Animal cells are ideal for recombinant protein production because they possess the ability to carry out post-translational modifications, a process that is often lacking in bacterial cells. This capability makes animal cells essential for the commercial synthesis of various biological products, including antibodies, hormones, growth factors, cytokines, and viral coat proteins, which are used in immunization.
There are several objectives behind expressing cloned genes in animal cells. Firstly, cloned genes enable the production of large quantities of labeled cRNA, which are useful as probes for molecular studies. Furthermore, the construction of expression libraries, particularly cDNA libraries, allows scientists to express the DNA of each clone and screen for specific structural or functional characteristics of the encoded protein. Beyond this, gene expression in animal cells facilitates the analysis of gene function at the protein level, supporting commercial protein production, the creation of antibodies, and the entrapment of interacting molecules.
However, expressing cloned genes presents challenges, particularly concerning the stability of the expression vector. Cells producing large amounts of foreign proteins often exhibit slower growth compared to wild-type cells. This growth reduction can lead to the selection of mutants that either lose the expression vector or produce reduced protein levels. A common solution is the use of inducible promoters, which allows researchers to grow large quantities of cells with the vector before triggering protein expression at the optimal time, followed by quick harvesting to minimize vector loss.
The success of any expression strategy also depends on controlling vector segregation and designing vectors that reduce the risk of spontaneous structural mutations. One key strategy involves the use of leader peptide sequences in the vector. These sequences facilitate the secretion of the expressed protein from the cell, which can enhance protein stability by preventing toxic buildup within the cell. Additionally, secretion simplifies the purification process of the protein from the cell culture. Vectors that incorporate a leader peptide are known as secretion vectors and are integral to the efficient production and purification of proteins.
Factors effecting gene expression
The following points outline key determinants affecting gene expression, each contributing to the overall functionality of proteins synthesized within a cell.
- Facilities of the laboratory and local expertise: The availability of advanced laboratory equipment and the expertise of personnel can significantly enhance the efficiency of gene expression experiments. Facilities equipped with cutting-edge technology can facilitate precise gene manipulation and analysis.
- Type of protein to be expressed: Different proteins exhibit unique structural and functional properties that may require specific conditions for optimal expression. For instance, membrane proteins often necessitate specialized systems to maintain their functionality.
- Whether the protein is toxic: Toxic proteins can pose challenges during expression due to their detrimental effects on host cells. Therefore, strategies must be employed to mitigate toxicity, such as using inducible expression systems that allow controlled protein production.
- Whether carbohydrate or other modifications are required: Post-translational modifications, including glycosylation, can be crucial for protein functionality. The expression system used must be capable of facilitating these modifications to produce biologically active proteins.
- Requirement of large yield of protein: For applications such as therapeutic development or industrial use, large quantities of protein may be necessary. This requirement influences the choice of expression system and cultivation conditions, emphasizing the need for scalable methods.
- How to purify protein: The purification process is critical for obtaining functional proteins free from contaminants. Techniques such as affinity chromatography or size-exclusion chromatography are commonly employed to isolate the desired protein efficiently.
- Production costs: The economic feasibility of gene expression projects is influenced by various factors, including the cost of materials, labor, and equipment. Budget constraints can dictate the choice of expression systems and protocols.
- Regulatory considerations: In many cases, particularly in biopharmaceuticals, compliance with regulatory standards is paramount. Understanding the guidelines set by agencies such as the FDA is essential for the approval of products derived from gene expression.
- Safety considerations: Ensuring the safety of both the researchers and the environment is critical during gene expression experiments. This includes assessing potential biohazards associated with the expressed proteins and implementing necessary containment measures.
- Purpose for which it is required: The intended application of the expressed protein—be it for research, therapeutic, or industrial purposes—significantly influences the design and execution of the expression process. Understanding the end goal allows for the optimization of conditions tailored to specific needs.
Eukaryote expression hosts
Eukaryotic cells serve as essential alternatives to bacterial hosts when bacterial systems fail to correctly process expressed proteins. Using eukaryotic expression hosts not only ensures proper protein folding and modifications but also allows researchers to study protein function within a more complex eukaryotic environment. The use of intracellular signal sequences can further direct the expressed proteins to specific organelles for targeted functions.
- Yeast Cells
Yeast is one of the most commonly used eukaryotic expression hosts due to its ease of manipulation and rapid growth.- Yeast cells can perform basic eukaryotic post-translational modifications that are absent in bacterial cells.
- They offer high protein yield with a relatively simpler and cheaper cultivation process compared to mammalian systems.
- Yeast is often used for producing proteins that require glycosylation, although yeast glycosylation patterns differ slightly from those in higher eukaryotes.
- Insect Cells
Insect cells, particularly using the baculovirus expression system, are widely used for protein expression.- This system is favored for producing large quantities of recombinant proteins with proper folding and complex post-translational modifications.
- Insect cells can be grown in suspension cultures, making them ideal for large-scale protein production.
- The baculovirus system is highly efficient, allowing the expression of multiple proteins simultaneously.
- Mammalian Cells
Mammalian cells provide the most suitable expression host for the production of human therapeutic proteins.- These cells ensure correct protein folding, proper glycosylation, and other human-specific post-translational modifications.
- Most mammalian expression systems require integration of the expression construct into the host cell genome, as episomal maintenance is rare for long-term expression.
- Mammalian vectors are based either on bacterial plasmids or viral transduction systems. Viral vectors, particularly recombinant integrating viruses, are often employed for stable expression.
- Mammalian expression vectors commonly use strong viral promoters (such as SV40 early promoter) to ensure robust protein production.
- Inducible promoters (e.g., heat shock promoter or lac promoter) provide control over the expression, activating only the desired foreign gene without interfering with the host’s endogenous genes.
- Features such as a strong translation initiation sequence (Kozak consensus) and polyadenylation sites are essential for efficient protein production. Some vectors also include introns, which may enhance gene expression in mammalian systems.
Analysis of gene regulation
The analysis of gene regulation is a key aspect of understanding how genes are controlled and expressed within an organism. By studying the interactions between regulatory elements and gene expression, researchers can gain insights into the mechanisms governing cellular functions. A common method for this analysis involves the use of reporter genes, which allow scientists to assess the impact of cloned regulatory elements by linking them to genes whose expression is easy to measure.
- Reporter Gene System
In this system, cloned regulatory elements are subcloned into vectors that contain a gene that can be easily measured, known as a reporter gene.- The reporter gene is chosen based on its ability to provide clear, quantifiable results and its absence in the host cell background.
- This setup helps in analyzing how specific regulatory elements control gene expression by observing changes in the activity of the reporter gene.
- Reporter Vectors for Regulatory Element Analysis
Different types of reporter vectors are used depending on the regulatory element being studied.- Promoter Probe Vectors: These vectors contain a reporter gene positioned downstream of a polylinker. Researchers can insert and test putative promoter elements to determine how they influence gene transcription.
- Enhancer Probe Vectors: These vectors include a minimal promoter and a reporter gene. A polylinker allows for the insertion of potential enhancer elements to see how they boost gene expression.
- Terminator Probe Vectors: Designed to analyze bacterial transcription terminators, these vectors help in studying the effects of regulatory sequences involved in stopping transcription.
- Methods of Gene Regulation Analysis
The activity of various regulatory elements is often compared by using reporter constructs.- In-vitro Transcription: This involves using different cell lysates to examine how regulatory elements influence gene expression. It is a straightforward method but offers limited information.
- Transient Transfection: The reporter construct is introduced into cells, allowing for short-term analysis of gene regulation.
- Reporter Transgene: For multicellular organisms, the construct is introduced into the germ line, providing a more detailed analysis of gene regulation in vivo.
- The transgene method offers a more accurate representation of how regulatory elements function within the chromatin structure, overcoming limitations of transient episomal vectors.
- Reporter transgenes also enable the study of spatial and temporal gene expression, revealing how regulatory elements behave under different conditions.
- However, reporter transgenes can sometimes lead to position or dosage effects, where the construct’s location in the genome or its copy number affects gene expression in unpredictable ways.
Gene transfer strategies
There are three main routes for gene transfer to animal cells, each with distinct methodologies and purposes.
- Direct DNA Transfer
This method involves the physical introduction of foreign DNA directly into the cell.- In cultured cells, DNA can be introduced through microinjection, which involves injecting DNA directly into the cell’s nucleus.
- For in-vivo applications, DNA is often delivered by bombardment with DNA-coated metal particles, a process called biolistics or gene gun technology.
- Direct transfer ensures precise delivery of genetic material but can be technically demanding and limited to a small number of cells.
- Transfection
Transfection refers to a variety of techniques, both chemical and physical, that encourage cells to take up DNA from their surroundings.- Chemical methods often use calcium phosphate or liposome-based reagents, which encapsulate the DNA and facilitate its uptake by the cell.
- Physical methods such as electroporation use electrical pulses to temporarily open cell membranes, allowing DNA to enter.
- Transfection is widely used in laboratory settings due to its versatility and ability to deliver DNA into many types of cells.
- Transduction
Transduction leverages the natural ability of viruses to infect cells and deliver their genetic material.- DNA is packaged into an animal virus, which is then used to infect the target cells, introducing the foreign DNA.
- This method mimics natural viral infection processes and is particularly effective for transferring DNA into cells that are difficult to transfect by other means.
- Transduction is commonly used in gene therapy and for stable gene integration.
- Gene Transfer Expression Systems
Various expression systems are used to achieve gene transfer in animal cells, depending on the goal (transient vs. stable expression) and the cell type involved.- Non-replicating Plasmid Vectors:
- In transient assays, non-replicating plasmid vectors are used in many cell lines to temporarily express a gene without integrating it into the genome.
- Stable transformation and long-term expression can be achieved using dominant selection markers that ensure only cells incorporating the plasmid survive.
- CHO cells (Chinese Hamster Ovary cells) are often used with DHFR/methotrexate systems for stable selection and high-level gene expression.
- Plasmids with Viral Replicons:
- SV40 Replicon: This system allows high-level transient expression in CHO cells.
- BPV (Bovine Papilloma Virus) Replicon: This vector is used for stable transformation in various murine cell lines.
- EBV (Epstein-Barr Virus) Replicon: Also used for stable transformation, particularly in murine cells, providing long-term gene expression.
- Viral Vectors:
- Adenovirus E1 Replacement: Transient expression can be achieved in 293 cells, a human cell line frequently used in gene expression studies.
- Adenovirus Amplicon: This is useful for in-vivo gene transfer in various mammalian cells.
- Baculovirus: Primarily used in insect cells for high-level transient expression, often for producing recombinant proteins.
- Vaccinia Virus: Utilized in mammalian cells for transient high-level gene expression.
- Lentivirus: Effective for stable transformation in non-dividing cells, making it a powerful tool in gene therapy and long-term genetic modification.
- Non-replicating Plasmid Vectors:
Construct design for high level trans gene expression in animal cells
Construct design for high-level transgene expression in animal cells involves several strategic considerations to optimize gene expression and ensure the proper functioning of the resulting protein. The following points outline the critical elements that should be incorporated into the design of expression constructs.
- Strong and Constitutive Promoter
A highly active promoter is essential for achieving maximum gene expression.- Commonly used promoters in mammalian cells include the SV40 early promoter and enhancer, the Rous sarcoma virus long terminal repeat (LTR) promoter, and the human cytomegalovirus (CMV) immediate early promoter.
- These promoters drive robust transcriptional activity, significantly enhancing the yield of the target protein.
- Inclusion of an Intron
The presence of an intron within the expression unit typically boosts transgene expression levels.- Introns are particularly important in constructs designed for use in transgenic animals, as they facilitate post-transcriptional modifications.
- Many mammalian expression vectors utilize heterologous introns, such as the SV40 small t-antigen intron or the human growth hormone intron, which contain sequences that match the consensus splice donor and acceptor sites.
- Polyadenylation Signal
A polyadenylation signal is necessary to generate a defined 3′ end of the mRNA transcript.- This poly(A) tail is crucial for the export of mRNA from the nucleus to the cytoplasm and enhances mRNA stability.
- Including this signal helps ensure that the transcript is adequately processed and functional for translation.
- Removal of Unnecessary Untranslated Regions (UTRs)
Both the 5′ and 3′ untranslated regions can contain sequences that inhibit translation due to the formation of secondary structures.- In animal systems, it is often beneficial to eliminate these UTR sequences from transgenic constructs to maximize expression efficiency.
- This removal allows for a more streamlined translation process, improving protein yield.
- Optimization for Translational Efficiency
Incorporating elements that enhance translational efficiency is vital for maximizing protein production.- The presence of a Kozak sequence (5′-CCRCCUAUGG-3′) immediately upstream of the start codon significantly increases the likelihood of successful translation initiation in mammalian cells.
- This optimization ensures that ribosomes effectively recognize and translate the mRNA.
- Incorporation of a Targeting Signal
To ensure proper post-translational modifications, targeting signals must be considered during construct design.- Proteins requiring specific modifications, such as glycosylation, must be directed to the appropriate cellular compartments, such as the secretory pathway.
- Many mammalian expression vectors integrate heterologous signal peptides to facilitate this targeting, ensuring that proteins are correctly modified according to their intended functions.
Map of some commonly used vectors in mammalian cell
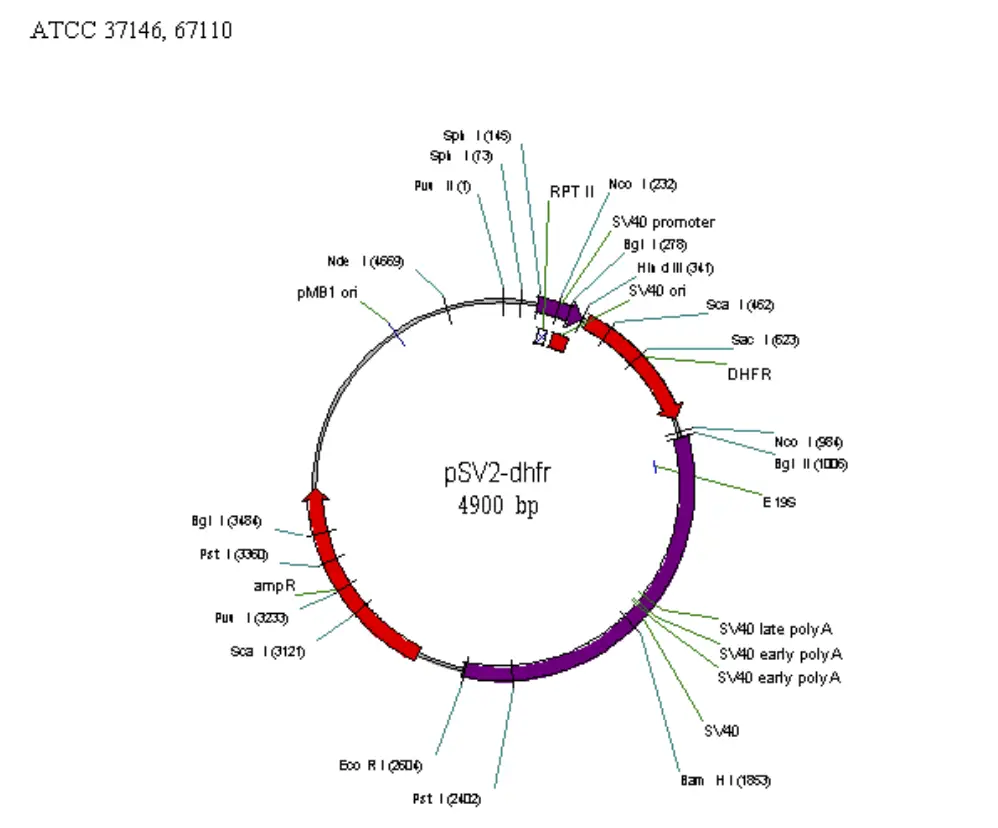
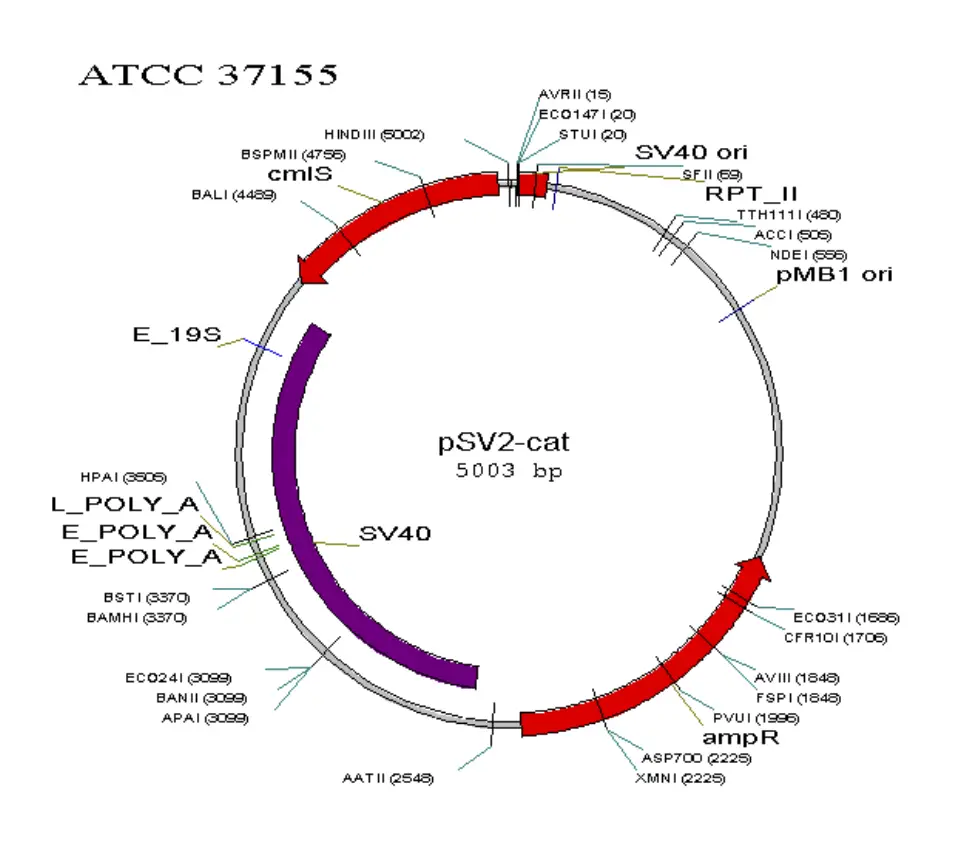
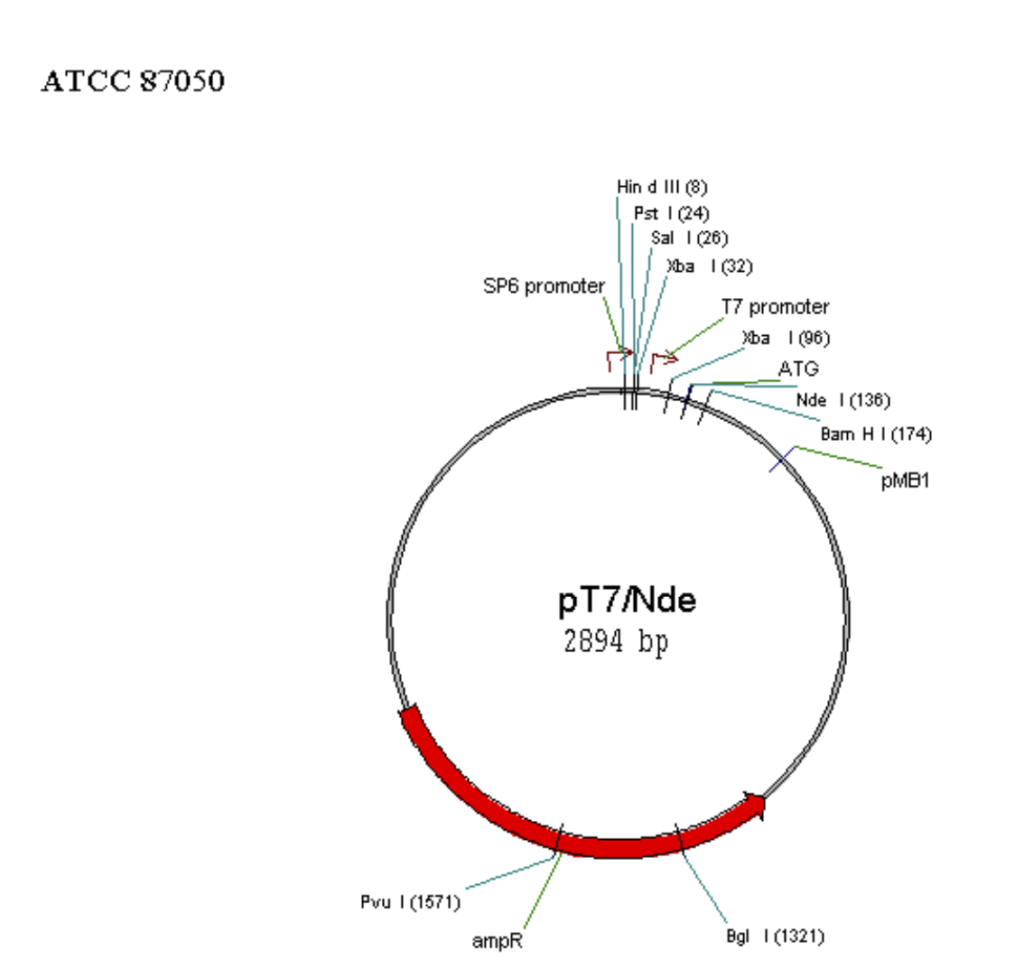
Requirements for mammalian expression system
The following points outline the essential requirements for a successful mammalian expression system.
- Cell Line Selection: The choice of an appropriate mammalian cell line is critical for successful protein expression. Commonly used cell lines include Chinese hamster ovary (CHO) cells and human embryonic kidney (HEK 293) cells, known for their ability to post-translationally modify proteins correctly. CHO cells, in particular, are widely employed in bioprocessing due to their high yield and compatibility with various expression vectors.
- CHO-Derived Lines: CHO-K1 and CHO pro-3 are engineered lines lacking dihydrofolate reductase (DHFR) activity, facilitating easier selection during the protein production process.
- HEK 293 Cells: These cells can be transfected efficiently, with up to 80% showing successful protein expression, making them ideal for both transient and stable transfection.
- Transfection Methods: Effective transfection techniques are necessary to introduce foreign DNA into the host cells. Common methods include:
- Polyethyleneimine (PEI) and Calcium Phosphate: These are standard techniques for delivering plasmid DNA into cells.
- Viral-Mediated Transduction: Systems like BacMam utilize recombinant baculoviruses to facilitate efficient protein expression in mammalian cells, suitable for producing proteins in milligram quantities.
- Vectors: The use of vectors is essential for transporting the gene of interest into the mammalian cell. The choice of vector impacts the efficiency of gene expression.
- Plasmid Vectors: These circular DNA molecules are often used in conjunction with strong viral promoters, such as those derived from SV40 or cytomegalovirus (CMV), to drive transcription.
- Viral Vectors: Adenoviral and retroviral vectors are utilized for stable expression, leveraging strong promoters to enhance gene expression over extended periods.
- Promoter Selection: The effectiveness of a mammalian expression system is significantly influenced by the choice of promoter. Strong promoters ensure high transcription rates, critical for producing sufficient protein levels.
- Viral Promoters: Commonly employed due to their robust transcription capabilities, including those from adenoviruses and retroviruses.
- Non-Viral Promoters: Emerging alternatives, such as the elongation factor-1 (EF-1) promoter, have shown potential in achieving high expression levels comparable to viral promoters.
- Post-Translational Modifications: Mammalian cells are uniquely capable of performing essential post-translational modifications, such as glycosylation, that are crucial for the functionality of many therapeutic proteins. Therefore, selecting cell lines that can properly modify these proteins is vital.
- Scalability and Yield: For industrial applications, the expression system must allow for scalable production. The design of the system should accommodate large-scale cultivation, often in bioreactors, to meet the demand for therapeutic proteins.
- Regulatory Compliance: Adhering to regulatory standards is essential when developing products intended for human use. Understanding the guidelines set by agencies like the FDA ensures that the expression system is compliant with safety and efficacy requirements.
- Safety Considerations: Evaluating the potential risks associated with expressing certain proteins, particularly those that may be toxic or have immunogenic effects, is crucial. Implementing appropriate safety measures protects both researchers and the environment.
Application of mammalian expression system
Below are the key applications of mammalian expression systems.
- Production of Therapeutic Proteins: Mammalian cells serve as the primary hosts for the commercial production of various therapeutic proteins, including monoclonal antibodies (mAbs) and hormones.
- Monoclonal Antibodies (mAbs): CHO cells are the most widely used for large-scale mAb production due to their high yield and ability to perform necessary post-translational modifications. Transient expression systems in COS cells can also be employed for small-scale mAb production, although these cells may lose efficacy over time.
- Optimization Techniques: The application of heterologous promoters, enhancers, and amplifiable genetic markers can enhance antibody production, surpassing yields from traditional hybridomas.
- Production of Urokinase: Urokinase, an essential serine protease involved in clot degradation, is increasingly produced using mammalian expression systems.
- CHO Cells as Hosts: Due to their favorable growth characteristics in bioreactors, CHO cells are preferred for producing recombinant urokinase, ensuring that the protein is adequately modified and functional for therapeutic use.
- Production of Follicle-Stimulating Hormone (FSH): FSH, crucial for reproductive health in both males and females, can be produced recombinantly in mammalian cells.
- Therapeutic Applications: Recombinant FSH produced in CHO cells is utilized for infertility treatments and provides a means to study glycoprotein hormone structure and function without contamination from endogenous hormones.
- Research Advancements: Studies have also focused on producing recombinant forms of FSH from various species, including cynomolgus monkeys, to explore hormonal functions further.
- Reporter Gene Applications: Novel mammalian cell lines can be engineered to express reporter genes, enabling the detection of environmental chemicals that activate specific receptors, such as aryl hydrocarbon or estrogen receptors.
- Treatment of Genetic Disorders: Recombinant proteins produced in mammalian systems are critical for treating genetic diseases.
- Hemophilia B Treatment: Recombinant human Factor IX (rhFIX), produced in human hepatic cell lines, is used for treating hemophilia B, highlighting the system’s capability to produce functional proteins for therapeutic applications.
Limitation of mammalian expression system
Below are the key limitations of mammalian expression systems.
- High Cost: The operational and production costs associated with mammalian expression systems are significantly higher compared to prokaryotic systems.
- Culture Maintenance: The requirement for specialized growth media and conditions for mammalian cells contributes to increased expenses.
- Facility Requirements: Maintaining sterile environments and sophisticated bioreactors adds further to the overall financial burden.
- Complex Technology: The technology required to manipulate and optimize mammalian cells is often intricate and resource-intensive.
- Transfection Techniques: Achieving efficient transfection and stable expression necessitates advanced techniques and expertise, which can complicate experimental workflows.
- Cell Line Development: The creation and maintenance of stable cell lines for protein expression can be time-consuming and technically challenging.
- Risk of Contamination: Mammalian expression systems are susceptible to contamination by animal-derived pathogens, which can pose significant risks in both research and clinical applications.
- Viral Contamination: The potential for contamination with animal viruses can lead to safety concerns, particularly in the production of therapeutic proteins intended for human use.
- Quality Control Challenges: Ensuring the sterility and safety of products derived from mammalian cells requires rigorous and ongoing monitoring, complicating the production process.
- Yield Limitations: While mammalian cells can produce complex proteins, the yields are often lower than those obtained from bacterial or yeast systems.
- Cell Growth Rates: Mammalian cells typically exhibit slower growth rates compared to prokaryotic cells, resulting in longer production times and lower overall yields.
- Process Optimization: The need for extensive optimization to achieve satisfactory protein levels can add to the duration and complexity of the production process.
- Regulatory Hurdles: The use of mammalian cells in therapeutic production entails navigating stringent regulatory requirements.
- Approval Processes: The lengthy and rigorous approval processes for products derived from mammalian systems can delay their entry into the market.
- Documentation Requirements: Comprehensive documentation and compliance with regulatory standards increase the complexity of the production and quality assurance processes.
- Khan KH. Gene expression in Mammalian cells and its applications. Adv Pharm Bull. 2013;3(2):257-63. doi: 10.5681/apb.2013.042. Epub 2013 Aug 20. PMID: 24312845; PMCID: PMC3848218.
- https://ddugu.ac.in/ePathshala_Attachments/E_PS554920@d96b9501i.pdf
- https://epub.ub.uni-muenchen.de/5004/1/004.pdf