, prions are highly resistant to conventional disinfection and sterilization methods that are effective against viruses, making them particularly challenging to control.
The number of infectious prions increases through a process of self-replication. As prions induce the misfolding of normal prion proteins (PrP^C) into the abnormal prion form (PrP^Sc), they create new infectious particles. This process leads to a progressive accumulation of prion proteins in the brain. The misfolded prions aggregate into clumps, forming amyloid plaques and causing cellular damage. As more normal proteins are converted into the prion form, the number of infectious prions grows, leading to a worsening of the disease and increased spread within the host organism.
The answer would depend on the specific list of diseases provided. However, prion diseases include Creutzfeldt-Jakob disease, kuru, and mad cow disease (bovine spongiform encephalopathy). Diseases such as HIV/AIDS, tuberculosis, and malaria are not caused by prions but by viruses or bacteria. Identifying whether a particular disease is prion-related requires knowledge of its causative agent.
Prions infect by converting normal prion proteins (PrP^C) in the host into the abnormal, disease-causing form (PrP^Sc). This conversion process is initiated when a prion encounters a normal protein, causing it to misfold and adopt the abnormal prion structure. The newly formed prions then go on to induce further misfolding of other normal prion proteins, creating a cascade of abnormal protein accumulation. This process disrupts cellular function, leads to neuronal damage, and results in the progressive neurodegeneration characteristic of prion diseases.
Prions infect organisms by introducing misfolded proteins into the host. These misfolded proteins, which are resistant to degradation and can aggregate into amyloid plaques, induce the conversion of normal prion proteins (PrP^C) into the pathological prion form (PrP^Sc). As this misfolding spreads, it leads to the accumulation of abnormal prions, disrupting brain function and causing neurodegenerative symptoms. The disease progresses as more and more normal proteins are converted, leading to extensive brain damage and eventual loss of neurological function.
Prions propagate and replicate through a process of protein misfolding. When a prion interacts with a normal prion protein (PrP^C), it induces a conformational change, converting the normal protein into the misfolded prion form (PrP^Sc). This newly formed prion can then induce further misfolding of other normal prion proteins. This chain reaction results in the amplification of the prion population, leading to the accumulation of prion proteins in the brain and causing neurodegenerative damage. The replication process does not involve nucleic acids, distinguishing it from the replication mechanisms of other infectious agents.
In microbiology, prions are studied as unconventional infectious agents that are composed solely of misfolded proteins and lack nucleic acids. They are significant because they challenge traditional concepts of infectious disease. Prions are responsible for a group of progressive neurodegenerative disorders that affect both humans and animals. Research in microbiology focuses on understanding how prions cause disease, their mechanisms of transmission, and methods for diagnosis and treatment. The study of prions has expanded the understanding of protein misfolding diseases and has implications for public health and safety.
Prions themselves are not visible under standard light microscopes due to their small size and the nature of their composition. However, their effects on brain tissue can be observed with specialized techniques. Infected brain tissue often shows characteristic changes, including the formation of amyloid plaques and spongiform changes (cavitation) in the brain's gray matter. Techniques such as electron microscopy, immunohistochemistry, and Western blotting can be used to visualize and analyze prion proteins and their associated pathology.
Prions are unique among infectious agents because they are composed entirely of protein and lack any nucleic acids, such as DNA or RNA. This absence of genetic material means they do not follow conventional replication processes like viruses or bacteria. Instead, prions propagate by inducing conformational changes in normal proteins, leading to a chain reaction of protein misfolding. This distinctive mode of infection and replication, combined with their resistance to conventional sterilization methods, sets prions apart from other pathogens and poses unique challenges in understanding and managing prion diseases.
- Requena JR, Wille H. The Structure of the Infectious Prion Protein and Its Propagation. Prog Mol Biol Transl Sci. 2017;150:341-359. doi: 10.1016/bs.pmbts.2017.06.009. Epub 2017 Jul 31. PMID: 28838667.
- Gill, A. C., & Castle, A. R. (2018). The cellular and pathologic prion protein. Handbook of Clinical Neurology, 21–44. doi:10.1016/b978-0-444-63945-5.00002-7
- Mehrpour, M., & Codogno, P. (2010). Prion protein: From physiology to cancer biology. Cancer Letters, 290(1), 1–23. doi:10.1016/j.canlet.2009
- Annus, Á., Csáti, A., & Vécsei, L. (2016). Prion diseases: New considerations. Clinical Neurology and Neurosurgery, 150, 125–132. doi:10.1016/j.clineuro.2016.09
- https://www.azolifesciences.com/article/What-are-Prions.aspx
- https://www.biocompare.com/Life-Science-News/558044-How-the-Prion-Protein-Clumps/
- http://www.meteck.org/prion.html
- https://thedaily.case.edu/first-atomic-level-imaging-of-lethal-prions-provide-sharpened-focus-for-potential-treatments/
- https://www.prionalliance.org/2013/11/26/what-are-prions/
- https://virology.ws/2016/09/15/structure-of-an-infectious-prion/
- https://www.cdc.gov/prions/about/index.html
- https://www.hopkinsmedicine.org/health/conditions-and-diseases/prion-diseases