What is phase contrast microscopy?
Okay, so picture this: back in the 1930s, scientists were struggling to study things like living cells or bacteria under a microscope. Why? Because those tiny critters are practically see-through! You couldn’t get a clear look without dunking them in harsh dyes—which, you know, kinda killed them. Not exactly helpful if you want to watch how cells actually behave.
Then along comes Frits Zernike, this Dutch physicist with a lightbulb moment. He figured out how to turn the “invisible” into visible. How? By playing with the way light bends as it passes through a sample. His phase-contrast microscope didn’t just make faint blobs slightly clearer—it revealed details nobody had ever seen before. Suddenly, labs could observe living cells splitting, microbes squirming around, all in real time. No stains, no fuss. It was like giving biologists superpowers.
Funny thing is, Zernike’s big idea wasn’t an instant hit. Some folks thought it was just a niche trick. But fast-forward to 1953, and boom—he’s holding a Nobel Prize in Physics. Turns out, his invention wasn’t just a “microscope upgrade.” It cracked open entire fields like cell biology and virology. Even now, when labs study anything from cancer to pond scum, odds are they’re using a modern twist on Zernike’s genius. Moral of the story? Sometimes the coolest discoveries are the ones that let us see the world differently.
Principle of Phase Contrast Microscope
Unstained living cells present a significant challenge in conventional brightfield microscopy due to their minimal light absorption. This lack of absorption generates only minute variations in light intensity, rendering them nearly indistinguishable against the background. To address this limitation, phase-contrast microscopy emerged as a groundbreaking solution. Rather than relying on light absorption, the technique exploits subtle phase shifts—imperceptible delays in light waves as they traverse transparent specimens—and translates these shifts into measurable differences in brightness. This optical innovation, first conceptualized by Dutch physicist Frits Zernike in 1934, circumvented the need for chemical staining, thereby preserving the viability of living samples. By transforming imperceptible phase alterations into visible contrast, Zernike’s method unlocked unprecedented opportunities for observing dynamic cellular processes in their native state.
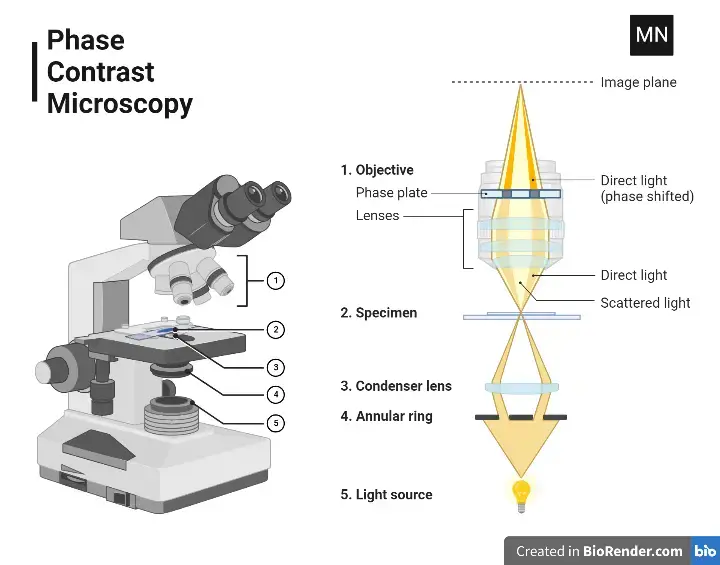
Light Path of Phase Contrast Microscope
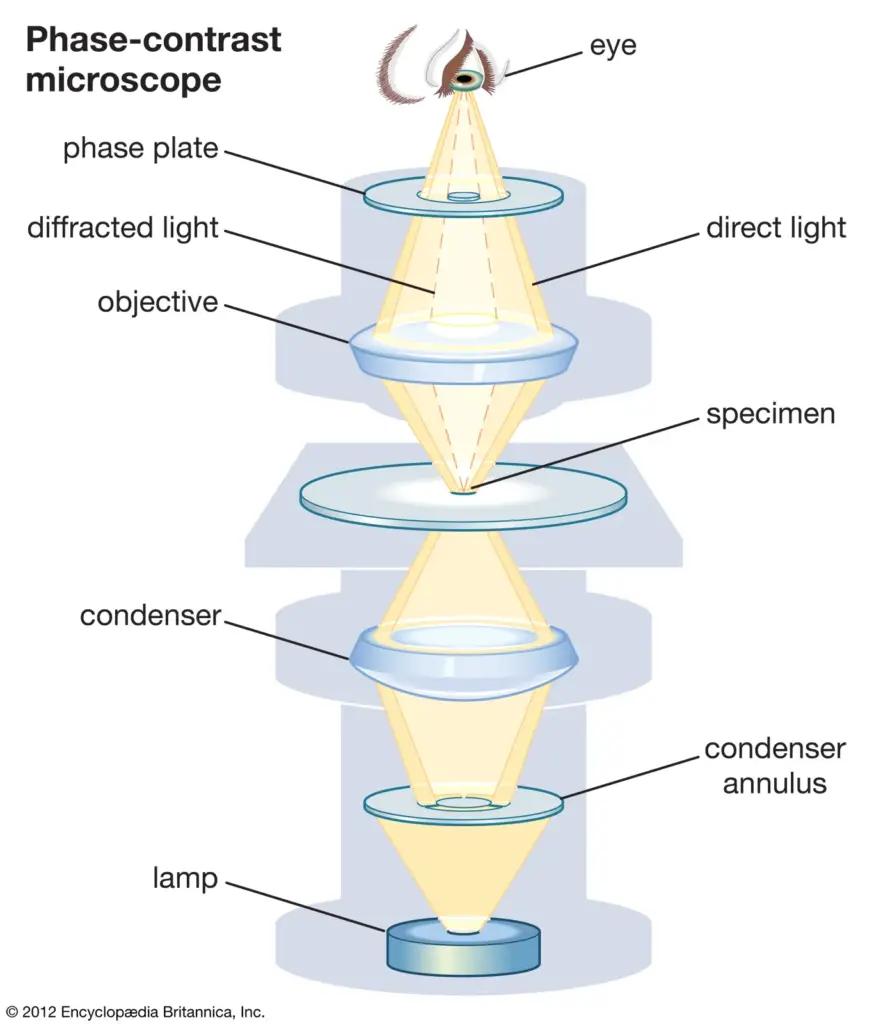
- A halogen or LED lamp supplies the necessary light to illuminate the specimen. This light is directed upwards through the microscope’s optical system.
- Positioned beneath the specimen, the condenser annulus—a ring-shaped aperture—focuses light into a hollow cone. This cone ensures precise illumination of the sample.
- Transparent structures within the specimen, such as cell membranes, alter the light’s phase. These shifts occur because light slows down when passing through denser materials, creating wave pattern differences.
- Both direct (unshifted) and diffracted (shifted) light rays are collected by the objective lens. Here, the lens focuses the light to produce an intermediate image.
- At the back of the objective lens, a phase plate introduces a quarter-wavelength phase shift only to the direct light. Diffracted light bypasses this adjustment, creating a detectable phase difference.
- When the two types of light recombine, phase differences are transformed into brightness variations. Denser regions appear darker, while lighter areas indicate less density.
- The final image is observed through an eyepiece or recorded using a camera. This allows unstained, live specimens to be studied without damage.
Types of Phase Contrast Microscope
- Positive Phase Contrast– In this setup, the phase plate applies a quarter-wavelength shift to direct light. Specimens with higher refractive indices—like cell nuclei—appear darker against a bright background. Researchers often use this type for dense samples, such as bacterial colonies or organelles, where clear dark-on-light contrast is needed.
- Negative Phase Contrast – Here, the phase shift is applied to diffracted light instead. This reverses the contrast: structures with higher density, such as cytoplasmic fibres, become brighter against a darker background. It’s particularly effective for visualising faint or translucent features that might be overlooked in positive phase contrast.
- Anoptral Phase Contrast– Anoptral systems use modified objective lenses to minimise halo artefacts—those distracting bright or dark rings around specimens. By reducing these optical distortions, sharper images of delicate samples, such as living cells or thin tissue sections, can be achieved. This makes it a preferred choice for high-detail microscopy.
- Variable Phase Contrast– Adjustable phase plates allow users to fine-tune the degree of phase shift. Flexibility is the key advantage here, as it accommodates samples with varying thickness or refractive properties. For instance, a lab studying both thick plant tissues and thin protozoan films might opt for this type to avoid switching microscopes.
- Long Working Distance Phase Contrast– These microscopes feature objectives with extra space between the lens and specimen. The design is ideal for thick samples, such as embryos or 3D cell cultures, or when tools like micromanipulators are used alongside imaging. The added clearance prevents physical contact between the lens and sample.
- Inverted Phase Contrast – With a flipped optical path—light source above the specimen—this type is optimised for viewing cells in culture dishes or flasks. Its design supports real-time observation of processes like cell division in adherent cultures, making it indispensable in cell biology labs.
Operating Procedure of Phase Contrast Microscopy
- Microscope Setup
Begin by positioning the instrument on a stable surface—vibrations can blur images. Dust or smudges on lenses should be gently removed with lens paper. Specimens must be thinly mounted on glass slides to prevent distortion during observation. - Light Source Adjustment
Activate the microscope’s illumination system, such as an LED or halogen lamp. Moderate brightness is key: excessive light creates glare, while insufficient light reduces contrast, masking subtle details. - Objective Lens Selection
Phase contrast objectives (e.g., 10x, 40x) are selected based on the desired magnification. Ensure the phase plate within the objective aligns with the condenser annulus size—a mismatch disrupts phase alignment. - Condenser Alignment
The condenser turret is rotated to choose the annulus corresponding to the objective. Adjust the condenser’s height using the focus knob until light converges sharply on the specimen. Proper alignment minimises artefacts. - Focusing the Specimen
Secure the slide on the stage with clips. Starting with the lowest magnification helps locate the sample. Coarse and fine focus knobs refine the image before higher magnifications are applied. - Phase Contrast Calibration
A phase telescope replaces one eyepiece to visualise the objective’s back focal plane. Centring screws on the condenser are adjusted until the annulus and phase plate rings overlap precisely. The eyepiece is reattached once alignment is complete. - Image Optimisation
Focus and lighting are fine-tuned to enhance clarity. Halos—bright or dark rings around specimens—can be reduced by slightly realigning the condenser or dimming the light source. - Observation and Documentation
Examine the specimen, noting structural features. Attach a camera to record time-lapse videos or still images, particularly useful for tracking processes like cell migration or division. - Post-Use Protocol
The lamp is switched off, and the stage lowered to its default position. Slides are removed, lenses cleaned if required, and the microscope covered to prevent dust accumulation.
How does phase contrast microscopy help scientists to visualize difficult specimens?
- Phase-contrast microscopy reveals transparent samples, like living cells, by making subtle phase shifts in light visible. To understand how this works, imagine light as waves. When light passes through a clear specimen, some waves scatter (foreground light) while others pass through unaffected (background light). These scattered waves are slightly delayed—about a quarter wavelength (90 degrees)—compared to the background due to differences in the specimen’s thickness or refractive index. Normally, this delay isn’t visible because our eyes can’t detect phase differences.
- Here’s where the microscope steps in. First, it separates the scattered light from the background light using a ring-shaped aperture in the condenser. This ring focuses a hollow cone of light onto the specimen. The unscattered background light continues straight, while the scattered light spreads out. Since scattered light is weak and phase-shifted, it blends with the background, creating minimal contrast.
- To fix this, the microscope modifies the two types of light differently. A phase-shift ring inside the objective lens delays the background light by another 90 degrees. This cancels out the initial delay, aligning the peaks and troughs of both light waves. When they recombine, they amplify each other (constructive interference), making specimen areas brighter against the background.
- But there’s more—background light is much stronger than scattered light, which can wash out details. A grey filter in the phase ring dims the background by 70-90%, balancing the intensities. This way, the scattered light stands out clearly.
- In negative phase contrast, the phase shift is reversed. Instead of aligning the waves, the background light is shifted +90 degrees, putting it completely out of phase with the scattered light. When combined, they cancel each other (destructive interference), creating a dark specimen against a bright background. This enhances edges and fine details, like outlining cell structures.
- By tweaking these shifts and filters, phase-contrast microscopy turns invisible phase changes into clear, high-contrast images—all without staining or harming delicate samples. It’s a bit like using special glasses to see hidden patterns in light waves, revealing the unseen world of transparent biology.
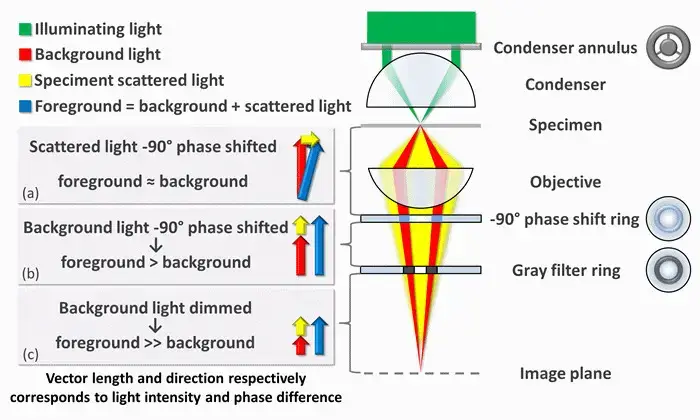
Parts of Phase Contrast Microscope
No. | Part | Description |
---|---|---|
1 | Phase Annulus in the Condenser | A black disc with a transparent ring that focuses light onto the specimen, creating a cone of light. It must match the phase plates in the objectives, with different sizes for different objectives. Provides partially coherent light to reduce optical noise and aligns with the phase plate for direct light alignment. |
2 | Phase Plate in the Objective | A transparent plate with a circular ring, often filled with phase-advancing or phase-delaying material. It modifies the light’s amplitude, creating positive or negative phase contrast for viewing denser organelles or features against varying backgrounds. The phase plate’s position is over the condenser’s image of the annulus, making the ring and annulus conjugate. |
3 | Phase Telescope | An eyepiece focusing the image of the back focal plane of the objective onto the observer’s retina, used for aligning the phase annulus with the phase ring. Some microscopes may use an integral Bertrand lens instead. |
4 | Green Filter | Utilizes green light (525 nm) for the phase contrast system, optimizing phase ring material for phase advancement or retardation. While green light is standard, phase contrast is also effective under white light. |
5 | Centering Tools | Tools provided for aligning the phase annulus in the condenser with the phase ring in the objective, essential for proper phase contrast imaging. This alignment is typically observed using the phase telescope. |
6 | Eyepiece | The part of the microscope through which the user views the specimen. |
7 | Head | The upper part of the microscope that holds the eyepiece and allows it to connect to the objectives. |
8 | Arm | The supportive structure that connects the head and the base, providing stability and a handle for carrying. |
9 | Base | The bottom part of the microscope that provides stability and supports the entire structure. |
10 | Nosepiece | The rotating part that holds the objectives and allows for easy switching between different magnifications. |
11 | Objective Lenses | The primary magnifying lenses that are positioned close to the specimen, each providing different levels of magnification. |
12 | Condenser Lens | Focuses light from the light source onto the specimen for clear illumination. |
13 | Specimen Stage | The platform where the specimen is placed for examination, typically equipped with clips to hold slides in place. |
14 | Stage Clips | Devices used to secure the specimen slide on the stage during examination. |
15 | Aperture | The hole in the stage through which light passes to illuminate the specimen from below. |
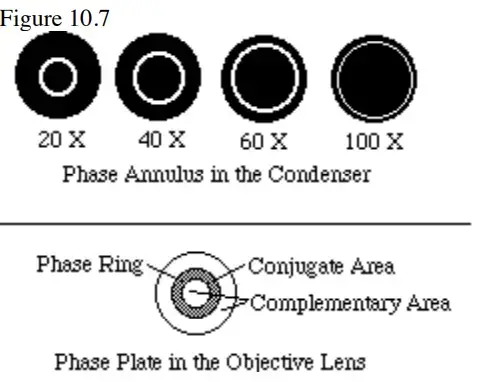
To grasp how phase contrast microscopy transforms transparent samples into clear images, let’s dissect its key parts and their roles. Each component collaborates to manipulate light waves, enhancing visibility without staining.
Phase Annulus (Condenser)
A circular plate with a dark background and a thin transparent ring. Positioned in the condenser, it filters incoming light into a hollow cone. This focused illumination ensures only specific light angles interact with the specimen, setting the stage for phase contrast.
Phase Plate (Objective)
Embedded in the objective lens, this plate adjusts light in two ways. It dims background light (reducing amplitude) and slightly delays light passing through the specimen (phase shift). These tweaks convert subtle sample density differences into visible contrast.
Phase Telescope
A temporary eyepiece used during setup. It acts like a magnifier to align the phase annulus’s ring with the phase plate’s corresponding structure. Misalignment blurs contrast—similar to tuning a radio for a clear signal.
Green Filter
Attached to the light source, this filter narrows illumination to green wavelengths (~550 nm). Green light minimizes lens distortions and matches the eye’s peak sensitivity, sharpening details.
Centering Tools
Adjustable screws or knobs on the condenser. These allow precise repositioning of the phase annulus to align with the phase plate—critical for crisp imaging.
Structural Framework:
- Eyepiece- The viewing lens (typically 10x magnification) that enlarges the image formed by the objectives.
- Head- Connects the eyepiece to the nosepiece. In binocular models, it splits the light path for dual-eye observation.
- Arm- A sturdy handle linking the base to the upper components. Always lift the microscope by this part to avoid instability.
- Base- A weighted platform providing stability, often housing the light source.
Optical and Positioning Components:
- Nosepiece- A rotating turret holding multiple objective lenses. Switching between lenses (e.g., 4x, 10x, 40x) alters magnification.
- Objective Lenses- Magnify the specimen at varying levels. Higher magnification lenses have shorter working distances.
- Condenser Lens- Focuses light into a tight beam directed at the specimen. Adjusting its height controls illumination intensity.
- Specimen Stage- A flat platform with mechanical controls to slide the sample horizontally (X-Y axis) for precise navigation.
- Stage Clips:– Metal clamps securing slides in place, preventing movement during high-magnification observation.
- Aperture (Iris Diaphragm)– An adjustable ring under the stage regulating light quantity. Wider apertures brighten images but reduce contrast; narrower apertures darken backgrounds to highlight edges.
Working Mechanism of the Phase Apparatus
Each component of the phase apparatus has a distinct effect. All of these processes combine to produce phase contrast. Depending on the design of the equipment, many forms of phase contrast can be produced.
Phase Annulus in the Condenser
- The annulus produces a hollow cone of illumination which converges on the specimen. Contrary to the dark field hollow cone, this ring of direct light reaches the objective lens’ aperture.
- The direct light generates a ring of illumination at the objective’s back focal plane. This ring of light is precisely the same size as the phase ring on the phase plate.
- In the specimen plane, the ring of light is at a focal point and, as a result, is a circular illumination patch that is solid.
- A portion of this light is diffracted by the specimen, and the diffracted light spreads across the entire rear focal plane of the objective and hence the entire phase plate (including over the phase ring).
Phase Plate in the Objective
- The phase ring has a thickness that differs from the rest of the phase plate such that it alters the phase of the direct light by 1/4 wave relative to the diffracted light (usually advancing the phase of the direct light).
- Additionally, the amplitude of the direct light is altered by making the phase ring darker than the remainder of the phase plate. There is usually a significant lot more direct light than diffracted light, making it darker.
- This direct light could overpower any amplitude difference caused by interference. The typical specimen retards the phase of diffracted light by 1/4 wave.
- The additional 1/4 wave advance provided to direct light by the phase ring leads direct and diffracted light to be approximately 1/2 wave out of phase. This configuration is optimal for maximising interference in the intermediate image plane.
Types of Phase Contrast
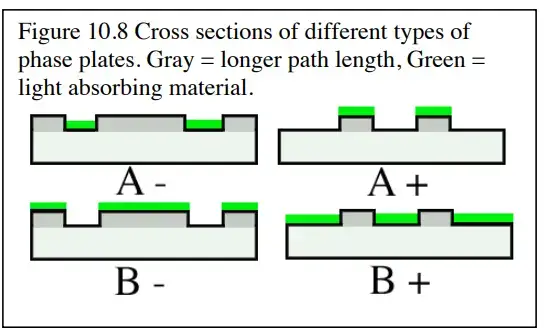
Setting Up Phase Contrast
Phase alignment occurs in the following ways for phase contrast:
- Kohler Bulb Alignment. Proper illumination must be obtained so the condenser is projecting almost parallel rays to the area where the specimen exists. This is achieved through the proper Kohler illumination, which positions the focused image of the light source at the condenser’s front focal plane. Thus, one needs to make sure the condenser aperture is wide open.
- Focusing on low power with the phase objective. Insert the correct phase ring into the phase annulus corresponding to your working objective.
- Remove an ocular, insert the phase telescope, and either focus the phase telescope on the phase ring or insert and focus the Bertrand lens.
- Assess the alignment of the phase ring to the phase annulus using a variety of alignment methods. For example, Figure 1 depicts a phase annulus (white) with a phase ring (gray) superimposed over it. This is out of alignment. Figure 2 shows what is aligned.
- Repeat steps 1-4 for all phase objectives and phase annuli. Once accomplished, the phase annuli will remain in alignment for quite a while. The only thing that will throw this alignment off is moving the objectives on the nosepiece.
- Switch to a 10X or 20X phase objective while in bright field and with the condenser still in place.
- Next, take off the diffuser from the illuminator and try to see the back focal plane of the objective through the phase telescope in order to center the filament on it. This is done through illumination controls; Figure 3 shows it misaligned and Figure 4 shows it aligned.
- You should now observe a thin filament coiled. The coils should be centered on the phase ring and fill the whole phase ring.
- Now change to the green filter. The width of the phase ring and the material it is composed of causes it to lead (or lag) green light by 1/4 wave. Change the eyepiece, switch to the appropriate phase annulus for the phase objective and enjoy.


Appearance of Phase Contrast Images
- Ideally, the appearance of a phase contrast image reflects the changes in refractive index and thickness of the specimen’s tiny features.
- It is commonly stated that phase contrast reveals edges. Below Figure depicts the most prevalent form of phase contrast, in which structures look darker than their surrounding medium.
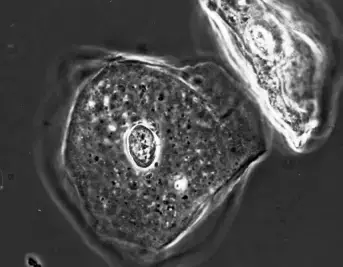
- Unfortunately, all phase contrast photos exhibit the same two artefacts. This is the result of the equipment used to make the image. Initially, a portion of the light that is diffracted by the specimen enters the phase ring and is consequently altered by it.
- Second, a portion of the direct light goes through the phase plate’s complementary region. The combination of these occurrences produces a phase image with the two artefacts listed below.
Phase Halo
- The phase halo is a diffuse ring that surrounds the specimen and substructures and is opposite in intensity to the specimen.
- In negative phase contrast, a black specimen on a light background will have a bright halo (note the nucleus of the cheek epithelial cell in figure 10.13).
- In positive phase contrast, a light specimen on a dark background will have a dark halo.
Shading Off
- The impact of shading occurs within the specimen itself. If the specimen is dark, there will be a central bright region that gradually fades into the dark body; if the specimen is light, there will be a central dark region that gradually fades into the light body.
- The shading-off effect is difficult to portray simply due to the specimen’s intricate arrangement of light and dark parts.
Applications of Phase Contrast Microscope
- Lets you peek at living cells without any dyes or chemicals
- Watch cells split, move, or do their thing in real-time
- Microbiologists spy on tiny organisms (like bacteria) up close
- Docs check blood samples stain-free for faster results
- Even works for studying plastics, fabrics, or other materials
- Helps labs track cell growth without tampering with them
- Eco scientists use it to study pond water or ocean plankton
Advantages
- Allows researchers to examine live cultured cells as it does not require fixation or staining.
- Allows for improved contrast with transparent, colorless preparations, as it makes internal structures more visible.
- Allows biological processes to be monitored as they occur within cells and tissues. Doesn’t kill the cells under observation.
- Is cheaper since it does not use expensive stains and dyes.
- Helps see very transparent or thin preparations that might be difficult to view under brightfield.
- Aids one’s understanding of such fine features of the cell, like cilia and flagella, which one would never be able to observe through other microscopic techniques.
Disadvantages
- Haloed samples- Samples may be haloed with a bright or dark haloing about features and lose detail.
- Not for thick samples – Phase contrast does not work well with thick sections because the phase shift may occur due to different focal planes and create a blurry image.
- Decreased resolution– Implementation of the phase contrast technique decreases the resolution of the imaging system based upon numerical aperture.
- Inverted contrast- The certainty that something is darker than the background may be true for high refractive index materials which could be rendered brighter versus darker.
- Expensive additional components – A phase contrast condenser and special objective lenses are expensive additions to the microscope.
- Not for stained slides- Stained slides work better with brightfield because phase contrast doesn’t pick up stained slides as well.
What is Phase Contrast? And When to use it?
Phase contrast refers to an imaging method in microscopy that enhances the visibility of small, transparent, or difficult-to-view samples by rendering the refractive index more obvious in differentiating between the materials that make up a sample. It involves viewing with something called “phase contrast light.” Phase contrast microscopy is typically utilized for tiny, transparent specimens or specimens that are otherwise difficult to see. This could include everything from human cells and tissue to microbes to tiny features of larger specimens.
For example, it can view live cells and tissues more effectively because it does not force the investigator to attempt staining or dyeing the specimen, which could be a hazardous process. Ultimately, the use of phase contrast microscopy advantages both the researcher and the practitioner because it improves observation of very small, transparent, or difficult-to-see samples and provides a greater appreciation of their structure and function.
How Is Phase-Contrast Implemented?
Two major limitations of the phase contrast microscope are that there must be a change in phase of the scattered light or the non-scattered light, but not both, and that the sample needs to be viewed under a specific phased orientation of light. This specifically uniform light did not exist until the laser came about in the 1960s.
They understood that a pinhole—even a tiny ring aperture that emitted light—produced a diverging light wave at first, yet it entered with a highly coherent phase—albeit a significant decrease in power. A lens could, in fact, in an instant, take such a circular wave and convert it to a plane wave. Thus, a plane wave of light is produced by an annulus, and phase-contrast is attempted with dim light and a coherent wave phase.
The annulus was as if they made a pinhole ring; every degree around the ring had the same phase, even if the phase oscillated in nonsensical patterns. To achieve a phase shift of the scattered or the intentional direct light, a phase-shifting optic (i.e., a glass disk) is placed along the pathway of the light where it becomes necessary for the intentional direct light. Below in Figure N is a linearized pathway of light where the intentional directed control light fades in from the left.
It scatters to the right under the interaction of the sample (solid lines to the right of the sample) and, subsequently, intentional direct (dashed) light. Here, the objective lens transforms the scattered light into a coherent wave as the intentional direct light converges to the optical center, the location of the phase-shifting material. Then, it remediates the phase uniformity of the scattered and intentional direct light, which enables the positioning of the detector to create contrast through interference. Below figure reveals a phase-contrast microscope utilizing annular illumination.
The location of the annular phase-shifting ring of a modern infinity-corrected microscope is at the rear focal plane of the objective. Therefore, to transform a typical bright field microscope into a phase-contrast microscope requires an annular diaphragm in the back aperture of the condenser and an optically matched internal phase plate.
The phase plate is typically a permanent etching that occurs to one of the internal lens elements of the objective, situated in-line with the optical path at the rear focal plane (this is the tube lens from above). This phase plate reduces the intensity of the undeviated light so that it more closely correlates with the intensity of the deviated light and therefore, even more, reduces the impact of the background light to the resultant image.
Because the particles in the specimen have different refractive indices, for example, the light that travels through the specimen will be refracted or diffracted in such a way as to create different optical paths. Yet the majority of these new optical paths will avoid the phase plate’s attenuating regions and instead proceed through the phase ring’s center where no attenuation occurs. It’s at the second imaging plane where the wavefronts add together that are diffracted to a greater or lesser extent by passing through the specimen as well as the laterally deflected undiffracted wavefronts.
There exists an aggregate phase shift of approximately 1 with the positive deviations—considering the 90° deviation which causes destructive interference—and an aggregate phase shift of almost 0 with the negative deviations which cause constructive interference. Therefore, even the slightest of RI changes can create drastic fluctuations in interference.
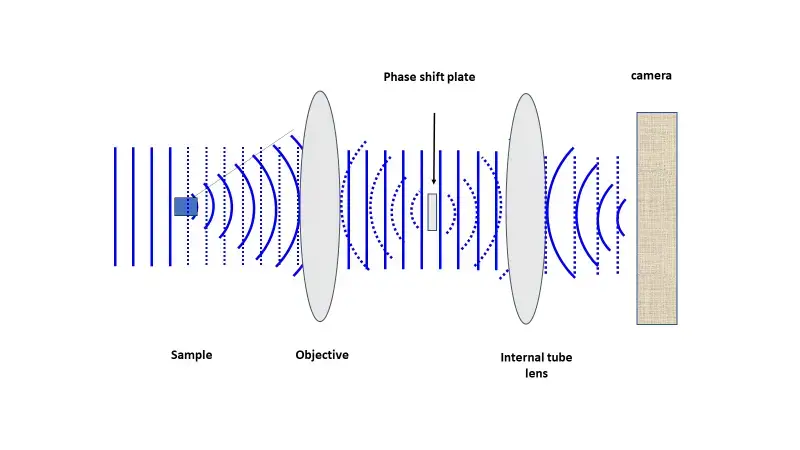
Thus, with positive phase contrast or negative phase contrast — the only two options for this methodology — those components with a higher RI than their surroundings will be dark in a bright field and bright in a dark field, respectively. This means that the majority of microscopes in use today have positive phase contrast, so the more obscured a specimen’s parts are, the more RI they have because to the human eye, it appears they’re taking in more. This is why the phase plate gets thinner in width and diameter as the numerical aperture and magnification increase.
The opposite is true of the aperture of the condenser annulus, as that gets larger as the magnification increases. Therefore, these are all factors of getting a proper phase contrast image, including the necessity of centering the annulus of the condenser so that the image of the annulus sits exactly over wherever the phase ring is. Below are samples of these various phase rings, the resulting image, and images of non-phase fields such as brightfield.
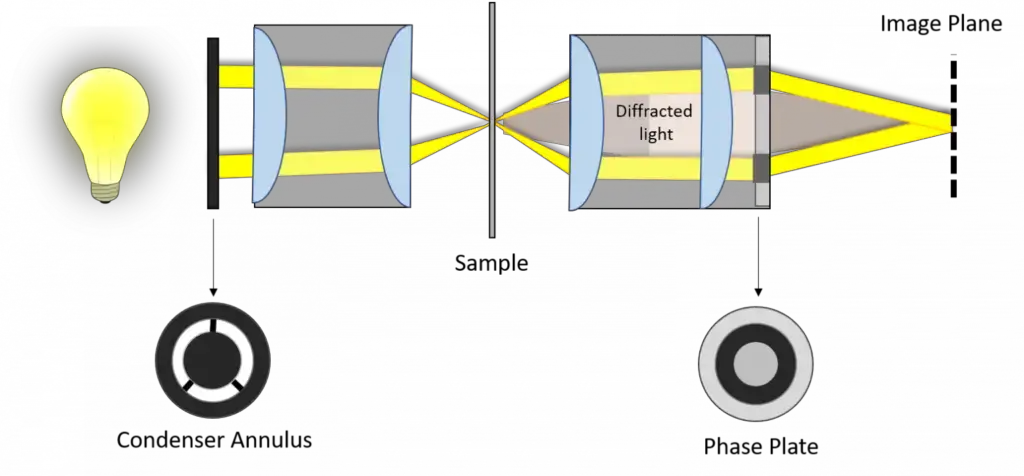
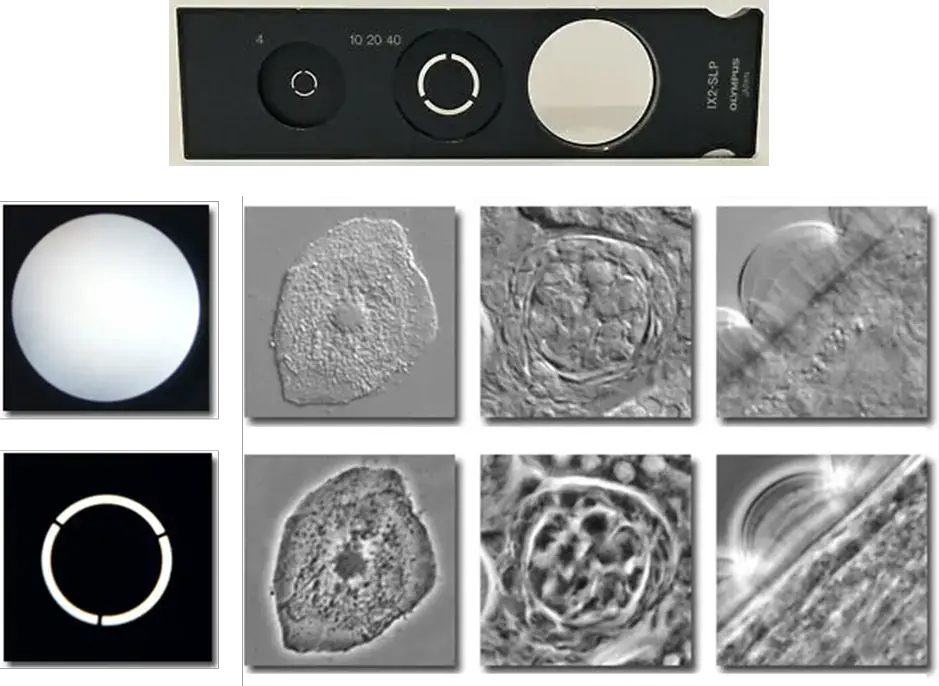
What Is Optical Path Length?
Light moves—within a vacuum—at 300,000 km/s. If it’s moving through another medium, however, it moves more slowly in respect to that substance’s refractive index (RI). In a sense, the denser the medium, the more it gets in light’s way. The greater the RI, the more it restrains light. Therefore, over time, one could argue that it takes light longer to travel at that consistent rate. Therefore, optical path length (OPL) equals the distance traveled in a medium multiplied by that medium’s refractive index. An OPL change will happen when two beams of the same wavelength start from the same location but take different paths to the same endpoint. As long as the path difference is not a complete multiple of the wavelength, a phase difference exists between them. Therefore, by having one beam travel a longer distance, one can induce in a specimen, among a slide, differences in phase of that which comes from the same specimen. This is how phase contrast relies upon.
What Is Light Phase?
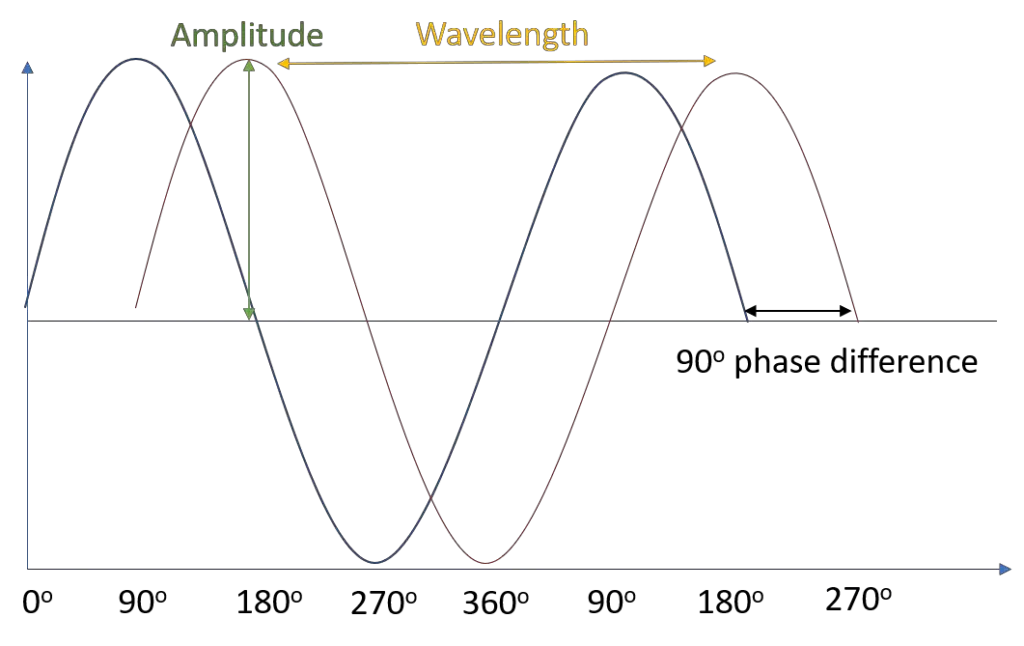
Mathematically, this is a sine wave. The larger the amplitude, the larger the intensity (the brightness perceived by the eye). Energy and color derive from the wavelength (and frequency, which is the reciprocal of wavelength). As shown in the figure, the red wave is out of phase with the blue wave. Phase has to do with when or where, at some point in the wave, something occurs relative to the sine wave (a maximum peak, minimum trough, etc.).
For example, two seagulls riding on the same wave. They may have the same wavelength and amplitude, distance on either side of the waterline; however, their phase is different. Mathematically, we measure phase in degrees or radians from a certain point along the sine wave; that is, if we declare the peak of the wave—where the seagull is tallest—to be 0°, then the trough is at 180° (π radians), with 90° (π/2 radians) being the halfway point between the two.
Therefore, the waves in Figure 1 are 90 degrees out of phase. Waves can combine together when they have like frequencies and phase relationships, and the resultant relationship depends on how in or out of phase the waves are relative to one another. For example, waves at the same frequency 180° out of phase align as a trough to a peak—and involve destructive interference—as they align at 0 amplitude since the two waves cancel one another out.
Yet, paradoxically, waves that are completely in phase align at peaks to peaks (0° phase difference) and thus, intrusively interfere and constructively add intensity measures together to create an overall wave of double amplitude.
How phase contrast works?
Phase contrast microscopy translates minor changes in phase into changes in amplitude (brightness), suggesting that the end result is a more contrasted image. Cells, when unstained and resisting absorbance, are considered phase objects. They are considered phase objects because they alter the phase of the light that they diffract—albeit, so minutely—typically one quarter wavelength out of sync with the surrounding background light.
Yet, the human eye responds only to intensity and wavelength changes and not to such intricately small phase differences. Phase contrast works by enhancing the interference in the light’s phase to produce a highly contrasted image. Essentially, the background light and the light emanating from the refracted specimen are out of phase. A phase plate, positioned just before the presumed image plane, retards (or boosts) the background light by one-quarter wavelength so that the enhanced differential between the two is even greater.
Then, at the image plane where one focuses a specimen, destructive (or constructive) interference occurs between the background and refracted light, thereby reducing (or increasing) the intensity of those areas where the specimen is relative to the background light. Before the specimen is encountered by the objective, light emitted by the tungsten-halogen lamp enters the condenser annulus of the substage condenser.
This means that nearly parallel, yet defocused light can be aimed at a specimen. The undiffracted light, now shining through the specimen (bright yellow region of the screen capture), does NOT become diffracted. These light rays enter the back aperture of the objective and form a bright, clear image. The rays that become diffracted encounter the plane of diffraction where they instead are focused at the image plane.
Thus, background illumination and diffracted light are separated. The annulus then increases the wavelength of the reference beam by a quarter wavelength. When the two beams recombine at the image plane, however, the diffracted and reference beams will undergo destructive or constructive interference.
Yet this is independent of which parts of the specimen were illuminated; instead, it counteracts the new wavelengths of the reference beam in relative intensity observed between portions of the specimen. For example, an annulus with a grey filter reduces the relative background intensity by 60-90%.
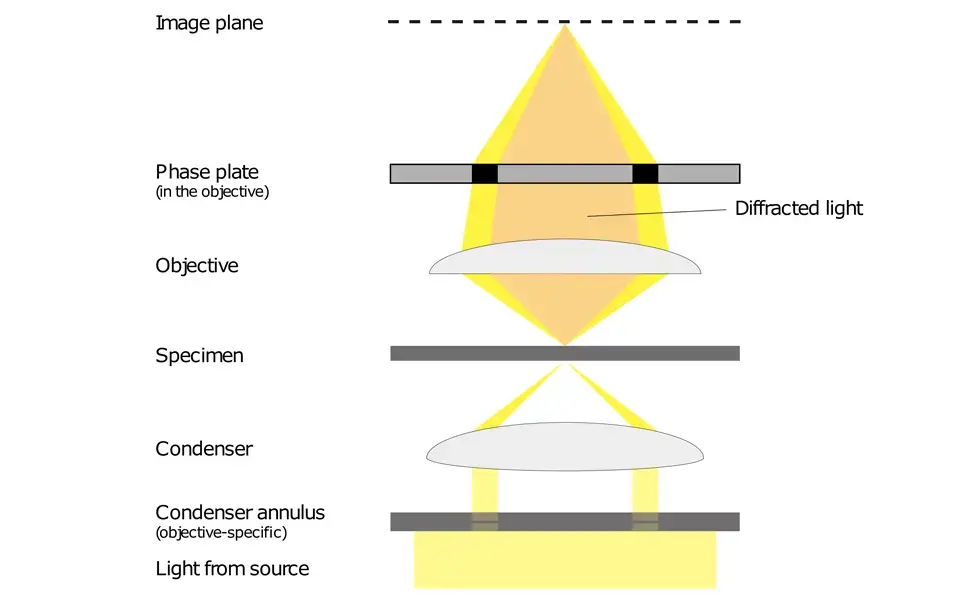
Phase contrast microscopy images
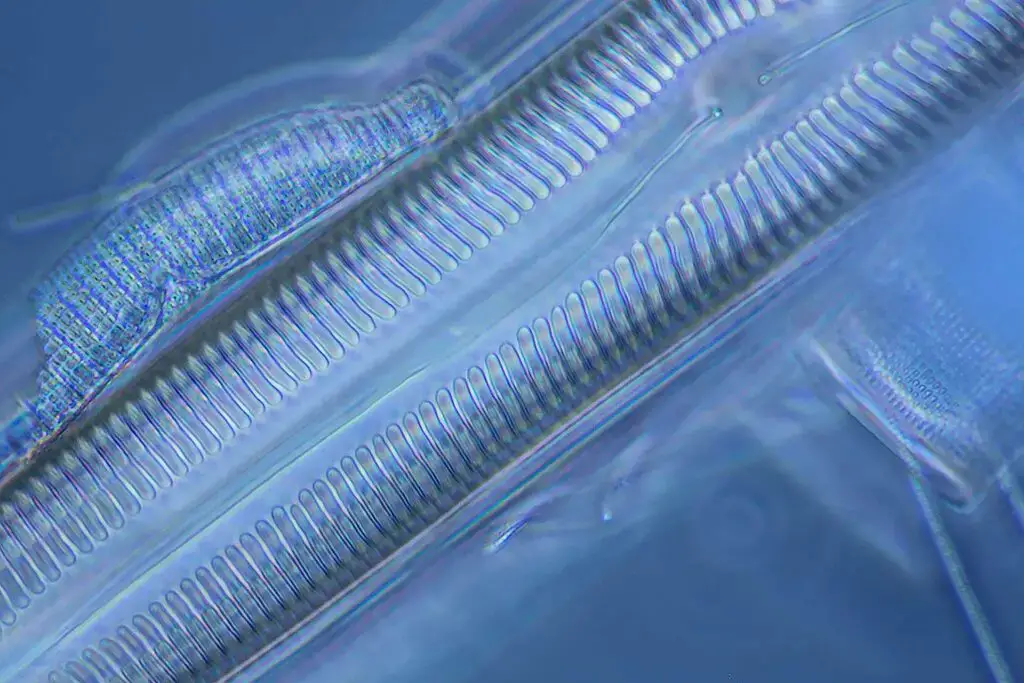
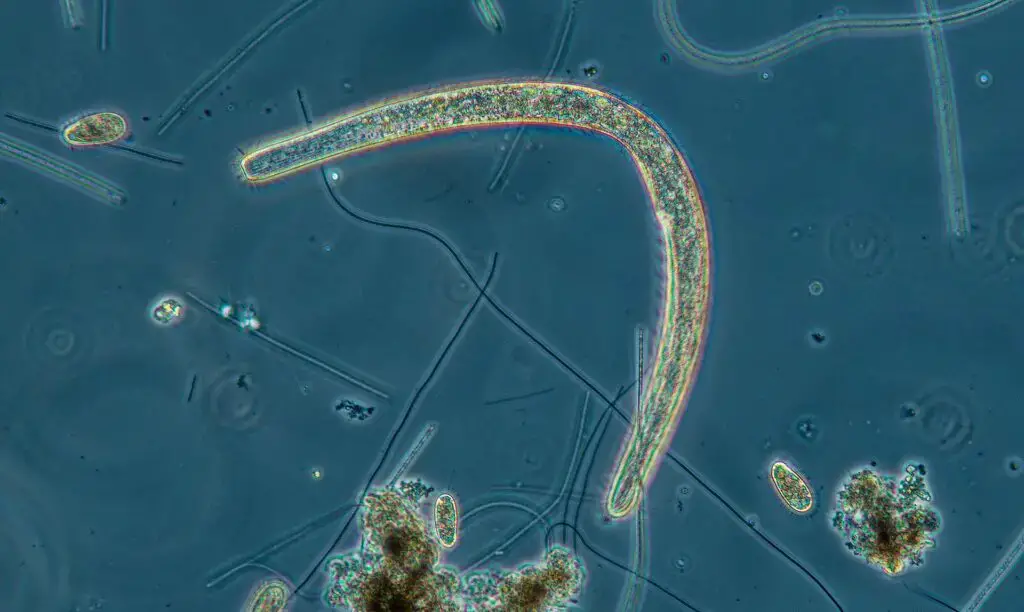
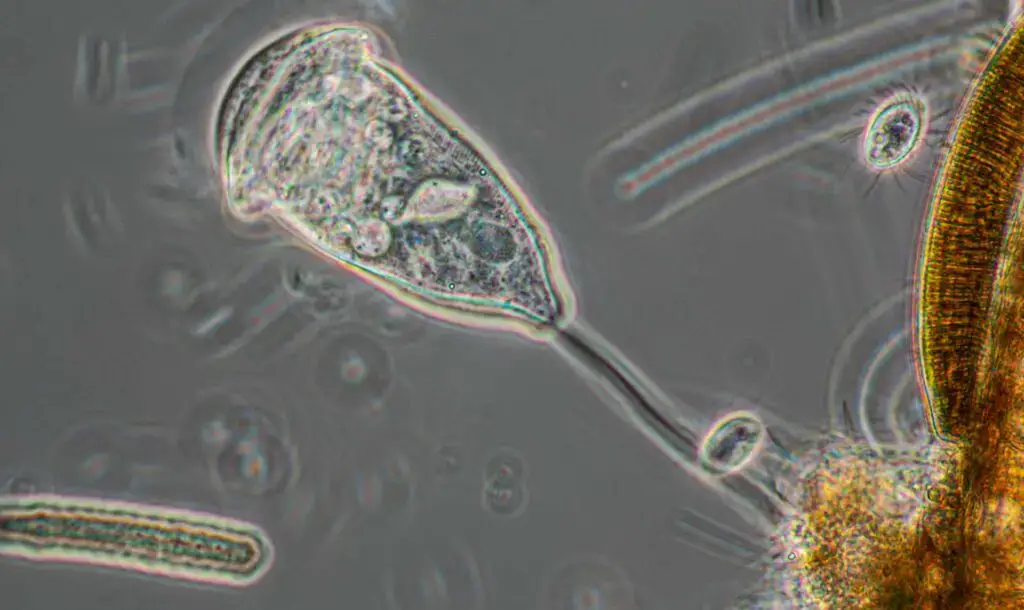
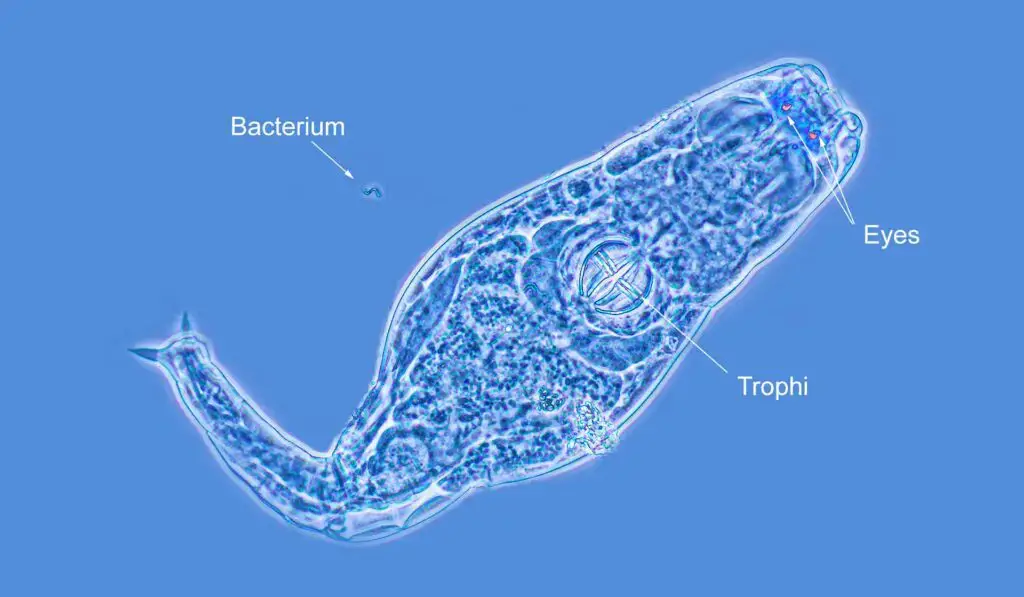
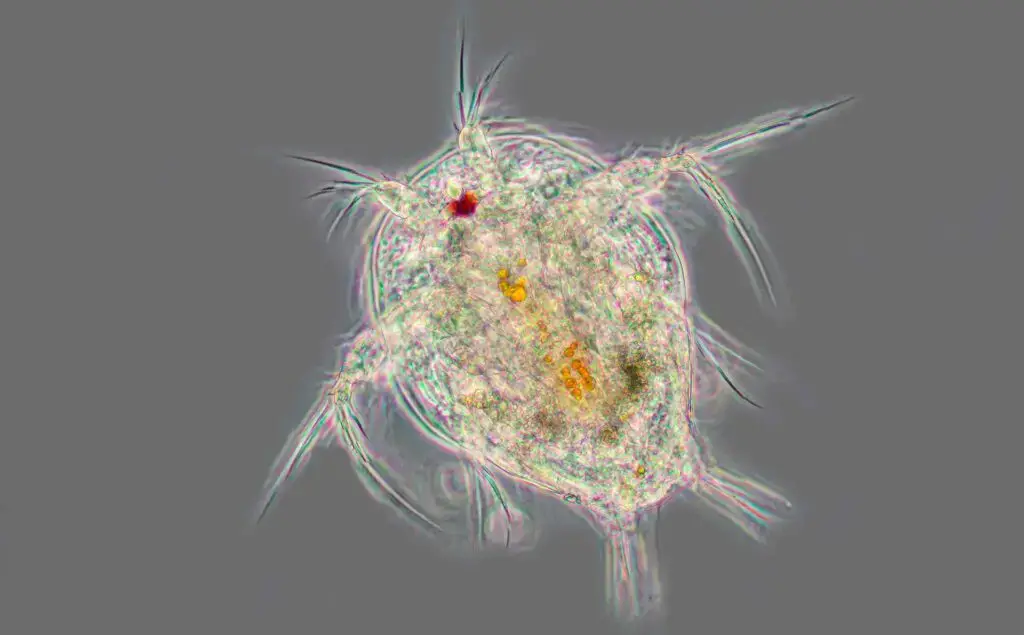
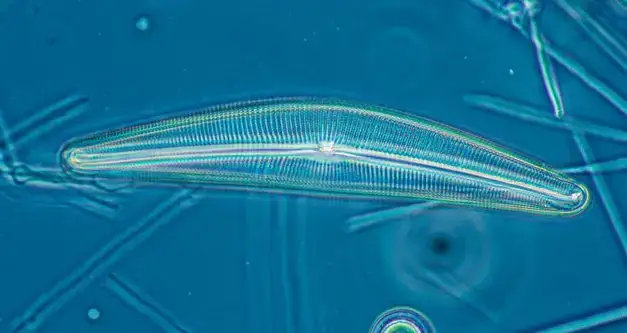
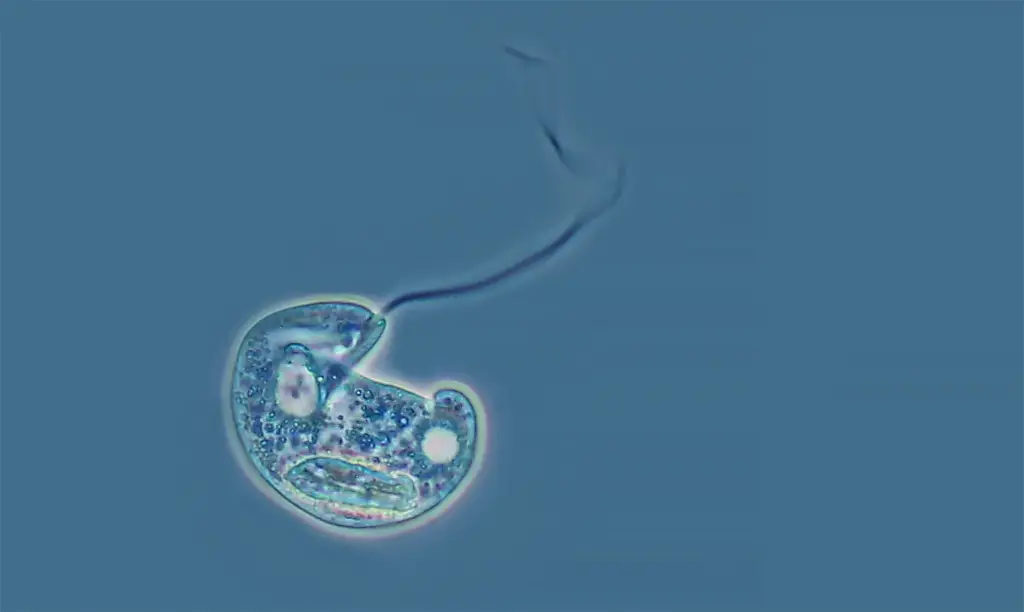
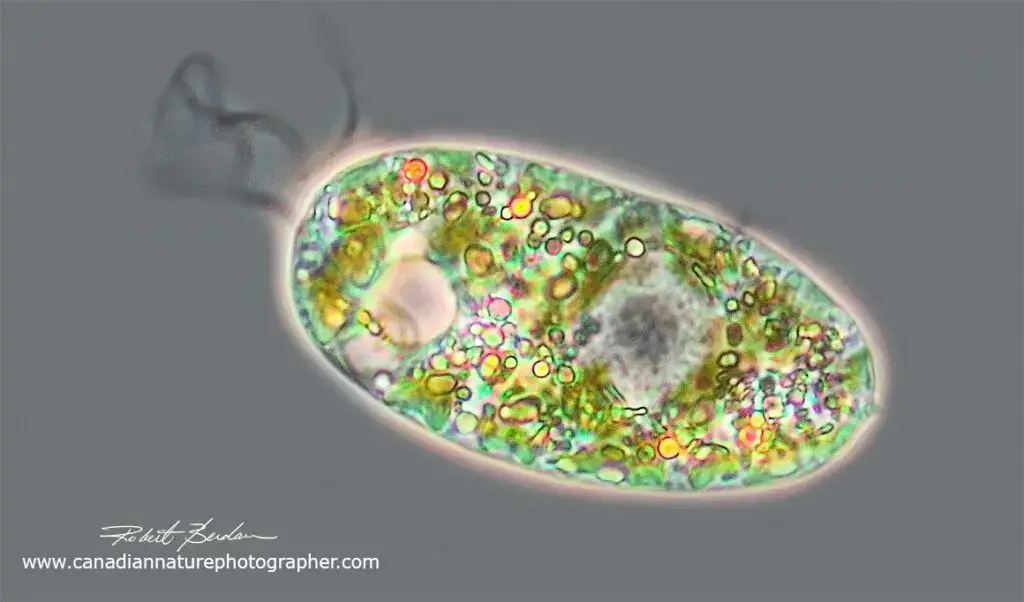
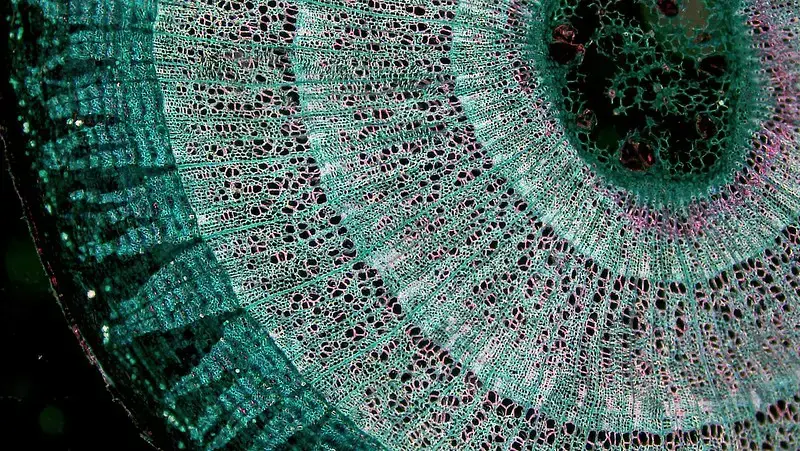
FAQ
What is phase contrast microscopy
Phase contrast microscopy is a microscopy technique that allows researchers to visualize small differences in the refractive index of a sample by using special optics to create contrast between phases of light passing through the sample. It is often used to visualize cells or other transparent or translucent biological samples that would otherwise be difficult to see with a regular light microscope.
In phase contrast microscopy, light from a lamp passes through a condenser and illuminates the sample. The light is then collected by a special objective lens that has a phase ring around its outer edge. The phase ring creates a phase shift in the light waves passing through the sample, which causes the light waves to interfere with each other. This interference creates a contrast between the phases of the light waves, making it easier to see small differences in the refractive index of the sample.
Phase contrast microscopy is a useful technique for studying living cells and other transparent or translucent samples because it allows researchers to see fine details within the sample without the need for staining or other techniques that might damage or alter the sample. It is often used in research on cell structure, development, and function, as well as in the study of microorganisms and other small biological specimens.
How does phase contrast microscopy help scientists to visualize difficult specimens?
Phase contrast microscopy helps scientists to visualize difficult specimens by creating contrast between the phases of light waves passing through the sample. This contrast makes it easier to see small differences in the refractive index of the sample, which can be difficult to see with a regular light microscope.
One reason that phase contrast microscopy is useful for visualizing difficult specimens is that it allows researchers to see transparent or translucent samples without the need for staining or other techniques that might damage or alter the sample. Staining can be difficult or impossible to use on living cells or other delicate specimens, and it can also alter the appearance of the sample in ways that make it difficult to interpret the results. Phase contrast microscopy, on the other hand, can be used to visualize living cells and other delicate specimens without causing any damage or alteration.
Phase contrast microscopy is also useful for visualizing small differences in the refractive index of a sample. The refractive index is a measure of how much a substance bends light as it passes through it. Some substances, such as cells and other biological materials, have a relatively low refractive index and are difficult to see with a regular light microscope. By using phase contrast microscopy, researchers can create contrast between the phases of light waves passing through the sample, making it easier to see small differences in the refractive index and visualize the sample more clearly.
Overall, phase contrast microscopy is a valuable tool for scientists who need to visualize delicate or transparent specimens, or who need to see small differences in the refractive index of a sample. It allows researchers to study living cells and other difficult-to-see specimens in greater detail, providing valuable insights into their structure, function, and other properties.
Which opening in the slider is appropriate for phase contrast microscopy using a 40x objective?
The appropriate opening in the slider for phase contrast microscopy using a 40x objective would depend on the specific microscope and phase contrast system being used. In general, the appropriate opening in the slider for phase contrast microscopy is the one that allows the light from the lamp to pass through the condenser and illuminate the sample at the optimal intensity.
To determine the appropriate opening in the slider for phase contrast microscopy using a 40x objective, you will need to consult the instruction manual for your microscope or consult with the manufacturer. In general, you may need to adjust the opening in the slider to fine-tune the intensity of the light passing through the condenser and onto the sample. It may also be necessary to adjust the focus and other settings on the microscope to optimize the image quality.
If you are using a phase contrast system that includes a special phase annulus or phase ring around the objective lens, you may need to adjust the position of the phase annulus or phase ring in order to optimize the contrast between the phases of light waves passing through the sample. This can also be done by consulting the instruction manual or the manufacturer’s recommendations.
Overall, it is important to carefully follow the instructions for your specific microscope and phase contrast system in order to achieve optimal image quality and contrast when using phase contrast microscopy.
When to use darkfield and phase contrast microscopy?
Darkfield microscopy and phase contrast microscopy are two microscopy techniques that are used to visualize small or transparent specimens that might be difficult to see with a regular light microscope. Both techniques use special optics to create contrast between the phases of light waves passing through the sample, which makes it easier to see small differences in the refractive index of the sample and visualize fine details within the specimen.
Darkfield microscopy is a technique that is used to visualize samples that are dark or transparent against a bright background. It is often used to visualize microorganisms and other small specimens that are difficult to see with a regular light microscope. In darkfield microscopy, light from a lamp is directed onto the sample from the side, rather than from directly above. This creates a bright background around the sample, making it easier to see the sample against the bright background.
Phase contrast microscopy is a technique that is used to visualize transparent or translucent samples that have small differences in their refractive index. It is often used to visualize living cells and other delicate specimens that might be damaged by staining or other techniques. In phase contrast microscopy, light from a lamp passes through a condenser and illuminates the sample. The light is then collected by a special objective lens that has a phase ring around its outer edge. The phase ring creates a phase shift in the light waves passing through the sample, which causes the light waves to interfere with each other and create contrast between the phases.
In general, darkfield microscopy is used when the sample is dark or transparent against a bright background, and phase contrast microscopy is used when the sample is transparent or translucent and has small differences in its refractive index. Both techniques can be useful for visualizing small or difficult-to-see specimens and can provide valuable insights into their structure, function, and other properties.
How are brightfield, darkfield, phase-contrast, and fluorescence microscopy similar?
Brightfield, darkfield, phase-contrast, and fluorescence microscopy are all microscopy techniques that are used to visualize small or difficult-to-see specimens. These techniques are similar in that they all use special optics and illumination to create contrast between different aspects of the sample, making it easier to see fine details and small differences within the specimen.
All four microscopy techniques use a microscope to magnify the image of the sample, and all four techniques can be used to study a wide range of samples, including cells, tissues, microorganisms, and other small specimens.
One way that these microscopy techniques are similar is that they all use lenses to focus light onto the sample and create an enlarged image of the sample. The lenses in a microscope are used to gather light from the sample and project it onto a viewing screen or digital detector, where it can be observed and studied.
Another way that these microscopy techniques are similar is that they all use special optics and illumination to create contrast between different aspects of the sample. Brightfield microscopy uses a bright background to create contrast between the sample and the background, while darkfield microscopy uses a dark background to create contrast between the sample and the background. Phase-contrast microscopy uses special optics to create contrast between the phases of light waves passing through the sample, and fluorescence microscopy uses a special type of light to excite fluorescent molecules within the sample and create contrast between the fluorescent and non-fluorescent parts of the sample.
Overall, brightfield, darkfield, phase-contrast, and fluorescence microscopy are all similar in that they are used to visualize small or difficult-to-see specimens and use special optics and illumination to create contrast within the sample. These techniques can provide valuable insights into the structure, function, and other properties of a wide range of samples and are widely used in research and other applications.
When to use phase contrast microscopy over dic?
Phase contrast microscopy and differential interference contrast (DIC) microscopy are two microscopy techniques that are used to visualize small or transparent specimens that might be difficult to see with a regular light microscope. Both techniques use special optics to create contrast between the phases of light waves passing through the sample, which makes it easier to see small differences in the refractive index of the sample and visualize fine details within the specimen.
There are several factors to consider when deciding whether to use phase contrast microscopy or DIC microscopy. One factor to consider is the type of sample you are studying. Phase contrast microscopy is often used to visualize living cells and other delicate or transparent specimens that might be damaged by staining or other techniques. It is also often used to study small differences in the refractive index of a sample, such as the boundaries between different cell structures. DIC microscopy, on the other hand, is often used to study samples with thin, transparent layers or samples with surface features that might be difficult to see with other techniques.
Another factor to consider is the level of detail you need to see in your sample. Phase contrast microscopy can be used to visualize fine details within a sample, such as the internal structure of cells or the surface features of microorganisms. DIC microscopy is also capable of visualizing fine details, but it is often better suited to studying larger features or structures within the sample.
A third factor to consider is the type of microscope you are using. Phase contrast microscopy and DIC microscopy require different types of objective lenses and special optics, so the choice between these techniques may depend on the capabilities of your microscope.
Overall, the decision to use phase contrast microscopy or DIC microscopy will depend on the specific needs of your research and the capabilities of your microscope. Both techniques can provide valuable insights into the structure, function, and other properties of small or transparent specimens, and the appropriate choice will depend on the specific characteristics of your sample and your research goals.
What is necessary for phase contrast microscopy?
Phase contrast microscopy is a microscopy technique that is used to visualize small or transparent specimens that might be difficult to see with a regular light microscope. In order to use phase contrast microscopy, you will need the following:
A microscope: Phase contrast microscopy requires a microscope with an objective lens that has a phase ring around its outer edge. This phase ring is used to create a phase shift in the light waves passing through the sample, which causes the light waves to interfere with each other and create contrast between the phases.
A light source: Phase contrast microscopy requires a light source to illuminate the sample. This can be a lamp or other light source that is built into the microscope, or it can be an external light source that is directed onto the sample through the microscope.
Special optics: Phase contrast microscopy also requires special optics, such as a condenser, to focus the light onto the sample and create an enlarged image of the sample.
A sample: Phase contrast microscopy can be used to study a wide range of samples, including cells, tissues, microorganisms, and other small specimens.
A viewing screen or digital detector: Phase contrast microscopy requires a viewing screen or digital detector to observe and study the image of the sample. This can be a traditional eyepiece or a digital camera or other electronic device that is attached to the microscope.
Overall, phase contrast microscopy requires a microscope with special optics and a phase ring around the objective lens, a light source to illuminate the sample, special optics to focus the light onto the sample, a sample to study, and a viewing screen or digital detector to observe the image of the sample. With these components, you can use phase contrast microscopy to visualize small or transparent specimens and study their structure, function, and other properties.
Why isnt phase-contrast microscopy used to view prokaryotes very often?
Phase contrast microscopy is a microscopy technique that is used to visualize small or transparent specimens that might be difficult to see with a regular light microscope. While phase contrast microscopy can be used to study a wide range of samples, including cells, tissues, microorganisms, and other small specimens, it is not typically used to view prokaryotes (bacteria and archaea) very often.
There are several reasons why phase contrast microscopy is not commonly used to view prokaryotes. One reason is that prokaryotes are relatively small and have a relatively simple structure, so they are often easier to see with other microscopy techniques, such as brightfield microscopy or darkfield microscopy.
Another reason is that prokaryotes are often difficult to stain or label, so it can be difficult to create contrast between different parts of the sample using phase contrast microscopy. Prokaryotes do not have a cell nucleus or other membrane-bound organelles, so they do not have the same type of internal structure as eukaryotic cells, which makes it difficult to create contrast between the different parts of the sample.
Finally, prokaryotes are often difficult to culture and maintain in the laboratory, which can make it challenging to prepare samples for phase contrast microscopy. Prokaryotes are sensitive to changes in temperature, pH, and other environmental factors, and they may not survive the preparation process for phase contrast microscopy.
Overall, while phase contrast microscopy can be used to study a wide range of samples, it is not commonly used to view prokaryotes due to the small size and simple structure of these organisms, the difficulty of staining or labeling them, and the challenges of preparing samples for microscopy.
- https://ibidi.com/content/213-phase-contrast
- https://www.microscopemaster.com/phase-contrast-microscope.html
- https://www.biologydiscussion.com/botany/practicals-botany/parts-and-working-of-phase-contrast-microscope-botany/54531
- https://www.microscopyu.com/techniques/phase-contrast/introduction-to-phase-contrast-microscopy
- https://en.wikipedia.org/wiki/Phase-contrast_microscopy
- https://www.slideshare.net/manjunathasanka/phase-contrast-microscope-41441936
- https://www.ruf.rice.edu/~bioslabs/methods/microscopy/phase.html
Cite this post:
BNO Team. (2025, January 24). Phase Contrast Microscopy- Principle, Parts, Uses. Biology Notes Online. Retrieved from https://biologynotesonline.com/phase-contrast-microscopy-principle-parts-uses/
BNO Team. "Phase Contrast Microscopy- Principle, Parts, Uses." Biology Notes Online, 24 January 2025, biologynotesonline.com/phase-contrast-microscopy-principle-parts-uses/.
BNO Team. "Phase Contrast Microscopy- Principle, Parts, Uses." Biology Notes Online (blog). January 24, 2025. https://biologynotesonline.com/phase-contrast-microscopy-principle-parts-uses/.