Oncologists and immunologists have long been optimistic about the potential of immunologic techniques to treat cancer patients. The primary interest in an immunologic approach stems from the fact that the majority of existing cancer treatments rely on medications that kill dividing cells or stop cell division, and these treatments have severe side effects on normal proliferating cells. As a result, cancer treatments cause substantial morbidity and mortality. Most normal cells will not be harmed by the majority of immune responses to tumour antigens. Immunotherapy has the potential to be the most tumor-specific treatment currently available. Numerous innovative tactics have been inspired by advancements in our knowledge of the immune system and the identification of antigens on tumour cells. Immunotherapy for tumours tries to enhance the host’s weakened immune response to malignancies (active immunity) or to provide tumor-specific antibodies or T cells (passive immunity). In this part, we explain some of the immunotherapy techniques for tumours that have been used in the past or are now under investigation.
Stimulation of Active Host Immune Responses to Tumors
Initial efforts to enhance antitumor immunity relied on nonspecific immunological activation. Vaccines made of dead tumour cells, tumour antigens, or dendritic cells cultured with tumour antigens have been provided to patients more recently, and techniques are being explored to boost immune responses against the tumour.
Vaccination with Tumor Antigens
- Immunization of persons with tumours with tumour antigens may result in increased immune responses against the tumour.
- The identification of peptides recognised by tumor-specific CTLs and the cloning of genes that encode tumor-specific antigens recognised by CTLs have yielded numerous possibilities for tumour vaccines; several examples have been described before in this chapter.
- Immunization with pure tumour antigens and adjuvants, one of the earliest vaccination strategies, is still being evaluated. The use of therapeutic dendritic cell vaccines to immunise cancer patients against their own malignancies is relatively new.
- In this method, patient-derived dendritic cells are either treated with tumour antigens or transfected with genes encoding these antigens before being reinjected into the patient.
- For instance, a vaccination based on cells is currently approved for the treatment of advanced prostate cancer. This vaccine consists of a preparation of a patient’s peripheral blood leukocytes that has been enriched for dendritic cells and then exposed to a recombinant fusion protein composed of granulocyte-macrophage colony-stimulating factor (GMCSF) and the tumor-associated antigen prostatic acid phosphatase.
- In clinical studies, the use of DNA vaccines made of plasmids of viral vectors encoding tumour antigens is an alternate method.
- Because encoded antigens are generated in the cytoplasm and enter the class I MHC antigen presentation pathway, cell-based and DNA vaccines may be the most effective means of inducing CTL responses.
- For antigens that are specific to particular tumours, such as antigens created by random point mutations in cellular genes, these immunisation approaches are impracticable because they require the identification of antigens from each tumour.
- On the other hand, tumour antigens shared by numerous malignancies, such as the MAGE, tyrosinase, and gp100 antigens on melanomas and the mutant Ras and p53 proteins in diverse tumours, are potentially beneficial immunogens for all patients with specific forms of cancer.
- A restriction of treating established cancers with vaccinations is that these vaccines must be therapeutic and not only preventative, and it is frequently difficult to elicit an immune response strong enough to kill all of the cells of a growing tumour.
- Vaccination with viral antigens or attenuated live viruses can prevent the development of malignancies resulting from viral infection.
- As previously indicated, newly developed HPV vaccines have the potential to reduce the incidence of HPV-induced malignancies, such as uterine cervical cancer.
- This strategy has proved highly effective in lowering the occurrence of feline leukaemia virus–induced hematologic malignant tumours in cats and preventing the herpesvirus-induced lymphoma known as Marek’s disease in poultry.
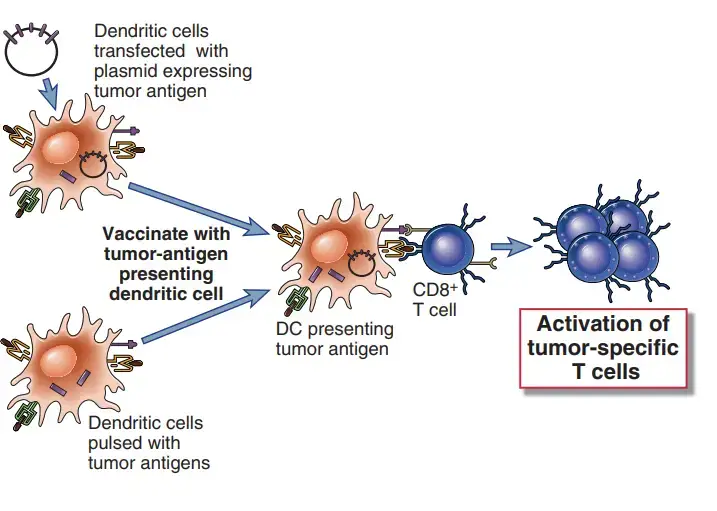
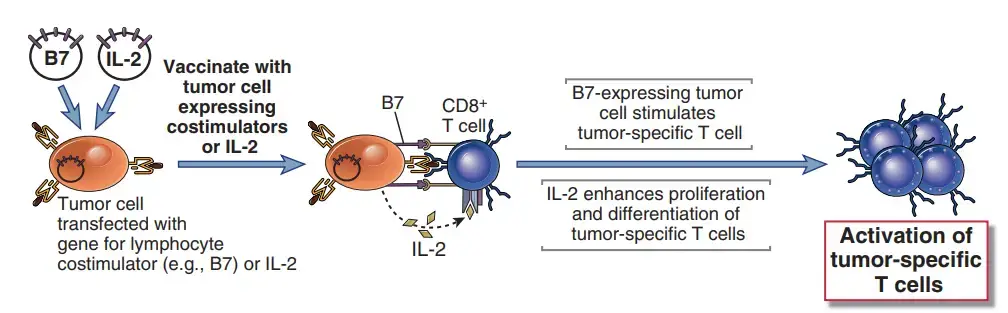
Augmentation of Host Immunity to Tumors with Costimulators and Cytokines
- By producing costimulators and cytokines in tumour cells and treating tumor-bearing individuals with cytokines that increase the proliferation and differentiation of T lymphocytes and NK cells, cell-mediated immunity to malignancies can be boosted.
- Tumor cells may produce poor immune responses because they lack costimulators and typically do not express class II MHC molecules, which prevents them from activating T helper cells.
- Artificially providing costimulation for tumor-specific T cells and providing cytokines that can promote the activation of tumor-specific T cells, particularly CD8+ CTLs, are two potential strategies for enhancing host responses to malignancies.
- Numerous cytokines can also cause nonspecific inflammatory responses, which may have antitumor action on their own.
- Animal trials in which tumour cells were transfected with genes encoding B7 costimulatory molecules and then utilised to vaccinate animals proved the efficiency of boosting T cell costimulation for anticancer immunotherapy.
- These B7-expressing tumour cells induce protective immunity against distantly injected unmodified tumour cells. The accomplishments with experimental tumour models have led to clinical studies in which a patient’s tumour sample is replicated in vitro, transfected with costimulator genes, irradiated, and then reintroduced into the patient. Such strategies may be effective even if the immunogenic antigens produced by tumours are unknown.
- It may be possible to employ cytokines to boost adaptive and innate immune responses against cancer. In trials with mice, the injection of live tumour cells transfected with cytokine genes (such as IL-2 GM-CSF) resulted in the rejection of formed tumours.
- This method has been tried with no results on cancer patients. Additionally, cytokines may be delivered systemically to treat a variety of human malignancies.
- These cytokines affect on vascular endothelium and other cell types.
- IL-2 has been shown to induce tumour regression in approximately 10% of patients with advanced melanoma and renal cell carcinoma and is currently approved as a treatment for these diseases.
- Combining IFN-γα with chemotherapy is approved for the treatment of malignant melanoma and carcinoid tumours. Additionally, it is used to treat lymphomas and leukemias.
- Inhibition of tumour cell proliferation, enhanced cytotoxic activity of NK cells, and increased class I MHC expression on tumour cells, which renders them more susceptible to destruction by CTLs, are likely the mechanisms behind the antitumor effects of IFN-α.
- Other cytokines, such as TNF and IFN-γ, are potent anti-tumor drugs in animal models, but their use in patients is restricted due to the presence of severe toxic side effects. In cancer treatment protocols, hematologic growth factors such as GM-CSF and G-CSF are used to reduce the duration of neutropenia and thrombocytopenia following chemotherapy or autologous bone marrow transplantation.
Blocking Inhibitory Pathways to Promote Tumor Immunity
- Another immunotherapeutic technique is predicated on the notion that tumour cells exploit normal immunological control or tolerance pathways to elude the immune response of the host.
- One series of mouse and human research targeted the B7 inhibitory receptor, CTLA-4, which typically inhibits immune responses to self antigens.
- Combining a tumour vaccine with an antibody that inhibits CTLA-4 produces a potent antitumor T-cell response that eradicates the tumour. Anti–CTLA-4 antibody has been utilised with moderate success in clinical trials with advanced tumour patients.
- The development of autoimmune reactions has been a typical side effect of this medication, which is predicted given the importance of CTLA-4 in maintaining self-tolerance.
- As we discussed before, the PD-L1/PD-1 pathway may also impair T cell responses against malignancies.
- Antibody inhibition of PD-1 is efficient in increasing T cell cytotoxicity against malignancies in mice, and clinical trials utilising this strategy in humans are under underway.
- The decrease of regulatory T cells may also boost anti-tumor immunity, and animal models are currently testing this theory.
Nonspecific Stimulation of the Immune System
- Local administration of inflammatory chemicals or systemic injection of drugs that behave as polyclonal lymphocyte activators may boost immune responses to malignancies.
- The injection of inflammatory chemicals, such as bacillus Calmette-Guérin (BCG), near the sites of tumour growth to induce nonspecific immune stimulation in patients with tumours has been attempted for many years.
- The BCG mycobacteria stimulate macrophages and enhance tumour cell death via macrophage-mediated mechanisms via activating macrophages.
- In addition, the microorganisms may enhance T cell responses to tumour antigens as adjuvants.
- Currently, intravenous BCG is utilised to treat bladder cancer. Previously discussed cytokine treatments represent another means of nonspecifically boosting immune responses.
Passive Immunotherapy for Tumors with T Cells and Antibodies
Passive immunotherapy involves the transfer of immune effectors, such as T cells and antibodies specific to the tumour, into patients. Rapid immunisation against cancers is not accompanied by long-lasting immunity. Certain anti-tumor antibodies are currently authorised for the treatment of specific tumours. Several additional passive immunotherapy techniques are being tested, with mixed results.
Adoptive Cellular Therapy
- Adoptive cellular immunotherapy is the transfer of anti-tumor-reactive immune cells from culture into a tumor-bearing host.
- The cells to be transferred are grown from the lymphocytes of tumor-afflicted patients.
- One procedure for adoptive cellular immunotherapy is to create LAK cells by cultivating peripheral blood leukocytes from tumor-bearing patients in high concentrations of IL-2 and then reinjecting the LAK cells into the patients.
- As previously stated, LAK cells are mostly produced from NK cells. Adoptive therapy with autologous LAK cells, in conjunction with in vivo injection of IL-2 or chemotherapeutic medicines, has produced remarkable outcomes in mice, including regression of solid tumours.
- Trials of human LAK cell treatment have mostly been limited to advanced cases of metastatic malignancies to date, and the efficacy of this method appears to differ from patient to patient.
- TILs are isolated from the inflammatory infiltrate prevalent in and around solid tumours, collected from surgical resection specimens, and expanded in IL-2.
- This strategy is based on the possibility of enriching TILs for tumor-specific CTLs and activated NK cells.
- Numerous centres currently employ TIL treatment for metastatic melanoma. In T cell receptor (TCR) gene therapy, T cells from a tumour patient are transduced in vitro with genes encoding a TCR specific for a tumour antigen, amplified, and then infused back into the patient.
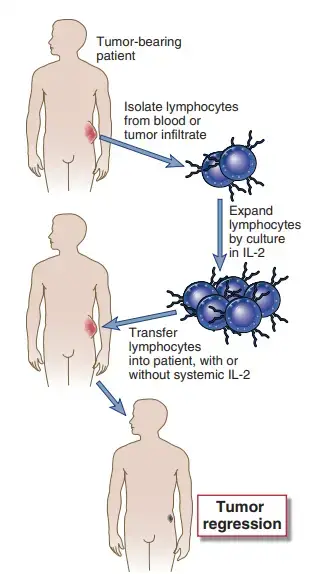
Graft-Versus-Leukemia Effect
- The infusion of alloreactive T cells in conjunction with hematopoietic stem cell transplantation can aid in the eradication of leukaemia in individuals.
- This action is focused at the allogeneic MHC molecules present on the recipient’s hematopoietic cells, including the leukaemia cells.
- The difficulty in using this treatment to improve clinical outcomes is minimising the risky graft-versus-host disease that may be mediated by the same donor T cells.
Therapy with Anti-Tumor Antibodies
- Tumor-specific monoclonal antibodies may be useful for the development of tumor-specific immunotherapies. The possibility of utilising antibodies as “magic bullets” has enticed researchers for many years and remains an active field of study.
- Currently, more than one hundred distinct monoclonal antibodies are being evaluated as therapeutic agents for cancer, either in animal studies or in human trials, and just a handful have been licenced for clinical use.
- Anti-tumor antibodies may remove tumours by the same effector mechanisms that eliminate microorganisms, such as opsonization and phagocytosis, activation of the complement system, and antibody-dependent cellular cytotoxicity.
- These processes are presumably at action in B cell lymphoma patients treated with anti-CD20, one of the most effective anti-tumor antibodies to date. Moreover, certain antibodies may directly trigger intrinsic apoptotic pathways in tumour cells; this is the postulated mechanism for the use of anti-CD30 to treat lymphomas, which is currently undergoing clinical trials.
- Herceptin, a monoclonal antibody specific for the oncogene product HER2/Neu, is an approved treatment for patients with breast cancer whose tumours show high levels of HER2/Neu.
- In addition to stimulating immunological effector mechanisms, anti–HER2/Neu antibodies inhibit the growthsignaling functions of the HER2/Neu molecule.
- Due to the fact that the anti-tumor antibodies used in the initial human trials were mouse monoclonal antibodies, an immune response frequently occurred against the mouse Ig, resulting in anti–mouse Ig antibodies that increased clearance of the anti-tumor antibodies or prevented binding of the therapeutic agent to its target.
- The use of “humanised” antibodies consisting of the variable regions of a mouse monoclonal antibody specific for the tumour antigen coupled with human Fc parts has reduced this issue.
- Antigen-loss variations of tumour cells that no longer express the antigens that the antibodies detect is one of the most challenging complications associated with the use of anti-tumor antibodies.
- It may be possible to circumvent this issue by combining antibodies specific for various antigens expressed on the same tumour.
- In an effort to improve the efficacy of antitumor antibodies, numerous variants have been developed. To enhance the delivery of cytotoxic compounds, radioisotopes, and antitumor medications to the tumour, tumor-specific antibodies can be linked to toxic molecules, radioisotopes, and antitumor drugs.
- Toxins such as ricin and diphtheria toxin are powerful inhibitors of protein synthesis and can be effective at extremely low dosages if coupled to anti-tumor antibodies and delivered to tumours as immunotoxins.
- This method necessitates the covalent attachment of a toxin (lacking its cell-binding component) to an anti-tumor antibody molecule without sacrificing toxicity or antibody specificity.
- Immunotoxin injected systemically is endocytosed by tumour cells, and the toxin component is transported to the intracellular site of action. For this strategy to be successful, numerous practical obstacles must be addressed.
- The antibody’s specificity must be such that it does not bind to normal cells. Prior to being eliminated from the blood by Fc receptor–containing phagocytic cells, a significant amount of antibody must reach the tumour target.
- Due to circulation via normal organs, the poisons, medicines, or radioisotopes bound to the antibody may exert systemic effects. Hepatotoxicity and vascular leak syndromes are typical complications of immunotoxin treatment.
- Immunotoxins can induce antibody responses against the immunotoxins and the injected antibodies. Due to these logistical challenges, immunotoxin clinical trials have had uneven and small success.
- Anti-idiotypic antibodies have been utilised to treat B cell lymphomas that express specific idiotypes of surface Ig. Because it is expressed solely on the neoplastic clone of B cells, the idiotype is a highly specific tumour antigen, and it was originally believed that anti-idiotypic antibodies would be useful therapeutic reagents with 100% tumour specificity.
- Anti-idiotypic antibodies are produced by immunising animals with a patient’s B cell malignancy and then depleting the serum of reactivity against all other human immunoglobulins. The strategy has not been widely effective, mostly due to the selective proliferation of tumour cells with changed idiotypes that are not identified by the anti-idiotypic antibody.
- This conclusion may be attributable in part to the high rate of somatic mutation in Ig genes and the fact that surface Ig is not required for tumour growth.
- Typically, tumour growth is dependent on growth factors, which are possible therapeutic targets. For the treatment of colorectal cancers, antibodies that inhibit the epidermal growth factor receptor are authorised.
- Tumors rely on the creation of new blood vessels that provide oxygen and nutrients to the tumour. This process, known as tumour angiogenesis, is dependent on VEGF and other specific growth factors.
- Several inhibitors of these angiogenic factors can decrease tumour development. Anti-VEGF antibodies are now authorised for clinical usage in conjunction with chemotherapeutic drugs to treat some metastatic cancers, despite their low efficacy.
- Additionally, anti-tumor antibodies are utilised to eliminate cancer cells from the bone marrow prior to autologous bone marrow transplantation.
- In this technique, a portion of the patient’s bone marrow is extracted, and the patient is administered sufficient doses of radiation and chemotherapy to kill tumour cells as well as the remaining normal marrow cells.
- To eliminate most or all tumour cells, the patient’s bone marrow cells are treated with antibodies or immunotoxins specific to tumour antigens.
- The tumor-free bone marrow is put back into the patient to restore the hematopoietic system that was devastated by radiation and chemotherapy.
The Role Of The Immune System In Promoting Tumor Growth
- Although much of the emphasis in tumor immunology has been on the role of the immune system in eradicating tumors, it is clear that the immune system may also contribute to the development of some solid tumors.
- In fact, chronic inflammation has long been recognized as a risk factor for development of tumors in many different tissues, especially those affected by chronic inflammatory diseases such as Barrett’s esophagus, Crohn’s disease, pancreatitis, and prostatitis, for example.
- Some cancers associated with infections are also considered to be a indirect result of the carcinogenic effects of the chronic inflammatory states that are induced by the infectious organisms.
- These include gastric cancer in the setting of chronic Helicobacter pylori infection and hepatocellular carcinomas associated with chronic hepatitis B and C virus infections.
- Although the mechanisms by which chronic inflammation can promote tumor development are not well understood, there are several possibilities, supported by data in rodent models.
- Cells of the innate immune system are considered the most direct tumor-promoting culprits among immune cells. Tumor Associated macrophages of the M2 phenotype as well as other cells are sources of VEGF, which promotes angiogenesis, and matrix metalloproteinases, which modify the extracellular tissue.
- Therefore, chronic activation of some innate immune cells is characterized by angiogenesis and tissue remodeling, which favor tumor formation and spread. Innate immune cells may also contribute to malignant transformation of cells by generating free radicals that cause DNA damage and lead to mutations in tumor suppressor genes and oncogenes.
- Some data suggest that cells of the innate immune system, including mast cells, neutrophils, and macrophages, secrete soluble factors that promote cell cycle progression and survival of tumor cells.
- The transcription factor NF-κB, which is a key mediator of innate immune responses, may play an important role in inflammation associated with cancer progression.
- The adaptive immune system can promote chronic activation of innate immune cells in several ways, including T cell–mediated activation of macrophages in the setting of persistent intracellular microbial infections as well as during early malignant disease even when infectious agents are not present.
- There is also experimental evidence that B lymphocytes may contribute to tumor progression by their secretion of factors that directly regulate proliferation programs in tumor cells as well as by their ability to chronically activate innate immune cells present in early tumors.
- Thus, the adaptive immune system may indirectly enhance the tumor-promoting activities of the innate immune system.
- The tumor-promoting effects of the immune system are paradoxical and a topic of active investigation at present. These effects of chronic inflammation are theoretically also excellent targets for pharmacologic intervention because there are a large variety of effective antiinflammatory drugs already available.
- The challenge for oncologists is to achieve a beneficial balance in which protective anti-tumor adaptive immune responses are not compromised while potentially harmful chronic inflammatory reactions are controlled.