What is Membrane Permeability?
- Membrane permeability refers to the rate at which molecules can passively diffuse across a biological membrane. This concept is crucial in understanding how substances move in and out of cells. The permeability of a membrane dictates the ease with which various molecules can traverse the membrane barrier, influencing cellular function and homeostasis.
- The cell membrane, composed primarily of a lipid bilayer, exhibits selective permeability. This characteristic means that it allows only specific molecules to enter or exit the cell while restricting others. For instance, small, nonpolar molecules, such as oxygen and carbon dioxide, can readily diffuse through the lipid bilayer due to their size and hydrophobic nature. In contrast, larger or charged molecules, such as glucose and ions, require specialized transport mechanisms to cross the membrane.
- The selective nature of the cell membrane is vital for maintaining cellular integrity and function. By regulating the entry and exit of substances, the membrane ensures that essential nutrients, ions, and water are available while preventing harmful substances from infiltrating the cell. This regulation is key for processes such as nutrient uptake, waste removal, and signal transduction.
- Additionally, membrane permeability is influenced by various factors, including temperature, the concentration gradient of the molecules, and the chemical properties of the membrane itself. For example, an increase in temperature typically enhances the kinetic energy of molecules, which can increase the rate of diffusion across the membrane. Conversely, changes in the composition of the membrane, such as the presence of cholesterol, can alter its fluidity and, subsequently, its permeability.
- Moreover, specific transport proteins embedded in the cell membrane play a crucial role in facilitating the movement of particular molecules. These proteins can function as channels or carriers, enabling the passive or active transport of substances. For instance, ion channels allow the selective passage of ions, while transporters may utilize energy to move molecules against their concentration gradient.
Cell Membrane Structure
The cell membrane, often referred to as the plasma membrane or biological membrane, plays a crucial role in maintaining the integrity of the cell by separating its interior from the external environment. Its structure is vital for selective permeability and fluidity, allowing the cell to regulate its internal conditions effectively. This membrane is primarily composed of lipids, proteins, carbohydrates, and cholesterol.
- Lipids
- The cell membrane consists of three primary classes of lipids: phospholipids, glycolipids, and sterols.
- Phospholipids are the fundamental building blocks of the membrane, composed of glycerol or sphingosine, fatty acids, phosphate, and alcohol. They form a bilayer due to their amphipathic nature, where the hydrophilic (water-attracting) heads face outward towards the aqueous environment, while the hydrophobic (water-repelling) tails are oriented inward, away from water.
- Glycolipids, which are formed by the covalent attachment of carbohydrates to lipids, contribute to the membrane’s structural integrity and play a role in cell recognition.
- Sterols, particularly cholesterol, are multi-hydroxy alcohols with complex ring structures that intercalate within the phospholipid bilayer. Cholesterol is essential for maintaining membrane fluidity and stability, preventing the fatty acids from packing too closely together.
- The cell membrane consists of three primary classes of lipids: phospholipids, glycolipids, and sterols.
- Proteins
- Proteins are integral components of the cell membrane, serving various functions essential for cellular operation.
- Intrinsic membrane proteins are embedded either partially or completely within the lipid bilayer. These proteins often function as channels or transporters, facilitating the movement of molecules across the membrane.
- Extrinsic membrane proteins are loosely associated with the membrane surface, playing roles in signaling and cell recognition. Their positioning allows them to interact with both the membrane and extracellular environment, contributing to various cellular processes.
- Proteins are integral components of the cell membrane, serving various functions essential for cellular operation.
- Carbohydrates
- Carbohydrates are located on the surface of the cell membrane, forming glycoproteins (carbohydrates attached to proteins) and glycolipids (carbohydrates attached to lipids).
- These carbohydrate chains can consist of 2 to 60 monosaccharide units, which may be linear or branched. They serve crucial functions, including protecting the cell from damage and mediating cell-cell adhesion.
- By forming a protective coating, carbohydrates enhance cellular communication and recognition, which is essential for tissue formation and immune responses.
- Carbohydrates are located on the surface of the cell membrane, forming glycoproteins (carbohydrates attached to proteins) and glycolipids (carbohydrates attached to lipids).
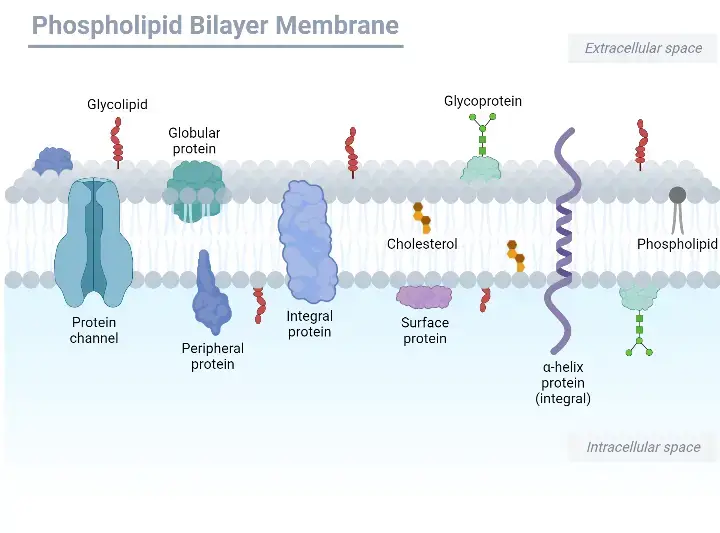
Factors Affecting Cell Membrane Permeability
Cell membrane permeability is a critical aspect of cellular function, influencing the movement of substances into and out of the cell. Several factors affect this permeability, including membrane composition, temperature, pH, and the characteristics of the molecules attempting to cross the membrane.
- Cell Membrane Composition
- Fatty Acid Composition: The types of fatty acids present in the membrane significantly influence its permeability. Membranes rich in unsaturated fatty acids, which have kinks in their structure, tend to be more permeable. This is due to the reduced Van der Waals interactions between the fatty acid chains, allowing for easier movement of substances. Conversely, saturated fatty acids, which pack tightly together, hinder the passage of molecules, thereby decreasing permeability.
- Cholesterol Levels: The concentration of cholesterol within the membrane also plays a pivotal role in permeability. High levels of cholesterol generally reduce membrane permeability in eukaryotic cells by stabilizing the membrane structure. Cholesterol limits the movement of phospholipids and other membrane components, which can lead to decreased fluidity. Temperature can modify this relationship; at lower temperatures, cholesterol can enhance fluidity, while at higher temperatures, it may restrict movement.
- Temperature
- The temperature of the environment can significantly alter membrane permeability. At low temperatures, around freezing (0 degrees Celsius), phospholipids become rigid and closely packed, resulting in decreased permeability. As temperatures rise, up to approximately 45 degrees Celsius, the membrane becomes more fluid as the phospholipid bilayer loosens, facilitating greater permeability. However, if temperatures exceed this range, membrane proteins may denature, causing structural deformation and a loss of selective permeability.
- pH Levels
- The pH of the surrounding environment is crucial for maintaining membrane integrity. The normal intracellular pH is around 7. Deviations of even one unit, such as lowering to 6 or raising to 8, can disrupt membrane structure, leading to reduced permeability and compromised function. This sensitivity to pH changes underscores the importance of homeostasis in cellular environments.
- Molecular Characteristics
- The properties of molecules attempting to cross the membrane, including their polarity, electric charge, and molecular mass, greatly affect diffusion rates. Small, nonpolar molecules like carbon dioxide (CO2), nitrogen (N2), and oxygen (O2) can diffuse rapidly through the lipid bilayer without the need for transport proteins. In contrast, charged molecules or larger substances exhibit slower diffusion rates, as their size and charge hinder their ability to traverse the hydrophobic core of the membrane.
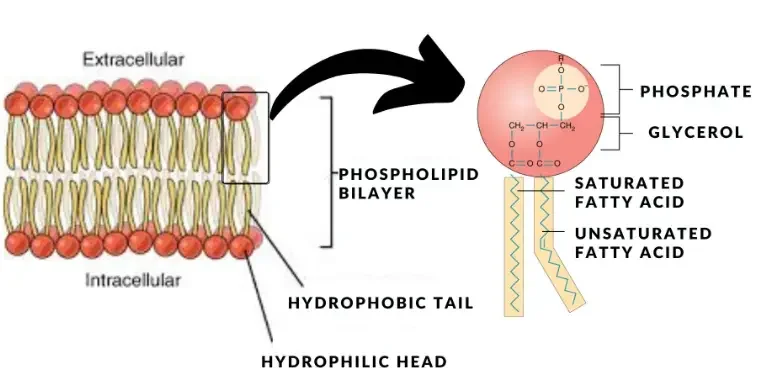
Approaches to Artificially Improve Membrane Permeability
Enhancing the permeability of cell membranes is crucial for optimizing drug delivery systems and improving therapeutic outcomes. As scientists explore innovative methodologies, various strategies have been developed to facilitate the transport of different types of cargo across the membrane. These approaches aim to increase bioavailability, allowing drugs and therapeutic agents to reach their target locations more effectively.
- Small Molecule Cargo
- Membrane permeation is vital for the effective delivery and distribution of drugs within an organism. By improving permeability, scientists can enhance the bioavailability of drug molecules, ensuring that a greater concentration is available for biological action.
- One strategy involves conjugating compounds to known transporter substrates, which can facilitate improved permeation and release of the drug molecule within the membrane.
- Peptide Cargo
- Peptides typically do not cross cell membranes passively due to their size and polarity. Therefore, researchers focus on modifying the physical properties of peptides to enhance their permeability.
- For instance, cyclization of peptides, along with methylation of the nitrogen in the amide bond, has shown promise in improving their ability to traverse membranes.
- Additionally, altering the α-helical content and incorporating arginine residues within α-helices can further increase peptide permeability.
- Protein Cargo
- The substantial size and polarity of protein molecules pose significant challenges for passive membrane crossing. Due to the diverse physicochemical properties of various proteins, there is no one-size-fits-all approach for enhancing their permeability. Several strategies have been proposed:
- Mechanical Disruption: Techniques such as microinjection, electroporation, microfluidic devices, and nanowires can physically disrupt the cell membrane, facilitating protein delivery.
- Peptide-Based Strategies: Cell-penetrating peptides, typically consisting of 30 or fewer amino acids, can traverse membranes through energy-independent pathways. Examples include the TAT peptide and the amphiphilic CPP Pep-1 peptide, both capable of transporting various molecules across the plasma membrane.
- Protein-Based Strategies: This method utilizes pore- or channel-forming proteins derived from bacteria. Notable examples include engineered bacterial channels like MscL and the cholesterol-dependent cytolysin family of pore-forming toxins. Human proteins with high net positive charges, such as supercharged Green Fluorescent Proteins (GFP), have also been identified to facilitate protein translocation.
- Virus-Based Strategies: Packaging proteins within virus-like particles and attaching them to engineered bacteriophage T4 heads can enhance the delivery of proteins to the cytoplasm, leveraging the natural uptake mechanisms of viruses.
- Lipid- and Polymer-Based Strategies: These methods involve encapsulating protein cargo within liposomes or forming complexes with proteins. A combination of cationic and neutral lipids has been shown to translocate negatively charged proteins across the plasma membrane effectively. Furthermore, polymer-based compounds like polyethyleneimine (PEI) exploit the proton sponge effect, which buffers protons, causing osmotic swelling in endosomes, thereby facilitating the release of protein molecules. Nanocapsules that degrade in response to specific protease activity or changes in pH and redox potential have also been investigated for delivering proteins and transcription factors.
- Inorganic Material-Based Strategies: Various inorganic materials, including silica, carbon nanotubes, quantum dots, and gold nanoparticles, have been utilized to assist in the translocation of protein cargo across membranes.
- The substantial size and polarity of protein molecules pose significant challenges for passive membrane crossing. Due to the diverse physicochemical properties of various proteins, there is no one-size-fits-all approach for enhancing their permeability. Several strategies have been proposed:
What is Selectively Permeable Membrane?
A selectively permeable membrane, often referred to as a semipermeable or differentially permeable membrane, is a fundamental biological structure that regulates the movement of substances into and out of a cell. This characteristic is crucial for maintaining the cell’s internal environment, or homeostasis, allowing only certain molecules to pass through while restricting others. The cell membrane serves as a barrier that not only encloses the cellular contents but also interacts dynamically with the external environment.
At the core of a selectively permeable membrane’s function is its ability to allow the passage of specific substances via various transport mechanisms. Two primary types of transport mechanisms are active transport and passive transport, each serving distinct functions in cellular processes.
Active transport involves the movement of ions or molecules against their concentration gradient, which necessitates an expenditure of energy, typically derived from the hydrolysis of adenosine triphosphate (ATP). A prime example of this is the Na+/K+ ATPase enzyme, which actively transports sodium ions out of the cell while bringing potassium ions in, thus maintaining the necessary ion concentration gradients essential for cellular function.
In contrast, passive transport facilitates the movement of substances without the use of cellular energy. This process occurs through mechanisms such as free diffusion and facilitated diffusion. Free diffusion pertains to the movement of small, uncharged molecules—like ethanol and carbon dioxide—directly across the lipid bilayer of the membrane. This movement occurs down the concentration gradient until equilibrium is reached.
Facilitated diffusion, on the other hand, requires specific proteins embedded in the membrane that act as carriers. These proteins help transport larger or polar molecules, such as glucose or ions, across the membrane more efficiently than simple diffusion would allow. For instance, the transport of oxygen into red blood cells is facilitated by hemoglobin, which binds to oxygen and allows for its movement across the membrane.
Thus, the selective permeability of membranes is critical for cellular function. By regulating the entry and exit of various substances, selectively permeable membranes play a pivotal role in maintaining homeostasis within the cell. This functionality underscores the importance of understanding membrane dynamics, as they are foundational to many physiological processes and cellular activities. Therefore, the study of selectively permeable membranes not only enhances comprehension of basic biological principles but also provides insight into complex cellular interactions and mechanisms.
Examples of Selectively-Permeable Membranes
Below are key examples of selectively-permeable membranes and their functions within biological systems.
- Mitochondria: Often referred to as the powerhouse of the cell, mitochondria are essential for cellular respiration, a process that generates adenosine triphosphate (ATP) through biochemical reactions. The selectively-permeable membrane of the mitochondrion allows specific proteins and ions to enter, facilitating metabolic processes while preserving the delicate internal chemical balance required for optimal function. This regulation is crucial for efficient energy production and overall cellular metabolism.
- Neurons: Neurons communicate through electrochemical signals that require precise ion exchange across their membranes. When a neuron is stimulated, ions such as sodium and potassium selectively pass through the membrane, creating an action potential that propagates the signal. After transmitting the signal, the neuron must return to its resting potential, a process again regulated by its selectively-permeable membrane. This cycle of excitation and recovery is vital for proper nerve function and communication within the nervous system.
- Cardiac Muscles: The heart muscle relies on the rhythmic contraction and relaxation of cardiac cells to maintain a steady heartbeat. Selectively-permeable membranes in cardiac muscle cells regulate the flow of ions, ensuring that the electrical signals for contraction are timely and coordinated. This regulation is essential for sustaining a consistent heartbeat and overall cardiovascular health, illustrating the importance of selective permeability in muscle function.
Structure of Selectively-Permeable Membrane
The selectively-permeable membrane, primarily known as the cell membrane, plays a crucial role in maintaining cellular integrity and regulating the movement of substances. Its complex structure has been the subject of extensive study, culminating in models that illustrate its dynamic nature. Below are key components that characterize the structure of selectively-permeable membranes, particularly the fluid mosaic model, which provides a comprehensive understanding of their organization and function.
- Historical Context: The existence of the selectively-permeable membrane was hypothesized in the late 19th century, with its chemical composition becoming clearer in 1915. Initial models, like the one proposed by James Danielli and Hugh Davson in 1935, likened the membrane’s structure to a “sandwich,” with proteins serving as the “bread” and lipids as the “filling.” This analogy helped establish a foundation for understanding membrane structure.
- Fluid Mosaic Model: In the 1970s, S.J. Singer and Garth L. Nicolson refined the model into what is now widely recognized as the fluid mosaic model. This model posits that the membrane comprises a mosaic of various components, including proteins, phospholipids, carbohydrates, and cholesterol. It emphasizes the fluidity of the membrane, allowing for movement and flexibility while maintaining selective permeability.
- Lipid Bilayer: The membrane is primarily composed of phospholipids, which are characterized by their amphipathic nature—having hydrophilic heads that face outward toward the aqueous environment and hydrophobic tails that orient inward, away from water. This arrangement creates a bilayer structure, essential for forming a barrier that separates the interior of the cell from the external environment.
- Proteins: Integral and peripheral proteins are essential structural components of the selectively-permeable membrane.
- Intrinsic Proteins: These proteins are embedded within the lipid bilayer and are typically involved in metabolic processes. They can function as channels, carriers, or receptors, contributing to the membrane’s selective permeability.
- Extrinsic Proteins: These proteins are loosely attached to the surface of the membrane, interacting with intrinsic proteins or the lipid bilayer. They play roles in signaling and maintaining the membrane’s structural integrity.
- Carbohydrates: Carbohydrate molecules are found on the extracellular surface of the membrane, either attached to proteins (forming glycoproteins) or to lipids (forming glycolipids). These carbohydrates are crucial for cell recognition and communication, providing identity markers for the cell.
- Cholesterol: Interspersed within the phospholipid bilayer, cholesterol molecules help stabilize the membrane’s structure, influencing its fluidity. This component is particularly important in maintaining the integrity of the membrane under varying temperature conditions.
- Variability in Composition: The specific proportions of proteins, lipids, and carbohydrates within the selectively-permeable membrane can vary significantly depending on the type of cell. For instance, human cells typically contain about 50% proteins, 40% lipids, and 10% carbohydrates. Conversely, myelin, which insulates axons, consists of 76% lipids and only 18% proteins, demonstrating the diversity in membrane composition and function across different cell types.
- Thickness: The thickness of the selectively-permeable membrane ranges from 5 to 10 nanometers. To provide context, the diameter of a human red blood cell is approximately 8 micrometers, highlighting the relative thinness of the membrane compared to the overall cell structure.
Functions of Selectively-Permeable Membrane
Below are the primary functions of the selectively-permeable membrane, elucidating its significance in cellular processes.
- Anchoring the Cytoskeleton: The selectively-permeable membrane provides anchoring points for the cytoskeleton, a network of fibers that maintains cell shape and structural integrity. This connection is vital for attaching the extracellular matrix and neighboring cells, facilitating the formation of tissues.
- Protection and Homeostasis: One of the primary functions of the selectively-permeable membrane is to protect the cell from harmful substances. By regulating the transport of materials, it maintains homeostasis within the cell. Toxic substances are expelled, while essential nutrients are imported, ensuring the internal environment remains balanced and conducive to cellular function.
- Maintaining Intracellular Integrity: The membrane’s selective permeability allows it to uphold the distinct internal environment of the cell compared to the external surroundings. This separation is crucial for preserving the conditions necessary for various biochemical processes.
- Barrier Formation: Selectively-permeable membranes serve as barriers between cellular organelles and the external environment. This segregation is vital for the proper functioning of organelles, allowing them to operate under optimal conditions without interference from external factors.
- Transport Across the Nuclear Membrane: In eukaryotic cells, the selectively-permeable membrane plays a crucial role in the transport of molecules across the nuclear membrane. During transcription, the membrane selectively allows specific nucleotides, proteins, and nucleic acids to enter the nucleus while ensuring the timely export of transcription products. This regulation is vital for gene expression and cellular activity.
- Maintaining Nucleocytoplasmic Equilibrium: The selectively-permeable membrane effectively distinguishes the environment of the nucleus from that of the cytoplasm. By maintaining this equilibrium, the membrane supports overall cellular functions, ensuring that various processes occur efficiently and appropriately.
- Facilitating Cell Communication and Signaling: Selectively-permeable membranes play an integral role in cell communication. By allowing specific signaling molecules to bind to receptors on the membrane surface, cells can communicate and coordinate responses to various stimuli, facilitating interaction with neighboring cells and the external environment.
- Membrane Permeability. (2022, December 31). Axolotl Academica Publishing. https://bio.libretexts.org/@go/page/16107
- Pattus, F. (2001). Membrane permeability. Current Opinion in Cell Biology, 13(4), 387–388. doi:10.1016/s0955-0674(00)00225-8
- Membrane Permeability. (2022, November 9). https://phys.libretexts.org/@go/page/1983
- https://byjus.com/question-answer/what-is-permeability-of-cell-membrane/
- https://www.biologyonline.com/dictionary/selectively-permeable-membrane
- https://www.researchgate.net/figure/Figure-A5-Membrane-permeability_fig5_2939077
- https://www.researchgate.net/figure/Permeability-of-biological-membranes-that-allow-or-prevent-the-passage-of_fig4_281119586
- https://www.biologyonline.com/dictionary/permeability
- https://conductscience.com/factors-affecting-cell-membrane-permeability-and-fluidity/
- https://inspiritvr.com/membrane-permeability-study-guide/