What are Cytokines?
- Cytokines are a diverse group of small proteins that play a crucial role in cell signaling. They are involved in mediating communication between cells and regulating various physiological processes. Cytokines are generally unable to cross the cell membrane due to their size, so they interact with specific receptors on the surface of target cells to exert their functions.
- These proteins are essential for autocrine, paracrine, and endocrine signaling, meaning they can act on the same cell that produces them (autocrine), nearby cells (paracrine), or distant cells through the bloodstream (endocrine). Cytokines are particularly important as immunomodulating agents and are involved in the regulation of immune responses.
- There are different types of cytokines, including chemokines, interferons, interleukins, lymphokines, and tumor necrosis factors. They are produced by various cells in the body, such as immune cells (macrophages, B lymphocytes, T lymphocytes, mast cells), endothelial cells, fibroblasts, and stromal cells. It’s worth noting that a single cytokine can be produced by multiple cell types.
- Cytokines act through cell surface receptors and have a significant impact on the immune system. They help modulate the balance between humoral (antibody-mediated) and cell-based immune responses. Additionally, cytokines regulate the maturation, growth, and responsiveness of specific cell populations within the immune system. They can enhance or inhibit the actions of other cytokines in complex ways, contributing to the intricacies of immune regulation.
- While cytokines share similarities with hormones and growth factors in terms of cell signaling, they differ in their characteristics. Hormones are typically produced by specific cell types and circulate in higher concentrations throughout the body. In contrast, cytokines have a more localized effect near the cells that produce them and act at lower concentrations.
- Cytokines have crucial implications in health and disease. They play vital roles in host immune responses to various conditions such as infection, inflammation, trauma, sepsis, cancer, and reproduction. Their dysregulation or imbalance can lead to immune disorders and contribute to the development or progression of diseases.
- The term “cytokines” originates from the ancient Greek words “cyto” (meaning “cell” or “cavity”) and “kines” (meaning “movement”). This name reflects their involvement in cellular communication and their role in coordinating cellular responses.
Definition of Cytokines
Cytokines are small proteins that play a crucial role in cell signaling, mediating communication between cells and regulating various physiological processes.
What is Cytokinesis?
- Cytokinesis is the process by which a cell divides into two daughter cells following mitosis or meiosis. It is a crucial step in cell division and ensures the proper distribution of genetic material and cellular components to the newly formed cells.
- During cytokinesis, the cell undergoes a series of coordinated events to physically separate the cytoplasm and organelles into two distinct daughter cells. This process can vary depending on the cell type and organism, but it generally involves the formation of a contractile ring composed of actin and myosin filaments. The contractile ring contracts, leading to the formation of a cleavage furrow that gradually deepens and eventually separates the cell into two daughter cells.
- Cytokinesis is regulated by various factors, including cytokines. Cytokines are small proteins that play a crucial role in cell signaling and communication. While cytokines are mainly known for their involvement in the immune system, they also have important functions in other biological processes, including cell division.
- Cytokines mediate their effects by binding to specific receptors on the cell surface. These interactions trigger a cascade of intracellular signals that regulate gene expression and cellular behavior. In the context of cytokinesis, certain cytokines can influence the assembly and contraction of the contractile ring, ensuring proper cell division.
- The precise mechanisms by which cytokines regulate cytokinesis are still being investigated, but it is believed that they modulate the activity of proteins involved in the contractile ring formation, such as actin and myosin. By affecting the dynamics of these proteins, cytokines can promote or inhibit the completion of cytokinesis.
- It’s important to note that cytokinesis is distinct from cytokines. Cytokinesis refers to the physical process of cell division, while cytokines are small proteins involved in cell signaling. Cytokines can influence cytokinesis and other cellular processes by transmitting signals between cells and regulating gene expression.
- In summary, cytokinesis is the process of cell division, and cytokines are small proteins that mediate cell signaling. While cytokines play various roles in the immune system, they also contribute to the regulation of cytokinesis by influencing the assembly and contraction of the contractile ring. Understanding the interplay between cytokines and cytokinesis is essential for unraveling the complex mechanisms of cell division and its regulation.
Definition of Cytokinesis
Cytokinesis is the process of cell division in which a single cell divides into two daughter cells, each with its own nucleus and cytoplasm.
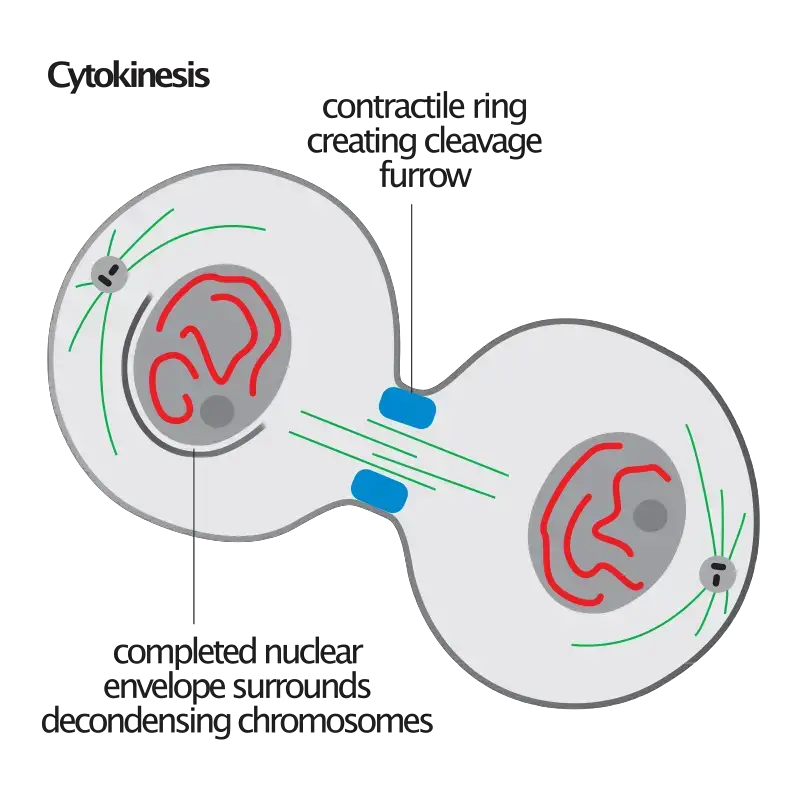
Nomenclature of Cytokines
- The nomenclature of cytokines has evolved over time, initially classifying them as lymphokines, interleukins, and chemokines based on their presumed function, cell of secretion, or target of action. However, due to the redundancy and pleiotropism observed in cytokines, these distinctions have become less relevant.
- The term “interleukin” was initially used to describe cytokines that were believed to primarily target white blood cells (leukocytes). However, the term is now commonly used for newer cytokine molecules and does not necessarily reflect their specific functions. The majority of interleukins are produced by T-helper cells and play important roles in immune regulation.
- Lymphokines are cytokines produced by lymphocytes, while monokines are cytokines produced exclusively by monocytes. These terms were used to describe cytokines based on their cell of origin. However, given the broad range of cells capable of cytokine production, these distinctions are not always accurate.
- Interferons are a specific class of cytokines that are involved in antiviral responses. They play a critical role in host defense against viral infections and can inhibit viral replication within infected cells.
- Colony stimulating factors (CSFs) are cytokines that support the growth and differentiation of specific cell types in semisolid media. They are involved in the regulation of hematopoiesis, the process of blood cell formation.
- Chemokines are cytokines that mediate chemoattraction, also known as chemotaxis, between cells. They are responsible for directing the migration of immune cells to specific sites of infection or inflammation. Chemokines play a crucial role in immune responses and the regulation of cell movement and positioning within tissues.
- In summary, the nomenclature of cytokines was initially based on their presumed function, cell of secretion, or target of action. However, due to the complexity and overlapping functions of cytokines, these distinctions have become less relevant. Cytokines can have diverse functions and are produced by various cell types, contributing to the intricate network of cellular communication and immune regulation.
Properties of Cytokines
Cytokines possess several important properties that contribute to their diverse functions in cell signaling and immune regulation:
- Autocrine, Paracrine, and Endocrine Action: Cytokines can exert their effects on the same cell that produces them (autocrine action), neighboring cells (paracrine action), or distant cells throughout the body (endocrine action). This enables them to communicate and coordinate responses within local environments or throughout the entire organism.
- Pleiotropy: Cytokines exhibit pleiotropic effects, meaning a single cytokine can act on multiple cell types or different cell types can secrete the same cytokine. This allows for the modulation of various cellular processes and the regulation of multiple physiological functions by a single cytokine.
- Redundancy: Different cytokines can stimulate similar functions, resulting in redundancy. This redundancy ensures that even if one cytokine is absent or ineffective, other cytokines can compensate and fulfill the necessary cellular functions. It provides robustness to the immune system and maintains functionality in case of variations or disruptions.
- Cascade Production: Cytokines are often produced in cascades or sequential patterns. The release of one cytokine can stimulate the production and release of other cytokines. This cascade production amplifies the immune response, allowing for a coordinated and dynamic cellular reaction to various stimuli.
- Antagonistic and Synergistic Actions: Cytokines can work both antagonistically and synergistically. Some cytokines may have opposing effects, with one cytokine inhibiting the action of another. On the other hand, cytokines can also have synergistic effects, where the combined action of multiple cytokines produces a more potent or enhanced response compared to their individual effects.
- Receptor-Mediated Effects: Each cytokine has specific cell surface receptors that they bind to, initiating intracellular signaling pathways. These interactions between cytokines and receptors can upregulate or downregulate the activity of genes within the cell, influencing cellular processes, immune responses, and overall cell behavior.
In summary, cytokines exhibit autocrine, paracrine, and endocrine actions, possess pleiotropic effects, display redundancy, are often produced in cascades, can work antagonistically or synergistically, and interact with specific receptors to modulate gene activity. These properties allow cytokines to participate in diverse cellular signaling processes and regulate various aspects of immune responses and other physiological functions.
Classification of Cytokines
Structural Classification of Cytokines
Cytokines can be classified into different structural categories based on their similarities in three-dimensional structure. These structural classifications help in understanding their functional properties and relationships. The main structural classifications of cytokines are as follows:
- Four-α-Helix Bundle Family: This family of cytokines exhibits a three-dimensional structure consisting of a bundle of four α-helices. Within this family, there are three subfamilies:
- IL-2 Subfamily: This is the largest subfamily, including cytokines such as IL-2, erythropoietin (EPO), and thrombopoietin (TPO). Some non-immunological cytokines are also part of this subfamily. The IL-2 subfamily can further be categorized into long-chain and short-chain cytokines based on their topology. Certain members of this subfamily share the common gamma chain as part of their receptor.
- Interferon (IFN) Subfamily: This subfamily consists of interferons, including IFN-alpha, IFN-beta, and IFN-gamma. Interferons are important for antiviral responses and immune regulation.
- IL-10 Subfamily: This subfamily includes IL-10, a cytokine involved in regulating immune responses and inflammation.
- IL-1 Family: The IL-1 family comprises cytokines such as IL-1 and IL-18. These cytokines play roles in inflammatory processes and immune regulation.
- Cysteine Knot Cytokines: This group includes members of the transforming growth factor beta (TGF-β) superfamily, including TGF-β1, TGF-β2, and TGF-β3. These cytokines are characterized by a specific structural motif called the cysteine knot. They are involved in various cellular processes, such as cell growth, differentiation, and tissue development.
- IL-17 Family: The IL-17 family of cytokines is still being studied and characterized. These cytokines have a specific effect on promoting the proliferation of T-cells that cause cytotoxic effects.
By classifying cytokines into different structural categories, we can gain insights into their functional characteristics, receptor interactions, and potential therapeutic applications. Understanding the structural diversity of cytokines contributes to our understanding of their roles in immune responses, inflammation, and various physiological processes.
Functional Classification of Cytokines
Cytokines can be classified based on their functional properties, which provides valuable insights into their roles in immune responses and various physiological processes. One commonly used functional classification divides immunological cytokines into two subsets: type 1 and type 2 cytokines.
- Type 1 Cytokines: These cytokines enhance cellular immune responses. Examples of type 1 cytokines include tumor necrosis factor alpha (TNFα) and interferon-gamma (IFN-γ). They play crucial roles in activating immune cells and promoting cellular immunity against infections and tumors.
- Type 2 Cytokines: These cytokines enhance antibody responses. Examples of type 2 cytokines include transforming growth factor-beta (TGF-β), interleukin-4 (IL-4), interleukin-10 (IL-10), and interleukin-13 (IL-13). Type 2 cytokines are involved in regulating humoral immune responses, including the production of antibodies by B cells and the differentiation of helper T cells.
It is noteworthy that there is often a reciprocal relationship between type 1 and type 2 cytokines, with cytokines in one subset tending to inhibit the effects of those in the other subset. Dysregulation of this balance is being intensively studied for its potential role in the pathogenesis of autoimmune disorders, where the immune system mistakenly attacks the body’s own tissues.
In addition to their immunological roles, cytokines also have important functions in inflammation and oxidative stress. Inflammatory cytokines, induced by oxidative stress, can trigger the release of other cytokines, leading to a cascade of immune responses. This plays a crucial role in chronic inflammation, fever, and the production of acute phase proteins by the liver. Cytokines are involved in both pro-inflammatory and anti-inflammatory pathways, and dysregulation of cytokine signaling can contribute to pathological conditions such as chronic pain and inflammation.
Receptors of Cytokines
Cytokine receptors play a crucial role in mediating the effects of cytokines by facilitating their binding to target cells. Here is a description of the different families of cytokine receptors based on their three-dimensional structures:
- Immunoglobulin (Ig) Superfamily: These receptors are structurally similar to immunoglobulins (antibodies) and are present on various cells and tissues throughout the body. They share homology with cell adhesion molecules and some cytokines. Examples of cytokines that bind to Ig superfamily receptors include interleukin-1 (IL-1) receptors.
- Hemopoietic Growth Factor (Type 1) Family: Receptors belonging to this family have conserved motifs in their extracellular amino acid domain. The IL-2 receptor is a member of this family. Deficiency of the γ-chain of the IL-2 receptor is responsible for X-linked Severe Combined Immunodeficiency (X-SCID).
- Interferon (Type 2) Family: This family of receptors specifically binds to interferon-γ (IFN-γ) and interferon-β (IFN-β). Interferons are involved in antiviral responses and immune regulation.
- Tumor Necrosis Factor (TNF) (Type 3) Family: These receptors share a cysteine-rich extracellular binding domain. The family includes receptors for tumor necrosis factors as well as non-cytokine ligands such as CD40, CD27, and CD30.
- Seven Transmembrane Helix Family: This receptor family, characterized by seven transmembrane helices, is ubiquitous in the animal kingdom. It includes G protein-coupled receptors (GPCRs) that are involved in signaling for hormones and neurotransmitters. Some chemokine receptors, including CD4 and CCR5, which are binding proteins for HIV, also belong to this family.
- Interleukin-17 Receptor (IL-17R) Family: The IL-17R family is distinct from other cytokine receptor families and shows little homology with them. Members of this family, including IL-17RA, IL-17RB, IL-17RC, IL-17RD, and IL-17RE, have conserved structural motifs such as an extracellular fibronectin III-like domain, a transmembrane domain, and a cytoplasmic SERIF domain. They specifically bind to IL-17 cytokines.
What happens during cytokinesis?
- Cytokinesis is the final phase of cell division, occurring after mitosis (division of the nucleus). The cytoplasm of the cell divides into two daughter cells, each with its own nucleus, during cytokinesis.
- In animal cells, a structure known as the contractile ring forms around the cell’s equator and contracts, drawing the plasma membrane inward and forming a cleavage furrow that ultimately divides the cell in half.
- In plant cells, cytokinesis differs slightly. In place of a contractile ring, a cell plate forms in the center of the cell and expands outward until it reaches the cell wall. The cell plate then fuses with the cell wall to form a new cell membrane that divides the daughter cells.
- Overall, cytokinesis guarantees that the genetic material is evenly divided across the two daughter cells, allowing for the continuation of normal cellular processes and growth.
Stages of cytokinesis
- Beginning and development of the cleavage furrow: The initial physical change noticed during cytokinesis is the emergence of the cleavage furrow on the cell surface. The furrow begins to deepen, encircling the cell until it entirely divides in half.
- Reduction and restriction: Likewise known as abscission. The contractile ring, which is composed of actin, myosin, and regulatory proteins, is responsible for cytokinesis in animal cells. This ring is produced beneath the surface of an animal cell during cell division. These rings can compress the cell in half by contracting and constricting it.
- Membrane Insertion: Concurrently, by the fusion of intracellular vesicles, a new membrane is generated and inserted into the cell membrane close to the contractile ring. Once cytoplasmic division occurs, the new membrane permits the cell to expand.
Proteins Involved in Cytokinesis
A large cortical complex called actin, myosin, septins, and other proteins organize these cytoskeletal components into the contractile ring. Many researchers believe that proteins found in the plasma membrane are responsible for the adhesion of the contractile ring to the cell membrane. It is speculated that the contractile ring is similar to other cortical-actin structures in the cell, such as focal adhesions and the accompanying stress fibers.
1. Actinomycin D
- Actin’s role in cytokinesis has been recognized for quite some time and is validated by a number of studies. (1) In virtually all cell types, actin is concentrated in a ring at the site of cell division. (2) Anti-actin medications, such as cytochalasin, impede cytokinesis. (3) Actin mutations in budding yeast generate massive multinucleated cells with cytokinesis and polarity problems. (4) In various species, mutations in genes encoding a range of actin-associated proteins impact cytokinesis.
2. Myosin
- Traditional theories have proposed that cytoplasmic myosin II, the non-muscle form of classical long-tailed myosin, drives cytokinesis.
- Phosphorylation of essential amino acids controls motile activity and multimer formation, making myosin activity a likely candidate for a main control point during cytokinesis.
- Myosin II in the cytoplasm is a hexamer made up of two pairs of polypeptides, the 200 kDa heavy chain (MHC), the 18 kDa essential light chain (ELC), and the 20 kDa regulatory light chain (RLC).
3. Septins
- Genetic information for septins, a class of proteins involved in interactions between the plasma membrane and the cortical cytoskeleton, is present in the genomes of most eukaryotic species (including cytokinesis).
- Most septin proteins have a C-terminal coiled-coil domain, and they all share the characteristic P-loop consensus sequences that are indicative of nucleotide-binding ability.
Additional proteins
Several other cytoskeletal proteins congregate in the vicinity of the cleavage furrow, but their function during cytokinesis is unknown. Proteins that cross-link or bundle actin filaments include a-actinin, filamin, and the recently discovered anillin; actin-membrane linkers include talin and ERM proteins; proteins that regulate F-actin assembly or organization include acidic calponin, which inhibits the ATPase activity of phosphorylated myosin; and transmembrane glycoproteins like CD43 play important roles in cell adhesion and signal transduction. It is important to remember that most of these proteins have not been shown to be contractile ring components using ultrastructural data.
Cytokinesis in Animal Cells
- There is not much difference in the process of cytokinesis between the two types of cell division (mitosis and meiosis). The division plane is established by cellular signals that instruct the cell where to divide.
- The cytokinetic furrow will form around this plane and then pinch off to separate the cells. Abscission is the last step of cytokinesis in animal cells.
- The plasma membranes undergo fission during abscission, and the actin-myosin contractile ring responsible for making the cytokinetic furrow is fully contracted.
- Researchers have yet to pinpoint what triggers cells to choose a certain dividing plane. It’s a complicated procedure that requires a lot of microtubules and cell messages to complete.
- After this location is established, the contractile ring of actin and myosin can be formed. The contraction of muscle cells is caused by the action of the motor proteins actin and myosin.
- Actin filaments abound inside muscle cells, and the protein myosin may bind them together with the help of some ATP. When cells divide in animals, they use the same mechanism.
- At the cell division plane, actin filaments coil into a ring. The myosin proteins subsequently begin to contract, contracting the actin filaments into a tighter ring.
- Finally, the ring was completely devoid of cytoplasm and organelles. The actin-myosin ring and the microtubules that it constricts are all that remain. In order for the cells to split, it is also necessary to divide what is called the midbody structure.
- This takes place as a result of the abscission procedure. There is a disruption in the protein chain and a closing of the plasma membrane. When the glue that separates cells is gone, the cells can finally split apart.
- In some multicellular organisms, cells stay close to one another and even maintain cytoplasmic connections (called gap junctions) with one another. These short connections can originate from fragments of the endoplasmic reticulum that become caught in the midbody, or they can develop independently at a later time.
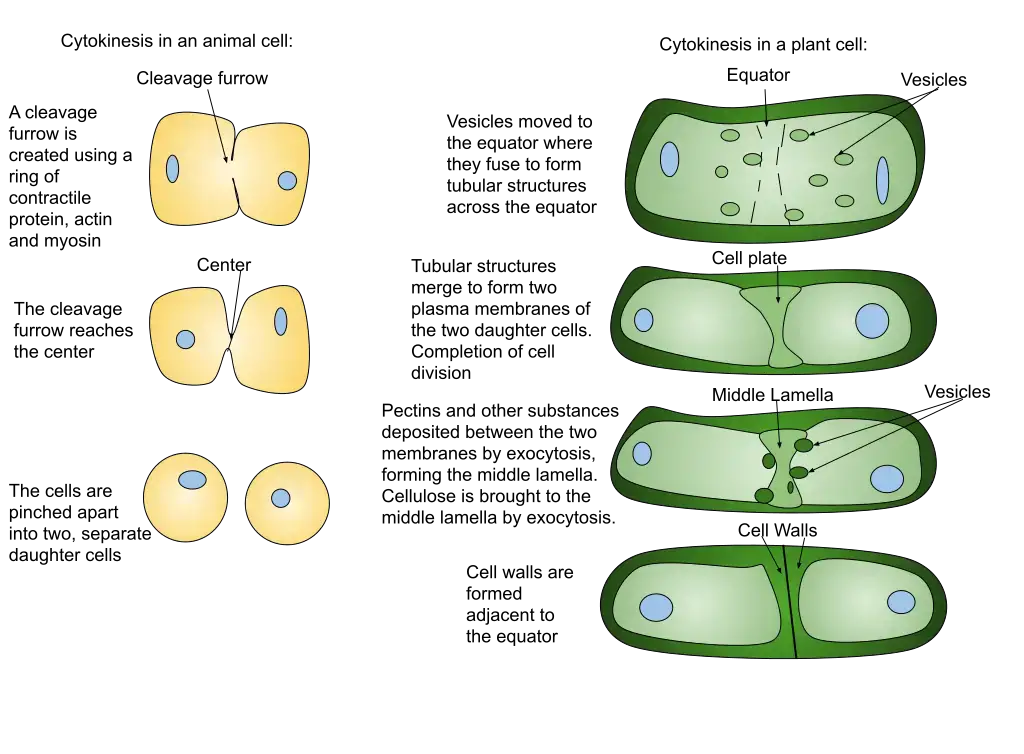
Cytokinesis in plant cells
- Although plant cells are more stiff than animal cells, they nonetheless undergo a cytokinesis process. The cell wall provides a supplementary protective covering for plants.
- When a plant cell divides, a new extracellular structure must be created to help shape the new cell. For this purpose, plants employ specialized structures called phragmoplasts, which are microtubule spindle organelles.
- Plegmoplasts deliver vesicles containing fresh cell plate material from the old cell wall. Matrixes composed of these elements, such as cellulose, are intricate and durable due to their interactions. When a cell is divided by a plate, the plasma membrane closes and the two halves are no longer attached.
- Similar to the centrosomes in animal cells, the phragmoplast regulates microtubule development and atrophy. The endoplasmic reticulum and the Golgi apparatus are responsible for producing and storing the materials needed to form the new cell plate.
- Next they’re forwarded to the phragmoplast, which constructs the cell plate from the center outward. The image above illustrates this point. The microtubules of the phragmoplast will extend from the center of the cell plate until they reach the plasm membrane.
- After severing this membrane, the cell wall will link to all the cells in its vicinity. Plasmodium sarcoma-associated endoplasmic reticulum (like gap junctions) forms between the cells when endoplasmic reticulum is trapped. The plasmodesmata in plants have been proposed as a means of intercellular communication.
Cytokines and pain
- Cytokines, which are small proteins involved in cell signaling, have been implicated in the modulation of pain. Specifically, pro-inflammatory cytokines are known to play a significant role in the development and maintenance of pathological pain. Three pro-inflammatory cytokines, namely IL-1β, IL-6, and TNF-α, have been extensively studied in relation to pain processes.
- IL-1β is primarily released by monocytes, macrophages, and various nonimmune cells during inflammation, injury, and infection. It has been found that IL-1β is expressed in nociceptive neurons in the dorsal root ganglia (DRG). Following peripheral nerve injury or trauma, the expression of IL-1β is enhanced in microglia and astrocytes in the central nervous system. IL-1β can induce hyperalgesia (increased sensitivity to pain) when administered through different routes. It has also been observed that IL-1β stimulates the production of substance P and prostaglandin E2 (PGE2) in neurons and glial cells. To counteract the effects of IL-1β, the specific IL-1 receptor antagonist (IL-1ra) competes with IL-1β for receptor binding without initiating cellular changes. Administration of IL-1ra and other anti-inflammatory cytokines has shown to prevent or reduce cytokine-mediated inflammatory hyperalgesia and nerve injury-induced mechanical allodynia.
- IL-6 is known to play a central role in the response of neurons to nerve injury. Suppression of the IL-6 receptor (IL-6R) through the use of anti-IL-6R antibodies has been shown to reduce regenerative effects. IL-6 is involved in the activation of microglia and astrocytes and regulates the expression of neuronal neuropeptides. Studies have demonstrated that IL-6 contributes to the development of neuropathic pain behaviors following peripheral nerve injury. In animal models, injecting IL-6 into the spinal cord induces tactile allodynia (painful response to normally non-painful stimuli) and thermal hyperalgesia (increased sensitivity to painful stimuli).
- TNF-α, also known as cachectin, is an inflammatory cytokine that plays a significant role in certain pain models. It acts through two cell surface receptors, TNFR1 and TNFR2, and regulates apoptotic pathways, inflammation through NF-kB activation, and stress-activated protein kinases (SAPKs). TNF-α receptors are expressed in both neurons and glia. TNF-α has been implicated in both inflammatory and neuropathic hyperalgesia. In animal models, injection of complete Freund’s adjuvant (CFA) leads to elevated levels of TNF-α, IL-1β, and nerve growth factor (NGF) in the inflamed area. Neutralizing TNF-α with anti-TNF-α antibodies delays the onset of inflammatory hyperalgesia and reduces IL-1β levels. Injection of TNF-α itself produces mechanical and thermal hyperalgesia. TNF binding protein (TNF-BP), an inhibitor of TNF-α, eliminates the hyperalgesia typically observed after administration of lipopolysaccharide (LPS). Co-administration of TNF-BP and an IL-1 antagonist has been shown to reduce mechanical allodynia in animal models of nerve injury.
- In addition to pro-inflammatory cytokines, chemokines are also involved in pain modulation. Chemokines are a group of cytokines that induce chemotaxis, the migration of leukocytes. Certain chemokines, such as MIP-1α, MCP-1, and GRO/KC, are up-regulated in neuroinflammatory conditions, demyelinating diseases, CNS trauma, and injured peripheral nerves. Receptors for these chemokines are expressed on DRG neurons, and they play key roles in neuropathic pain and neuroinflammatory conditions.
- On the other hand, anti-inflammatory cytokines have been shown to regulate the pro-inflammatory cytokine response and possess pain-reducing properties. IL-10, an anti-inflammatory cytokine, represses the expression of TNF-α, IL-6, and IL-1 by activated macrophages. Administration of IL-10 has been demonstrated to suppress the development of pain facilitation in animal models of peripheral neuritis, spinal cord injury, and peripheral nerve injury. Blocking spinal IL-10 has been found to prevent and reverse established neuropathic pain behaviors. Clinical studies have also indicated that low blood levels of IL-10 and IL-4, another anti-inflammatory cytokine, may be associated with chronic pain conditions.
- In summary, pro-inflammatory cytokines, such as IL-1β, IL-6, and TNF-α, have been implicated in pathological pain, including inflammatory hyperalgesia and neuropathic pain. Chemokines also play a role in pain modulation, particularly in neuroinflammatory and neuropathic pain conditions. Anti-inflammatory cytokines, including IL-10 and TGF-β, have pain-reducing properties and can counteract the effects of pro-inflammatory cytokines. Understanding the interplay between cytokines and pain can provide insights into the mechanisms underlying chronic pain conditions and contribute to the development of novel therapeutic approaches.
Chemokines
- Chemokines are a group of cytokines that play a crucial role in immune responses and inflammation by inducing chemotaxis, which is the directed migration of cells in response to chemical signals. These small proteins are released by various cells, particularly macrophages, in response to infection, injury, or other inflammatory stimuli.
- Once released, chemokines create a concentration gradient, with the highest concentration at the site where they were originally released. Local cells, equipped with chemokine receptors, are attracted to these proteins and migrate towards the source, where they are most needed for immune defense or tissue repair.
- Chemokine receptors are G protein-coupled receptors located on the surface of target cells. When a chemokine binds to its specific receptor, it triggers a signaling cascade inside the cell. This cascade involves the activation of phospholipase C, which leads to the release of calcium ions from intracellular stores. Elevated calcium levels then initiate a series of downstream effects, including cytoskeletal changes, pseudopod formation (projection of cell membrane), and enhanced cell adhesion through integrins.
- These cellular responses allow the attracted cells to move towards the site of chemokine release in a guided manner. By following the concentration gradient, the cells can effectively migrate to the areas where their functions are required, such as sites of infection or tissue damage.
- One well-known example of a chemokine is Interleukin-8 (IL-8). IL-8 is produced by macrophages during the acute phase response to infection. It acts as a potent chemoattractant for neutrophils, attracting them to the site of infection to help eliminate pathogens and initiate the immune response.
- Overall, chemokines play a crucial role in immune cell recruitment and coordination during inflammatory processes. By guiding the migration of cells to the appropriate sites, chemokines contribute to the proper functioning of the immune system and the resolution of inflammation.
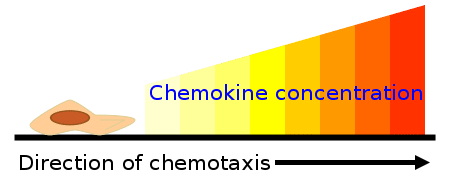
Chemokine actions
The actions of chemokines involve a series of steps and mechanisms that facilitate their effects within tissues. Let’s explore the general mechanism of chemokine actions:
- Induction of Chemokine Production: Pro-inflammatory chemokines are induced or upregulated in response to infection or trauma. This induction can be triggered by other pro-inflammatory cytokines such as TNF-α and IFN-γ or microbial products like lipopolysaccharide. In contrast, homeostatic chemokines are constitutively produced at basal levels without a specific stimulus.
- Chemokine Secretion and Activation: Once produced, chemokines are secreted by cells into the local environment. They can bind to glycosaminoglycans (GAGs) on the cell surface or extracellular matrix, creating a chemokine gradient.
- Chemokine Receptor Binding: Cells within the vicinity express chemokine receptors, which are G protein-coupled receptors. When chemokines bind to their specific receptors on target cells, it triggers a signaling cascade.
- Signal Transduction: Chemokine binding to its receptor activates intracellular signaling pathways. This often involves the activation of phospholipase C, which leads to the release of calcium ions (Ca2+) from intracellular stores.
- Downstream Effects: The release of calcium ions initiates a range of downstream effects within the target cells. These effects include cytoskeletal changes, pseudopod formation, and enhanced cell adhesion through integrins.
- Cell Migration: The cytoskeletal changes and enhanced adhesion allow cells to undergo chemotaxis, a process in which cells migrate toward the source of the chemokine gradient. The chemokine concentration is highest at the source, where the chemokines were originally released and where the attracted cells are most needed.
By following this mechanism, chemokines play various roles within tissues. Pro-inflammatory chemokines recruit immune cells, such as neutrophils and monocytes, to the site of infection or injury. Homeostatic chemokines attract cells required for angiogenesis and contribute to the growth of new blood vessels. Moreover, chemokines facilitate immune surveillance by allowing T cells and dendritic cells to migrate and circulate through secondary lymphoid organs in search of potential pathogens. They also contribute to the development of lymphoid organs and the positioning of cells within lymphoid tissues.
It’s important to note that some chemokines can have both pro-inflammatory and homeostatic roles, depending on the context and the specific chemokine-receptor interactions involved.
Interferons
Interferons are a group of cytokines that are released by host cells in response to various pathogens such as viruses, bacteria, and tumor cells. They play a crucial role in the immune response against these pathogens. Interferons are categorized into three main groups based on their complementary receptors: type I (including interferon-α and interferon-β), type II (interferon-γ), and type III (interferon λ). While each type has unique characteristics, type III interferons share similar actions with type I and type II interferons.
- Type I Interferons (Interferon-α and Interferon-β): Type I interferons are produced by a variety of cells, including immune cells such as leukocytes, fibroblasts, and epithelial cells. They are released in response to viral infections and act as potent antiviral agents. Type I interferons inhibit viral replication within infected cells and also play a role in preventing the spread of the virus to neighboring cells. They enhance the immune response by activating natural killer (NK) cells, promoting antigen presentation, and increasing the expression of major histocompatibility complex (MHC) molecules on the cell surface.
- Type II Interferon (Interferon-γ): Interferon-γ is primarily produced by activated T cells and natural killer (NK) cells. It has diverse immunomodulatory functions. Interferon-γ plays a critical role in activating macrophages, enhancing their antimicrobial activity, and promoting antigen presentation. It also stimulates the production of antibodies by B cells and enhances the cytotoxic activity of NK cells. Additionally, interferon-γ plays a role in regulating the adaptive immune response and promoting the differentiation of T helper cells.
- Type III Interferons (Interferon λ): Type III interferons, also known as interferon λ, have similar actions to type I and type II interferons. They are produced by a variety of cells, including epithelial cells and certain immune cells. Like type I interferons, type III interferons have antiviral effects and can inhibit viral replication within infected cells. They also contribute to the activation of immune cells and the enhancement of the immune response against pathogens.
Overall, interferons are essential components of the immune response. They are released by host cells in response to pathogens and tumor cells, and their actions help in limiting infection, promoting immune activation, and enhancing the overall antiviral and antimicrobial defenses of the body.
Interferon actions
Interferons, including type I and type II interferons, have specific mechanisms and steps by which they exert their actions in the immune response:
- Type I Interferons (Interferon-α and Interferon-β):
- a. Production: Various cells, such as fibroblasts, endothelial cells, and macrophages, can produce type I interferons in response to viral infections or microbial products.
- b. Binding and Signaling: Once secreted, type I interferons bind to interferon receptors on the same infected cell (autocrine signaling) and neighboring cells (paracrine signaling).
- c. Gene Expression Changes: Binding of type I interferons to their receptors triggers intracellular signaling pathways, leading to changes in gene expression within the cell.
- d. Antiviral Effects: The changes in gene expression induced by type I interferons result in the destruction of viral mRNA and the inhibition of host and viral protein translation. This helps limit viral replication within infected cells.
- e. Upregulation of NK Cell Ligands and MHC I: Type I interferons also upregulate the expression of natural killer (NK) cell ligands and major histocompatibility complex class I (MHC I) molecules on the surface of infected cells. This makes virus-infected cells more visible to NK cells and cytotoxic T cells, enhancing their ability to detect and eliminate the infected cells.
- Type II Interferon (Interferon-γ):
- a. Production: Interferon-γ, a type II interferon, is primarily produced by NK cells, cytotoxic T cells, and Th1 cells in response to the cytokines IL-12 and IL-18.
- b. Activation of Macrophages: Interferon-γ activates macrophages by binding to specific receptors on their surface.
- c. Enhanced Pathogen Killing: Activation of macrophages by interferon-γ enhances their ability to kill pathogens. It promotes pinocytosis (fluid uptake) and lysosome function within macrophages, aiding in the destruction of engulfed pathogens.
- d. Upregulation of MHC II: Type II interferons also upregulate the expression of major histocompatibility complex class II (MHC II) molecules on the surface of antigen-presenting cells. This facilitates effective antigen presentation and phagocytosis by macrophages and other antigen-presenting cells.
It is important to note that there is some overlap between the actions of type I and type II interferons, as both are involved in antiviral and anti-tumor responses. Together, these interferons play critical roles in orchestrating immune responses against viral infections and tumors, promoting the elimination of infected or malignant cells and enhancing overall immune defense mechanisms.
Interleukins
Interleukins are a diverse group of cytokines produced by T-lymphocytes, monocytes, and macrophages. They play crucial roles in immune regulation and communication between cells. Here are some key functions of interleukins:
- Promotion of B and T Lymphocyte Production and Differentiation:
- IL-1α/β, IL-4, IL-7, and IL-21 are interleukins that promote the production and differentiation of B and T lymphocytes.
- These interleukins stimulate the proliferation and maturation of lymphocytes, contributing to the development of a robust immune response.
- Activation of Neutrophils and Natural Killer Cells:
- Interleukins such as IL-2, IL-8, and IL-12 play a role in activating neutrophils and natural killer (NK) cells.
- Neutrophils are important immune cells involved in fighting bacterial infections, and NK cells are responsible for recognizing and destroying infected or cancerous cells.
- IL-2, IL-8, and IL-12 enhance the activity and cytotoxic capabilities of these immune cells, enabling them to effectively eliminate pathogens and abnormal cells.
- Production of Detectable Signs:
- Interleukin-6 (IL-6) is an example of an interleukin that produces detectable signs within the body.
- IL-6 is involved in initiating the acute phase response, a series of systemic changes that occur during infection or inflammation.
- IL-6 increases body temperature, leading to fever, which can inhibit microbial growth and enhance immune function.
- Additionally, IL-6 stimulates the liver to produce acute phase proteins such as C-reactive protein (CRP), which is associated with inflammation and can serve as a marker for various diseases.
- Promotion of Vascular Permeability:
- Certain interleukins can promote vascular permeability, allowing for increased movement of immune cells and molecules.
- This increased permeability leads to swelling at the site of infection or inflammation, facilitating the recruitment of immune cells to the affected area.
- The faster recruitment of immune cells helps in the initiation of an immune response, as these cells can more effectively combat pathogens and provide localized immune defense.
Overall, interleukins play critical roles in immune regulation, cell communication, and the orchestration of immune responses. Their diverse functions contribute to the effectiveness of the immune system in combating infections, regulating inflammation, and maintaining overall immune homeostasis.
Examples of Important Cytokines and Their Roles
Cytokines are important signaling molecules that regulate various immune and inflammatory processes in the body. Here are some examples of important cytokines and their roles:
- IL-1α/β: Produced by macrophages, IL-1 induces fever, activates T cells and macrophages, and plays a role in inflammation.
- IL-2: Secreted by T helper 1 cells, IL-2 promotes the growth of T cells, B cells, and natural killer (NK) cells, thereby enhancing immune responses.
- IL-4: Produced by T helper 2 cells, IL-4 activates B cells, leading to their growth and differentiation. It also induces the differentiation of CD4 T cells into T helper 2 cells, which are involved in allergic and humoral immune responses.
- IL-6: Released by macrophages, IL-6 contributes to fever, stimulates the production of acute phase proteins, activates lymphocytes, and promotes antibody production.
- IL-8: Produced by macrophages, IL-8 acts as a chemoattractant for neutrophils and activates them, playing a crucial role in recruiting neutrophils to sites of infection or inflammation.
- IL-10: Secreted by T helper 2 cells and macrophages, IL-10 inhibits immune function and helps regulate inflammatory responses.
- IL-12: Produced by macrophages, IL-12 activates NK cells and promotes the differentiation of CD4 T cells into T helper 1 cells, which are involved in cell-mediated immune responses.
- IL-17: Released by T helper 17 cells, IL-17 induces an inflammatory response and recruits neutrophils to the site of inflammation.
- Interferon-α and Interferon-β: Produced by various immune cells such as T cells, B cells, and monocytes/macrophages, interferons inhibit viral replication and play a role in antiviral immune responses.
- Interferon-γ: Secreted by T helper 1 cells and NK cells, interferon-γ activates macrophages and NK cells, inhibits viral replication, and enhances the expression of major histocompatibility complex (MHC) molecules.
- TNF-α/β: Produced by T helper cells and macrophages, TNF (tumor necrosis factor) activates macrophages, induces nitric oxide production, promotes inflammation, and plays a role in fever and shock responses.
These cytokines demonstrate the diverse functions they serve in immune regulation, inflammation, and host defense mechanisms.
Function of Cytokines
Cytokines play diverse and important roles in various physiological processes, particularly in immune responses and inflammation. Here are some key functions of cytokines:
- Inflammation Regulation: Cytokines such as interleukin-6 (IL-6) and tumor necrosis factor-alpha (TNF-α) are involved in promoting and upregulating inflammatory reactions. They contribute to the recruitment and activation of immune cells, vasodilation, and increased vascular permeability at the site of inflammation.
- Immune Response Modulation: Cytokines act as mediators and regulators of both innate and adaptive immune responses. They are produced by different immune cells, including helper T cells and macrophages. Cytokines of the innate immune system, produced mainly by mononuclear phagocytes, help initiate and coordinate the early immune response to infectious agents. Cytokines of the adaptive immune system, produced mainly by T lymphocytes in response to specific antigen recognition, regulate the activation and differentiation of immune cells for a targeted immune response.
- Nerve Regeneration and Neuronal Activity: Cytokines have been implicated in nerve regeneration and neuronal activity modulation. In the context of peripheral nerve injury, cytokines produced by macrophages, mast cells, and endothelial cells at the injury site play a role in facilitating nerve regeneration. Additionally, cytokines can modulate the activity of neurons in both the central and peripheral nervous systems, influencing synaptic plasticity, neuroinflammation, and neuronal survival.
- Chemotaxis and Leukocyte Activation: Chemokines, a subset of cytokines, function as signaling molecules for leukocyte activation and migration. They play a crucial role in directing immune cell movement and recruitment to sites of inflammation, infection, and tissue damage. Chemokines are also involved in demyelinating and neuroinflammatory diseases, where they contribute to the recruitment and activation of immune cells in affected tissues.
- Hematopoiesis Stimulation: Certain cytokines play a role in hematopoiesis, the process of blood cell formation. They are produced by bone marrow stromal cells, leukocytes, and other cells, and they stimulate the growth and differentiation of immature leukocytes, ensuring a balanced and functional immune cell population.
Overall, cytokines function as crucial mediators and regulators of immune responses, inflammation, nerve regeneration, and hematopoiesis. They play essential roles in maintaining immune homeostasis and coordinating the body’s response to pathogens, injury, and other challenges.
FAQ
What is cytokinesis?
Cytokinesis is the process by which the cytoplasm of a eukaryotic cell divides into two daughter cells during cell division.
What triggers cytokinesis?
Cytokinesis is triggered by the completion of nuclear division or mitosis, which ensures that each daughter cell receives a complete set of chromosomes.
How does cytokinesis differ between animal and plant cells?
In animal cells, cytokinesis is achieved through the formation of a contractile ring of actin and myosin filaments, which pinches the cell membrane inward to form a cleavage furrow. In plant cells, cytokinesis involves the formation of a cell plate, which grows outward from the center of the cell to divide it in two.
What is the role of microtubules in cytokinesis?
Microtubules are involved in the organization and movement of the spindle apparatus during mitosis, which is necessary for the proper segregation of chromosomes. During cytokinesis, microtubules may also play a role in directing the positioning of the contractile ring or cell plate.
What are the stages of cytokinesis?
Cytokinesis can be divided into four stages: initiation, ingression, furrow ingression, and completion.
What happens during the initiation stage of cytokinesis?
During the initiation stage, a signal is sent to the cell membrane to begin the process of cytokinesis. This signal is often triggered by the completion of mitosis or the formation of a specific protein complex.
What happens during the ingression stage of cytokinesis?
During the ingression stage, a contractile ring or cell plate begins to form, which will eventually divide the cell in two. This process involves the assembly of actin and myosin filaments or the fusion of vesicles to form the cell plate.
What happens during the furrow ingression stage of cytokinesis?
During the furrow ingression stage, the contractile ring or cell plate continues to grow inward, creating a deepening furrow that eventually separates the two daughter cells.
What happens during the completion stage of cytokinesis?
During the completion stage, the contractile ring or cell plate reaches its maximum depth, dividing the cell into two daughter cells. The cell membrane may then undergo repair or remodeling to ensure the proper functioning of both daughter cells.
What factors can affect cytokinesis?
Cytokinesis can be affected by a variety of factors, including changes in cell size or shape, alterations in the cytoskeleton or membrane composition, or the presence of external cues or signals. Dysfunction or abnormalities in cytokinesis can lead to a range of developmental or disease-related conditions.
References
- Srivastava, V., & Robinson, D. N. (2013). Cytokinesis. Encyclopedia of Biological Chemistry, 622–626. doi:10.1016/b978-0-12-378630-2.00499-0
- D’Avino PP, Giansanti MG, Petronczki M. Cytokinesis in animal cells. Cold Spring Harb Perspect Biol. 2015 Feb 13;7(4):a015834. doi: 10.1101/cshperspect.a015834. PMID: 25680833; PMCID: PMC4382743.
- Alberts B, Johnson A, Lewis J, et al. Molecular Biology of the Cell. 4th edition. New York: Garland Science; 2002. Cytokinesis. Available from: https://www.ncbi.nlm.nih.gov/books/NBK26831/
- https://www.biologyonline.com/dictionary/cytokinesis
- https://ib.bioninja.com.au/standard-level/topic-1-cell-biology/16-cell-division/cytokinesis.html
- https://courses.lumenlearning.com/wm-biology1/chapter/reading-cytokenesis/
- https://biologydictionary.net/cytokinesis/
- https://www.thoughtco.com/cytokinesis-in-a-cell-cycle-373541