What is Microbodies?
- The cells of Protozoa, fungi, plants, as well as the liver and kidney of vertebrates, include membrane-bound spherical structures of 0.2 to 1.5 m in diameter that are closely associated with endoplasmic reticulum, mitochondria, chloroplast, or both.
- These organelles, known as microbodies, have a central granular or crystalloid core containing enzymes.
- Similar to mitochondria, microbodies utilise molecular oxygen, but instead of cytochromes and the ability to synthesise ATP, they contain flavin-linked oxides and catalases for the hydrogen peroxide metabolism as well as enzymes for the fatty acid metabolism.
- Peroxisomes are distinct in numerous ways from mitochondria and chloroplasts. Notably, these organelles are only covered by a single membrane, and they lack DNA (genome) and ribosomes.
- However, they resemble ER in that they are membrane-bound organelles that replicate themselves.
Historical
- Since the mid-1950s, electron microscopists have seen minute structures or entities within cells that have been accurately dubbed microbodies on the basis of their morphology.
- C.de Duve and P. Baudhuin (1966) developed the term peroxisome for mammalian microbodies and examined their structure and function.
- Tolbert’s group in Michigan was the first to extract leaf peroxisomes from spinach leaf homogenate (i.e., from mesophyll cells) in 1968.
- Beevers found glyoxysomes, which contain the glyoxylate cycle, in the endoplasm cells of germinating castor bean in 1969. (Ricinus).
Microbodies: Structure And Types
- The shape of microbodies is spherical or oblate. They are surrounded by a single membrane and contain an amorphous or granular interior or matrix.
- The presence of catalase enzyme distinguishes microbodies from other cell organelles the most clearly. Catalase is visible under an electron microscope when cells are stained with DAB (i.e., 3, 3′-diaminobenzidine).
- The result is electron opaque and shows as dark areas in cells containing catalase.
- Microbodies have been detected by electron microscopy and isolated from several mammalian organs, including liver, kidney, gut, and brain, using this method.
- The following steps are involved in isolating microbodies from animal and plant tissues:
- To prevent disturbance of microbodies, tissues are meticulously crushed.
- The homogenate is subjected to differential centrifugation to yield a microbody-rich fraction of the cell homogenate.
- On a discontinuous or continuous sucrose density gradient, the enhanced fraction is subjected to isopycnic ultracentrifugation.
- Recent biochemical studies have separated peroxisomes and glyoxysomes as two distinct types of microbodies. These two organelles differ in their complement of enzymes and the type of tissue in which they are present.
- Animal cells and the leaves of higher plants have peroxisomes. There are catalases and oxidases present (e.g., D-amino oxidase and urate oxidase).
- In both instances, they contribute to the oxidation of substrates, generating hydrogen peroxide that is afterwards neutralised by catalase activity:
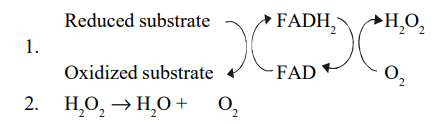
- Peroxisomes remain connected with the ER, chloroplasts, and mitochondria in plant cells and participate in photorespiration.
- Glyoxysomes are exclusive to plant cells and are especially plentiful in developing seeds that store fats as a food reserve.
- They also contain glyoxylate cycle enzymes in addition to catalases and oxidases. Glyoxysomes continue to be closely connected with lipid bodies, spherosomes, and include enzymes for fatty acid metabolism and gluconeogenesis (i.e., formation of glucose from various non-carbohydrate precursors as succinate in this case).
- In addition to peroxisomes and glyoxysomes, cytosomes, phragmosomes, and crystal-containing bodies are all words that have been used to characterise microbodies.
Peroxisomes
- Peroxisomes are solitary membrane-bound organelles that are found in eukaryotic cells.
- They contain digestive enzymes for degrading harmful substances within the cell, as well as oxidative enzymes for metabolic activity.
- They are a diverse category of organelles, differentiated from other cell organelles by the presence of marker enzymes.
- Peroxisomes serve a crucial function in lipid synthesis and are also involved in catalase’s conversion of reactive oxygen species such as hydrogen peroxide into less dangerous molecules such as water and oxygen.
- Typically, peroxisomes exist as separate organelles, such as in fibroblasts. In liver cells, peroxisomes appear as linked tubules known as peroxisome reticulum.
- Peroxisomes are present in numerous animal and plant cells.
- In addition to Euglenophyta, Protozoa, brown algae, fungi, liverworts, mosses, and ferns, they are found in all photosynthetic cells of higher plants, including etiolated leaf tissue, coleoptiles, and hypocotyls, tobacco stem and callus, and ripening pear fruits.
Structure of Peroxisomes
- Variable in size and shape, peroxisomes typically have a circular cross-section with a diameter between 0.2 and 1.5 m (0.15 to 0.25 m in most mammalian tissues; 0.5 m in rat liver cells).
- Their granular matrix is enclosed by a single limiting unit membrane comprising lipid and protein molecules.
- In some instances (e.g., festuciod grasses), the matrix is composed of many threads or fibrils, but in others, an amorphous nucleoid or a dense inner core with a crystalloid structure is detected (e.g., tobacco leaf cell).
- Little is known about the core’s function, except that it is the location of the enzyme urate oxidase in rat liver peroxisomes and the majority of the catalase in some plants.
Functions of Peroxisomes
Peroxisomes have been found to engage in two types of biochemical processes:
1. Hydrogen peroxide metabolism
- Peroxisomes are typically composed of one or more enzymes (D-amino acid oxidase and urate oxidase) that use molecular oxygen to extract hydrogen atoms from certain organic substrates (R) in an oxidative process that generates hydrogen peroxide (H2O2): RH2+O2 → R + H2O2
- Catalase (40% of total peroxisome protein) exploits the H2O2 produced by other enzymes in the organelle to oxidise a range of different compounds, including alcohols, phenols, formic acid, and formaldehyde, via the “peroxidative” reaction: H2O2 + R′ H2 → R′ + 2H2O
- This type of oxidative response is particularly significant in liver and kidney cells, whose peroxisomes are responsible for detoxifying numerous blood-borne toxins. In this manner, over half of the alcohol one consumes is converted to acetaldehyde. Catalase transforms H2O2 to H2O when excessive H2O2 accumulates within the cell: 2H2O2 → 2H2O + O2
H2O2and aging
- Mitochondria and ER membranes produce the majority of cytosolic H2O2, although there are additional H2O2-producing enzymes in the cytoplasmic matrix.
- Other enzymes, such as glutathione peroxidase, are capable of metabolising both organic hydroperoxides and H2O2 in the cytosol (cytoplasmic matrix) and mitochondria. Catalase functions as a “safety valve” for coping with the huge volumes of H2O2 produced by peroxisomes.
- The enzyme superoxide dismutase is primarily responsible for regulating the formation of superoxide anion (O2 -) in mitochondria and cytosol (cytoplasmic matrix). In aerobic tissues, all of these defensive enzymes are present in high concentrations.
- Recent research has suggested a link between peroxides and free radicals (such as superoxide anion—O2 -) and the ageing process.
- On the DNA molecule, these radicals may induce mutations that affect transcription into mRNA and translation into proteins.
- Additionally, free radicals and peroxides can disrupt membranes by inducing lipid and protein peroxidation.
- Consequently, reducing substances such as vitamin E or enzymes such as superoxide dismutase may play a role in maintaining a cell’s health.
2. Glycolate cycle
- Peroxisomes of plant leaves contain catalaze along with glycolate pathway enzymes, including glycolate oxidase, glutamate glyoxylate, serine-glyoxylate and aspartate-ketoglutarate aminotransferases, hydroxy pyruvate reductase and malic dehydrogenase.
- They also contain the coenzymes FAD, NAD, and NADP. In a series of reactions involving chloroplasts, peroxisomes, mitochondria, and cytosol, the glycolate cycle is believed to produce the amino acids glycine and serine from the non-phosphorylated intermediates of the photosynthetic carbon reduction cycle, i.e. glycerate to serine or glycolate to glycine and serine.
- In addition to producing C1 chemicals, the glycolate pathway acts as the source of precursors for nucleic acid biosynthesis.
Photorespiration
- In green leaves, peroxisomes carry out a process known as photorespiration, which is a light-stimulated CO2 production distinct from the CO2 production by mitochondria in the dark.
- In photorespiration, the two-carbon photosynthesis product glycolic acid (glycolate) is released from chloroplasts and oxidised into glyoxylate and H2O2 by the peroxisomal enzyme glycolic acid oxidase. Eventually, glyoxylate is converted to CO2 and formate:
CH2OH. COOH + O2 → CHO – COOH + H2O2
CHO — COOH + H2O2 → HCOOH + CO2 + H2O
- Photorespiration derives its name from the fact that light stimulates glycolic acid production in chloroplasts. Two fundamental organelles are involved in the entire process: chloroplasts and peroxisomes.
- Lastly, photorespiration is driven by atmospheric circumstances characterised by a high O2 tension and a low CO2 tension.
- O2 appears to compete with CO2 for ribulose diphosphate carboxylase, the primary enzyme in CO2 fixation during photosynthesis.
- Enzyme use of oxygen results in the formation of an unstable intermediate that degrades into 3-phosphoglycerate and phosphoglycolate.
- By removing its phosphate group, the latter tends to raise the glycolate concentration, making more glycolate available for further oxidation and CO2 release.
- Photorespiration is a wasteful process for the plant cell since it affects the efficiency of photosynthesis greatly (i.e., it returns a portion of fixed CO2 to the atmosphere).
- C3 plants are particularly susceptible to low CO2 concentrations, whereas C4 plants are significantly more efficient in this regard.
C. β-oxidation
- Peroxisomes of rat liver cells contain β-oxidation enzymes for fatty acid metabolism. Using molecular oxygen and NAD as electron acceptors, they are capable of oxidising palmitoyl-CoA (or fatty acyl-CoA) to acetyl-CoA.
- This process generates acetyl-CoA, which is eventually delivered to the mitochondria, where it joins the citric acid cycle. If, on the other hand, acetyl-CoA remains in the cytosol, it is transformed back into fatty acids and then neutral fats.
- Except for one crucial exception, the -oxidation process of peroxisomes is extremely similar to the one that happens in mitochondria.
- The flavin dehydrogenase in mitochondria sends its electrons to the respiratory chain. It does not react with oxygen molecules.
- In peroxisomes, the dehydrogenase immediately interacts with O2 and produces H2O2. Mitochondria lack catalase and are consequently incapable of preventing the generation of harmful hydrogen peroxide. This is not a concern for peroxisomes.
D. Other functions
- Mammalian cells do not contain D-amino acids; nonetheless, the liver and kidney peroxisomes possess D-amino acid oxidase.
- It is believed that this enzyme is intended for D-amino acids present in the bacterial cell wall.
- Consequently, the assumed function of this enzyme is to initiate the degradation of D-amino acid, which may result from the breakdown and absorption of peptidoglycan by intestinal bacteria.
- Uric acid oxidase (uricase) is essential for the degradation of purines throughout the catabolic route. Thus, peroxisomes are extraordinarily varied organelles, and different cells of the same organism may contain vastly different enzyme complements.
- They are also exceptionally adaptable to shifting environments. Yeast cells fed on sugar, for instance, contain minute peroxisomes.
- However, when certain yeasts are grown on methanol, they produce big peroxisomes that oxidise methanol; when grown on fatty acids, they produce enormous peroxisomes that convert fatty acids to acetyl-CoA.
Biogenesis of Peroxisomes
- At one time, it was believed that the membrane “shell” of peroxisomes is generated by endoplasmic reticulum (ER) budding, while the “content” or matrix is imported from the cytosol (cytoplasmic matrix).
- However, there is now evidence to imply that new peroxisomes invariably develop from pre-existing ones, through the growth and fission of ancient organelles comparable to mitochondria and chloroplasts.
- Peroxisomes are therefore a cluster of organelles with a constant membrane and changing enzymatic content.
- All of its structural and functional proteins are encoded by nuclear genes and produced in the cytosol (cytoplasmic matrix) (i.e., on the free ribosomes).
- The proteins present in the peroxisome lumen or membrane are acquired post-translationally from the cytosol (cytoplasmic matrix).
- The haeme-free monomers are imported into the lumen of peroxisomes, where they assemble into tetramers in the presence of haeme.
- Catalase and several peroxisomal proteins contain a signal sequence (consisting of three amino acids) at their carboxyl termini that sends them to the peroxisome.
- On the cytosolic surface of peroxisomes are receptors that identify the signal on imported proteins.
- All peroxisome membrane proteins, including signal receptor proteins, are directly imported from the cytosol (cytoplasmic matrix).
- The lipids required for the production of new peroxisomal membrane are also imported from the cytosol (cytoplasmic matrix), potentially via phospholipid transfer proteins from their sites of manufacture in the ER membranes.
Symbiotic origin
- According to one theory, peroxisomes are a remnant of an ancient organelle that was responsible for the entire oxygen metabolism in the early ancestors of eukaryotic cells.
- When oxygen created by photosynthetic bacteria first began to build in the earth’s primordial atmosphere, it would have been extremely hazardous to the majority of cell forms.
- Peroxisomes may have served to decrease the oxygen concentration in these cells while also utilising its chemical reactivity to carry out beneficial oxidative processes.
- This concept also posits that the later evolution of mitochondria rendered the peroxisome essentially useless, as many of the same activities that were previously carried out in peroxisomes without producing energy were now related to ATP generation via oxidative phosphorylation.
Glyoxysomes
- Glyoxysomes are found in yeast, Neurospora, and the oil-rich seeds of numerous higher plants.
- In morphological characteristics, they resemble peroxisomes, except that their crystalloid core consists of dense rods with a diameter of 6.0 m.
- They contain enzymes for fatty acid metabolism and gluconeogenesis, which is the conversion of stored lipid molecules of germinating seed spherosomes into molecules of carbohydrates.
Functions of Glyoxysomes
Glyoxysomes execute the subsequent biochemical functions in plant cells:
1. Fatty acid metabolism
- During germination of oily seeds, lipase (glycerol ester hydrolase) converts the stored lipid molecules of spherosomes into glycerol and fatty acids.
- The phospholipase enzyme hydrolyzes the phospholipid molecules.
- In the -oxidation process, the long chain fatty acids liberated by hydrolysis are broken down by the sequential elimination of two carbon or C2 pieces.
β-Oxidation
- During the β-oxidation process, the fatty acid is activated by fatty acid thiokinase into fatty acyl-CoA, which is then oxidised by FAD-linked fatty acyl-CoA dehydrogenase into trans-2-enoyl-CoA.
- Enzyme enoyl hydratase or crotonase hydrates trans-2-enoyl-CoA to generate L-3-hydroxyacyl-CoA, which is then oxidised by a NAD-linked L-3-hydroxyacyl-CoA dehydrogenase to provide 3-ketoacyl-CoA.
- Under the influence of thiolase or β-keto thiolase, the 3-keto acyl-CoA loses a two-carbon fragment, generating acetyl-CoA and a new fatty acyl-CoA with two less carbon atoms than the original.
- This new fatty acyl-CoA is then recycled via the same set of chemical events until the last two molecules of acetyl-CoA are formed. The entire -oxidation pathway can be depicted as follows:
- In plant seeds, glyoxysomes are responsible for β-oxidation. In contrast, β-oxidation occurs in glyoxysomes and mitochondria in other plant cells.
- For the oxidation of reduced flavorprotein formed as a result of fatty-acyl-CoA dehydrogenase activity, glyoxysomal β-oxidation requires oxygen. In mitochondria, β-oxidation occurs in animal cells.
- In plant cells, acetyl-CoA, the result of the β-oxidation chain, is not oxidised by the Krebs cycle because it remains spatially separated from the Krebs cycle enzymes. Instead, acetyl-CoA is transformed into succinate via the glyoxylate cycle.
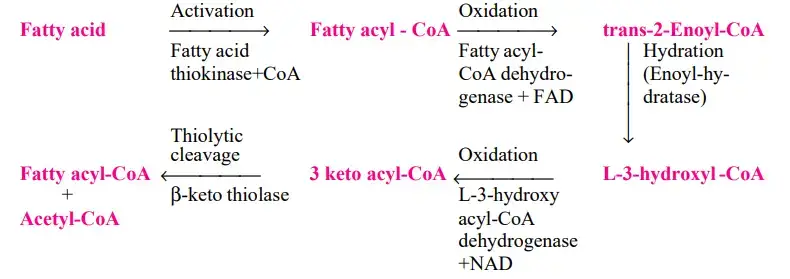
2. Glyoxylate cycle
- Citrate is produced from oxaloacetate and acetyl-CoA by citrate synthase in the glyoxylate pathway.
- The aconitase enzyme then converts the citrate into isocitrate.
- Isocitrate is then converted enzymatically to glyoxylate and succinate by the isocitratase enzyme.

- Malate synthase converts glyoxylate and another molecule of acetyl-CoA into a molecule of malate:

- To complete the cycle, malate dehydrogenase converts this malate to oxaloacetate. In conclusion, the glyoxylate route involves: 2 Acetyl-CoA + NAD+ → Succintiate + NADH + H+
- Succinate is the final result of fatty acid metabolism within the glyoxysome and is not further processed by this organelle. The synthesis of hexose or gluconeogenesis requires the conversion of succinate to oxaloacetate, which occurs in the mitochondria since glyoxysomes lack fumarase and succinic dehydrogenase.
- Without carbon loss, two molecules of oxaloacetate are produced from four molecules of acetyl-CoA. In the phosphoenol pyruvate carboxykinase reaction, this oxaloacetate is transformed to phosphoenol pyruvate with the loss of two molecules of CO2: 2 Oxaloacetate + 2ATP = 2 Phosphoenol pyruvate + 2CO2 + 2ADP
- The following reactions convert phosphoenol pyruvate into monosaccharides (such as glucose and fructose), disaccharides (sucrose), and polysaccharides (starch):
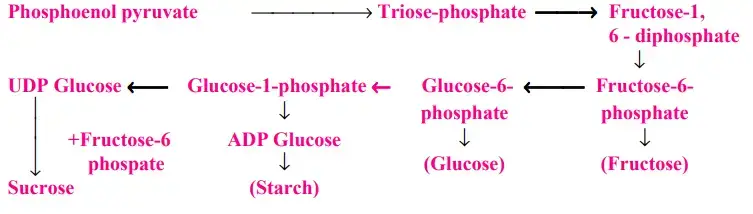
FAQ
What are peroxisomes?
Peroxisomes are membrane-bound organelles found in most eukaryotic cells. They are responsible for various metabolic functions, including the breakdown of fatty acids and the detoxification of harmful substances.
What is the structure of peroxisomes?
Peroxisomes have a single membrane and contain a matrix that contains enzymes for various metabolic reactions.
How do peroxisomes function in lipid metabolism?
Peroxisomes are responsible for the beta-oxidation of fatty acids, which generates acetyl-CoA and hydrogen peroxide. They also play a role in the synthesis of bile acids, cholesterol, and plasmalogens.
What is the role of peroxisomes in detoxification?
Peroxisomes contain enzymes that help to break down harmful substances, including alcohol and other toxins.
What are glyoxysomes?
Glyoxysomes are specialized peroxisomes found in the cells of plants and fungi. They are involved in the conversion of stored fats into carbohydrates for energy production.
What is the structure of glyoxysomes?
Glyoxysomes are similar in structure to peroxisomes, with a single membrane and a matrix containing enzymes for lipid metabolism.
How do glyoxysomes function in plant metabolism?
Glyoxysomes are responsible for the conversion of stored fats in germinating seeds into carbohydrates for energy production.
What is the importance of glyoxysomes in plant growth?
Glyoxysomes play a critical role in the growth of plants by providing the necessary energy for seedling development and growth.
What is the difference between peroxisomes and glyoxysomes?
While both organelles are involved in lipid metabolism, peroxisomes are found in most eukaryotic cells and are involved in various metabolic functions, including detoxification. Glyoxysomes are only found in plants and fungi and are specifically involved in the conversion of stored fats into carbohydrates for energy production.
What happens if peroxisomes or glyoxysomes are dysfunctional?
Dysfunctional peroxisomes can lead to various metabolic disorders, including Zellweger syndrome and other peroxisomal disorders. Dysfunctional glyoxysomes can lead to impaired plant growth and development.