What is Chloroplast?
- A chloroplast, as a distinct and essential organelle within plant and algal cells, plays a pivotal role in the process of photosynthesis. This membrane-bound plastid possesses the photosynthetic pigment chlorophyll, which is the principal agent responsible for capturing solar energy.
- Through a complex sequence of biochemical reactions, chloroplasts transform this absorbed sunlight into chemical energy stored in molecules like ATP (Adenosine Triphosphate) and NADPH (Nicotinamide Adenine Dinucleotide Phosphate), liberating oxygen from water molecules in the process. Subsequently, these energy-rich molecules are employed in the synthesis of organic compounds from carbon dioxide, a vital process referred to as the Calvin cycle.
- The structural characteristics of a chloroplast are marked by the presence of two distinct membranes and a considerable concentration of chlorophyll. This unique configuration enables chloroplasts to perform photosynthesis efficiently. In contrast, other plastids, such as leucoplasts and chromoplasts, exhibit minimal chlorophyll content and do not engage in photosynthesis.
- Chloroplasts are dynamic entities within plant cells, exhibiting mobility and even undergoing division to proliferate. Environmental factors, including light color and intensity, significantly influence their behavior. Like mitochondria, chloroplasts possess their own DNA, believed to be inherited from their ancestral cyanobacterium, which was engulfed by an early eukaryotic cell. Intriguingly, chloroplasts are not synthesized de novo within plant cells but are instead inherited by daughter cells during the process of cell division.
- A noteworthy aspect of chloroplasts is their remarkable evolutionary history. With a single exception (the amoeboid Paulinella chromatophora), all chloroplasts can be traced back to a single endosymbiotic event involving the engulfment of a cyanobacterium by a eukaryotic host cell. This event has led to the widespread distribution of chloroplasts among an extensive array of organisms, even those not directly related to each other, a consequence of numerous secondary and tertiary endosymbiotic events.
- The term “chloroplast” derives from the Greek words “chloros” (meaning green) and “plastes” (meaning “the one who forms”). Its initial definitive description was provided by Hugo von Mohl in 1837, who identified chloroplasts as discrete entities within plant cells, referred to as “Chlorophyllkörnen” or “grains of chlorophyll.” Subsequently, in 1883, Andreas Franz Wilhelm Schimper introduced the term “chloroplastids” (Chloroplastiden), which was further refined by Eduard Strasburger in 1884 to “chloroplasts” (Chloroplasten), the designation that persists in modern scientific literature.
- In summary, chloroplasts are essential cellular organelles, pivotal to the process of photosynthesis, and possess a rich and intricate history, tracing their origins to a single endosymbiotic event. Their structural and functional attributes, combined with their dynamic behavior and genetic inheritance, make them a subject of profound interest in the study of plant biology and evolution.
Definition of Chloroplasts
Chloroplasts are membrane-bound organelles within plant and algal cells responsible for photosynthesis, converting sunlight into energy while releasing oxygen from water.
Location of chloroplasts
The precise location of chloroplasts within plant cells is a crucial aspect of their function in photosynthesis. Chloroplasts are primarily found in the green components of plants and play a pivotal role in conferring the characteristic green coloration associated with photosynthetic elements. The distribution and concentration of chloroplasts within plant cells are as follows:
- Cell Types Containing Chloroplasts:
- Chloroplasts are typically present in certain cell types within multicellular plants. The primary cell types containing chloroplasts are parenchyma cells.
- In some instances, chloroplasts may also be found in collenchyma tissues.
- Plant cells containing chloroplasts are referred to as achromatoma cells.
- Chloroplast Abundance:
- The number of chloroplasts within a typical plant cell can vary but usually ranges between 10 and 100 chloroplasts per cell.
- Stem Chloroplasts in Certain Plants:
- In specific plant species, such as cacti, chloroplasts can be located in the stems.
- However, in the majority of plants, chloroplasts are primarily concentrated in the leaves, which are the main sites of photosynthesis.
- Distribution in Leaf Tissue:
- In leaves, chloroplasts are highly concentrated, with each square millimeter of leaf tissue potentially containing up to half a million chloroplasts.
- Chloroplasts in leaves are typically found in the mesophyll layer, which is responsible for the majority of photosynthetic activity.
- Additionally, chloroplasts can be found in the guard cells that form stomata, specialized structures that regulate gas exchange in leaves.
- Mesophyll Cells:
- Within the mesophyll layer of leaves, palisade mesophyll cells are known to contain a substantial number of chloroplasts. A single palisade mesophyll cell can have anywhere from 30 to 70 chloroplasts, optimizing their capacity for photosynthesis.
- Guard cells, which are responsible for controlling the opening and closing of stomata, contain fewer chloroplasts, typically numbering between 8 and 15 per cell, alongside a reduced amount of chlorophyll.
- Bundle Sheath Cells in C4 Plants:
- In certain plant species, known as C4 plants, chloroplasts can also be observed within the bundle sheath cells of leaves.
- These bundle sheath cells are responsible for conducting the Calvin cycle, a critical step in photosynthesis.
- In C4 plants, chloroplasts in bundle sheath cells enable more efficient carbon fixation.
- Epidermal Leaves:
- Chloroplasts are generally absent from the epidermal cells of leaves, where their primary function is to provide protection and serve as the outermost layer of plant tissue.
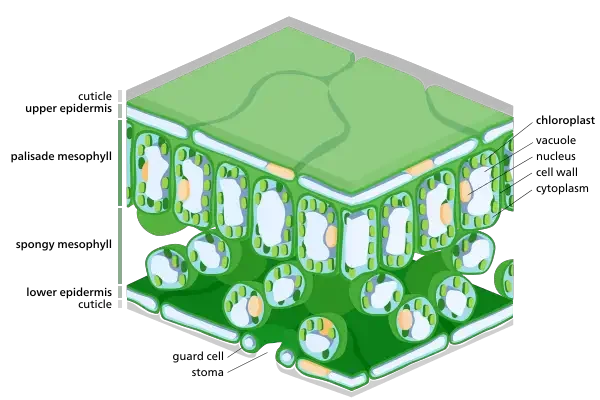
Characteristics of chloroplasts
Chloroplasts exhibit distinct characteristics that set them apart within the realm of plastids, making them essential players in the realm of photosynthesis and cellular metabolism.
- Green Pigments and Photosynthesis: One of the defining features of chloroplasts is their vibrant green coloration, attributed to the abundance of chlorophyll pigments, primarily chlorophyll A and chlorophyll B. These pigments are crucial components that capture energy from light during photosynthesis, initiating the conversion of solar energy into chemical energy.
- Supplementary Pigments: In addition to chlorophyll, chloroplasts house other pigments, such as carotenoids. These serve as accessory pigments by capturing sunlight and channeling the absorbed energy to chlorophyll molecules. This cooperative effort enhances the efficiency of light absorption and energy conversion in chloroplasts.
- Tissue Distribution: Chloroplasts are primarily found in plant tissues, where they play a pivotal role in the photosynthetic process. While they are present in all green tissues, they exhibit a higher concentration in parenchyma cells located within the mesophyll of leaves. This concentration optimizes their exposure to light and facilitates photosynthesis.
- Morphological Features: Chloroplasts exhibit characteristic morphological features. They typically range from 1 to 2 micrometers in thickness and about 5 to 7 micrometers in diameter. These organelles are enveloped by the chloroplast envelope, which consists of two membranes separated by an intermembrane space.
- Thylakoid Structure: A distinctive feature of chloroplasts is the presence of internal thylakoid membranes, which are extensively folded and form closed disks referred to as thylakoids. In many higher plants, these thylakoids are organized into tight stacks known as grana (singular: granum). These grana are interconnected by stromal lamellae, extensions that traverse the stroma and link one granum to another.
- Stroma and Lumen: Within the chloroplast, the thylakoid stroma surrounds an aqueous central region called the thylakoid lumen. The stroma is a matrix containing various dissolved proteins, starch grains, and copies of the chloroplast’s genome. It serves as the site for several essential metabolic reactions, including the Calvin cycle, which converts carbon dioxide into organic compounds.
Chloroplast Morphology
Chloroplast morphology exhibits a remarkable diversity across plant species, showcasing variations in size, shape, and number. These characteristics play a crucial role in the adaptability of chloroplasts to the specific requirements of different plant cells and environments. The key aspects of chloroplast morphology are as follows:
- Size and Shape Variability: Chloroplasts demonstrate significant variation in both size and shape among different plant species. In higher plants, they typically appear as biconvex or planoconvex structures. However, their morphology can vary widely, encompassing filamentous, saucer-like, ovoid, discoid, spheroid, star-like, girdle-shaped, spiral ribbon-like, reticulate, or cup-shaped forms.
- Dimensional Measurements: Chloroplasts typically possess a diameter ranging from 5 to 10 micrometers (µm) and a thickness of about 2 to 3 µm. These measurements are not uniform and can be influenced by factors such as plant species, cell type, and environmental conditions.
- Size Disparities in Different Plants: The size of chloroplasts can vary between plant cells, depending on factors such as polyploidy and light conditions. In general, chloroplasts in cells of polyploid and shade-adapted plants tend to be larger compared to those in cells of diploid and sun-adapted plants.
- Variation in Chloroplast Number: The number of chloroplasts within plant cells can differ not only between species but also within individual cells. This variability is influenced by the physiological state of the cell. For example, Chlamydomonas, a unicellular alga, typically possesses a single chloroplast, while Spirogyra, a filamentous green alga, can have between 1 and 16 chloroplasts per cell.
- Chloroplast Density: The density of chloroplasts in plant tissues can be astonishingly high. For instance, in the leaves of Ricinus communis, it is estimated that there are approximately 400,000 chloroplasts per square millimeter of surface area. This high density is a reflection of the crucial role of chloroplasts in photosynthesis and energy production.
- Chloroplast Division and Degeneration: Chloroplast number within a cell is not static but can change dynamically. Chloroplasts can divide to increase their numbers when they are insufficient to meet the metabolic demands of the cell. Conversely, when chloroplasts become excessively abundant, degeneration processes may occur to maintain an optimal balance.
Chloroplast Structure
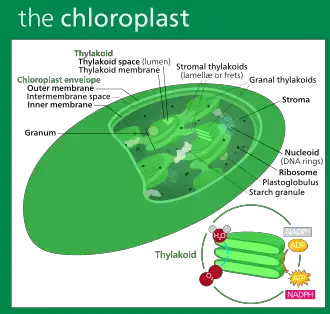
The chloroplast, a vital organelle responsible for photosynthesis in plant cells, exhibits a complex and highly organized structure composed of three main components:
- Envelope:
- The chloroplast is enveloped by a double membrane structure, consisting of an outer and inner lipid bilayer.
- The space between these two layers is known as the intermembrane space, facilitating the exchange of molecules between the chloroplast and the cytosol.
- Notably, the envelope membrane contains a small quantity of carotenoid pigments, imparting a yellowish color to the isolated membrane. However, chlorophyll pigments and cytochromes are notably absent.
- The envelope membrane accounts for only a minor fraction, approximately 1 to 2 percent, of the total protein content of the chloroplast.
- In some specific cases, such as in glaucophyte algal chloroplasts, a peptidoglycan layer is present between the chloroplast membrane layers.
- Stroma:
- The central and predominant portion of the chloroplast is occupied by a matrix called the stroma, surrounding the thylakoid membranes.
- The stroma is a colorless, dense, and granular ground substance, containing a diverse array of components.
- More than 50% of the stroma consists of proteins, essential for various metabolic processes within the chloroplast.
- Additionally, the stroma houses 70 ribosomes, circular DNA molecules, mRNA and tRNA molecules, water, minerals, and enzymes.
- It serves as the site for the dark reactions of photosynthesis (Calvin cycle), as well as the synthesis of fatty acids, sugars, starch, and proteins.
- The stroma also contains structural proteins synthesized within the chloroplast through the action of its ribosomes and DNA.
- Thylakoids:
- The third integral component of the chloroplast is the thylakoid system, a highly folded internal membrane characterized by the presence of closed disc-shaped sacs known as thylakoid membranes.
- The stroma and the outer surface of the thylakoid membranes are in close contact.
- The inner surface of the thylakoid membranes encloses an intrathylakoid space, creating a third compartment within the chloroplast.
- Thylakoids can be stacked one upon another to form structures known as grana (singular: granum). Alternatively, they may remain unstacked, forming intergranal or stromal thylakoids, interconnected by membranous tubules called intergrana or stroma lamellae.
- Each granum consists of multiple disc-shaped thylakoid sacs stacked atop one another.
- The thylakoid membranes house all the enzymatic components essential for photosynthesis, including chlorophyll and other pigments.
- Light reactions of photosynthesis occur within the thylakoid membranes, where the interaction between chlorophyll and other components takes place.
- Thylakoids can fuse and rearrange to form various structures, contributing to the organization of the chloroplast’s internal membranes.
Lastly, it is noteworthy that chloroplasts, akin to mitochondria, are considered semi-autonomous organelles. They possess their own DNA and machinery for synthesizing certain essential proteins, while other proteins are dependent on nuclear DNA and cytoplasmic ribosomes. This semi-autonomous nature is a defining characteristic of chloroplasts, making them a subject of significant interest in the study of cell biology and organelle function.
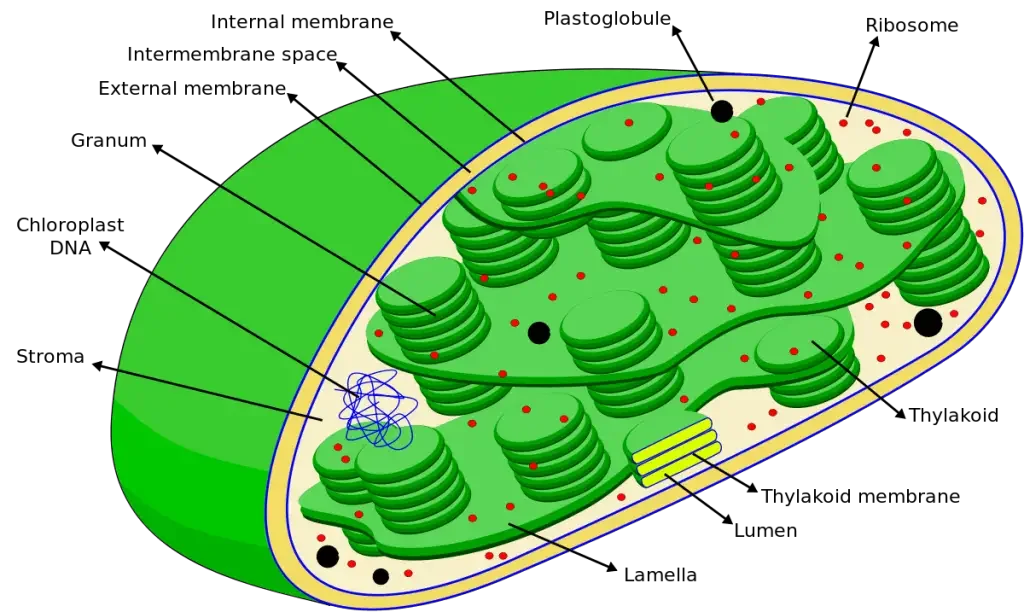
Types of pigments
Pigments play a pivotal role in the photosynthetic machinery of plants and photosynthetic microorganisms. These pigments, found in various organelles and cell structures, are responsible for absorbing light energy, which is subsequently utilized in the synthesis of food molecules. Here, we examine several types of pigments commonly encountered in the realm of photosynthesis:
- Chlorophyll: Chlorophyll is the primary pigment responsible for the characteristic green coloration of plants. It is primarily situated within the thylakoid membranes of chloroplasts, where photosynthesis takes place. Chlorophyll is predominantly composed of two main types:
- Chlorophyll a: This pigment constitutes approximately 75% of the chlorophyll in chloroplasts and is fundamental to the photosynthetic process.
- Chlorophyll b: Comprising roughly 25% of chlorophyll, chlorophyll b plays a supporting role in capturing light energy. Both chlorophyll a and b are critical for efficient light absorption during photosynthesis.
- Carotenoids: Carotenoids are a group of pigments that coexist with chlorophylls within the thylakoid membranes. They are responsible for imparting yellow and orange hues to many plant structures, such as fruits and leaves. Carotenoids are associated with vitamin A and serve essential functions, including:
- Light Absorption: Carotenoids can absorb specific wavelengths of light that chlorophylls cannot, thereby broadening the spectrum of light captured for photosynthesis.
- Photoprotection: Carotenoids are involved in photoprotective mechanisms, helping to dissipate excess light energy and prevent damage to photosystems.
- Xanthophylls: Xanthophylls represent a subgroup of carotenoids and are particularly prevalent in brown and green algae. These pigments contribute to the diverse coloration observed in these photosynthetic organisms.
- Phycobilins: Phycobilins are specialized pigments found exclusively in certain photosynthetic organisms, notably red algae and Cyanobacteria. Two prominent members of the phycobilin family are:
- Phycoerythrin: Phycoerythrin imparts the characteristic red coloration to red algae, making them easily distinguishable. It efficiently absorbs light in the blue and green regions of the spectrum.
- Phycocyanin: Phycocyanin is responsible for the blue-green coloration of Cyanobacteria. Like phycoerythrin, it plays a vital role in light absorption during photosynthesis in these organisms.
Functions of Chloroplast
Chloroplasts, as the key organelles in photosynthetic eukaryotic cells, fulfill several crucial functions that are integral to the process of photosynthesis and its broader ecological implications:
- Photosynthesis: The primary and foundational function of chloroplasts is photosynthesis. They serve as the sites where light energy from the sun is absorbed and converted into chemical energy. This process involves the synthesis of organic molecules, primarily glucose, from carbon dioxide and water using light energy. Chloroplasts contain the pigments, including chlorophyll, necessary for capturing and harnessing this light energy.
- Conversion of Light Energy: Chloroplasts play a pivotal role in the conversion of light energy into chemical energy in the form of ATP (Adenosine Triphosphate) and NADH (Nicotinamide Adenine Dinucleotide). This energy is indispensable for various cellular processes, including growth and maintenance.
- Oxygen Production: During photosynthesis, chloroplasts engage in the photolysis of water molecules, releasing oxygen as a byproduct. This process is essential for the respiration of aerobic organisms, contributing to the oxygen content in the biosphere.
- Oxygen and Carbon Dioxide Balance: Chloroplasts actively participate in maintaining the delicate balance of oxygen and carbon dioxide in the biosphere. They produce oxygen while concurrently absorbing carbon dioxide during photosynthesis, playing a vital role in regulating atmospheric gases.
- ATP Production: Chloroplasts generate ATP through the process of photophosphorylation. ATP serves as a universal energy currency in cells and is utilized for numerous cellular activities.
- Mitigation of Global Warming: By absorbing carbon dioxide during photosynthesis, chloroplasts contribute to reducing the concentration of this greenhouse gas in the atmosphere. This action plays a role in mitigating global warming and its associated environmental impacts.
- Storage of Starch: In certain algal forms, chloroplasts house proteinaceous bodies called pyrenoids where starch is stored. This storage mechanism ensures a readily available energy source for the organism.
- Fatty Acid Synthesis: In specific cases, such as in spinach, chloroplasts are involved in the synthesis of fatty acids. This process is crucial for the production of lipids and other cellular components.
- Sustaining Greenery: Chloroplasts are the cellular components responsible for the natural green coloration of plants. This greenery is not only aesthetically pleasing but also indicative of active photosynthetic processes within the plant.
- Carbon and Sugar Synthesis: During the Calvin cycle, also known as the dark reaction, chloroplasts utilize carbon dioxide from the air to synthesize carbon compounds, including sugars. This step in photosynthesis is critical for the production of organic molecules that serve as the foundation of the food web.
Movement of Chloroplast
The movement of chloroplasts within plant and algal cells is a dynamic and finely regulated process that allows these organelles to optimize their exposure to light. This movement, often referred to as chloroplast positioning or chloroplast motility, is a response to changes in light intensity and plays a crucial role in protecting chloroplasts from excessive exposure to intense sunlight, which can lead to photooxidative damage. Here are the key aspects of chloroplast movement:
- Optimal Light Absorption:
- Chloroplasts are equipped with the ability to reposition themselves within the cell to maximize light absorption under varying light conditions.
- In low light conditions, chloroplasts spread out as a sheet, increasing their surface area exposed to light to capture as much energy as possible.
- Shelter from Intense Sunlight:
- When exposed to intense sunlight, chloroplasts exhibit a photoreceptor-mediated response to protect themselves from potential damage caused by excessive light.
- In this scenario, chloroplasts may align vertically along the cell wall or turn their sides to minimize their exposure to direct sunlight. This reorientation provides a form of shelter and minimizes the risk of photooxidative damage.
- Diversity of Chloroplasts:
- The ability of chloroplasts to disperse and reposition themselves in response to light is a likely factor contributing to the evolution of plants possessing a variety of smaller chloroplasts rather than a few large ones.
- This diversity in chloroplast size and distribution enhances their adaptability to changing light conditions.
- Controlled Response Mechanism:
- Chloroplast movement is considered one of the most tightly regulated stimulus-response mechanisms observed in plants.
- It allows chloroplasts to efficiently utilize available light resources while avoiding potential harm from excessive exposure.
- Influence of Light Wavelengths:
- Chloroplast movement is influenced by different wavelengths of light. In some plants, such as arid-adapted species, movement is controlled by phototropins, blue light photoreceptors responsible for phototropism.
- In certain algae, mosses, flowers, and ferns, red light, in addition to blue light, can influence chloroplast movement. However, long wavelengths of red light can hinder movement rather than enhancing it.
- Blue light typically induces chloroplasts to seek shelter, while red light can encourage them to spread out to maximize light absorption.
- Microfilament Involvement:
- Research on species like Vallisneria gigantea, an aquatic flowering plant, has revealed that chloroplast movement begins within minutes of light exposure.
- Initially, the movement may not exhibit a clear directionality, but it is associated with the use of microfilament tracks within the cell.
- Microfilaments are involved in creating an intricate honeycomb-like structure around chloroplasts as they move, potentially aiding in anchoring them in the desired positions.
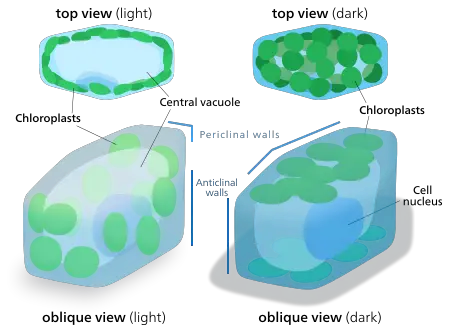
The photosynthetic machinery of chloroplasts
The photosynthetic machinery of chloroplasts is a highly complex and meticulously organized system responsible for converting light energy into chemical energy stored in the form of sugars. This intricate process can be divided into two main stages: the light reactions and the dark reactions, also known as the Calvin cycle.
Light Reactions:
- Thylakoid Membranes: The light reactions take place within the thylakoid membranes of chloroplasts, where specialized pigment molecules, including chlorophyll, are embedded.
- Energy Carriers: The primary goal of the light reactions is to capture light energy and convert it into chemical energy stored in energy carriers. These carriers include adenosine triphosphate (ATP) and nicotinamide adenine dinucleotide phosphate (NADPH).
- Photophosphorylation: Similar to mitochondria, chloroplasts utilize an H+ ion gradient to generate ATP through a process known as photophosphorylation. Light energy energizes electrons extracted from water molecules, creating an electron transport chain. The transport chain pumps hydrogen ions (H+) into the thylakoid space, creating a concentration gradient. These ions then flow back into the stroma through ATP synthase, driving ATP synthesis.
- NADP+ Reduction: Electrons from the transport chain are used to reduce NADP+ to NADPH. NADPH is an essential electron carrier in the subsequent dark reactions.
- Cyclic Photophosphorylation: In certain cases, electrons can flow back down electron transport chains, generating more ATP through cyclic photophosphorylation. This process is particularly common in C4 plants that require more ATP than NADPH.
Dark Reactions (Calvin Cycle):
- Stroma: The Calvin cycle, or dark reactions, takes place in the stroma of the chloroplast, which is the fluid-filled region surrounding the thylakoid membranes.
- Carbon Fixation: The cycle begins with the enzyme ribulose-1,5-bisphosphate carboxylase/oxygenase (RuBisCO) fixing carbon dioxide (CO2) into five-carbon Ribulose bisphosphate (RuBP) molecules. These molecules are initially unstable and break down into three-carbon molecules called 3-phosphoglyceric acid (3-PGA).
- G3P Synthesis: ATP and NADPH generated in the light reactions are utilized to convert 3-PGA into glyceraldehyde-3-phosphate (G3P), a three-carbon sugar molecule. Most G3P molecules are recycled to regenerate RuBP using additional ATP, while a fraction exits the cycle as the end product of photosynthesis.
- Sugars and Starches: G3P molecules can combine to form larger sugar molecules like glucose and fructose, which can further assemble into sucrose. Additionally, glucose monomers within the chloroplast can link together to create starch, which accumulates as starch grains.
- Photorespiration: In cases of high oxygen concentration, the enzyme RuBisCO can mistakenly incorporate oxygen instead of CO2 into RuBP, leading to a wasteful process called photorespiration. Several mechanisms, such as C4 carbon fixation and Crassulacean acid metabolism, have evolved in different plant lineages to mitigate photorespiration and enhance photosynthetic efficiency.
In summary, the photosynthetic machinery of chloroplasts is a highly orchestrated process involving the capture of light energy, generation of energy carriers (ATP and NADPH), carbon fixation, and the synthesis of sugars. This remarkable system enables plants to convert light and atmospheric CO2 into the essential energy and organic compounds needed for their growth and survival.
Chloroplast genome and membrane transport
The chloroplast genome and membrane transport are integral components of the intricate cellular machinery responsible for the functioning of these organelles. Here, we explore the characteristics of the chloroplast genome and the crucial role of membrane transport in chloroplast physiology:
- Chloroplast Genome:
- The chloroplast genome is a distinctive feature of these organelles, characterized by its typically circular shape (though linear versions have been observed).
- The size of the chloroplast genome varies but can range from 120 to 200 kilobases (kb) in length.
- However, it’s important to note that the modern chloroplast genome has undergone significant reduction and simplification over evolutionary time.
- Many chloroplast genes have been transferred to the nucleus of the cell during evolution, leading to the dependency of chloroplasts on nuclear-encoded proteins for their function.
- Nuclear-Encoded Proteins:
- As a consequence of gene transfer to the nucleus, nuclear-encoded proteins have become essential for the proper functioning of chloroplasts.
- These nuclear-encoded proteins are synthesized in the cytoplasm and then need to be transported into the chloroplast to fulfill their specific roles.
- The outermost membrane of the chloroplast is relatively permeable to small molecules and contains transmembrane channels that facilitate the entry of various molecules, including nuclear-encoded proteins.
- Inner Chloroplast Membrane:
- In contrast to the outer membrane, the inner membrane of the chloroplast is highly selective and restricts the transport of molecules.
- Transport across the inner membrane is tightly regulated and specific, with only certain proteins, such as nuclear-encoded ones, being equipped to pass through transmembrane channels.
In summary, the chloroplast genome has evolved over time, with many genes being transferred to the cell nucleus. This evolution has resulted in the dependence of chloroplasts on nuclear-encoded proteins for their essential functions. Membrane transport within chloroplasts is a critical process that allows for the entry of nuclear-encoded proteins and other molecules necessary for chloroplast function. While the outer membrane is relatively permeable, the inner membrane is more selective and tightly controls the transport of specific molecules, contributing to the precise regulation of chloroplast processes.
Chloroplasts Division
Chloroplast division is a highly regulated and intricate process essential for the replication and distribution of chloroplasts within plant and algal cells. Here, we delve into the scientific details of chloroplast division:
- Origin of Chloroplasts:
- Chloroplasts in photosynthetic cells do not directly originate from etioplasts or proplastids.
- Typically, a shoot meristematic cell contains 7 to 20 proplastids that undergo division to yield the 30 to 70 chloroplasts found in mature photosynthetic plants.
- When a cell divides, chloroplast division ensures the equitable distribution of chloroplasts between the two daughter cells.
- Chloroplast Division in Single-Celled Algae:
- In single-celled algae, chloroplast division is the primary mechanism for generating new chloroplasts.
- These algae lack proplastid differentiation, so when the cell divides, its chloroplast also divides, and each daughter cell inherits a mature chloroplast.
- Chloroplast Replication in Multi-Celled Plants:
- Chloroplasts in multi-celled plants, including higher plants like Arabidopsis, can divide independently of the cell cycle.
- Unlike nuclear DNA, chloroplast DNA replication is not synchronized with the cell’s replication cycle.
- Initiation of Chloroplast Division:
- Chloroplast division commences with the formation of filaments by two proteins, FtsZ1 and FtsZ2, along with the assistance of the ARC6 protein, resulting in the creation of Z-rings within the chloroplast’s stroma.
- The positioning of Z-rings is regulated by the Min system, ensuring even division.
- MinD prevents the formation of FtsZ filaments, while MinE allows Z-rings to develop at the chloroplast’s center.
- Plastid-Dividing Rings (PD Rings):
- Two plastid-dividing rings, the inner and outer PD rings, are formed during chloroplast division.
- The inner PD ring is located on the inner side of the chloroplast’s internal membrane, while the outer PD ring encircles the outer chloroplast membrane.
- Constriction of the chloroplast begins as these rings squeeze it.
- Additional Division Structures:
- In some species like Cyanidioschyzon merolae, an extra plastid-dividing ring exists in the chloroplast’s intramembrane space, contributing to division.
- Final Phase of Division:
- In the last phase of constriction, dynamin proteins form a ring around the outer PD ring, aiding in chloroplast separation.
- The Z-ring and inner PD rings disassemble, and chloroplast DNA plasmids are distributed to the daughter chloroplasts.
- Dynamin proteins cleave the chloroplast into two daughter chloroplasts, with a small portion of the outer division ring remaining suspended between them.
- Role of Division Rings:
- Among the rings involved in chloroplast division, only the outer plastid-dividing ring persists throughout the constriction phase.
- Constriction does not commence until the formation of the surrounding plastid-dividing ring.
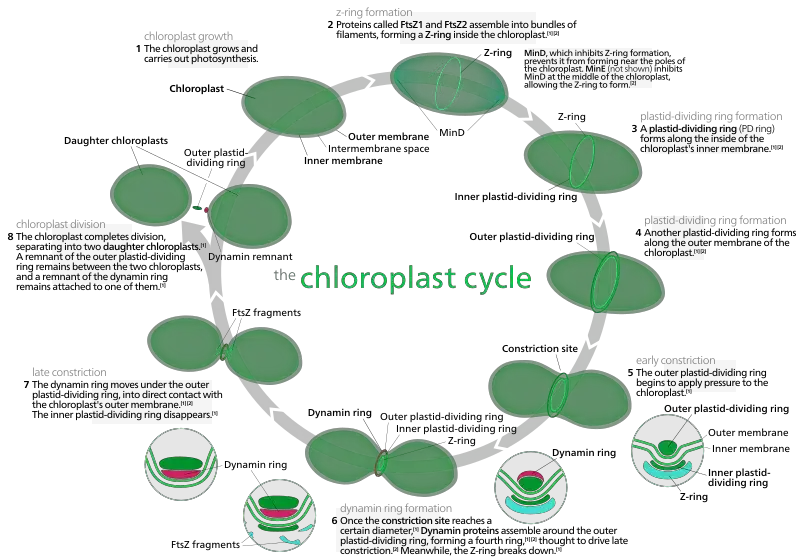
How are mitochondria and chloroplasts similar?
Mitochondria and chloroplasts, two essential organelles within eukaryotic cells, exhibit striking similarities despite their distinct roles and functions. Here are some key points highlighting their commonalities:
- Presence in Eukaryotic Cells:
- Both mitochondria and chloroplasts are membrane-bound organelles found exclusively in eukaryotic cells. They are absent in prokaryotic cells.
- Energy Production:
- Mitochondria are renowned as the “powerhouses” of the cell due to their primary role in energy production. They generate the majority of ATP, the cell’s energy currency, through oxidative phosphorylation.
- Chloroplasts, on the other hand, play a pivotal role in the photosynthetic process. They convert light energy into chemical energy stored in the form of organic compounds like glucose.
- Endosymbiotic Origin:
- Both organelles are believed to have originated from free-living bacteria that were engulfed by early eukaryotic cells. This theory, known as the endosymbiotic theory, explains the presence of their own DNA and certain structural features.
- Independence and Reproduction:
- Mitochondria and chloroplasts possess their own DNA and the machinery required for replication. They can reproduce independently within the cell.
- This autonomy is a relic of their bacterial ancestors, as free-living bacteria replicate autonomously.
- Double Membrane Structure:
- Both organelles exhibit a double membrane structure. The outer membrane likely originated from the engulfing cell’s membrane during endosymbiosis.
- The inner membrane, on the other hand, is thought to have evolved from the plasma membrane of the ancestral bacteria that were engulfed.
- Similarities in Membrane Transport:
- Both mitochondria and chloroplasts have specialized protein channels and transport systems that facilitate the movement of molecules across their membranes.
- These transport systems are essential for their respective metabolic processes, such as electron transport chains and ATP synthesis.
Facts about chloroplasts
- Chloroplasts are organelles found in eukaryotic cells, primarily those of plants and some algae.
- Photosynthesis, the process by which plants and some algae transform light energy into chemical energy in the form of organic compounds, is mediated by chloroplasts.
- During photosynthesis, chloroplasts contain pigments called chlorophylls that are responsible for the absorption of light energy.
- It is believed that the double membrane structure of chloroplasts is the result of their genesis as free-living photosynthetic bacteria that were ingested by eukaryotic cells.
- Similar to bacterial DNA and ribosomes, chloroplasts have their own DNA and ribosomes. This is believed to be another consequence of their origin as free-living microorganisms.
- Chloroplasts are extremely dynamic organelles capable of modifying their shape, size, and number in response to various environmental signals.
- In addition to photosynthesis, chloroplasts are involved in a vast array of metabolic processes, including the synthesis of amino acids, lipids, and secondary metabolites.
- It is believed that chloroplasts played a crucial role in the evolution of life on Earth by creating oxygen during photosynthesis, a crucial component in the genesis of aerobic respiration.
- Chloroplasts can coordinate cellular operations by communicating with organelles such as the nucleus and mitochondria.
- Chloroplasts are prone to damage from environmental stresses such as high light intensity, drought, and temperature extremes; thus, plants have evolved a variety of defensive mechanisms to limit this damage.
Quiz
FAQ
Do animal cells have chloroplasts? are chloroplasts found in animal cells?
No, because animals are heterotrophic , they cannot prepare their own food. They directly or indirectly depend on plant for food.
What do chloroplasts do?
Chloroplasts are plant cell organelles that convert light energy into relatively stable chemical energy via the photosynthetic process.
Which of the following are common traits of chloroplasts and mitochondria?a. Both are surrounded by a single membrane.b. Both reproduce by meiosis.c. Both have their own DNA.d. Both are found in plant and animal cells.e. Proteins for both are synthesized on ribosomes in the rough ER.
Ans: c. Both have their own DNA.
Explanation:
Both chloroplast and mitochondria are semi-autonomous organelles which means that they both have their own DNA. Both of these organelles have a circular DNA and make some of their own proteins by themselves. Both are double membrane-bound organelles. Chloroplast has a third membrane system called thylakoid.
Chloroplasts are found in the plant cells only as they are the site for photosynthesis. Mitochondria are present in both plant and animal cells and are the site for cellular respiration to produce energy to support the vital functions of cells.
Where are chloroplasts found?
Chloroplasts are present in the cells of all green tissues of plants and algae. Chloroplasts are also found in photosynthetic tissues that do not appear green, such as the brown blades of giant kelp or the red leaves of certain plants. In plants, chloroplasts are concentrated particularly in the parenchyma cells of the leaf mesophyll (the internal cell layers of a leaf).
Do chloroplasts have dna?
Each chloroplast contains a single DNA molecule present in multiple copies. The number of copies varies between species; however, the pea chloroplasts from mature leaves normally contain about 14 copies of the genome. There can be in excess of 200 copies of the genome per chloroplast in very young leaves.
Why are chloroplasts green?
Chloroplasts are green because they contain the pigment chlorophyll, which is vital for photosynthesis. Chlorophyll occurs in several distinct forms. Chlorophylls a and b are the major pigments found in higher plants and green algae.
Which statements are true for chloroplasts? select the three that apply.?they are the sites of reactions that convert chemical energy from food molecules to atp.they have membranous sacs called thylakoids that are surrounded by a fluid called stroma.their matrix contains enzymes that function in cellular respiration.they are the sites of reactions that convert solar energy into chemical energy.their inner membrane has infoldings called cristae.they contain the green pigment chlorophyll?
Ans:
They are the sites of reactions that convert solar energy into chemical energy.
-They contain the green pigment chlorophyll.
-They have membranous sacs called thylakoids that are surrounded by a fluid called stroma.Explanation; -Chloroplasts are organelles found in plant cells, that work to convert light energy of the Sun into sugars that can be used by cells. The entire process is called photosynthesis and it all depends on the little green chlorophyll molecules in each chloroplast.-The chloroplasts have inner and outer membrane with an empty intermediate space in between. Inside the chloroplast are stacks of thylakoids, called grana, as well as stroma, the dense fluid inside of the chloroplast. Thylakoids contain the chlorophyll, which is a green pigment that is necessary for the plant to go through photosynthesis.
Photosynthesis is to chloroplasts as cellular respiration is to…a.chloroplasts b. glucose c. mitochondria d. lactic acid
Ans: mitochondria
Do prokaryotes have chloroplasts?
Prokaryotic cells have no chloroplasts or mitochondria. Despite this, many of them can do aerobic respiration of the same type that mitochondria do. Some can do photosynthesis the way chloroplasts do.
Do fungi have chloroplasts?
Fungi are multicellular,with a cell wall, organelles including a nucleus, but no chloroplasts.
What does the chemiosmotic process in chloroplasts involve?
The chemiosmotic process in chloroplasts involves a proton gradient across the thylakoid membrane.
What is the likely origin of chloroplasts?
The most widely accepted theory about the origin of chloroplasts is the endosymbiotic theory. According to this theory, chloroplasts are thought to have originated from free-living photosynthetic bacteria that were engulfed by a eukaryotic cell and eventually developed a symbiotic relationship.
The endosymbiotic theory suggests that a eukaryotic cell engulfed a photosynthetic prokaryotic cell and formed a symbiotic relationship with it. Over time, the prokaryotic cell evolved into the modern-day chloroplast, which is now an organelle within eukaryotic cells.
Evidence supporting the endosymbiotic theory includes similarities between chloroplasts and photosynthetic bacteria in terms of structure, biochemistry, and genetic material. Chloroplasts also have their own DNA and ribosomes, which are similar to those found in bacteria.
Overall, the endosymbiotic theory is widely accepted as the most likely explanation for the origin of chloroplasts.
Do cyanobacteria have chloroplasts?
No, cyanobacteria do not have chloroplasts in the same sense as eukaryotic cells. Chloroplasts are specialized organelles found in eukaryotic cells, particularly in plant cells, which are believed to have originated from the endosymbiosis of photosynthetic bacteria.
Cyanobacteria, on the other hand, are a type of prokaryotic organism that are capable of photosynthesis. They contain specialized structures called thylakoids where the photosynthetic process takes place. While thylakoids are similar in structure to chloroplasts, they are not the same thing.
It is worth noting that cyanobacteria are sometimes referred to as “blue-green algae” due to their similar appearance to algae, which are eukaryotic organisms that do have chloroplasts. However, it’s important to remember that cyanobacteria are prokaryotic, and not eukaryotic like algae.
What observation led researchers to propose that chloroplasts evolved from cyanobacteria?A) Both produce proteins.B) Both contain mitochondria.C) Both provide structure to cells.D) Both perform photosynthesis.
Ans: (D) Both perform photosynthesis is the observation that led researchers to propose that chloroplasts evolved from cyanobacteria.
Both mitochondria and chloroplasts _____.a. use an H+ gradient to produce ATPb. obtain electrons from waterc. release oxygen as a by-productd. reduce NAD+, forming NADP
Ans: a. use an H+ gradient to produce ATP
Do bacteria have chloroplasts?
No, bacteria do not have chloroplasts. Chloroplasts are specialized organelles found in eukaryotic cells, particularly in plant cells and some algae. They are believed to have originated from the endosymbiosis of photosynthetic bacteria that were engulfed by eukaryotic cells and eventually evolved into specialized organelles.
Bacteria, on the other hand, are a type of prokaryotic organism that do not have organelles like chloroplasts. Some bacteria are capable of photosynthesis, such as cyanobacteria, which have specialized structures called thylakoids where photosynthesis takes place. However, these structures are not the same as chloroplasts.
It’s important to note that while bacteria and eukaryotic cells are fundamentally different in terms of their cellular structure and organization, they both play important roles in the functioning of living organisms. Bacteria, for example, are involved in many important processes such as nutrient cycling, decomposition, and nitrogen fixation, while eukaryotic cells are involved in a wide range of functions including energy production, protein synthesis, and cell division.
References
- Finkeldey, R. (2013). Brenner’s Encyclopedia of Genetics || Chloroplasts. , (), 525–527. doi:10.1016/B978-0-12-374984-0.00231-X
- Rascio, N. (2013). Encyclopedia of Biological Chemistry || Chloroplasts. , (), 506–510. doi:10.1016/b978-0-12-378630-2.00141-9
- PARK, RODERIC B. (1966). The Chlorophylls || Chloroplast Structure. , (), 283–311. doi:10.1016/b978-1-4832-3289-8.50015-1
- Staehelin, L. A. (2003). Chloroplast structure: from chlorophyll granules to supra-molecular architecture of thylakoid membranes. Photosynthesis Research, 76(1–3), 185–196. https://doi.org/10.1023/A:1024994525586
- Discovery of Chloroplast DNA, Genomes and Genes | Discoveries in Plant Biology. (2019). Worldscientific.Com. https://www.worldscientific.com/doi/abs/10.1142/9789812813046_0002
- Jensen, P. E., & Leister, D. (2014). Chloroplast evolution, structure and functions. F1000Prime Reports, 6. https://doi.org/10.12703/p6-40
- Evidence for endosymbiosis. (2020). Berkeley.Edu. https://evolution.berkeley.edu/evolibrary/article/_0_0/endosymbiosis_04
- Cooper GM. The Cell: A Molecular Approach. 2nd edition. Sunderland (MA): Sinauer Associates; 2000. Chloroplasts and Other Plastids. Available from: https://www.ncbi.nlm.nih.gov/books/NBK9905/
- Alberts B, Johnson A, Lewis J, et al. Molecular Biology of the Cell. 4th edition. New York: Garland Science; 2002. Chloroplasts and Photosynthesis. Available from: https://www.ncbi.nlm.nih.gov/books/NBK26819/
- https://micro.magnet.fsu.edu/cells/chloroplasts/chloroplasts.html
- https://www.toppr.com/guides/biology/cell-the-unit-of-life/chloroplast-definition-structure-functions/
- https://www.nagwa.com/en/explainers/812162636509/
- https://www.twinkl.co.in/teaching-wiki/chloroplast
- https://platoonline.com/chloroplasts
- https://www.britannica.com/science/chloroplast
- https://www.sciencedirect.com/topics/medicine-and-dentistry/chloroplast
- https://flexbooks.ck12.org/cbook/ck-12-biology-flexbook-2.0/section/2.19/primary/lesson/chloroplasts-bio/
- https://www.sciencefacts.net/chloroplast.html
미토콘드리아는 어머니를 통해, 다른 생명에 전달되는데, 독립 세포인 엽록체는 어떻게 다음 세대 나무에게 이동하나요?
미토콘드리아는 실제로 인간을 포함한 대부분의 유기체에서 어머니에서 자손으로 전달됩니다. 이 과정은 엽록체를 포함하는 식물과 기타 광합성 유기체에서는 약간 다릅니다.
엽록체는 미토콘드리아와 마찬가지로 식물과 조류의 세포에서 발견되는 세포 소기관입니다. 그들은 빛 에너지가 화학 에너지로 변환되는 과정인 광합성을 담당합니다. 엽록체의 유전은 종에 따라 달라질 수 있습니다.
대부분의 식물에서 엽록체는 동물의 미토콘드리아와 유사하게 모계를 통해 유전됩니다. 이는 엽록체가 일반적으로 모식물의 밑씨에 있는 난세포를 통해 자손에게 전달된다는 것을 의미합니다. 수정 과정에서 (아버지 식물에서 나온) 꽃가루의 정자 세포는 핵 DNA만 접합체에 제공하고 엽록체와 같은 세포소기관에는 제공하지 않습니다. 결과적으로 자손의 엽록체는 일반적으로 모식물의 엽록체와 유전적으로 동일합니다.
그러나 이 규칙에는 예외가 있습니다. 일부 식물에서는 엽록체가 아버지 또는 부모 모두로부터 물려받을 수 있습니다. 엽록체의 이러한 부계 또는 양부모 유전은 덜 일반적이지만 특정 종에서 관찰되었습니다.