What is Gel Electrophoresis System?
- Gel electrophoresis is a widely used laboratory technique that plays a crucial role in the separation and analysis of biomacromolecules such as DNA, RNA, and proteins. It utilizes the principles of electrophoresis, which involves the migration of charged particles in a solution towards an opposing electrode under the influence of an electric field.
- The concept of electrophoresis was first developed by Arne Tisselius, a Swedish biophysicist, in the 1930s while he was studying blood proteins. Tisselius’ pioneering work in electrophoresis led to his recognition and the receipt of the Nobel Prize in Chemistry in 1948.
- In gel electrophoresis, a gel serves as the medium through which the separation of molecules takes place. The gel can be composed of agarose or other substances that form a matrix with small pores. The size and charge of the molecules determine their movement through the gel when an electric field is applied. The negatively charged nucleic acid molecules, such as DNA and RNA, migrate through the gel with shorter molecules moving faster and farther due to their easier passage through the gel pores. This sieving effect is the fundamental principle behind the separation of nucleic acid molecules in gel electrophoresis. On the other hand, proteins are separated primarily based on their charge in agarose gels since the pores of the gel are too small to effectively sieve proteins.
- Gel electrophoresis has a wide range of applications in various scientific fields. In clinical chemistry, it is utilized to separate proteins based on their charge or size, employing methods like isoelectric focusing agarose. In biochemistry and molecular biology, gel electrophoresis is commonly used to separate a mixed population of DNA and RNA fragments by their length, allowing researchers to estimate the size of DNA and RNA fragments or to isolate specific fragments for further analysis. Gel electrophoresis can also be employed for the separation of nanoparticles.
- The use of gels in gel electrophoresis serves multiple purposes. First, gels act as an anticonvective medium, minimizing thermal convection that can be caused by the application of the electric field. This ensures a more controlled and accurate separation process. Additionally, gels act as a sieving medium, slowing down the movement of molecules based on their size. Furthermore, gels provide a stable support structure for the molecules after electrophoresis, allowing for post-electrophoresis staining or further characterization using techniques like mass spectrometry, RFLP, PCR, cloning, DNA sequencing, or Southern blotting.
- Overall, gel electrophoresis is a powerful technique in molecular biology and biochemistry laboratories, enabling scientists to separate and analyze biomacromolecules based on their size and charge. Its versatility and wide range of applications make it an indispensable tool for researchers in various scientific disciplines.
Definition of Gel Electrophoresis System
Gel electrophoresis is a laboratory technique that uses a gel as a medium to separate and analyze biomacromolecules such as DNA, RNA, and proteins based on their size and charge. An electric field is applied to move the molecules through the gel, with smaller or differently charged molecules migrating faster and farther than larger ones. It is a widely used method in molecular biology and biochemistry for various applications, including DNA fragment analysis, protein separation, and molecular characterization.
Principle of Gel Electrophoresis
The principle of gel electrophoresis is based on the fact that biomolecules, such as DNA, RNA, and proteins, carry electric charges due to their ionizable functional groups. In a solution containing biomolecules, these functional groups result in the presence of positively or negatively charged ions depending on the pH.
When a solution of charged biomolecules is subjected to an electric field, these molecules migrate in the opposite direction of the positive or negative pole. The rate of migration of each molecule depends on its mass and net charge. Negatively charged particles, like nucleic acids, move towards the anode (positive pole), while positively charged particles move towards the cathode (negative pole). This differential migration based on charge and mass allows for the separation of biomolecules with similar properties.
To overcome the limitations of earlier support matrices used in zone electrophoresis, gels are employed as a support matrix in gel electrophoresis. In zone electrophoresis, liquid samples are mixed with a loading buffer and applied to a specific area or zone on the gel matrix, which is pre-soaked with electrophoresis running buffer. Once the electric field is applied, the molecules start migrating through the gel. The electric field is removed when the desired separation is achieved.
During migration, molecules with similar characteristics segregate into distinct bands or zones on the gel matrix. This separation is based on the differences in their migration rates, which are determined by their particular properties. After electrophoresis, the gel matrix is typically stained to visualize the separated bands or zones, allowing for further quantitative analysis.
The principle of gel electrophoresis, utilizing the charge and size properties of biomolecules, provides a powerful tool for separating and analyzing DNA, RNA, proteins, and other biomacromolecules in various research and diagnostic applications.
Parts of Gel Electrophoresis Apparatus
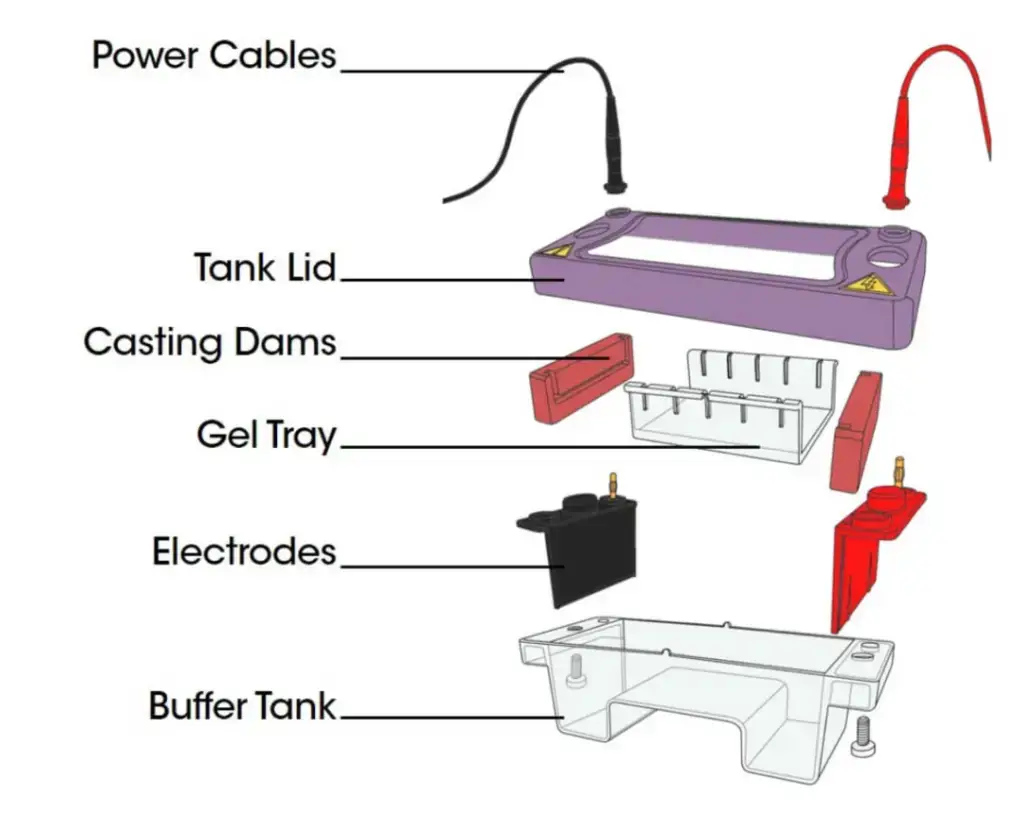
1. Power supply
- The power supply is an essential component in gel electrophoresis systems, providing a steady and controlled electric current to facilitate the migration of charged biomolecules through the gel matrix.
- To maintain a constant migration pace, electrophoresis requires a consistent power supply with either constant current, voltage, or power. The power supply is connected to the gel box using lead cables, typically color-coded with red for the anode (positively charged electrode) and black for the cathode (negatively charged electrode).
- The power supply delivers the electric current from the power source to the gel box through these wires. It is important to ensure that the correct polarity is maintained during the connection. In gel electrophoresis, DNA and RNA molecules, which are negatively charged, are directed towards the positively charged electrode. Therefore, the black wire is attached to the rear of the gel box, allowing these molecules to migrate towards the front of the gel box where the positively charged red wire is attached.
- It is crucial to use a steady power supply to avoid fluctuations in the electric current, which could disrupt the separation process or affect the accuracy of the results. When the current passing through the gel increases, it leads to an increase in resistance and the production of more heat. This heat can cause the dissolved ions in the buffer to thermally agitate, leading to increased evaporation of water from the equipment. Consequently, the ion concentration in the buffer may rise.
- By ensuring a stable power supply, researchers can maintain the desired migration conditions and obtain reliable and reproducible results in gel electrophoresis experiments.
2. Support Media
- Buffers are essential components in various scientific and analytical processes, particularly in fields such as chemistry, biochemistry, and biology. They play a crucial role in maintaining the pH of a system and controlling the electrical charge on solutes. The properties of an ideal buffer are carefully designed to ensure accurate and reliable results.
- One of the primary functions of a buffer is to preserve the analyte’s ability to dissolve. It prevents precipitation or aggregation of the analyte, which could hinder its detection or alter its behavior. Additionally, a good buffer maintains a constant buffering capacity throughout the analysis, ensuring consistent and reliable results.
- Buffers are available in two main types: acidic buffers and basic buffers. Acidic buffers, including citrate, acetate, formate, and phosphate, are used to maintain a lower pH. On the other hand, basic buffers such as tris, borate, and tricine are employed to keep pH levels high. The selection of the appropriate buffer depends on the desired pH range and the specific requirements of the analysis.
- Buffers are typically composed of monovalent ions to maintain equal valency (ionic strength) and molality. This composition ensures stability and consistency in the buffering capacity. However, it’s important to handle prepared buffers with care, especially when they are not in use. Buffers should be chilled to inhibit bacterial growth, as they can create a favorable environment for microbial contamination.
- The use of cold buffers in procedures offers certain advantages. Firstly, it increases the resolution of samples, leading to improved separation and detection of analytes. Additionally, the cold temperature helps reduce solvent evaporation, maintaining the integrity and concentration of the analytes throughout the analysis.
- When it comes to reusing buffers, it is generally safe to reuse them in large volumes up to four times. However, in smaller volumes or for sensitive applications, it is recommended to discard the buffer after a single use. Reusing buffers carries the risk of contamination and degradation, which could impact the accuracy of the results.
- It’s important to note that the high heat generated during certain processes poses a risk to heat-labile chemicals. However, the higher ionic strength of the buffer can be advantageous in obtaining a sharper resolution. Careful consideration should be given to strike a balance between preserving the integrity of the analytes and achieving the desired separation and resolution.
- In conclusion, buffers are crucial components in scientific analyses, providing control over pH and electrical charge. The ideal buffer preserves analyte solubility, maintains consistent buffering capacity, and does not interfere with the detection of intended analytes. Proper handling and storage of buffers are essential to avoid contamination and degradation. By understanding the properties and considerations associated with buffers, scientists can ensure accurate and reliable results in their analytical work.
3. Buffers
- Support media play a crucial role in various scientific and analytical techniques, aiding in the separation and analysis of molecules. These media, such as starch, polyacrylamide, agarose, and cellulose acetate membranes, provide a scaffold or matrix that facilitates the separation process.
- One defining characteristic of support media is their high water content, typically exceeding 90%. This characteristic is crucial as it allows the media to maintain its structure and porosity, enabling the separation of molecules. The high water content ensures that the media remains hydrated and in an optimal state for molecular filtration.
- Support media function as molecular sieves, selectively allowing the passage of certain molecules based on their size. Due to their porous nature, small molecules can diffuse through the support media, while larger molecules are hindered and cannot pass through. This differential permeability is essential for various applications, including chromatography and electrophoresis.
- In order to ensure accurate separation and prevent unwanted interactions, support media must maintain electrical neutrality. This neutrality helps to prevent the influence of electrical charges on the migration or binding of molecules within the media. By maintaining a neutral environment, the support media ensures that separation processes are primarily driven by molecular size and other relevant factors, rather than electrical forces.
- Among the support media commonly used in electrophoresis, agarose gel has gained significant popularity. Agarose, derived from seaweed, is a polysaccharide that forms a gelatinous matrix when mixed with water. This gel structure provides a stable medium for the separation of biomolecules based on their size and charge during electrophoresis. Agarose gel electrophoresis is widely utilized in various fields, including molecular biology, genetics, and biochemistry, to analyze DNA, RNA, and proteins.
- The choice of support media depends on the specific requirements of the separation technique and the nature of the molecules being analyzed. Different media offer varying pore sizes and gel strengths, enabling researchers to tailor the separation conditions to their specific needs.
- In conclusion, support media are indispensable tools in the field of molecular separation and analysis. Through their porous structure, high water content, and electrical neutrality, they enable the selective separation of molecules based on size, charge, and other relevant factors. The use of support media, such as agarose gel, has revolutionized techniques like electrophoresis, allowing researchers to study and understand the intricate details of biological molecules with greater precision.
a. Starch Gel
- Starch gel has a significant historical significance as it was the first gel medium employed for electrophoresis, a technique used for the separation and analysis of proteins. Starch gel facilitated the separation of proteins based on their charge-to-mass ratio and molecular size.
- To prepare starch gel, a colloidal suspension was created by boiling a mixture of starch granules in a buffer solution. As the suspension cooled, the starch chains, particularly the branched chains of amylopectin, intertwined, resulting in the formation of a semisolid gel matrix. This gel matrix provided the necessary support for the separation of proteins during electrophoresis.
- To prevent undesirable effects such as swelling or shrinking of the gel matrix, petroleum jelly was often added. This addition helped maintain the stability and consistency of the gel, ensuring accurate separation of proteins.
- Starch gel offered several advantages for protein separation. It enabled the formation of sharp zones, allowing for clear differentiation between different protein bands. The gel’s resolving power was also high, meaning that it could separate proteins with similar molecular weights but different charge-to-mass ratios effectively.
- However, despite its advantages, the use of starch gel in electrophoresis has diminished over time. One of the primary reasons for this decline is the challenging nature of preparing the gel with reproducibility. Achieving consistent gel preparation can be difficult, leading to variations in the separation results. This lack of reproducibility has prompted researchers to explore alternative gel media that offer greater consistency and reliability.
- As a result, other gel media, such as polyacrylamide and agarose, have become more commonly used in modern electrophoresis techniques. These media offer improved reproducibility, ease of preparation, and better control over gel parameters, leading to more reliable and consistent results.
- In conclusion, starch gel played a crucial role as the first gel medium used for electrophoresis. It allowed for the separation of proteins based on their charge-to-mass ratio and molecular size. While it offered advantages such as sharp zones and high resolving power, the challenging nature of reproducibly preparing the gel has led to its decreased usage in contemporary electrophoresis. Nonetheless, its historical significance and contributions to the development of separation techniques remain noteworthy.
b. Agarose
- Agarose, derived from red seaweeds, is a natural linear polymer that forms an essential component of agar. It consists of chains of galactose and 3,6-anhydro-galactose. When isolated, agarose is typically stored as a dry powder.
- Agarose gel, widely used in gel electrophoresis, is prepared by dissolving the agarose powder in a suitable buffer solution. The solution is then heated until the agarose powder is completely dissolved. Once the solution cools down to room temperature, it solidifies into a gel matrix. The concentration of agarose in the solution buffer determines the pore size of the resulting gel, making it a critical factor in the separation of molecules.
- For distinguishing between DNA and RNA molecules, agarose gel is commonly used at concentrations ranging from 0.8% (W/V) to 5% (W/V). This range allows for effective separation based on the size of the nucleic acid molecules.
- Compared to polyacrylamide gels, agarose gels generally offer relatively poor resolution. However, they have several advantageous properties that make them widely used in gel electrophoresis. Agarose exhibits a low gelling temperature, meaning it solidifies at relatively low temperatures, making it easy to handle during gel preparation. It also possesses a neutral charge, minimizing unwanted interactions with charged molecules. Furthermore, agarose forms stable gels, ensuring that the gel matrix maintains its integrity during the electrophoresis process.
- Another notable characteristic of agarose is its versatility. It can be used in both solid and liquid forms, depending on the experimental requirements. In the liquid form, agarose can be used for techniques like pulsed-field gel electrophoresis, where large DNA fragments are separated.
- In conclusion, agarose is a natural linear polymer derived from red seaweeds and is an essential component of agar. Its ability to form stable gels, low gelling temperature, neutral charge, and versatility make it a perfect material for gel electrophoresis. While it may have relatively lower resolution compared to polyacrylamide gels, agarose gel remains a widely used medium for separating nucleic acid molecules in various scientific disciplines.
c. Cellulose Acetate
- Cellulose acetate has played a significant role in the development of electrophoresis techniques, particularly in the separation of proteins. It was first introduced by Kohn as a means to separate hemoglobin and detect abnormal forms of the protein in blood serum.
- Cellulose acetate is derived from cellulose, a natural polymer found in plant cell walls. To produce cellulose acetate, filter papers composed entirely of cellulose are subjected to acetylation. Acetylation involves introducing acetyl groups to specific locations on the glucose rings of cellulose, typically at the C-3 and C-6 positions.
- Compared to other commonly used electrophoretic matrices like agarose and polyacrylamide, cellulose acetate has larger pores. This means that the gel matrix formed by cellulose acetate allows for the separation of molecules based on their size more easily. The larger pores facilitate the movement of proteins and other biomolecules through the gel, enabling effective separation during electrophoresis.
- Cellulose acetate electrophoresis has been widely used in various fields, including clinical diagnostics and research. It offers advantages such as simplicity, cost-effectiveness, and relatively faster separations compared to other techniques. The larger pores in cellulose acetate gels also allow for the detection of certain proteins that may not be effectively separated using matrices with smaller pores.
- In recent years, other electrophoretic matrices, such as polyacrylamide and agarose, have gained more popularity due to their improved resolution and flexibility in application. However, cellulose acetate still holds its place as a useful medium for certain applications, particularly when larger pore sizes are desirable or when specific proteins or biomarkers are being targeted.
- In summary, cellulose acetate has been an important component in the development of electrophoresis techniques, especially in the separation of proteins. It is derived from acetylated cellulose filter papers and offers larger pores compared to other matrices, allowing for effective separation based on size. While newer matrices have gained prominence, cellulose acetate remains a valuable tool in specific applications within the field of electrophoresis.
d. Polyacrylamide
- Polyacrylamide is a gel matrix widely used in electrophoresis, particularly in the separation of DNA and proteins. It is formed through the copolymerization of acrylamide monomers in the presence of a crosslinking agent known as N, N-methylene-bis-acrylamide, or “bis-acrylamide.” The resulting gel is clear and transparent.
- The size of the pores in polyacrylamide gels is determined by the concentration of acrylamide, which needs to be in proportion to the amount of crosslinking agent used. Controlling the acrylamide concentration allows researchers to modulate the pore size, which is critical for achieving the desired separation of molecules based on their size.
- The concentration of acrylamide gel used in electrophoresis varies depending on the specific application and the target molecules. When separating DNA and proteins, a relatively small amount of acrylamide gel is typically employed, ranging from 3% to 15%. This range is suitable for effectively separating molecules within the size range of DNA and proteins.
- In sodium dodecyl sulfate-polyacrylamide gel electrophoresis (SDS-PAGE), proteins are separated under denatured conditions, primarily based on their size. In this technique, a higher percentage of acrylamide gel, typically ranging from 10% to 20%, is used. The higher acrylamide concentration allows for better resolution and separation of proteins with different sizes, aiding in the analysis of protein samples.
- Polyacrylamide gels offer several advantages in electrophoresis. They provide a stable and consistent gel matrix that facilitates the migration of molecules. The gels are chemically inert and can be prepared with a high degree of reproducibility. The transparency of polyacrylamide gels allows for easy visualization and analysis of separated molecules.
- It is worth noting that polyacrylamide gels are typically formed by polymerization through a chemical reaction and require careful handling of the acrylamide and crosslinking agent due to their potential toxicity in their liquid state. However, once polymerized, the resulting gel matrix is safe to use.
- In conclusion, polyacrylamide gels are commonly used in electrophoresis techniques for the separation of DNA and proteins. The concentration of acrylamide controls the pore size, enabling the desired separation based on molecule size. Whether used for separating DNA or proteins, polyacrylamide gels provide a versatile and reliable medium for various analytical applications in the field of molecular biology and biochemistry.
4. Electrophoresis chamber
- An electrophoresis chamber is an essential component in the process of electrophoresis, providing a controlled environment for the separation and analysis of biomolecules. It typically consists of a plastic container or tank that is filled with a buffer solution.
- The buffer solution serves multiple purposes in the electrophoresis chamber. Firstly, it creates a conductive environment necessary for the movement of charged biomolecules during electrophoresis. Secondly, it helps maintain a consistent pH level, which is crucial for optimal separation. Additionally, the buffer solution helps dissipate heat generated during the electrophoresis process, preventing the gel matrix from overheating.
- One notable feature of an electrophoresis chamber is its transparent lid, allowing researchers to visually monitor the migration process of biomolecules. The transparent lid enables real-time observation of the separation, enabling researchers to make adjustments if necessary or assess the progress of the separation.
- The electrophoresis chamber is wired to a power supply, which provides the electrical current needed for the separation process. The power supply generates an electric field that causes charged biomolecules to migrate through the gel matrix within the chamber. The chamber’s design ensures that the electrical current is evenly distributed throughout the gel, promoting consistent and reproducible results.
- The dimensions and design of electrophoresis chambers can vary depending on the specific application and the size of the gel being used. Some chambers are designed for horizontal electrophoresis, where the gel is placed horizontally between two electrodes. Others are designed for vertical electrophoresis, where the gel is positioned vertically between the electrodes. The choice of chamber depends on factors such as the type of electrophoresis technique, the sample size, and the desired separation conditions.
- In conclusion, the electrophoresis chamber is a crucial component in the process of electrophoresis. It provides a controlled environment for the separation of biomolecules, allowing researchers to visualize the migration process and regulate the conditions for optimal separation. Wired to a power supply, the chamber enables the application of an electric field to facilitate the movement of charged biomolecules through the gel matrix.
5. Electrodes
- Electrodes are crucial components in the process of electrophoresis, playing a fundamental role in separating molecules based on their charge. In gel electrophoresis, two platinum electrodes are commonly used: the anode and the cathode.
- The anode is the positively charged electrode, while the cathode is the negatively charged electrode. These electrodes create an electric field within the electrophoresis system, which drives the movement of charged molecules through the gel matrix.
- Due to their opposite charges, the anode and cathode attract and bind ions with corresponding charges. Positive ions are attracted to the anode, while negative ions are attracted to the cathode. This differential attraction ensures that charged molecules within the gel experience a force that propels them toward the respective electrode of opposite charge.
- As the electric current passes through the gel, the charged molecules migrate toward their respective electrodes based on their charge. This migration enables the separation of biomolecules, such as DNA fragments or proteins, according to their charge-to-mass ratio or size, depending on the specific technique being employed.
- Platinum electrodes are often chosen for their excellent conductivity, chemical stability, and resistance to corrosion. These properties allow for reliable and consistent performance during electrophoresis experiments. Other electrode materials, such as gold or stainless steel, may also be used depending on the specific application or experimental requirements.
- In summary, electrodes, particularly the anode and cathode, are integral to the process of electrophoresis. They create the electric field necessary to drive the migration of charged molecules through the gel matrix. The anode attracts positive ions, while the cathode attracts negative ions, ensuring the separation of biomolecules based on their charge. Platinum electrodes are commonly used due to their conductivity and stability, contributing to the success and accuracy of electrophoresis experiments.
6. Container for staining and de-staining gel
- A container for staining and de-staining gels is an essential tool used in the process of gel electrophoresis. It provides a suitable environment for applying staining reagents to visualize and analyze separated biomolecules. Here are some key aspects of containers used for staining and de-staining gels:
- Trays and containers are commonly used for gel staining and de-staining. These containers come in various forms, including lidded boxes and open-form boxes, depending on the specific requirements and preferences of the researcher.
- Containers for staining and de-staining gels are typically constructed with a propylene base. Propylene, or polypropylene, is a durable and chemically resistant material that can withstand exposure to various staining reagents, chemicals, and stains commonly used in gel electrophoresis experiments.
- Transparency is an important characteristic of these containers. They are designed to be transparent, allowing researchers to easily monitor and visualize the staining and de-staining process without the need to open the container. The transparency enables real-time observation and assessment of the progress and intensity of the staining or de-staining reaction.
- Closely fitted lids or covers are often incorporated into these containers. The tight-fitting lids help to prevent evaporation and contamination during the staining or de-staining process. They create a sealed environment within the container, ensuring that the staining reagents or de-staining solutions remain in contact with the gel without any external interference.
- Containers for staining and de-staining gels are designed to be resistant to chemicals and stains. They are specifically engineered to withstand exposure to various staining agents and de-staining solutions without experiencing degradation or compromising the integrity of the container. This chemical resistance ensures that the container remains functional and durable throughout multiple experiments.
- In summary, containers used for staining and de-staining gels are essential tools in gel electrophoresis experiments. They come in different forms, such as lidded boxes and open-form boxes, and are typically constructed with a propylene base. These containers are transparent, closely fitted, and resistant to chemicals and stains, providing a reliable and convenient environment for staining and de-staining gels in a controlled and efficient manner.
7. Gel Caster and Comb
- The gel caster and comb are essential tools used in the preparation of gel matrices for electrophoresis experiments. They play a crucial role in creating wells within the gel for sample loading and ensuring the integrity of the gel matrix during the separation process. Here are some key aspects of gel casters and combs:
- The gel caster serves as a container or mold where the gel is poured and allowed to solidify. It is designed to hold the gel solution in a defined shape and size, ensuring consistent and reproducible gel matrices for electrophoresis. The gel caster is typically made of a durable material such as acrylic or glass and is compatible with the chosen gel chemistry.
- Once the gel solution is prepared, it is poured into the gel caster. The gel caster is then placed inside the electrophoresis apparatus, where the gel will solidify or polymerize, depending on the gel chemistry being used. The gel caster helps create a uniform gel matrix that will serve as the medium for sample separation during electrophoresis.
- The comb is an accessory used in conjunction with the gel caster to create wells within the gel matrix. The comb is made of a rigid material such as plastic or stainless steel and has evenly spaced teeth or prongs. These teeth penetrate the gel solution when inserted into the gel caster, creating wells of consistent size and spacing.
- The number and size of the wells are determined by the comb used. Different comb configurations allow for the creation of varying numbers of wells, accommodating different sample loading requirements. The comb is inserted into the gel solution before it solidifies, ensuring that the wells are formed during the gel’s polymerization process.
- The wells created by the comb serve as the designated locations for loading samples onto the gel. Samples containing biomolecules, such as DNA fragments or proteins, are loaded into the wells using a pipette or other appropriate loading method. The wells provide defined regions where the biomolecules will separate during electrophoresis, facilitating analysis and identification.
- Gel casters and combs are designed to be compatible with the specific gel chemistry being used, ensuring optimal gel formation and sample loading. They are reusable tools that can be cleaned and sterilized between experiments to maintain hygiene and prevent contamination.
- In summary, gel casters and combs are essential tools in gel electrophoresis experiments. The gel caster holds the gel solution, allowing it to solidify into a defined gel matrix within the electrophoresis apparatus. The comb creates wells within the gel, providing designated locations for sample loading. Together, these tools ensure the reproducibility and integrity of the gel matrix, enabling effective separation and analysis of biomolecules during electrophoresis.
Types of Electrophoresis
There are several types of gel electrophoresis, namely:
- Agarose gel electrophoresis
- Paper gel electrophoresis
- Pulse-field gel electrophoresis (PFGE)
- SDS- PAGE
- Polyacrylamide Gel Electrophoresis (PAGE)
- Immunoelectrophoresis (Rocket Electrophoresis)
- 2D- electrophoresis
- Difference Gel Electrophoresis (DIGE)
1. Agarose gel electrophoresis
- Agarose gel electrophoresis is a widely used technique in molecular biology and biochemistry for the separation and analysis of nucleic acids and proteins. Agarose, a natural linear polysaccharide derived from red seaweed agar, forms the gel matrix that allows the molecules to migrate through an electric field based on their size and charge.
- To prepare an agarose gel, a casting tray with a comb is used to create slots or wells where the samples will be loaded. The concentration of agarose in the gel can vary depending on the desired resolution. Higher agarose concentrations offer greater resolving power, allowing for the separation of smaller fragments with higher precision.
- Agarose gel electrophoresis is particularly suitable for the separation of linear nucleic acid fragments, such as DNA. It is commonly employed for DNA fragment analysis ranging from 100 base pairs to 20 kilobase pairs. For larger DNA fragments, a modified technique called pulsed-field gel electrophoresis is often used.
- However, agarose gel electrophoresis is not limited to nucleic acids. It can also be utilized for the separation of proteins. In isoelectric focusing (IEF), a form of electrophoresis, a low concentration agarose gel is employed to separate amphoteric molecules based on their isoelectric point (pI). This technique is useful for studying proteins with different charges.
- Moreover, advancements have been made in agarose gel systems to accommodate the separation of large proteins. By combining agarose gel with sodium dodecyl sulfate (SDS), proteins ranging from 200-4,000 kDa can be effectively separated. This method provides a valuable tool for the analysis of larger proteins in various research applications.
- Overall, agarose gel electrophoresis is a versatile and widely used technique in molecular biology and biochemistry. Its ability to separate nucleic acids and proteins based on size and charge makes it an essential tool for DNA fragment analysis, protein separation, and other research endeavors in the field of life sciences.
2. Paper gel electrophoresis
Paper gel electrophoresis is a technique commonly used in clinical settings to investigate serum and other bodily fluids for diagnostic purposes. It offers certain advantages and disadvantages compared to other types of gel electrophoresis. Here are some key characteristics of paper gel electrophoresis:
- Clinical Application: Paper gel electrophoresis is frequently employed in clinical laboratories to analyze serum and other bodily fluids for the presence of proteins and other biomolecules. It aids in the identification and characterization of specific proteins, allowing for the diagnosis of various diseases and medical conditions.
- Non-Transparent and Non-Toxic: Unlike other gel materials such as agarose or polyacrylamide, paper used in paper gel electrophoresis is non-transparent. This characteristic prevents real-time visualization of the electrophoretic process. Additionally, the paper material is non-toxic, ensuring the safety of both the researchers and the samples being analyzed.
- Convenient Storage: Paper gel electrophoresis is advantageous in terms of storage. Unlike gel matrices that require specific conditions and may degrade over time, paper gels are relatively easy to store. They can be air-dried and kept at room temperature, offering convenience and long-term stability.
- Protein Adsorption: One of the limitations of paper gel electrophoresis is the significant adsorption of proteins onto the paper matrix. The hydroxyl (OH) groups present in cellulose, the main component of paper, have a tendency to bind with proteins. This interaction slows down the electrophoretic motion of proteins, causing them to trail and reducing the resolution of separated bands.
- Poor Conductivity: Paper itself has poor electrical conductivity compared to other gel materials. This limited conductivity can affect the efficiency of the electrophoretic process, leading to longer separation times or incomplete separation of biomolecules.
- Background Staining: Paper gel electrophoresis is known for its tendency to produce background staining. This staining can interfere with the visualization and analysis of separated bands, making it challenging to accurately interpret the results.
In summary, paper gel electrophoresis is a technique commonly used in clinical settings for analyzing serum and bodily fluids. While it offers convenience in terms of storage and non-toxicity, it also has drawbacks such as protein adsorption, poor conductivity, and background staining. Researchers must carefully consider these factors when selecting the appropriate gel electrophoresis technique for their specific application.
3. Polyacrylamide gel electrophoresis (PAGE)
Polyacrylamide gel electrophoresis (PAGE) is a powerful technique used for the separation and analysis of nucleic acids and proteins. Here are some key aspects of PAGE:
- Polyacrylamide Gel Formation: Polyacrylamide gels are formed through the polymerization of acrylamide monomers in the presence of a crosslinking agent called bisacrylamide. The polymerization reaction is catalyzed by ammonium persulphate (APS) and N,N,N’,N’-tetramethylenediamine (TEMED). The concentration of polyacrylamide gel is determined by the proportion of acrylamide monomers and bisacrylamide crosslinker.
- Gel Casting: To create a polyacrylamide gel, a polymerization solution is poured between two vertically positioned glass plates that are sealed at the bottom or in a glass cassette. The solution is allowed to polymerize, forming the gel matrix. In a vertical gel system, a comb is placed on top of the gel to create sample wells where the individual samples can be applied and concentrated. In a horizontal gel system, samples are typically applied onto a strip of filter paper or other materials that are placed directly on top of the gel.
- Applications of PAGE: Polyacrylamide gels are versatile and find applications in the electrophoretic separation of both nucleic acids and proteins. For nucleic acids, agarose gels are often preferred due to the toxicity associated with non-polymerized polyacrylamide gels. However, polyacrylamide gels can be modified to achieve superior resolution. Denaturing polyacrylamide gels, for example, are capable of distinguishing single-stranded DNA molecules that differ by just one nucleotide, making them suitable for DNA sequencing techniques such as Maxam-Gilbert and Sanger sequencing.
- Protein Separation: Polyacrylamide gels are commonly used for the electrophoretic separation of proteins. They offer greater adaptability to various aspects of protein characterization. Non-denaturing or native polyacrylamide gels can distinguish proteins based on differences in their natural structure. On the other hand, higher percentage polyacrylamide gels (10%-20%) containing sodium dodecyl sulfate (SDS) can denature proteins and separate them based on size, rather than conformation. SDS-PAGE is widely used for protein analysis and is an essential tool for protein characterization.
4. Pulsed-field gel electrophoresis (PFGE)
- Pulsed-field gel electrophoresis (PFGE) is a powerful technique for the separation and analysis of high molecular weight DNA, including large DNA fragments and even entire chromosomes. It was first introduced by Shwartz and Cantor in 1984 and has since become an invaluable tool in various scientific fields.
- The principle behind PFGE lies in the manipulation of the electrical field between the electrodes in an agarose gel. By alternating the direction and strength of the electric field, DNA molecules are guided through the gel matrix in a controlled manner, allowing for the separation of DNA fragments that are otherwise difficult to resolve using traditional gel electrophoresis techniques.
- PFGE is particularly useful for the separation and analysis of DNA with many megabases, making it an essential method for studying large genomes, chromosome rearrangements, and genetic stability. Its ability to handle such high molecular weight DNA sets it apart from other electrophoresis techniques.
- One of the major advantages of PFGE is its precision and reproducibility. It consistently produces accurate results, making it a reliable technique for various applications. In the field of food microbiology, PFGE is employed to study the molecular biology of pathogens found in food, allowing for the identification and tracking of specific strains responsible for outbreaks. In the fermentation industry, PFGE helps to monitor the genetic stability of organisms used in the process, ensuring the desired characteristics are maintained.
- PFGE also finds applications in genetic mapping studies, where it is used to detect chromosome rearrangements, perform restriction fragment length polymorphism (RFLP) analysis, and conduct DNA fingerprinting. These techniques aid in understanding the genetic makeup of organisms, identifying genetic markers, and studying genetic variation.
- Furthermore, PFGE plays a crucial role in epidemiology, particularly in the identification of linked strains during hospital outbreaks. By comparing the DNA fingerprints of different strains, healthcare professionals can track the spread of infections and implement appropriate control measures.
- In summary, pulsed-field gel electrophoresis (PFGE) is a versatile and widely used technique for the separation and analysis of high molecular weight DNA. Its applications span across various scientific fields, including molecular biology, microbiology, genetics, and epidemiology. With its precise and reproducible results, PFGE continues to contribute to our understanding of DNA structure, genetic variation, and the dynamics of microbial populations.
5. Sodium dodecyl sulfate- Polyacrylamide gel electrophoresis (SDS-PAGE)
- Sodium dodecyl sulfate-polyacrylamide gel electrophoresis (SDS-PAGE) is a widely used technique in biochemistry for the separation and analysis of proteins. This method, originally developed by U.K. Laemmli and often referred to as the Laemmli Method, utilizes polyacrylamide gel and sodium dodecyl sulfate (SDS) to achieve protein separation based on polypeptide chain length.
- In SDS-PAGE, the gel consists of two regions: the upper stacking gel and the lower separating gel. The stacking gel has larger pores and a slightly acidic pH of 6.8, while the separating gel has smaller pores and a slightly basic pH of 8. This pH difference helps to create a stable pH gradient within the gel, which is important for achieving efficient protein separation.
- Proteins are denatured and uniformly coated with SDS, a detergent, during the sample preparation step. SDS disrupts the tertiary structure of proteins by breaking the disulfide bonds, resulting in the proteins being unfolded and coated with a negative charge proportional to their polypeptide chain length.
- During electrophoresis, proteins migrate through the polyacrylamide gel matrix in response to an electric field. Since the charge-to-mass ratio is relatively uniform for all proteins due to the SDS coating, their migration is primarily determined by their size. Smaller proteins migrate faster and further, while larger proteins migrate more slowly and have shorter migration distances.
- SDS-PAGE is commonly used for protein analysis and characterization. One of its main applications is to estimate the molecular weight of proteins. By comparing the migration of unknown protein samples with that of known molecular weight markers, the approximate molecular weight of the proteins can be determined.
- Furthermore, SDS-PAGE is useful for assessing the purity of protein samples. If a sample contains a single protein, only one band corresponding to that protein should be observed on the gel. Contaminants or impurities may appear as additional bands or smears, indicating the presence of other proteins or degradation products.
- In addition to SDS-PAGE, isoelectric focusing (IEF) is often employed to determine the isoelectric point (pI) of proteins. The pI is the pH at which a protein has no net charge. IEF utilizes a pH gradient in the gel to separate proteins based on their pI values. Proteins migrate to the position in the gel where the pH matches their pI, resulting in a sharp band or spot. This technique is particularly useful for distinguishing isoenzymes, fractionating proteins, and separating amphoteric substances.
- In summary, sodium dodecyl sulfate-polyacrylamide gel electrophoresis (SDS-PAGE) is a widely utilized technique for protein separation and analysis. It allows for the determination of molecular weight and assessment of protein purity, while the combination with isoelectric focusing (IEF) enables the characterization of proteins based on their isoelectric points. SDS-PAGE has become an indispensable tool in various areas of biochemistry, molecular biology, and protein research.
6. 2D gel electrophoresis
- 2D gel electrophoresis is a powerful technique used in the field of proteomics to analyze complex protein mixtures. It was developed by combining the principles of 2DGel, isoelectric focusing (IEF), and SDS-PAGE (sodium dodecyl sulfate polyacrylamide gel electrophoresis).
- The first step in 2D gel electrophoresis involves the separation of proteins based on their charges using IEF. This technique takes advantage of the fact that proteins have different isoelectric points (pI), which is the pH at which they have no net charge. During IEF, an immobilized pH gradient (IPG) gel is used, which creates a pH gradient across the gel. The protein mixture is then applied to the gel, and an electric field is applied. Proteins migrate in the gel until they reach a region where the pH matches their isoelectric point, causing them to stop moving. This results in the separation of proteins based on their charges.
- In the second step of 2D gel electrophoresis, the separated proteins from the IEF gel are further separated based on their molecular weights using SDS-PAGE. Prior to this step, the proteins in the IEF gel are treated with SDS, a detergent that denatures the proteins and imparts a negative charge to them. The SDS-treated proteins are then loaded onto a polyacrylamide gel that contains small pores. An electric field is applied, causing the proteins to migrate through the gel matrix based on their size. Smaller proteins move more easily through the pores and migrate faster, while larger proteins encounter more resistance and migrate slower. This separation based on molecular weight allows for the resolution of individual proteins within the mixture.
- The combination of IEF and SDS-PAGE in 2D gel electrophoresis enables the separation of proteins in two dimensions, resulting in a two-dimensional gel. In this gel, proteins that have similar charges are grouped together along one axis, while proteins with similar molecular weights are grouped together along the other axis. The end result is a grid-like pattern of protein spots, each representing a distinct protein within the mixture.
- By comparing 2D gel electrophoresis patterns from different samples, researchers can identify differences in protein expression levels and detect post-translational modifications. These differences can provide valuable insights into various biological processes and disease states. To visualize the separated proteins, the gel is usually stained with dyes such as Coomassie Brilliant Blue or silver stain.
- In summary, 2D gel electrophoresis is a versatile technique that allows for the analysis of complex protein mixtures. By combining the principles of IEF and SDS-PAGE, it enables the separation of proteins based on their charges and molecular weights, providing researchers with valuable information about protein composition and expression.
7. Immuno-electrophoresis (Rocket Electrophoresis)
- Immuno-electrophoresis, also known as rocket electrophoresis, is a technique used to separate and analyze protein antigens based on their interaction with specific antibodies. This method combines electrophoresis and immunodiffusion to create visible precipitin arcs, resembling rockets, which allow for the identification and quantification of antigens.
- The process of immuno-electrophoresis begins with the preparation of a semi-solid medium, often agar, which contains suitable antibodies dissolved in molten agar solution. This agar-antibody mixture is then poured onto a horizontal plate, creating a gel matrix. Wells are drilled into the gel, and the antigens to be tested are injected into these wells.
- The antigens present in the sample acquire a negative charge at alkaline pH conditions. When an electric field is applied across the gel, the negatively charged antigens migrate towards the anode (positive electrode). As the antigens move through the gel, they encounter the antibodies that are embedded in the gel matrix. If the antibodies are specific to the antigens, they bind together to form an antigen-antibody complex.
- The formation of the antigen-antibody complex leads to the precipitation of the complex within the gel. The immuno-precipitates appear as arcs, often referred to as “rocket” arcs, due to their elongated shape. The position and intensity of the arcs correspond to the concentration and mobility of the antigen in the original sample.
- To visualize and enhance the detection of the immuno-precipitates, the gel is typically stained with a suitable dye, such as Coomassie Brilliant Blue (CBB). The dye binds to the precipitated complexes, making them visible as dark bands or arcs against a lighter background.
- The rocket-like appearance of the arcs in immuno-electrophoresis is a result of the migration of the antigen-antibody complexes in the electric field. The length of the arcs is proportional to the concentration of the antigen in the original sample, allowing for semi-quantitative analysis.
- Immuno-electrophoresis is a valuable technique in immunology and diagnostics as it enables the identification and measurement of specific antigens. By comparing the position and intensity of the precipitin arcs in different samples, researchers can determine the presence, concentration, and relative abundance of antigens. This information is crucial for various applications, including the diagnosis of diseases and the evaluation of immune responses.
- In summary, immuno-electrophoresis, or rocket electrophoresis, combines electrophoretic separation with immunodiffusion to detect and quantify antigens. The formation of antigen-antibody complexes leads to the precipitation of these complexes within a gel matrix, resulting in visible rocket-shaped arcs. This technique provides valuable information about the presence and concentration of antigens in biological samples and has broad applications in immunological research and clinical diagnostics.
8. Difference Gel Electrophoresis (DIGE)
- Difference Gel Electrophoresis (DIGE) is a specialized technique that was developed to overcome certain limitations associated with 2D-PAGE (two-dimensional polyacrylamide gel electrophoresis). DIGE primarily aims to address the quantitative aspect of differential-expression investigations and mitigate the analytical fluctuations encountered in traditional 2D-PAGE.
- One of the key features of DIGE is its ability to visualize multiple protein samples individually within a single gel. This is achieved by labeling up to three distinct protein samples with fluorescent dyes that are size and charge-matched. Commonly used fluorescent dyes for DIGE include Cy3, Cy5, and Cy2. Each dye is attached to the proteins in a specific sample, allowing them to be distinguished from one another during the subsequent analysis.
- The DIGE workflow involves combining the labeled protein samples into a single mixture, which is then loaded onto a gel and subjected to 2D electrophoresis. The 2D electrophoresis separates proteins based on their isoelectric point (pI) in the first dimension and their molecular weight in the second dimension. This separation enables the visualization and comparison of protein expression patterns across the different samples.
- By incorporating multiple samples in a single gel, DIGE provides several advantages over traditional 2D-PAGE. Firstly, it eliminates the need to run separate gels for each sample, reducing technical variability and enhancing experimental reproducibility. Secondly, DIGE allows for direct comparison between samples on the same gel, minimizing variations arising from gel-to-gel variability. This feature is particularly valuable in differential-expression studies, where quantifying changes in protein expression levels between different conditions or treatments is of interest.
- Following electrophoresis, the gel is scanned using a fluorescence imaging system that can detect the emission signals of the different fluorescent dyes. The scanned images are then analyzed using specialized software, which facilitates the quantification and comparison of protein expression levels across the samples. The software assigns different colors to the spots representing proteins from each sample, enabling the identification of differentially expressed proteins.
- In summary, Difference Gel Electrophoresis (DIGE) was developed to address the quantitative aspects of differential-expression studies and overcome some of the challenges associated with 2D-PAGE. By employing fluorescent dyes to label multiple protein samples and combining them in a single gel, DIGE allows for direct comparison of protein expression levels and improves the accuracy and reproducibility of experimental results.
Operating procedures of Electrophoresis
The operating procedures of electrophoresis, specifically focusing on DNA gel electrophoresis, can be summarized as follows:
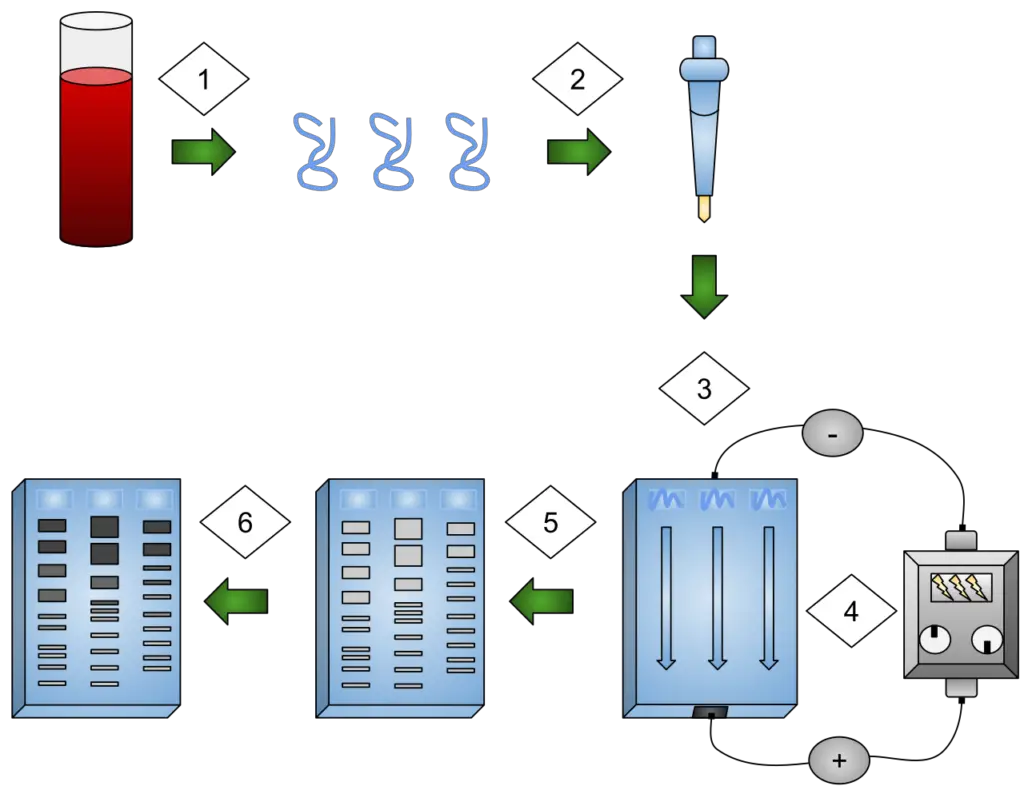
- Preparing the samples for running: The DNA samples are isolated and preprocessed, such as through PCR (polymerase chain reaction) or enzymatic digestion. The samples are then mixed with a loading dye containing a basic blue dye, which aids in visualizing the movement of the samples through the gel.
- Preparing the agarose gel solution: A solution of agarose in TAE (Tris-acetate-EDTA) buffer is prepared. The concentration of agarose in the TAE buffer determines the porosity of the gel and is chosen based on the expected size range of the DNA fragments. The agarose-TAE solution is heated to dissolve the agarose and then cooled to a suitable temperature.
- Casting the gel: The agarose-TAE solution is poured into a casting tray, and a comb is inserted to create wells at one end of the gel. The gel solution is allowed to cool and solidify, forming a gel slab with wells at the top.
- Setting up the electrophoresis chamber: The solidified gel is placed into an electrophoresis chamber filled with TAE buffer. The gel is positioned so that the wells are closest to the negative electrode (cathode) of the chamber.
- Loading the gel: The DNA samples, along with a DNA ladder as a size reference, are loaded into the wells using a micropipette. The samples are carefully placed into individual wells.
- Electrophoresis: The electrophoresis chamber is connected to a power supply, with the negative and positive leads attached to the chamber. The power supply is turned on, creating an electric field across the gel. The negatively charged DNA fragments migrate through the gel, moving away from the negative electrode (cathode) towards the positive electrode (anode).
- Stopping electrophoresis and visualizing the DNA: Once the DNA fragments have migrated to a desired distance, the power supply is turned off. The gel is carefully removed from the chamber and placed in an ethidium bromide solution, which intercalates with the DNA and allows visualization under UV light. Alternatively, ethidium bromide can be added directly to the agarose gel solution during gel preparation. The gel is then exposed to UV light, and a photograph is taken to capture the DNA bands. The DNA ladder helps estimate the sizes of the DNA fragments in the sample lanes.
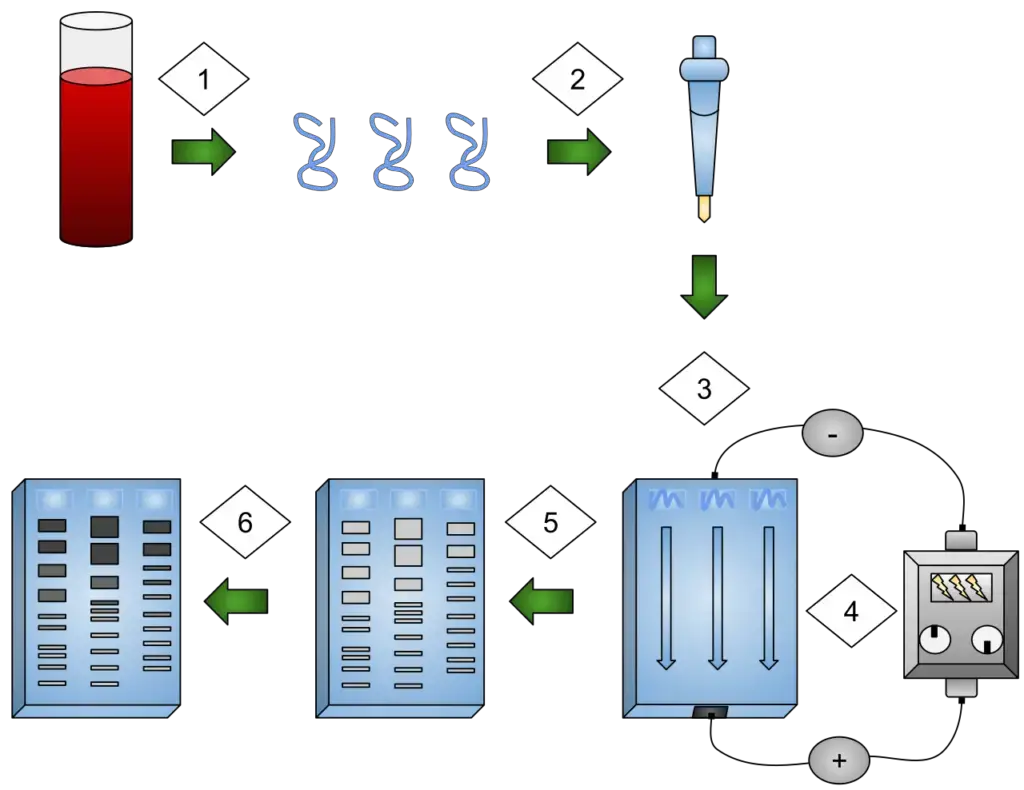
Modifications of gel electrophoresis
Gel electrophoresis is a versatile technique that can be modified in various ways to suit the specific objectives of the analysis. Here are some common modifications of gel electrophoresis:
- Two-dimensional gel electrophoresis: Two-dimensional gel electrophoresis involves performing an additional round of electrophoresis in a direction perpendicular to the first round, known as second-dimensional electrophoresis. This technique provides additional information about the samples being analyzed. The gel from the first round can be used directly for the second-dimensional analysis or can be processed or modified before the second round. This modification is applicable to both agarose and polyacrylamide gel electrophoresis.
- Buffer system: continuous and discontinuous system: Gel electrophoresis is typically performed in a homogenous buffer system, as in the case of zone electrophoresis. However, it can also be conducted in a non-homogenous or discontinuous buffer system. This involves using different gel-casting and electrophoresis running buffers and combining two gels made from different buffers. One well-known example of discontinuous gel electrophoresis is SDS-polyacrylamide gel electrophoresis (SDS-PAGE), where the gel consists of a stacking gel on the upper part and a separating gel on the lower part. Stacking gels have larger pores and serve as sample concentration sites, while separation gels have smaller pores for electrophoretic separation.
- Gel condition: native and denaturing gels: Gel electrophoresis can be modified by using denaturants or dissociating agents in the system. Denaturants are added to gel-casting, sample loading, and running buffers to destabilize intramolecular bonds of nucleic acids and proteins. Denaturing conditions force nucleic acids to exist as single-stranded molecules and proteins to unfold, allowing mobility during electrophoresis based solely on size. In contrast, native gels allow the analysis of nucleic acids and proteins based on their conformation, providing functional information about the biomolecules.
- Gradient gels: Gradient gels are polyacrylamide gels where the gel concentration varies uniformly throughout the gel. This is achieved by pouring a higher-concentration gel at the bottom, followed by a mixture of higher and lower concentrations. The gel is polymerized, resulting in a higher concentration at the bottom and a lower concentration at the top. Gradient gels create a zone of sharpening effect, increasing resolving power compared to a single-percentage gel. They enable the electrophoretic separation of proteins with similar masses and the determination of the molecular diameter of proteins in their native states.
These modifications of gel electrophoresis allow researchers to tailor the technique to their specific experimental needs, enhancing the resolution, quantification, and analysis of nucleic acids and proteins.
Applications of Electrophoresis
Electrophoresis is a versatile technique with numerous applications in various fields of research and analysis. Here are some notable applications of electrophoresis:
- DNA fingerprinting: Electrophoresis is widely used in DNA fingerprinting, which involves separating DNA fragments to investigate crime scenes, establish paternity, and perform forensic analysis. By comparing the patterns of DNA bands obtained through electrophoresis, unique profiles can be created for individuals, aiding in criminal investigations and determining familial relationships.
- Genetic variations and protein analysis: Electrophoresis is utilized to detect genetic variations and analyze proteins implicated in health and illness. It helps in understanding genetic disorders, identifying disease markers, and studying protein expression patterns in different biological samples.
- Nucleic acid and protein detection and purification: Electrophoresis is employed for the detection and purification of nucleic acids and proteins in scientific research. It enables the separation and isolation of specific target molecules, allowing further analysis and characterization.
- Pathogen detection: Electrophoresis plays a crucial role in identifying pathogens in blood, tissues, and sources like food. By analyzing the presence and characteristics of nucleic acids or proteins associated with pathogens, electrophoresis aids in diagnosing infectious diseases and monitoring food safety.
- Identification and purification of biomolecules: Electrophoresis is instrumental in identifying and purifying proteins and nucleic acids for further analysis. It serves as a preliminary step before techniques such as mass spectrometry or DNA sequencing, enabling a more detailed examination of these biomolecules.
- Blotting methods and evolutionary studies: Electrophoresis is used in blotting techniques such as Southern blotting (DNA), Northern blotting (RNA), and Western blotting (protein) to analyze macromolecules and study gene expression patterns. Additionally, electrophoresis contributes to evolutionary studies by aiding in DNA profiling, allowing differentiation of species and understanding evolutionary relationships.
- Evaluation of PCR results: Polymerase Chain Reaction (PCR) is a widely used technique for amplifying DNA sequences. Electrophoresis is employed to evaluate the results of PCR by separating and visualizing the amplified DNA fragments, confirming the success of the amplification process.
- Vaccine development and manufacturing: Electrophoresis plays a role in vaccine development and manufacturing processes. It helps analyze vaccine components, monitor protein purification, and assess the quality and integrity of the final product.
- Taxonomy-DNA profiling: Electrophoresis is utilized in taxonomy-DNA profiling to differentiate species and study evolutionary relationships. By analyzing DNA fragments obtained through electrophoresis, researchers can compare genetic information across different organisms, aiding in taxonomy and evolutionary studies.
Advantages of Electrophoresis
Electrophoresis offers several advantages that make it a widely used technique in various scientific fields. Here are some key advantages of electrophoresis:
- Affordability: Electrophoresis is a reasonably affordable technique compared to many other analytical methods. It uses relatively simple equipment and reagents, making it accessible to a wide range of researchers and laboratories.
- Direct comparison of results: Electrophoresis allows for the direct comparison of results obtained from different samples or experiments. By visualizing the separated molecules on a gel, researchers can easily compare band patterns, migration distances, and intensities, enabling quick analysis and interpretation of data.
- Ease of use: Electrophoresis is a relatively straightforward technique to carry out. With basic knowledge and training, researchers can perform electrophoresis experiments efficiently. The equipment required is typically simple and user-friendly, making it accessible to both experienced scientists and beginners.
- Compatibility with various sample types: Electrophoresis can be used to test DNA from a wide range of sample types, including blood, tissues, cells, forensic evidence, and food samples. This versatility allows for the analysis of diverse biological and environmental materials.
- Superior resolution: Electrophoresis offers excellent resolution for separating biomolecules, such as DNA fragments, RNA molecules, and proteins. The technique can distinguish molecules with small size differences, providing high-resolution separation and allowing for precise analysis of samples.
- Wide range of pore sizes: Electrophoresis is available in a variety of gel matrices with different pore sizes. This enables the separation of molecules of various sizes, accommodating the analysis of different biomolecules and allowing researchers to optimize the technique for their specific needs.
- Stability under different conditions: Electrophoresis gels exhibit stability over a wide range of pH, temperature, and ionic strength. This stability ensures consistent and reliable separation results, even under varying experimental conditions, enhancing the reproducibility of experiments.
- Transparency to light: Electrophoresis gels are transparent to light, allowing for easy visualization and documentation of the separated molecules. Researchers can use various staining methods or fluorescent dyes to visualize the bands, facilitating accurate analysis and documentation of results.
- Chemical inertness: Electrophoresis gels are chemically inert, meaning they do not interact significantly with the separated molecules or introduce artifacts. This property ensures that the observed migration patterns reflect the intrinsic properties of the biomolecules being analyzed, enhancing the reliability of the results.
- Electric neutrality and hydrophilicity: Electrophoresis gels are electrically neutral and hydrophilic in nature. This property helps maintain stable and consistent migration of charged biomolecules through the gel, ensuring accurate separation and preventing sample loss or distortion.
Overall, the advantages of electrophoresis, including affordability, ease of use, compatibility with various sample types, superior resolution, and stability under different conditions, contribute to its wide applicability and popularity in diverse scientific disciplines.
Limitations of Electrophoresis
Electrophoresis is a widely used technique in molecular biology and biochemistry for separating and analyzing macromolecules, primarily nucleic acids and proteins. However, it also has certain limitations that researchers should be aware of when designing experiments or interpreting results. The following are some of the key limitations of electrophoresis:
- Limited sample analysis: One of the drawbacks of electrophoresis is its limited capacity for sample analysis compared to other techniques like in situ hybridization (ISH). For example, in ISH, researchers can examine gene expression in multiple regions of a tissue sample, such as different brain regions. In contrast, electrophoresis methods can only analyze a limited number of regions simultaneously.
- Measurements are not precise: While electrophoresis can effectively separate proteins based on their molecular weights, the technique has limitations when it comes to precise measurements. Techniques like gel electrophoresis and Western blotting can separate proteins with similar molecular weights, but determining the precise mass of proteins often requires additional methods like mass spectroscopy after protein purification.
- A substantial starting sample required: Unlike DNA and RNA, which can be amplified before electrophoresis, amplification of proteins is impractical. As a result, electrophoresis often requires a sizable tissue sample to obtain sufficient amounts of proteins for analysis. This limitation reduces the technique’s utility, and researchers frequently turn to alternative methods like flow cytometry and immunohistochemistry to analyze protein expression in individual cells.
- Limited visualization facility: Electrophoresis is not well-suited for measuring small molecules such as hormones, neurotransmitters, and ions. These molecules often do not respond adequately to the electrophoresis preparation, commonly known as SDS-PAGE (sodium dodecyl sulfate polyacrylamide gel electrophoresis). Even if they did, their small size makes it challenging to separate them properly, often causing them to migrate out of the gel’s bottom.
- Low throughput: Another limitation of electrophoresis is its relatively low throughput compared to other techniques like polymerase chain reaction (PCR) and flow cytometry. PCR and flow cytometry processes are massively parallel or serial, respectively, allowing for the generation of data at a much faster rate. In contrast, electrophoresis is slower in generating research data and establishing intricate relationships.
Despite these limitations, electrophoresis remains a valuable technique for separating and analyzing macromolecules in many research areas. Researchers should consider these limitations and choose complementary techniques when necessary to overcome the constraints of electrophoresis.
Precautions
When working with electrophoresis systems, it is essential to take certain precautions to ensure safety and obtain accurate results. Here are some precautions to consider:
- Use nonconducting surfaces: It is recommended to work on nonconducting floors and benches made of materials like wood or plastics. This helps prevent electrical currents from flowing through unintended paths, reducing the risk of electrical hazards.
- Avoid unintended grounding points: When operating near an electrophoresis system, it is crucial to be cautious of unintentional grounding points and conductors. These can include sinks, water sources, or other waste outlets. Maintaining distance from such sources minimizes the risk of electrical interference and potential accidents.
- Gentle sample loading: While loading samples onto the gel, it is important to avoid exerting excessive force. Pushing too hard can damage the wells, affecting the separation and migration of the molecules. Careful and gentle sample loading ensures well integrity and accurate results.
- Personal protective equipment (PPE): When preparing the gel and handling potentially hazardous substances, it is important to wear appropriate personal protective equipment. This includes gloves to protect the skin from chemicals and contaminants, face masks to prevent inhalation of harmful substances, and goggles to shield the eyes from splashes or spills.
- Handling EtBr safely: Ethidium bromide (EtBr) is a commonly used fluorescent dye in electrophoresis to visualize nucleic acids. However, it is important to be aware that EtBr is carcinogenic and mutagenic. Therefore, it is crucial to take appropriate precautions when handling it. This may include using protective gloves, avoiding direct contact with skin or mucous membranes, and following proper disposal procedures for EtBr-contaminated waste.
By following these precautions, researchers can ensure a safer working environment and minimize potential risks associated with electrophoresis. It is always important to review and adhere to specific safety guidelines provided by the equipment manufacturer and relevant regulatory agencies when working with electrophoresis systems and related chemicals.
Troubleshooting of Electrophoresis
Troubleshooting is an important aspect of electrophoresis to identify and address issues that may arise during the process. Here are some common troubleshooting scenarios in electrophoresis and their corresponding remedies:
No Bands or Gel Front:
- Possible Cause: Proteins have run off the gel.
- Remedy: Decrease the gel running time or voltage. Check the orientation of the leads connected to the power supply. Increasing the acrylamide percentage of the gel may also help.
- Possible Cause: Not enough protein was loaded on the gel.
- Remedy: Load a higher amount of protein into each well and ensure the accuracy of the protein concentration in each sample.
- Possible Cause: Sample has diffused away from the well prior to applying power.
- Remedy: Decrease the time between loading the sample and starting electrophoresis. If necessary, run fewer gels or fewer lanes simultaneously.
- Possible Cause: Not enough SDS in the sample.
- Remedy: Ensure an adequate concentration of SDS in the sample for proper protein migration.
Sample Doesn’t Sink to the Bottom of the Well:
- Possible Cause: Not enough glycerol in the sample.
- Remedy: Increase the concentration of glycerol in the sample.
Sample Leaking Out of Well:
- Possible Cause: Wells damaged during comb removal.
- Remedy: Remove the gel comb carefully to avoid well damage. Rinse the wells gently with electrophoresis buffer before sample loading to detect any damaged wells.
- Possible Cause: Wells damaged during sample loading.
- Remedy: Load the sample carefully, ensuring that the pipette tip does not touch the bottom or sides of the wells.
Bands are Smeared Vertically:
- Possible Cause: Incomplete protein solubility blocks proteins from entering the gel.
- Remedy: Ensure adequate sonication/homogenization and centrifugation during cell lysate preparation to remove particulate matter.
- Possible Cause: Protein degradation.
- Remedy: Increase or add protease/phosphatase inhibitors during cell lysate preparation. Avoid repeated freeze/thaw cycles of the cell lysate and Western blot samples.
- Possible Cause: Too much protein loaded.
- Remedy: Decrease the amount of protein loaded per well. Ensure accurate protein concentration of the sample.
- Possible Cause: Gel running too fast.
- Remedy: Increase the gel run time.
- Possible Cause: Poor quality or old gel.
- Remedy: Use a fresh gel.
Too Many Bands:
- Possible Cause: Protein degradation.
- Remedy: Increase or add protease/phosphatase inhibitors during cell lysate preparation. Avoid repeated freeze/thaw cycles of the cell lysate and Western blot samples.
- Possible Cause: Protein aggregation.
- Remedy: Ensure an adequate concentration of reducing agent, such as DTT, to reduce disulfide bonds. Heat Western blot samples in a water bath before loading onto the gel.
Gel Running Unusually Slowly:
- Possible Cause: Buffers too concentrated.
- Remedy: Dilute the buffers.
- Possible Cause: Current too low.
- Remedy: Increase the voltage of the gel apparatus.
Gel Running Unusually Fast:
- Possible Cause: Buffers too dilute.
- Remedy: Concentrate the buffers.
- Possible Cause: Current too high.
- Remedy: Decrease the voltage of the gel apparatus.
Protein Bands Too Close Together (Not Completely Resolved):
- Possible Cause: Insufficient run time.
- Remedy: Run the gel for a longer period of time.
- Possible Cause: Incorrect gel pore size.
- Remedy: Use a gradient gel with a higher acrylamide percentage at the bottom than at the top. This allows for adequate resolution of a broader range of protein sizes.
Distorted Bands at the Edges of the Gel:
- Possible Cause: Edge effects.
- Remedy: Do not leave empty wells. Load unused wells with a small amount of protein to prevent edge effects on neighboring lanes.
Bands Form Smiling Shapes:
- Possible Cause: Excessive heat.
- Remedy: Reduce the voltage to prevent “smiling” gel patterns. Ensure proper concentrations of detection reagents to avoid bleaching of target bands.
By identifying the potential causes and implementing the appropriate remedies, researchers can overcome issues that may arise during electrophoresis and obtain accurate and reliable results.
FAQ
What is electrophoresis?
Electrophoresis is a technique used to separate and analyze molecules, such as proteins or nucleic acids, based on their size, charge, or other physical properties using an electric field.
What are the different types of electrophoresis?
There are several types of electrophoresis, including agarose gel electrophoresis, polyacrylamide gel electrophoresis (PAGE), SDS-PAGE, native PAGE, capillary electrophoresis, and isoelectric focusing (IEF).
What is the purpose of electrophoresis?
The main purpose of electrophoresis is to separate and characterize biological molecules based on their physical properties. It is used in various applications, such as DNA sequencing, protein analysis, genetic fingerprinting, and drug development.
How does electrophoresis work?
Electrophoresis works by applying an electric field to a gel or capillary containing the sample. The charged molecules migrate through the gel or capillary based on their charge and size, resulting in separation.
What is the role of a gel in electrophoresis?
The gel provides a matrix through which the molecules can migrate during electrophoresis. Agarose and polyacrylamide gels are commonly used, and their pore size or composition can be adjusted to suit different applications.
How are samples loaded onto an electrophoresis gel?
Samples are loaded into wells created in the gel using a pipette or a specialized sample-loading device. Care must be taken to ensure accurate and consistent loading of samples.
What is the purpose of staining in electrophoresis?
Staining is used to visualize the separated molecules on the gel. DNA or RNA can be stained with dyes like ethidium bromide, while proteins can be visualized using Coomassie Brilliant Blue or silver staining methods.
How is the separated material analyzed after electrophoresis?
After electrophoresis, the separated molecules can be further analyzed using techniques such as Western blotting, Southern blotting, or PCR to study specific molecules of interest.
What are the limitations of electrophoresis?
Some limitations of electrophoresis include the limited number of samples that can be analyzed simultaneously, difficulties in precise quantification, and challenges in separating very small or large molecules.
How do I troubleshoot common issues in electrophoresis?
Common issues in electrophoresis can include poor separation, smearing of bands, or unexpected results. Troubleshooting steps may involve optimizing gel composition, adjusting running conditions, or reviewing sample preparation techniques.