What are Plant-water Relations?
- Water plays a crucial role in the physiological processes of plants, acting as a medium in which essential compounds dissolve. In plant cells, protoplasm, which is a mixture of water and various molecules, is fundamental to cellular functions. Despite the high water content of certain plants—like watermelon, which contains about 92% water—most herbaceous plants have only 10 to 15% dry matter in their fresh weight. This water distribution varies, with woody parts of the plant containing less water compared to the more hydrated soft tissues.
- Plants, particularly terrestrial ones, absorb significant quantities of water daily. However, a substantial portion of this water is lost through transpiration, which is the evaporation of water from leaf surfaces. For instance, a mature corn plant can take up over three liters of water per day, while a mustard plant can absorb its weight in water in just five hours. Given the high water demand, it is often a limiting factor for plant growth and productivity in both natural and agricultural environments.
- The concept of plant-water relations involves understanding how plants manage their water resources. This includes the uptake of water from the soil, its internal transport, and its loss through evaporation. Water status in plants is typically described in terms of ‘water potential,’ a pressure-based measurement that is always negative. Water movement through plants and soil is driven by gradients in hydrostatic pressure over larger distances, while microscopic movements, such as across semipermeable membranes, are driven by water potential gradients.
- Evaporation from leaves is regulated by stomata, and if water loss is not replenished through the soil-to-leaf water transport, it can lead to wilting. Plant physiology—the study of live processes—encompasses various aspects of water relations, including diffusion, osmosis, absorption, transpiration, and the ascent of sap.
- Plants absorb water and minerals from the soil through their root systems, aided by root hairs. Plant cells are composed of three main components: the vacuole, protoplasm, and cell wall. The protoplast, which includes the plasma membrane and protoplasm, is crucial for cellular function. These components are separated by plasma membranes, such as the tonoplast between the vacuole and protoplasm, and the plasma lemma between the protoplasm and cell wall.
- Additionally, plant cells are interconnected through plasmodesmata, allowing for communication between cells. The plasma membrane’s selective permeability controls the passage of materials. Water is vital for maintaining turgor pressure in plant cells, providing temporary mechanical support and ensuring cell function. Despite its importance, most absorbed water eventually exits the plant through transpiration, underscoring the dynamic balance plants must maintain between water uptake and loss.
Importance of water to plants
- Constituent of Protoplasm: Water constitutes about 90-95% of the protoplasm within plant cells. This high water content is fundamental for maintaining cellular structure and function.
- Medium for Translocation: Water acts as a crucial medium for the transport of minerals from the soil to various parts of the plant. Additionally, it facilitates the movement of nutrients produced by the leaves through the plant’s vascular system.
- Enzyme Activity: Many metabolic reactions within plants occur in an aqueous environment. Water is vital for enzyme activity, which drives various biochemical processes necessary for plant growth and development.
- Raw Material for Photosynthesis: Water is one of the essential raw materials for photosynthesis. During this process, water is split to release oxygen and provide electrons for the synthesis of glucose, which is crucial for the plant’s energy needs.
- Byproduct of Cellular Respiration: Water is also a byproduct of cellular respiration, a process where glucose is broken down to release energy. The rate of respiration in plants is influenced by the availability of water, affecting overall energy production.
- Control of Plant Movements: Water regulates several plant movements. For example, the opening and closing of stomata, which are crucial for gas exchange, and the mechanisms controlling flower opening and fruit dehiscence are mediated by changes in water pressure within plant cells.
- Seed Germination: Water is essential for seed germination. It activates enzymes that break down stored nutrients in seeds, allowing for the growth of the embryo and the emergence of the seedling.
- Cell Turgidity: Maintaining cell turgidity relies on adequate water supply. Turgidity, which is the pressure exerted by the fluid inside the cell against the cell wall, is crucial for maintaining structural integrity and is the initial step in promoting plant growth.
Properties of Water
- Hydrogen Bonding: Water molecules form strong hydrogen bonds, which significantly impact various biological processes. These bonds are crucial for water’s high latent heats of evaporation and freezing, allowing plants to endure thermal stress and frost conditions.
- Cohesive Strength: Water exhibits high cohesive strength, enabling it to withstand substantial tensions within the xylem vessels of plants. This cohesive property helps maintain a continuous water column throughout the plant, essential for effective water transport.
- Surface Tension: At the air-water interface, water has a high surface tension, creating a robust surface film. This characteristic ensures that small soil pores or plant tissues retain water even under high tension, which is critical for maintaining hydration in various plant environments.
- Molecular Buffering: On a molecular level, water molecules surround macromolecules such as proteins and DNA, forming a hydration shell. This shell acts as a buffer, preventing macromolecules from clumping together and thus maintaining their three-dimensional structures necessary for biochemical reactions.
- Polarity and Solvent Properties: Water is a polar molecule, with a slight electrical imbalance across its structure. This polarity, combined with hydrogen bonding capabilities, makes water an excellent solvent for ions and small organic molecules, including sugars. This property supports the development of osmotic pressure, which is vital for plant turgidity in saline or drought conditions.
- Lipid Bilayers Formation: The polar nature of water facilitates the formation of lipid bilayers in cellular membranes. Fatty acids, with their nonpolar hydrocarbon tails and polar carboxylic acid groups, arrange themselves into bilayers with hydrophilic heads facing outward and hydrophobic tails inward. This arrangement is fundamental to cell membrane structure.
- Viscosity: Water’s viscosity affects its flow rate in response to pressure gradients. This property is important for the efficient transport of water through plant vascular systems.
- Dissociation and pH: Water’s ability to dissociate into hydrogen and hydroxyl ions plays a central role in the pH scale. This dissociation is fundamental to various biochemical processes and the regulation of cellular environments.
- Diffusion: Water and solute molecules are in constant thermal motion, which facilitates the diffusion of solutes and the equalization of concentration gradients. This diffusion is crucial for nutrient uptake and waste removal in plant cells.
Property | Value and Units |
---|---|
Cohesive Strength | >25 MPa |
Surface Tension | 0.073 N m⁻¹ at 20°C |
Viscosity | 0.0018 Pa s at 0°C |
0.0010 Pa s at 20°C | |
Diffusion Coefficient of Small Solutes | ∼1×10⁻⁹ m² s⁻¹ |
Molar Volume (Pure Water at 20°C) | 1.81×10⁻⁵ m³ mol⁻¹ |
Latent Heat of Evaporation at 20°C | 2.5 MJ kg⁻¹ |
Latent Heat of Melting | 0.34 MJ kg⁻¹ |
Saturated Vapour Pressure of Pure Water | 0.61 kPa at 0°C |
1.23 kPa at 10°C | |
2.34 kPa at 20°C | |
4.24 kPa at 30°C | |
7.38 kPa at 40°C |
Concept of water relations
Water relations in plants encompass the various physical processes that manage the movement and distribution of water within the plant and its environment. These processes include diffusion, osmosis, and imbibition. Each process plays a crucial role in maintaining cellular function and overall plant health.
1. Diffusion
Diffusion represents a fundamental process in the movement of molecules and ions, occurring in solids, liquids, and gases. It involves the passive movement of molecules from a region of higher concentration to a region of lower concentration, driven by the intrinsic kinetic energy of the particles involved. This section elucidates the mechanisms, factors influencing diffusion, and its significance in biological systems.
Mechanisms and Characteristics
- Passive Movement: Diffusion is characterized as a passive process. Molecules move without the need for external energy input, relying solely on their kinetic energy. This movement continues until equilibrium is achieved, where the concentration of molecules is uniform throughout the medium.
- Concentration Gradient: The driving force behind diffusion is the concentration gradient. Molecules move from areas of higher concentration to those of lower concentration. The rate of this movement is directly proportional to the concentration difference between the two regions.
- Random Motion: Molecules in diffusion exhibit random, non-directional movement. Each molecule moves independently, and the overall direction is determined by statistical probability rather than coordinated movement.
- Influence of Medium: Diffusion rates vary with the medium. For instance, diffusion occurs more rapidly in gases compared to liquids due to lower density and fewer intermolecular interactions in gases.
- Diffusion Pressure: The tendency of solute particles to move from an area of high concentration to an area of low concentration is quantified by diffusion pressure. Pure solvents exhibit higher diffusion pressure compared to solutions, reflecting the decreased tendency of solutes to diffuse in the presence of added substances. This reduction is quantified as diffusion pressure deficit (DPD).
Factors Affecting Diffusion
- Temperature: The rate of diffusion is positively correlated with temperature. Higher temperatures increase the kinetic energy of molecules, thereby accelerating their movement.
- Concentration Gradient: A steeper concentration gradient results in a faster diffusion rate. The larger the difference in concentration, the quicker the molecules will diffuse to achieve equilibrium.
- Medium Density and Size of Solute: Diffusion is inversely proportional to the density of the medium and the size of the solute particles. A denser medium or larger solute particles slow down the diffusion process.
- Molecular Weight: Heavier molecules diffuse more slowly compared to lighter ones. The molecular weight of the solute inversely affects the rate of diffusion.
- Distance: The distance over which diffusion occurs also influences the rate. Greater distances result in slower diffusion rates as the time required to traverse the space increases.
Biological Significance
- Cellular Transport: In biological systems, diffusion is essential for the movement of substances between cells. Nutrients, ions, and waste products traverse cell membranes via diffusion, facilitating cellular function and homeostasis.
- Transpiration: In plants, diffusion is crucial for water loss through transpiration. Water vapor exits plant leaves through stomata, driven by the concentration gradient between the leaf interior and the external environment.
- Gas Exchange: Diffusion also underlies the exchange of gases such as oxygen (O₂) and carbon dioxide (CO₂) during photosynthesis and respiration. Gases move across cellular membranes and within plant tissues based on concentration gradients, supporting vital physiological processes.
Practical Examples
- KMnO₄ in Water: When a crystal of potassium permanganate (KMnO₄) is placed in water, it disperses throughout the liquid, creating a purple hue due to the diffusion of KMnO₄ molecules.
- Incense in a Room: Burning incense releases aromatic molecules that diffuse throughout a room, making the scent detectable at various locations due to the movement of these molecules from high to low concentration areas.
2. Osmosis
Osmosis is a specialized type of diffusion, specifically involving the movement of water molecules through a selectively permeable membrane. This process plays a critical role in the physiological functions of living organisms, particularly in plants, where it influences water absorption, cell turgidity, and overall cellular homeostasis.
Fundamental Concepts
- Osmosis Defined: Osmosis refers to the diffusion of water from a region of lower solute concentration (higher water potential) to a region of higher solute concentration (lower water potential) across a semi-permeable membrane. This movement continues until equilibrium is reached, where the water potential on both sides of the membrane is equal.
- Semi-permeable Membrane: A key component in osmosis, the semi-permeable membrane allows the passage of water molecules but restricts the movement of solute particles. This selective permeability is crucial for maintaining the balance of fluids and solutes within cells and their surrounding environments.
- Concentration Gradient: Osmosis is driven by the concentration gradient of solutes. Water moves towards the side with a higher solute concentration, effectively balancing the concentrations on both sides of the membrane.
- Osmotic Pressure (OP): Osmotic pressure is the pressure required to prevent the osmotic movement of water into a solution. It is directly proportional to the solute concentration and is a key factor in maintaining the structural integrity of cells.
Types of Osmosis
Various types of osmosis can be distinguished based on the direction of solvent movement and the nature of the membranes involved. The following are the primary types of osmosis:
- Endosmosis
- Definition: Endosmosis refers to the movement of solvent molecules from an external source into a cell or across a semi-permeable membrane.
- Mechanism: This process occurs when the external solution has a lower concentration of solutes compared to the internal solution of the cell. The solvent molecules move into the cell to equalize the solute concentration on both sides of the membrane.
- Functional Impact: Endosmosis can lead to cell swelling as the influx of water increases the internal pressure.
- Exosmosis
- Definition: Exosmosis is the movement of water from within the cell to the external environment across the membrane.
- Mechanism: This occurs when the external solution has a higher concentration of solutes compared to the internal solution of the cell. Water exits the cell to balance the solute concentrations.
- Functional Impact: Exosmosis can cause cell shrinkage or plasmolysis due to the loss of internal water.
- Cell-to-Cell Osmosis
- Definition: This type involves osmosis between adjacent cells. It is characterized by the movement of water between cells that are closely situated.
- Mechanism: Differences in osmotic pressure between neighboring cells can drive the flow of water. If one cell has a higher osmotic pressure than another, water will move from the cell with lower osmotic pressure to the cell with higher osmotic pressure.
- Functional Impact: This process helps in maintaining fluid balance and nutrient distribution among cells in tissues.
- Reverse Osmosis
- Definition: Reverse osmosis is a process where pressure is applied to force water through a semi-permeable membrane, against its natural osmotic gradient.
- Mechanism: By applying external pressure greater than the osmotic pressure, water molecules move from a region of higher solute concentration to a region of lower solute concentration. This is often used for purifying saline water into potable water.
- Functional Impact: Reverse osmosis is widely used in water treatment and desalination processes to remove impurities and produce clean water.
- Types of Membranes Involved in Osmosis
- Impermeable Membrane: A membrane that does not allow any substances to pass through it.
- Permeable Membrane: A membrane that allows all substances, both solutes and solvents, to pass through freely.
- Semi-Permeable Membrane: A membrane that permits the passage of solvent molecules but restricts the movement of solute molecules.
- Selectively Permeable Membrane: A membrane that allows the passage of certain types of molecules or ions while blocking others, based on size, charge, or other properties.
Osmosis in Cellular Context
- Turgor Pressure (TP): As water enters a cell during endosmosis, it creates a hydrostatic pressure within the cell, known as turgor pressure. This pressure pushes the cell membrane against the cell wall, maintaining the cell’s rigidity and structural integrity. Turgor pressure is vital for maintaining the turgid state of plant cells, which is essential for plant stability and growth.
- Wall Pressure (WP): Wall pressure is the counterforce exerted by the cell wall against the turgor pressure. In turgid cells, wall pressure equals turgor pressure, preventing further water influx and maintaining cellular stability.
- Diffusion Pressure Deficit (DPD): DPD, also referred to as suction pressure, is the difference between the osmotic pressure and turgor pressure within a cell. It determines the direction and rate of water movement through osmosis. The higher the DPD, the greater the tendency for water to enter the cell.
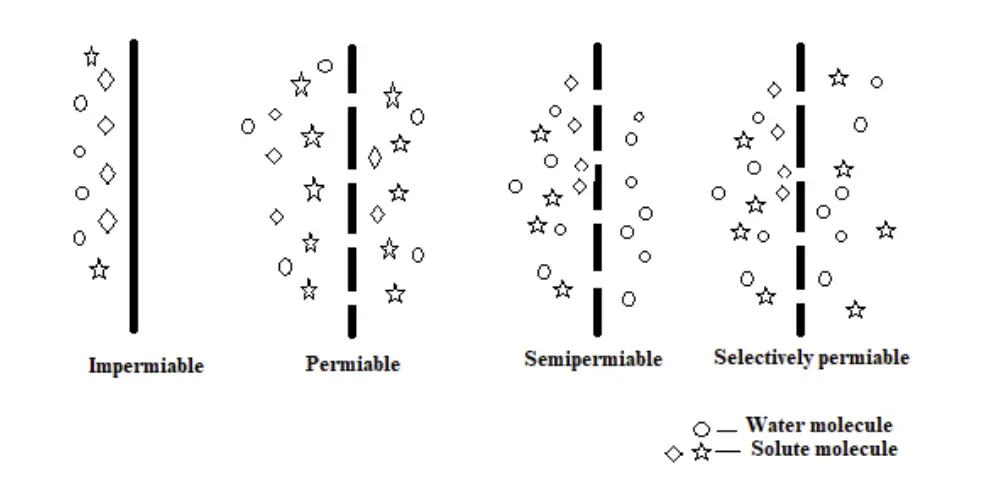
Types of solution based on the concentration
Solutions are categorized based on their osmotic concentration relative to that of the cell or surrounding environment. Here are the primary types of solutions:
- Hypertonic Solution
- Definition: A hypertonic solution has a higher osmotic concentration (solute potential) compared to another solution or the cell sap (tonoplasm).
- Mechanism: When a cell is immersed in a hypertonic solution, the osmotic pressure outside the cell exceeds that inside. Consequently, water moves out of the cell through exosmosis.
- Functional Impact: As water exits the cell, it becomes flaccid or shriveled. This is due to the loss of internal turgor pressure. Hypertonic solutions are significant in processes like preserving food, where dehydration inhibits microbial growth.
- Hypotonic Solution
- Definition: A hypotonic solution has a lower osmotic concentration compared to another solution or the cell sap.
- Mechanism: In a hypotonic environment, the osmotic pressure outside the cell is lower than inside. This results in water entering the cell by endosmosis.
- Functional Impact: The influx of water causes the cell to swell and become turgid, which is crucial for maintaining cell structure and function in plant cells. The hypotonic nature of soil, for instance, facilitates water absorption by plant roots.
- Isotonic Solution
- Definition: An isotonic solution has an osmotic concentration equal to that of another solution or the cell sap.
- Mechanism: In an isotonic environment, the osmotic pressure inside and outside the cell is balanced. Consequently, there is no net movement of water across the cell membrane.
- Functional Impact: Cells in isotonic solutions remain stable, maintaining their normal shape and function. This equilibrium is essential for processes such as intravenous fluid administration, where maintaining fluid balance is critical.
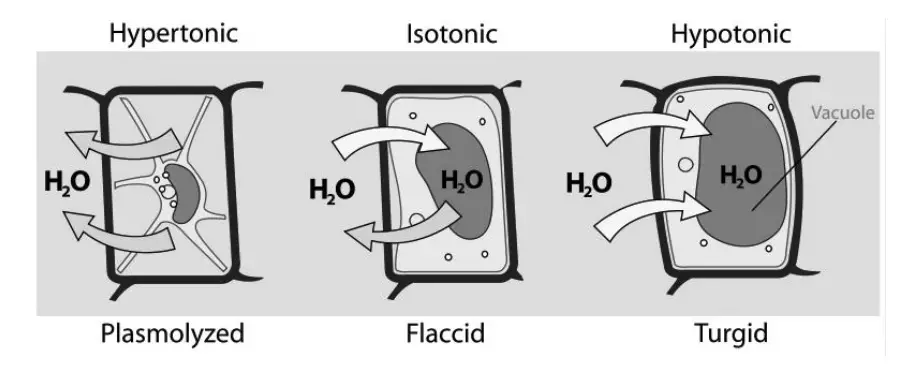
Significance of Osmosis in Plants
The following points outline the significance of osmosis in plants:
- Water Absorption by Roots
- Process: Osmosis facilitates the absorption of large quantities of water from the soil into plant roots. Root cells have a higher osmotic pressure compared to the surrounding soil solution, leading to water movement into the root cells.
- Function: This water uptake is essential for maintaining cell turgor and supporting overall plant health. It also provides the necessary hydration for various biochemical processes within the plant.
- Cell-to-Cell Movement of Water
- Process: Osmosis enables the movement of water and dissolved substances between adjacent plant cells. This movement occurs through plasmodesmata, which are channels connecting plant cells.
- Function: It ensures the distribution of nutrients and metabolic products throughout the plant, aiding in internal transport and cellular communication.
- Stomatal Function
- Process: The opening and closing of stomata, which are pores on the surface of leaves, are regulated by the turgor pressure of guard cells. Osmosis is involved in adjusting this turgor pressure.
- Function: Proper stomatal function is crucial for gas exchange, allowing carbon dioxide to enter for photosynthesis and facilitating the release of oxygen and water vapor.
- Maintenance of Turgidity
- Process: Osmosis contributes to the turgidity of plant cells by ensuring that the internal pressure is maintained. Water influx into the cell vacuole creates turgor pressure against the cell wall.
- Function: Turgidity helps maintain the structural integrity and shape of plant organs such as leaves and stems. It is vital for the plant’s ability to stand upright and capture light effectively.
- Resistance to Environmental Stress
- Process: Osmotic pressure within plant cells can increase as a response to environmental stress such as drought and frost. This is achieved through the accumulation of osmolytes and adjustments in cellular solute concentrations.
- Function: Enhanced osmotic pressure improves the plant’s resistance to water scarcity and extreme temperatures, allowing it to survive and adapt to adverse conditions.
- Seedling Emergence
- Process: Osmosis contributes to the turgidity of cells in young seedlings, which aids in their emergence from the soil.
- Function: The increased turgor pressure helps seedlings push through the soil, ensuring successful germination and establishment of new plants.
Osmotic pressure is a key concept in understanding the behavior of solutions and the movement of solvents through semi-permeable membranes. It is defined as the hydrostatic pressure developed in a solution due to the osmotic flow of solvent when separated from a pure solvent by a semi-permeable membrane. This pressure arises because the solvent molecules move to equalize the solute concentration on both sides of the membrane.
- Definition and Measurement
- Definition: Osmotic pressure is the pressure required to prevent the flow of solvent into a solution through a semi-permeable membrane. It is caused by the movement of solvent molecules from an area of lower solute concentration to an area of higher solute concentration.
- Measurement: Osmotic pressure is typically measured in units such as atmospheres, bars, or Pascals. An instrument used to measure osmotic pressure is known as an osmometer.
- Terminology
- Osmotic Pressure: This term was introduced by Pfeiffer and represents the pressure exerted by the solvent as it moves to equalize solute concentrations.
- Osmotic Potential: Modern terminology often prefers “osmotic potential” (Ψs), which is numerically equivalent to osmotic pressure but with an opposite sign. Osmotic pressure is positive, while osmotic potential is negative.
- Relationship with Solute Concentration
- Proportionality: Osmotic pressure is directly proportional to the concentration of dissolved solutes in a solution. Thus, a more concentrated solution will have a higher osmotic pressure.
- Effect of Solute Addition: The addition of insoluble solutes does not affect osmotic pressure. Osmotic pressure depends only on the concentration of solute particles that can dissolve and contribute to the osmotic gradient.
- Osmosis and Solvent Movement
- Direction of Movement: During osmosis, solvent molecules move from a solution with lower osmotic pressure (hypotonic solution) to a solution with higher osmotic pressure (hypertonic solution). This movement continues until equilibrium is reached or until the pressure equalizes.
Plasmolysis is a significant physiological phenomenon observed in plant cells when they are placed in a hypertonic solution. This process involves the shrinkage of the protoplast, the living content of the cell, due to the osmotic movement of water out of the cell. The following points detail the stages, effects, and applications of plasmolysis:
- Definition and Initial Stage
- Process: When a plant cell is immersed in a hypertonic solution, water exits the cell through exosmosis, causing the protoplast to shrink.
- Incipient Plasmolysis: In this early stage, the protoplast begins to detach from the cell wall at the corners as it contracts. The cell reaches its minimum volume at this stage, and further exosmosis leads to noticeable shrinkage.
- Complete Plasmolysis
- Process: If the exosmosis continues, the protoplast undergoes further contraction. It eventually pulls away entirely from the cell wall, forming a spherical shape within the cell wall.
- Characteristics: At this stage, the cell sap, protoplasm, and nucleus are concentrated in the center of the cell, leaving a space between the protoplast and the cell wall filled with the external hypertonic solution.
- Recovery and Deplasmolysis
- Process: When plasmolyzed cells are placed in water, endosmosis occurs, leading to the re-entry of water into the cell. This rehydration causes the protoplast to regain its normal shape and position against the cell wall.
- Characteristics: This recovery process, known as deplasmolysis, restores the cell to its original turgid state.
- Significance and Applications
- Indicator of Membrane Properties: Plasmolysis helps in assessing the semi-permeable nature of the plasma membrane, as it demonstrates how the membrane responds to osmotic pressures.
- Practical Applications:
- Preservation: The process is utilized in food preservation methods, such as salting meat and fish or adding concentrated sugar solutions to jams and jellies. These methods inhibit the growth of fungi and bacteria by causing plasmolysis of these microorganisms.
- Determination of Osmotic Pressure: Plasmolysis is used to determine the osmotic pressure (OP) of cell sap, providing insight into the osmotic properties of the cell.
- Educational Value
- Understanding Cell Behavior: Observing plasmolysis and deplasmolysis provides valuable information about cell behavior under varying osmotic conditions. It aids in understanding how cells adapt to changes in their environment and the role of osmotic pressure in maintaining cell integrity.
3. Imbibition
Imbibition is a fundamental process in plant physiology, representing a specific type of diffusion where water is absorbed by dry or partially dry materials, such as seeds, wood, and other plant tissues. This process is essential for the initial stages of seed germination and plays a crucial role in the movement of water within plant cells and tissues.
Key Concepts of Imbibition
- Imbibition Defined: Imbibition is the process by which water molecules are absorbed by hydrophilic (water-attracting) colloidal substances, such as cellulose, proteins, and other organic materials. This absorption occurs along a diffusion gradient, where water moves from an area of higher concentration to an area of lower concentration.
- Adsorbants: The materials that absorb water during imbibition are known as adsorbants. Common adsorbants in plant biology include fibers, cellulose in cell walls, and the protoplasm within cells. These substances have a high affinity for water, which enables them to draw in and retain moisture.
- Imbibitional Pressure (IP): As water is absorbed by the adsorbant, it creates pressure within the material, known as imbibitional pressure. This pressure is significant in the context of seed germination and the swelling of plant tissues, as it facilitates the movement of water into the cells.
Conditions Necessary for Imbibition
- Water Potential Gradient: For imbibition to occur, there must be a water potential gradient between the surface of the adsorbant and the surrounding liquid. Water potential is a measure of the potential energy of water in a system, and a gradient ensures that water will move from an area of higher potential (more available water) to an area of lower potential (less available water).
- Compatibility Between Adsorbant and Liquid: The adsorbant must be compatible with the liquid being imbibed. This compatibility is typically due to the hydrophilic nature of the adsorbant, which allows it to attract and hold onto water molecules. Without this compatibility, the process of imbibition cannot take place effectively.
The Role of Imbibition in Plant Physiology
- Germination of Seeds: Imbibition is the first step in seed germination. When a dry seed comes into contact with water, it absorbs the water through imbibition, causing the seed coat to swell and eventually rupture. This absorption is crucial for activating the metabolic processes that lead to germination and the growth of the seedling.
- Water Transport in Plant Tissues: Imbibition also plays a role in the transport of water through plant tissues, particularly in the cell walls. The cell wall’s ability to absorb water by imbibition facilitates the movement of water throughout the plant, contributing to overall hydration and nutrient transport.
- Exothermic Nature of Imbibition: Imbibition is an exothermic process, meaning that it releases heat as water is absorbed. This release of heat can be observed during the swelling of dry seeds or other plant materials when they come into contact with water.
Importance and Applications of Imbibition
- Seed Germination: The process of imbibition is vital for the successful germination of seeds. Without the initial absorption of water through imbibition, the seed’s metabolic processes cannot be activated, and germination will not occur.
- Cellular Hydration: Imbibition contributes to the hydration of plant cells, particularly in dry or drought conditions. By absorbing water from the surrounding environment, cells can maintain their turgidity and structural integrity.
- Agricultural Practices: Understanding imbibition is important in agricultural practices, especially in the pre-soaking of seeds to ensure rapid and uniform germination. Farmers and horticulturists often use this knowledge to optimize seed germination and improve crop yields.
Experiments Demonstrating Osmosis
Osmosis, a vital process in biological systems, can be effectively demonstrated through a series of classic experiments. These experiments highlight the movement of water across a semipermeable membrane from a region of lower solute concentration to a region of higher solute concentration. Two well-known experiments— the Egg Membrane Experiment and the Potato Osmoscope Experiment—illustrate the principles of osmosis in a clear and observable manner.
1. Egg Membrane Experiment
The Egg Membrane Experiment is a straightforward demonstration of osmosis, utilizing the natural semipermeable membrane found within an egg.
- Preparation of the Egg:
- Begin by carefully forming a hole on the pointed end of an egg.
- Extract the egg’s contents, including the white and yolk, leaving behind an empty eggshell.
- Submerge the empty eggshell in a dilute solution of hydrochloric acid (HCl) to dissolve the hard shell composed of calcium carbonate, leaving the underlying membrane intact.
- Setting Up the Experiment:
- Rinse the egg membrane thoroughly with water.
- Insert a glass tube into the membrane and secure it tightly with a thread, ensuring the end of the tube remains inside the egg membrane.
- Use a thistle funnel to fill the egg membrane sac with a concentrated sugar solution, allowing it to rise slightly into the glass tube.
- Suspend the sugar-filled egg membrane sac in a beaker of water, ensuring that the liquid levels inside and outside the sac are initially the same.
- Observations:
- Over time, the water from the beaker begins to move through the egg membrane into the sugar solution, causing the liquid level in the glass tube to rise.
- This rise continues until an equilibrium is reached, demonstrating the process of osmosis.
- To quantify osmotic pressure, one can apply pressure from the top of the funnel to halt the movement of water into the sac. The pressure required to completely stop this movement is termed as osmotic pressure, which is numerically equivalent to the osmotic potential but carries a negative sign.
- Reverse Osmosis:
- By reversing the setup—placing water inside the egg membrane sac and a sugar solution in the beaker—water will move from inside the sac to the outside environment.
- This process is known as reverse osmosis, where water moves against the concentration gradient, demonstrating the versatility of osmotic processes.
2. Potato Osmoscope Experiment
The Potato Osmoscope Experiment is another classic demonstration of osmosis, utilizing a potato tuber as a natural osmoscope.
- Preparation of the Potato:
- Select a potato tuber and peel it, creating a flat surface on one end.
- Carve a deep and broad cavity at the other end, ensuring the cavity walls are thin and the bed reaches close to the flat surface.
- Setting Up the Experiment:
- Fill the cavity with a concentrated sugar solution.
- Place the potato in a beaker of water, ensuring the water level inside the cavity is initially the same as the water level outside the potato.
- Observations:
- Over time, water begins to move from the beaker into the cavity of the potato due to the higher density of the sugar solution inside.
- The liquid level in the cavity rises, illustrating endosmosis, where water moves into the potato to equalize the concentration gradient.
- The potato tissue functions as a semipermeable membrane, allowing water to pass through while restricting the movement of solutes.
Importance and Applications
These experiments vividly demonstrate the principles of osmosis, providing clear visual evidence of how water moves through semipermeable membranes in response to concentration gradients. Such demonstrations are crucial for understanding fundamental biological processes, such as nutrient absorption in plants, water balance in cells, and the mechanisms underlying various physiological phenomena.
What is Water Potential?
Water potential (Ψ\PsiΨ) is a measure of the potential energy of water in a system, reflecting its capacity to perform work. It determines the direction and movement of water within plant tissues. Water potential is represented by the Greek letter Ψ and is expressed in units of pressure, typically in pascals (Pa).
Components of Water Potential:
- Matrix Potential (Ψ_m):
- Definition: Matrix potential represents the reduction in water potential due to the presence of colloidal substances within plant cells. These substances create attractive forces that decrease the availability of water.
- Expression: It is expressed as a negative value (–base) since it reduces the free energy of water.
- Solute Potential (Ψ_s):
- Definition: Also known as osmotic potential, this component accounts for the reduction in water potential due to the presence of dissolved solutes. The more solutes present, the lower the water potential.
- Expression: Solute potential is also expressed as a negative value (–base). It can be calculated using the van’t Hoff equation: Ψ_s = -miRT, where mmm is molality, iii is the ionization constant, RRR is the gas constant, and TTT is the temperature in Kelvin.
- Pressure Potential (Ψ_p):
- Definition: This represents the pressure exerted by the fluid within the cell against the cell wall, known as turgor pressure. It contributes positively to the water potential.
- Expression: It is typically a positive value (+base) but can be negative in certain contexts, such as tension in the xylem.
- Gravity Potential (Ψ_g):
- Definition: This is the contribution to water potential due to gravitational forces. It is usually negligible unless discussing the movement of water in very tall plants.
- Expression: Gravity potential is often ignored in most physiological contexts.
Equation for Water Potential:
The total water potential (Ψw) is the sum of its components: Ψw=Ψp+Ψs+Ψg
Importance of Water Potential:
- Cell-to-Cell Water Movement: Water moves from areas of higher water potential (less negative) to areas of lower water potential (more negative). This movement is essential for maintaining hydration and nutrient transport in plants.
- Turgidity and Growth: In a turgid cell, the pressure potential (Ψp) and solute potential (Ψs) balance out, resulting in no net water movement (Ψw=0). Conversely, in a flaccid cell, the pressure potential is zero, and the water potential equals the solute potential (Ψw=Ψs).
Absorption of water by plants
The absorption of water by plants is a crucial physiological process that sustains their growth and overall health. The primary organ involved in this process is the root system, which interacts with various forms of soil water. Understanding the different types of soil water and their availability is essential for comprehending how plants acquire the water necessary for their functions.
Types of Soil Water
- Gravitational Water:
- Definition: Gravitational water refers to water that moves through the soil due to the force of gravity.
- Characteristics: This type of water is primarily found in the larger soil pores known as macropores. It drains rapidly away from the soil profile, often reaching the water table.
- Availability to Plants: Gravitational water is typically not available to plants because it is quickly lost from the soil unless the soil is very compact.
- Capillary Water:
- Definition: Capillary water is held within the smaller soil pores, or micropores, and constitutes the soil solution.
- Characteristics: It is retained in the soil due to the cohesive and adhesive forces of water molecules. As soil moisture decreases, the size of the pores increases, leading to a conversion of capillary water into gravitational water, which then drains downward.
- Availability to Plants: Capillary water is the primary source of water for plants. It is readily available to plant roots due to its presence in the soil solution adjacent to the root system.
- Hygroscopic Water:
- Definition: Hygroscopic water forms a thin film around soil particles and is bound tightly due to adhesion.
- Characteristics: This water is held so strongly to soil particles that it is generally not accessible to plant roots. It is found on soil particles rather than in the soil pores.
- Availability to Plants: Hygroscopic water is largely unavailable to plants, especially in soils with low porosity, such as clays, which contain a higher percentage of hygroscopic water.
Mechanisms of Water Absorption
- Root Uptake:
- Process: Water absorption begins when plant roots take up capillary water from the soil. This process involves osmosis, where water moves from an area of higher water potential in the soil to an area of lower water potential within the plant root cells.
- Function: The root system’s extensive surface area and specialized structures, such as root hairs, enhance the efficiency of water uptake.
- Soil-Water Relationship:
- Influence of Soil Types: The type and texture of soil impact the availability and movement of water. For example, sandy soils with large pores retain less capillary water compared to clayey soils with smaller pores.
- Soil Moisture Management: Effective water management in agricultural practices involves understanding the balance between different soil water types to ensure adequate moisture levels for plant growth.
Factors affecting water absorption
The factors that regulate water absorption can be categorized into external and internal factors.
1. External Factors
- Availability of Soil Water: The availability of water in the soil is a primary determinant of water absorption by plants. When soil is physically dry, water absorption is significantly reduced due to the minimal presence of water. Similarly, in physiologically dry soil, which occurs when there is high salinity, water absorption is also limited. In addition, low oxygen content in the soil can impede the rate of water absorption, as roots require oxygen for respiration, which powers the active uptake of water.
- Soil Temperature: Soil temperature plays a crucial role in water absorption. Low temperatures increase the viscosity of water, making it more difficult for plants to absorb. This reduced water mobility, combined with a decrease in root permeability and growth, results in minimal water absorption. Conversely, excessively high temperatures can damage plant tissues and reduce water absorption capacity, leading to stress and potential plant injury.
- Soil Aeration: Proper soil aeration is essential for effective water absorption. High levels of carbon dioxide (CO₂) in poorly aerated soils can decrease the rate of water absorption. In soils with poor aeration, the lack of oxygen further hinders root respiration and, consequently, the active transport of water into the plant.
- Concentration of Soil Solution: In soils with high salinity, water absorption is reduced due to the osmotic stress imposed on plant roots. Physiologically dry soils, characterized by high solute concentrations, create an unfavorable environment for water uptake, as the roots must overcome the osmotic gradient to absorb water.
2. Internal Factors
- Root Pressure: Root pressure is a driving force for water absorption in plants, especially in situations where transpiration is low, such as during the night. Root pressure pushes water upward from the roots to the rest of the plant, facilitating water absorption.
- Transpiration: Transpiration, the process of water loss from the aerial parts of the plant, creates a suction force known as the transpiration pull. This pull increases the suction pressure within the plant, promoting the upward movement of water from the roots to the leaves. Therefore, higher rates of transpiration positively affect water absorption by increasing the driving force for water movement.
- Metabolism: The rate of respiration in plant roots directly influences water absorption. Higher metabolic activity in the roots leads to increased energy availability for active transport processes, enhancing the rate of water uptake from the soil.
- Root Structure: The structure of the root system, including the root-to-shoot ratio, plays a significant role in water absorption. A higher root-to-shoot ratio generally results in greater water absorption, as more root surface area is available to absorb water from the soil.
Water Transport in plant cells
Below, we discuss the key methods of water transport in plant cells.
1. Active Non-Osmotic Absorption of Water
- Definition: Active non-osmotic absorption occurs when water is absorbed into plant cells even if the osmotic potential (O.P.) of soil water is higher than that of the cell sap.
- Mechanism: This process does not follow the osmotic gradient and requires the expenditure of metabolic energy. It is thought to involve cellular respiration to generate the necessary energy.
- Significance: This type of absorption allows plants to take up water even when external conditions are not optimal, thus supporting survival in varying environmental conditions.
2. Passive Absorption of Water
- Definition: Passive absorption of water primarily occurs when the rate of transpiration is high.
- Mechanism: The process is driven by the tension created in the water within the xylem due to rapid evaporation from the leaves. This tension is transmitted through the xylem from the roots to the leaves, pulling water upward.
- Significance: Passive absorption relies on the plant’s ability to lose water through transpiration and is crucial for maintaining water flow through the plant system.
3. Pathways of Water Movement in Roots
Water absorption by roots involves different pathways, each contributing to the overall process. The primary pathways are:
- Apoplastic Pathway
- Description: Water moves through the cell walls and intercellular spaces.
- Function: This pathway allows water to bypass the cell membrane, facilitating relatively quick movement through the root.
- Transmembrane Pathway
- Description: Water crosses the plasma membranes of cells.
- Function: This pathway involves selective permeability, which regulates the entry and exit of water and solutes, maintaining cellular homeostasis.
- Symplastic Pathway
- Description: Water moves through the plasmodesmata, which are channels connecting adjacent cells.
- Function: This pathway allows for the direct exchange of water and nutrients between cells, contributing to a cohesive transport system within the plant.
4. Integration of Pathways
- Combination of Pathways: The actual mechanism of water absorption in roots typically involves a combination of the apoplastic, transmembrane, and symplastic pathways.
- Relative Importance: While the exact contribution of each pathway can vary, the integration of these pathways ensures efficient water uptake and transport throughout the plant.
Mechanism of absorption of water
The absorption of water by plant roots is a vital process essential for maintaining plant hydration and nutrient transport. This process can be categorized into two main mechanisms: active absorption and passive absorption. Each mechanism operates under different conditions and utilizes distinct physiological processes.
- Active Absorption of Water
- Definition and Process: Active absorption involves the expenditure of metabolic energy to facilitate the movement of water into plant cells. This process predominantly occurs in the root hairs of the plant, where the cells are in direct contact with the soil water.
- Mechanism:
- High Osmotic Pressure: Root hair cells have a high osmotic pressure due to the concentration of solutes in their cell sap. This creates a lower water potential inside the root cells compared to the soil solution, which is hypotonic in nature.
- Endosmosis: Water moves from the soil into the root cells through endosmosis, driven by the osmotic gradient. This movement of water helps in maintaining the cell’s turgor and supporting physiological functions.
- Energy Requirement: Active absorption requires metabolic energy, typically derived from ATP. This energy is used to actively transport ions and maintain the osmotic gradient.
- Conditions: This type of absorption is more prominent under conditions where atmospheric humidity is high and stomata are closed, such as during nighttime. In these situations, transpiration is reduced, and water absorption must be actively maintained.
- Passive Absorption of Water
- Definition and Process: Passive absorption occurs without the direct expenditure of metabolic energy. It is associated with the plant’s transpiration process, which creates a suction force that drives water movement from the soil into the roots.
- Mechanism:
- Transpiration and Suction Force: Water loss from the leaves through transpiration creates a negative pressure or suction force in the mesophyll cells. This suction force is transmitted through the xylem vessels in the stem and roots.
- Water Movement: The negative pressure in the xylem vessels draws water from the cortical cells of the roots, which, in turn, draw water from the soil. This movement of water through the plant is facilitated by the continuous channel formed by the xylem and cortical cells.
- Role of Roots: In passive absorption, the roots function primarily as channels for water movement rather than actively driving the process. The majority of water uptake (approximately 98%) in rapidly transpiring plants occurs through passive absorption.
- Conditions: Passive absorption is most effective during periods of active transpiration when there is a significant loss of water from the plant’s leaves, creating a strong suction force.
Ascent of sap
The ascent of sap refers to the upward movement of water from the roots to the aerial parts of the plant. This essential process ensures that water and dissolved nutrients are distributed throughout the plant, including to the leaves and other upper structures. The ascent of sap involves several mechanisms and can be studied under two primary aspects: the path and the mechanism of ascent.
Path of Ascent of Sap
- Xylem as the Conduit
- Role of Xylem: The ascent of sap primarily occurs through the xylem vessels. Xylem tissues are specialized for the transport of water and minerals from the roots to the rest of the plant.
- Ringing Experiment: To demonstrate the role of xylem, the ringing experiment is conducted. In this procedure, a ring of bark (all tissues outside the vascular cambium) is removed from a stem. When a leafy twig with such a ring is placed in water, the leaves above the ring remain green and healthy. This observation confirms that the water is being transported upward through the xylem vessels below the ring.
Mechanism of Ascent of Sap
The ascent of sap involves complex processes, especially in tall trees where water needs to travel significant distances. Several theories have been proposed to explain this phenomenon:
- Vital Theories
- Relay Pump Theory: Proposed by Godlewski, this theory suggests that the upward movement of sap is driven by the pumping activity of living cells in the xylem parenchyma. These cells, along with medullary rays, alter their osmotic pressure to facilitate the ascent.
- Pulsation Theory: Introduced by Bose, this theory posits that the pulsatory activities of living cells in the inner cortical layer just outside the endodermis drive the upward movement of water.
- Root Pressure Theory
- Concept: Formulated by Priestly, this theory explains that root pressure contributes to the ascent of sap. Water absorbed by root hairs accumulates in the cortical cells, causing them to become turgid. The pressure exerted by these cells pushes the water into the xylem vessels. This intermittent pumping action creates sufficient pressure in the roots to force water upward through the plant.
- Physical Force Theories
- Cohesion-Tension Theory: Proposed by Dixon and Joly, and further developed by Dixon, this theory is widely accepted today. It is based on three main principles:
- Continuous Water Column: A continuous column of water is maintained from the roots through the stem to the leaves, primarily within the tracheary elements (vessels and tracheids). The column remains intact due to the forces of cohesion, adhesion, and surface tension.
- Cohesion and Adhesion Forces: Water molecules exhibit cohesion, where they stick to each other via hydrogen bonds, allowing the water column to withstand significant tension. Adhesion, the attraction between water molecules and the walls of tracheary elements, further supports the water column’s integrity.
- Transpiration Pull: Water vapor loss from the mesophyll cells of leaves through transpiration creates a negative osmotic potential, resulting in a negative water potential. This deficiency in the mesophyll cells draws water from the xylem, creating a pulling force or tension that is transmitted through the xylem vessels, lifting water from the roots to the leaves.
- Cohesion-Tension Theory: Proposed by Dixon and Joly, and further developed by Dixon, this theory is widely accepted today. It is based on three main principles:
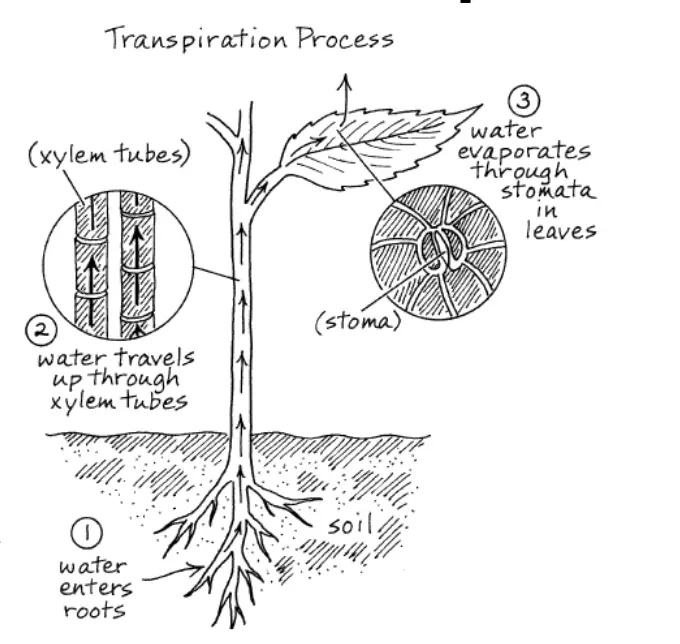
FAQ
What is the role of water in plant physiology?
Answer: Water is essential for various physiological processes in plants, including photosynthesis, nutrient transport, and temperature regulation. It acts as a solvent for nutrients, helps in the formation of plant tissues, and maintains cell turgidity, which is crucial for structural support and growth.
What are the different mechanisms of water absorption in plants?
Answer: Water absorption in plants occurs through two main mechanisms: active and passive absorption. Active absorption involves the use of metabolic energy to transport water into the roots, typically occurring under conditions of low atmospheric humidity. Passive absorption relies on transpiration to create a suction force that draws water up through the plant, with minimal metabolic energy expenditure.
How does osmosis affect water movement in plants?
Answer: Osmosis is the movement of water across a semi-permeable membrane from an area of lower solute concentration to an area of higher solute concentration. In plants, osmosis enables the absorption of water from the soil into the root cells, facilitates the movement of water between cells, and maintains cell turgor pressure, which is vital for plant stability and growth.
What is transpiration, and why is it important for plants?
Answer: Transpiration is the process through which water vapor is lost from the plant’s aerial parts, mainly through the leaves. It is crucial for cooling the plant, facilitating nutrient uptake, and maintaining water balance. Transpiration creates a negative pressure within the plant, which aids in the upward movement of water and minerals from the roots to the leaves.
What are the different types of transpiration, and how do they differ?
Answer: There are four main types of transpiration:
Stomatal Transpiration: Occurs through the stomata on the leaves and is the most significant form, contributing 50-97% of total transpiration.
Cuticular Transpiration: Happens through the cuticle on the surface of leaves and other parts, accounting for 3-10% of total transpiration.
Lenticular Transpiration: Takes place through lenticels in woody stems, contributing about 0.1% of total transpiration.
Bark Transpiration: Occurs through the corky bark of stems, with a rate that is often higher than lenticular transpiration due to a larger surface area.
- 1. Kochhar SL, Gujral SK. Plant–Water Relations. In: Plant Physiology: Theory and Applications. Cambridge University Press; 2020:3-50.
- https://www.embibe.com/exams/plant-water-relation/
- https://web.archive.org/web/20170808165040id_/http://rubisco.ugr.es/fisiofar/pagwebinmalcb/contenidos/Tema14/relaciones_hidricas.pdf
- https://sscwtumkur.org/sscwcms/uploads/activities/permanent/2020/05/22/116_1590156564_III_BSc_Botany_paper_7_Unit_1_plant_water_relation_-Ashwath_siddagannga.pdf
- https://ainfo.cnptia.embrapa.br/digital/bitstream/item/59779/1/InTech-Plant-water-relations-absorption-transport-and-control-mechanisms.pdf
- https://www.onlinebiologynotes.com/plant-water-relations-and-water-potential-diffusion-osmosis-and-imbibition/
- https://www.shivajicollege.ac.in/sPanel/uploads/econtent/949859765d0323ad4cea6cc22591f5e3.pdf
- https://www.slideshare.net/slideshow/plant-physiologywater-relations/251440537
- https://www.geeksforgeeks.org/plant-water-relations/
- https://egyankosh.ac.in/bitstream/123456789/71729/1/Unit-1.pdf
- https://www.vedantu.com/biology/plant-water-relations
- https://unacademy.com/content/neet-ug/study-material/biology/plant-water-relation/