The transport of ions across the cell membrane is a fundamental process necessary for maintaining cellular homeostasis. The plasma membrane, acting as a selectively permeable barrier, allows certain substances to enter or leave the cell, ensuring proper balance between the intracellular and extracellular environments. This selectivity is crucial for the regulation of essential molecules such as glucose and ions, while keeping metabolic intermediates inside the cell and expelling waste products. Without this selective permeability, cells would not be able to regulate their internal environment, leading to loss of homeostasis and eventual cell death.
The structure of the plasma membrane plays a significant role in its selective permeability. The membrane is primarily composed of a lipid bilayer, based on the fluid-mosaic model. This bilayer consists of phospholipids, each with a hydrophilic head and two hydrophobic tails. These molecules align themselves tail-to-tail, creating a hydrophobic core that prevents polar or charged molecules from passing through easily. While small non-polar molecules can diffuse through the lipid bilayer, ions and polar molecules require assistance due to their inability to pass through the hydrophobic barrier.
Membrane proteins play a critical role in facilitating the transport of ions and other polar molecules across the cell membrane. In simple diffusion, substances move down their concentration gradient without the need for energy input. However, ions and larger molecules often require more complex mechanisms, especially when moving against concentration or electrochemical gradients. This is where active transport comes into play, a process that involves the use of energy, usually in the form of ATP, to “pump” ions across the membrane.
For instance, ions like sodium (Na+), potassium (K+), and chloride (Cl-) are transported across the membrane through specific ion channels and transport proteins. The concentration of these ions differs between the intracellular and extracellular environments, with extracellular fluid having a higher concentration of Na+ and Cl- and a lower concentration of K+. This difference is maintained through the activity of transport mechanisms such as the sodium-potassium pump, which moves Na+ out of the cell and K+ into the cell, utilizing ATP for energy.
In some cases, energy for transport may not come directly from ATP but from the movement of another solute. This process is known as secondary active transport, where the movement of one solute down its electrochemical gradient drives the movement of another solute against its gradient. This mechanism is common in the transport of ions and molecules like glucose, which are coupled with ion gradients for efficient cellular uptake.
Therefore, the transport of ions across the cell membrane is a complex but essential process that ensures the proper functioning of cells. Through both passive and active transport mechanisms, the cell maintains the necessary ion gradients for metabolic processes, signal transduction, and overall cellular health.
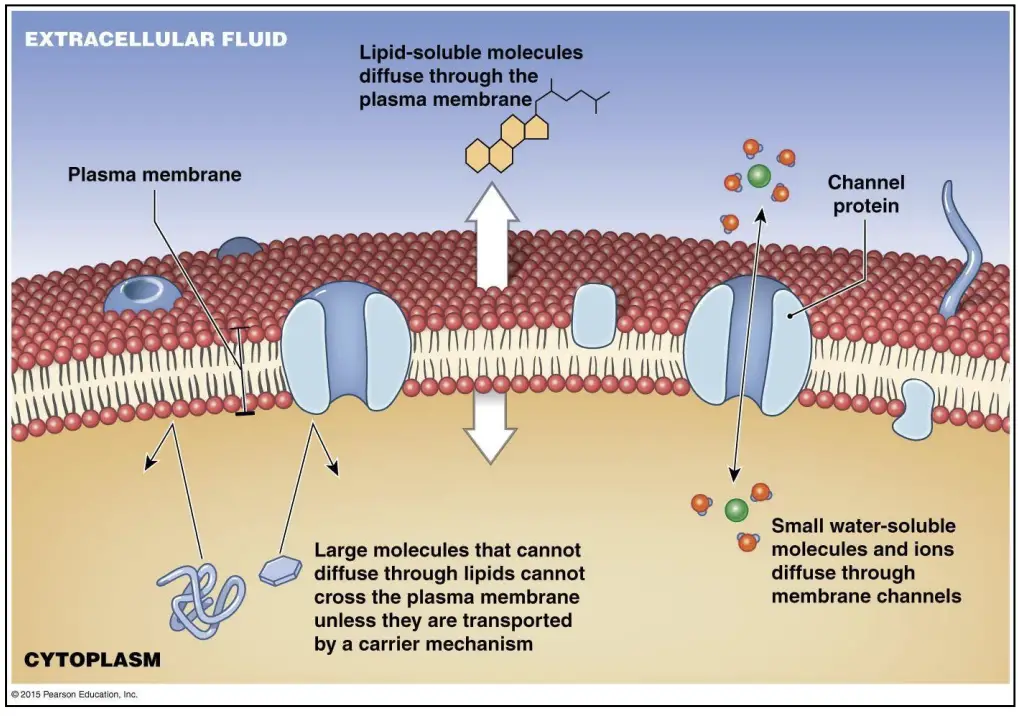
Membrane transport system
The membrane transport system governs the movement of molecules into and out of cells across the plasma membrane. Cells utilize different transport mechanisms to regulate this movement, depending on factors such as the type of molecule, its size, whether energy is required, and the method by which it crosses the membrane. The transport system can be divided into two main categories: Passive Transport and Active Transport.
A. Passive transport
Passive transport refers to the movement of ions, molecules, or other atomic substances across a cell membrane without the need for energy input from the cell. This process depends on the permeability of the membrane and the concentration gradient of the molecules involved. The following are the main types of passive transport:
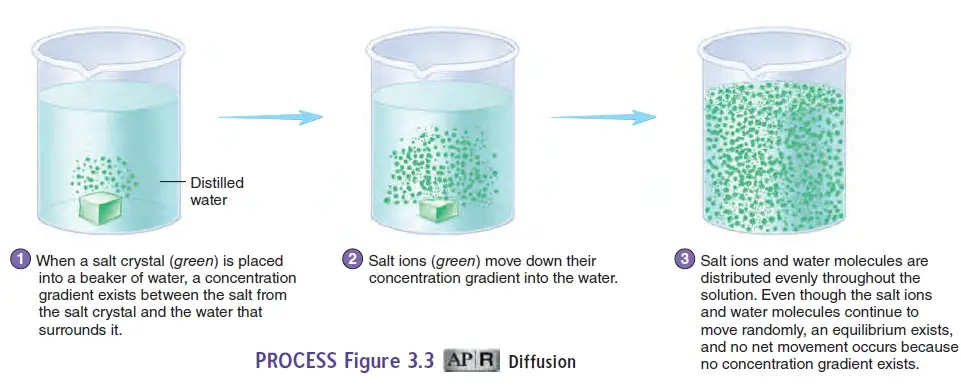
1. Simple Diffusion
- Definition: The movement of molecules from a region of higher concentration to a region of lower concentration until equilibrium is reached.
- Mechanism: Molecules move directly through the lipid bilayer of the membrane without assistance from membrane proteins.
- Examples: Water, oxygen (O₂), carbon dioxide (CO₂), and nitrogen (N₂) are typical molecules that move through simple diffusion.
- Factors Influencing Simple Diffusion:
- Concentration Gradient: The greater the difference in concentration between two regions, the faster the diffusion.
- Membrane Permeability: Higher permeability leads to faster diffusion.
- Function: Essential for the transport of small, non-polar molecules across the membrane.
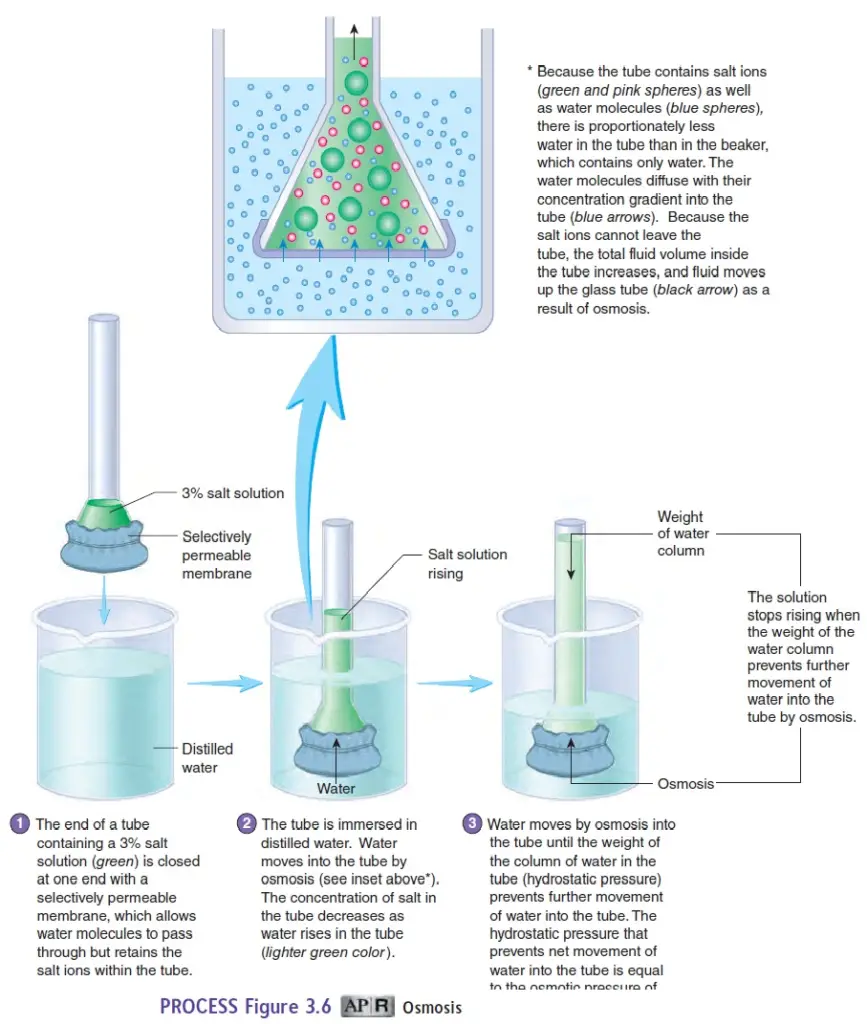
2. Facilitated Diffusion
- Definition: The movement of larger or polar molecules across the membrane via specific membrane proteins, without energy input.
- Mechanism: Involves transport proteins (carrier or channel proteins) that help molecules cross the membrane.
- Examples: Glucose, amino acids, and ions such as Na⁺ and K⁺.
- Key Features:
- Specificity: Each transporter protein is specific to the molecule it helps transport.
- Carrier Proteins: These proteins undergo a conformational change when binding with the transported molecule, allowing it to pass through.
- Channel Proteins: Create a pore through which molecules move.
- Function: Facilitated diffusion is crucial for moving molecules that cannot diffuse freely through the lipid bilayer due to their size or polarity.
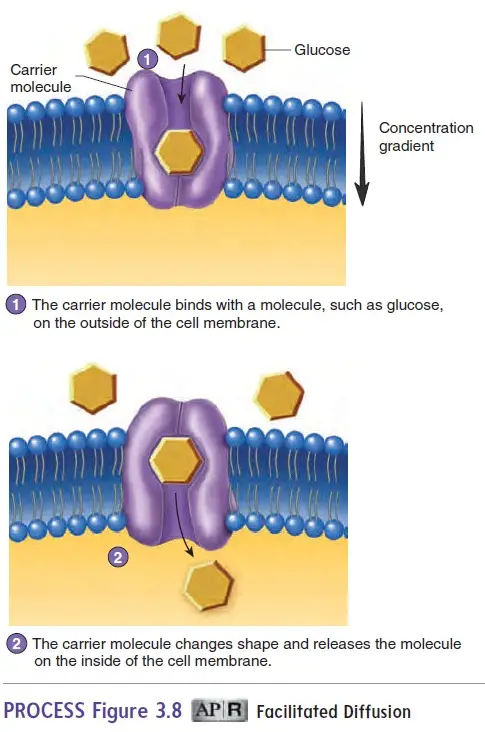
3. Osmosis
- Definition: The movement of water molecules across a semi-permeable membrane from an area of higher water potential (lower solute concentration) to an area of lower water potential (higher solute concentration).
- Mechanism: Water moves through the membrane or via water channels called aquaporins.
- Types of Solutions:
- Isotonic Solution: Water moves in and out of the cell at equal rates, resulting in no change in cell volume.
- Hypotonic Solution: Water enters the cell, causing it to swell and possibly burst (lysis).
- Hypertonic Solution: Water exits the cell, leading to cell shrinkage (plasmolysis).
- Function: Osmosis helps maintain the balance of fluids in and around cells, essential for cell survival and function.
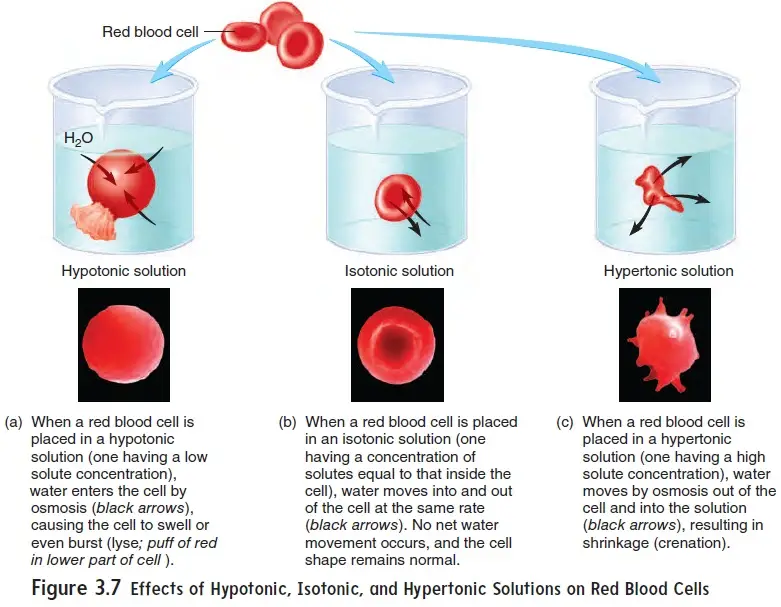
4. Filtration
- Definition: The process by which molecules are pushed through a membrane due to pressure differences across the membrane.
- Mechanism: Water and small solutes pass through the pores of the membrane, while larger molecules are retained.
- Example: Filtration occurs in the kidneys, where blood pressure forces small molecules such as water, salts, and urea through the membrane during urine formation.
- Function: Filtration is essential for separating waste products from the bloodstream and helps regulate body fluid balance.
Factors Influencing Passive Transport
- Concentration Gradient: The rate of diffusion increases with a steeper gradient.
- Molecule Size: Smaller molecules diffuse faster than larger ones.
- Temperature: Higher temperatures increase the kinetic energy of molecules, leading to faster diffusion.
- Membrane Surface Area: A larger surface area facilitates a higher rate of diffusion.
- Membrane Thickness: Thinner membranes allow for faster diffusion.
A. Active transport
Active transport is the process of moving molecules across a cell membrane against their concentration gradient, from regions of low concentration to high concentration. Unlike passive transport, active transport requires energy input, typically derived from adenosine triphosphate (ATP), to facilitate this movement. This process is essential for maintaining concentration gradients of ions and molecules that are crucial for cellular functions.
Types of Active Transport
Active transport is broadly classified into two types:
- Primary Active Transport
- Secondary Active Transport
1. Primary Active Transport
- Definition: Primary active transport directly uses the energy released from ATP hydrolysis to transport molecules across the membrane. This process moves substances from areas of lower concentration to higher concentration.
- Mechanism:
- The energy from ATP is used to change the conformation of transporter proteins, which actively pump specific ions or molecules across the membrane.
- One key example is the Sodium-Potassium (Na+/K+) Pump, which helps maintain the electrochemical gradient in cells.
- The pump binds to three sodium ions from inside the cell.
- ATP is hydrolyzed, and the energy released causes the protein to change its shape, releasing sodium ions outside the cell.
- Two potassium ions then bind to the altered protein, which reverts to its original shape, moving the potassium ions into the cell.
- This process results in the movement of three sodium ions out of the cell for every two potassium ions pumped in, which helps regulate the cell’s potential.
- Examples:
- Sodium-Potassium Pump: Maintains cellular electrochemical gradients.
- Calcium Pumps: Move calcium ions (Ca²⁺) from the cytosol into the extracellular fluid or organelles like the endoplasmic reticulum.
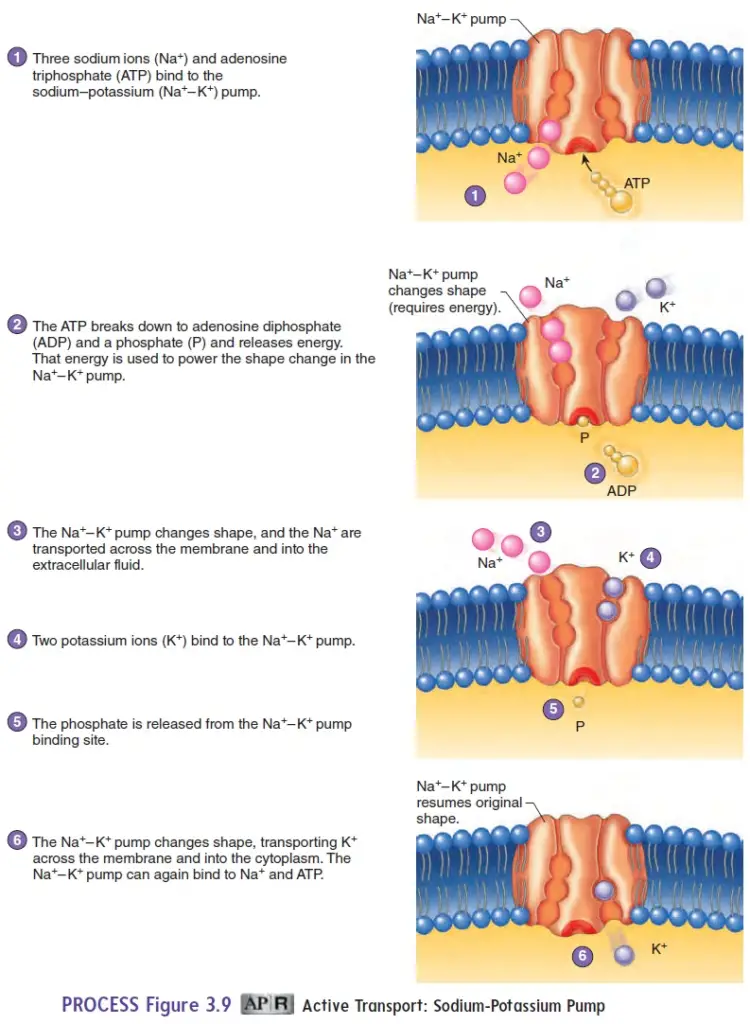
2. Secondary Active Transport
- Definition: Secondary active transport, or co-transport, does not use ATP directly. Instead, it uses the energy stored in the form of ionic gradients created by primary active transport. The movement of one molecule down its concentration gradient provides the energy to move another molecule against its concentration gradient.
- Mechanism:
- The process relies on the electrochemical potential difference generated by ion gradients (often sodium or hydrogen ions).
- The transport proteins involved in secondary active transport can move two molecules simultaneously.
- Types of Secondary Active Transport:
- Symport: Both molecules move in the same direction. For example, glucose and sodium ions can be co-transported into a cell together via a symporter protein.
- Antiport: The molecules move in opposite directions. An example is the exchange of calcium ions (Ca²⁺) and sodium ions (Na⁺), where calcium is moved out of the cell as sodium enters.
- Examples:
- Glucose-Sodium Symport: Glucose enters the cell against its gradient by coupling with sodium, which moves down its gradient.
- Calcium-Sodium Antiport: Sodium moves into the cell while calcium is pumped out, helping regulate intracellular calcium levels.
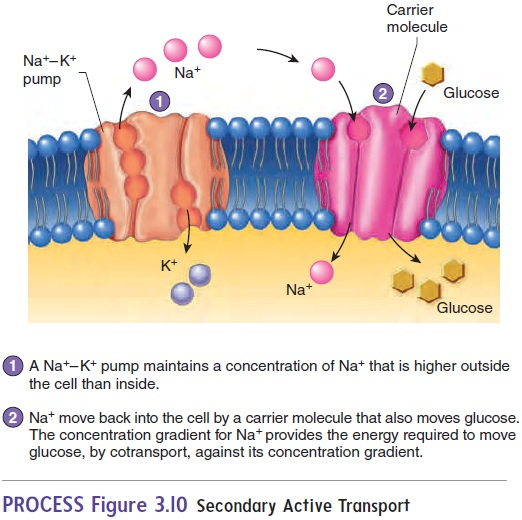
Functions of Active Transport
- Maintaining Ion Gradients: Active transport systems such as the Na+/K+ pump maintain vital ion gradients across the cell membrane, which are essential for processes like nerve impulse transmission and muscle contraction.
- Nutrient Absorption: In the intestines, active transport enables the absorption of nutrients, such as glucose, even when concentrations are higher inside the cells than in the intestinal lumen.
- Regulation of Cell Volume: By controlling the movement of ions, active transport helps regulate osmotic pressure and, consequently, cell volume.
Summary of Active Transport Mechanisms
Type | Energy Source | Molecule Direction | Example |
---|---|---|---|
Primary Active Transport | Direct ATP Hydrolysis | Moves molecules against the gradient using ATP | Sodium-Potassium Pump |
Secondary Active Transport | Electrochemical Gradient | Couples movement of one molecule down its gradient to move another against its gradient | Glucose-Sodium Symporter, Calcium-Sodium Antiporter |
Vesicle mediated Transport across cell membrane
Vesicle-mediated transport is essential for cellular processes that move substances across cell membranes in membrane-bound sacs called vesicles. This mechanism is crucial for transporting large molecules, small particles, and even entire cells. Vesicles, formed by the fluidity of cell membranes, allow substances to move in or out of the cell via two main processes: endocytosis and exocytosis.
1. Endocytosis
Endocytosis refers to the process where a cell engulfs substances from the external environment by forming vesicles. There are three key types of endocytosis, each playing a distinct role in cellular uptake:
- Phagocytosis (Cell Eating):
- This involves the ingestion of large particles, such as bacteria or cell debris.
- The cell membrane extends around the target particle, eventually enveloping it in a vesicle.
- The vesicle then moves into the cytoplasm, where the ingested material is broken down.
- Example: White blood cells use phagocytosis to engulf and destroy harmful pathogens.
- Pinocytosis (Cell Drinking):
- In this process, cells take in extracellular fluid along with dissolved substances.
- Small vesicles are formed, containing liquid rather than solid particles.
- Pinocytosis is less selective compared to phagocytosis and is a general way for cells to sample their environment.
- Receptor-Mediated Endocytosis:
- This highly specific form of endocytosis involves receptors on the cell surface binding to particular molecules.
- Once the molecule binds to its receptor, the cell membrane folds inward, forming a vesicle that transports the molecule into the cell.
- Example: Cholesterol uptake by cells occurs through receptor-mediated endocytosis.
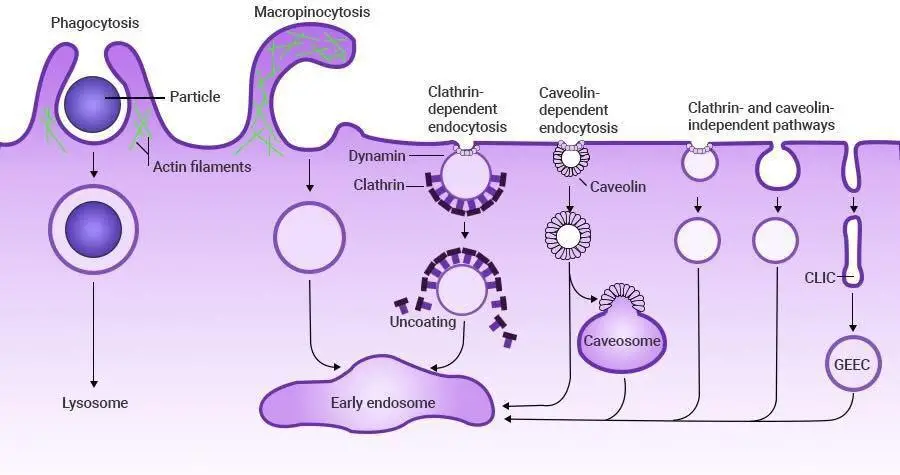
2. Exocytosis
Exocytosis is the process through which cells expel substances by vesicle fusion with the plasma membrane. This method is essential for eliminating waste, secreting hormones, and releasing digestive enzymes. Key points of exocytosis include:
- Secretory Vesicles:
- These vesicles accumulate materials like enzymes or hormones within the cell.
- The vesicle moves toward the cell membrane, where it fuses and releases its contents outside the cell.
- Example: The pancreas secretes digestive enzymes via exocytosis, and salivary glands release mucus.
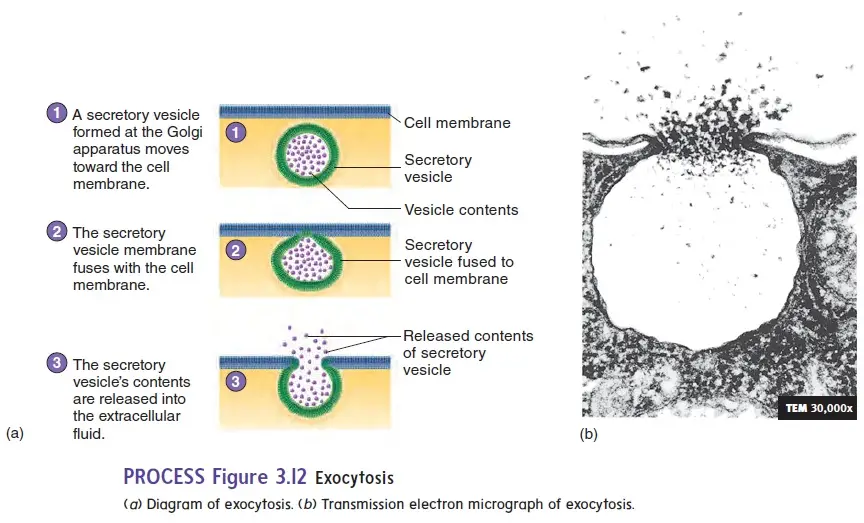
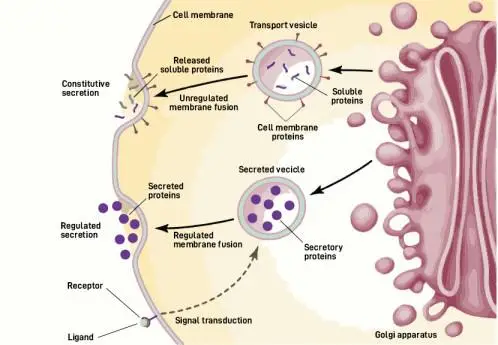
Factors Influencing Transport Across Cell Membrane
The primary factors include concentration gradients, molecular size, lipid solubility, and the charge of molecules. Each of these factors plays a distinct role in determining the efficiency and mode of transport.
1. Gradient Concentration
- High to Low Gradient Transport: Generally, molecules move from areas of high concentration to areas of low concentration. This process, known as passive transport, does not require energy. The driving force behind this movement is the concentration gradient, which naturally facilitates the equal distribution of molecules.
- Low to High Gradient Transport: In contrast, when molecules move from a low concentration to a high concentration, this process is termed active transport. Active transport necessitates energy, typically in the form of adenosine triphosphate (ATP), to move substances against their concentration gradient.
2. Size of Molecules
- Smaller Molecules: The rate of transport is significantly influenced by the size of the molecules. Smaller molecules can diffuse more rapidly through the cell membrane due to their reduced resistance and ease of movement across the lipid bilayer.
- Larger Molecules: Larger molecules face greater difficulty penetrating the cell membrane. They may require specialized mechanisms, such as facilitated diffusion or endocytosis, to traverse the membrane efficiently. This is due to the size of the pores and channels available in the membrane and the need for specific transport proteins.
3. Lipid Solubility
- Lipid-Soluble Molecules: Molecules that are lipid-soluble, such as steroids and certain vitamins, can diffuse directly through the lipid bilayer of the cell membrane. This is because the lipid bilayer is hydrophobic, and lipid-soluble substances can easily dissolve in and pass through this hydrophobic core.
- Hydrophilic Molecules: In contrast, hydrophilic molecules generally cannot pass through the lipid bilayer directly and require transport proteins or channels to facilitate their movement.
4. Charge of Molecules
- Charged Molecules: Molecules with an electrical charge (ions) cannot freely diffuse through the lipid bilayer due to its hydrophobic nature. These charged particles require specific transport proteins or ion channels to facilitate their movement across the membrane.
- Carrier Proteins: Carrier proteins, including those involved in facilitated diffusion and active transport, bind to these charged molecules and assist in their transit across the membrane. This process ensures that essential ions and molecules can be transported despite their inability to cross the lipid bilayer directly.
Different Carriers of Membrane
Membranes can have various types of carriers and transport proteins that facilitate the movement of substances across the cell membrane. Here are some key types:
- Channel Proteins: These proteins form pores in the membrane, allowing specific ions or molecules to pass through. Examples include voltage-gated ion channels and aquaporins (for water).
- Carrier Proteins: These proteins bind to specific molecules and undergo a conformational change to shuttle the molecules across the membrane. Examples include glucose transporters (e.g., GLUT1) and amino acid transporters.
- Transporters: These are often used interchangeably with carrier proteins, but can also refer to proteins involved in active transport. They can be uniporters (moving one type of molecule), symporters (moving two types of molecules in the same direction), or antiporters (moving two types of molecules in opposite directions). Examples include the sodium-potassium pump (Na+/K+ ATPase) and the sodium-glucose transport protein (SGLT).
- Pumps: These are a type of active transporter that use energy, usually from ATP hydrolysis, to move substances against their concentration gradient. Examples include the Na+/K+ pump and the calcium pump (Ca2+ ATPase).
- Facilitated Diffusion Proteins: These proteins help larger or polar molecules pass through the membrane without using energy. They include both channel proteins and carrier proteins.
- Active Transport Proteins: These proteins move substances against their concentration gradient using energy. They include pumps like the proton pump (H+ ATPase) and the calcium pump.
Different Channels and pumps of Membrane
Here is a detailed overview of the different types of channels and pumps found in cell membranes:
Channels
- Ion Channels
- Voltage-Gated Ion Channels: These channels open or close in response to changes in the membrane’s electrical potential. They are critical for the generation and propagation of action potentials in neurons and muscle cells.
- Example: Voltage-gated sodium channels are crucial for the rapid depolarization phase of action potentials.
- Ligand-Gated Ion Channels: These channels open in response to the binding of specific ligands (e.g., neurotransmitters). They play a key role in synaptic transmission and cellular signaling.
- Example: The nicotinic acetylcholine receptor, which opens in response to acetylcholine binding, allowing sodium ions to enter the cell.
- Mechanically-Gated Ion Channels: These channels respond to mechanical stress, such as stretch or pressure applied to the cell membrane, and are involved in mechanosensation.
- Example: Stretch-activated ion channels in sensory neurons detect mechanical changes in tissues.
- Leak Channels: These channels are always open, allowing ions to flow across the membrane according to their electrochemical gradients. They contribute to the resting membrane potential and overall ionic balance.
- Example: Potassium leak channels help maintain the resting membrane potential by allowing potassium ions to exit the cell.
- Voltage-Gated Ion Channels: These channels open or close in response to changes in the membrane’s electrical potential. They are critical for the generation and propagation of action potentials in neurons and muscle cells.
- Aquaporins
- Aquaporins: Specialized channels that facilitate the rapid movement of water molecules across the membrane. They are crucial for maintaining water balance and osmotic regulation in cells.
- Example: Aquaporin-1 (AQP1) is found in red blood cells and kidney cells, enabling efficient water transport.
- Aquaporins: Specialized channels that facilitate the rapid movement of water molecules across the membrane. They are crucial for maintaining water balance and osmotic regulation in cells.
Pumps
- Primary Active Transport Pumps
- Sodium-Potassium Pump (Na+/K+ ATPase): This pump uses ATP to transport three sodium ions out of the cell and two potassium ions into the cell, creating and maintaining electrochemical gradients essential for various cellular functions.
- Function: Maintains cellular volume, membrane potential, and provides the gradient necessary for secondary active transport.
- Proton Pump (H+ ATPase): This pump moves hydrogen ions (protons) out of the cell or into organelles, contributing to the acidification of the extracellular space or organelle lumen.
- Example: The proton pump in the stomach lining helps maintain acidic pH for digestion.
- Sodium-Potassium Pump (Na+/K+ ATPase): This pump uses ATP to transport three sodium ions out of the cell and two potassium ions into the cell, creating and maintaining electrochemical gradients essential for various cellular functions.
- Secondary Active Transport Pumps
- Sodium-Calcium Exchanger (NCX): Utilizes the sodium gradient established by the sodium-potassium pump to extrude calcium ions from the cell, playing a vital role in regulating intracellular calcium levels.
- Function: Maintains low intracellular calcium concentrations, which is crucial for various cellular processes, including muscle contraction and neurotransmitter release.
- Sodium-Glucose Cotransporter (SGLT): Uses the sodium gradient to transport glucose into cells against its concentration gradient. This cotransporter is essential for nutrient uptake in the intestines and kidneys.
- Function: Facilitates glucose absorption from the gut and reabsorption in the kidneys.
- Sodium-Calcium Exchanger (NCX): Utilizes the sodium gradient established by the sodium-potassium pump to extrude calcium ions from the cell, playing a vital role in regulating intracellular calcium levels.
- Vesicular Transport Pumps
- Vesicular ATPase (V-ATPase): This pump acidifies intracellular vesicles, such as lysosomes and endosomes, by transporting protons into their lumen. This acidification is necessary for the activation of digestive enzymes and other vesicular functions.
- Function: Essential for maintaining the acidic environment of organelles involved in digestion and receptor recycling.
- Vesicular ATPase (V-ATPase): This pump acidifies intracellular vesicles, such as lysosomes and endosomes, by transporting protons into their lumen. This acidification is necessary for the activation of digestive enzymes and other vesicular functions.
Importance of Transport of Ions across cell membrane
The transport of ions across the cell membrane is crucial for a variety of cellular functions and overall physiological processes. Here are some key reasons why this transport is important:
- Maintaining Electrochemical Gradients: Ion transport helps establish and maintain electrochemical gradients across the cell membrane. For example, the sodium-potassium pump (Na+/K+ ATPase) maintains a high concentration of potassium ions (K+) inside the cell and a high concentration of sodium ions (Na+) outside the cell, which is essential for various cellular functions.
- Nerve Impulse Transmission: In neurons, the movement of ions such as sodium (Na+) and potassium (K+) across the cell membrane is critical for generating and propagating action potentials. Changes in the ion concentrations lead to electrical signals that are transmitted along nerve cells.
- Muscle Contraction: Muscle cells rely on ion transport for contraction. Calcium ions (Ca2+) play a key role in initiating muscle contraction by interacting with proteins in muscle fibers. The regulation of calcium ion concentrations within muscle cells is essential for proper muscle function.
- Cell Volume Regulation: Ion transport helps regulate cell volume by controlling osmotic pressure. For instance, the movement of ions like sodium and potassium affects the osmotic balance of the cell, preventing excessive swelling or shrinking.
- pH Regulation: The transport of ions such as hydrogen ions (H+) helps regulate the pH of the cell and its surroundings. This is important for maintaining the proper pH environment for enzymatic and metabolic processes.
- Nutrient Uptake and Waste Removal: Ion transport can drive secondary active transport processes that move nutrients into the cell and remove waste products. For example, the sodium gradient established by the sodium-potassium pump can be used to transport glucose into the cell via co-transporters.
- Signal Transduction: Ions can act as signaling molecules within the cell. For instance, changes in intracellular calcium levels can activate various signaling pathways and influence cellular responses.
- Maintaining Cell Integrity: Proper ion balance is crucial for maintaining the structural and functional integrity of the cell membrane and its associated structures.
- Wang, Yizhou & Blatt, Mike & Chen, Zhong-Hua. (2018). Ion Transport at the Plant Plasma Membrane. 10.1002/9780470015902.a0001307.pub3.
- Cooper GM. The Cell: A Molecular Approach. 2nd edition. Sunderland (MA): Sinauer Associates; 2000. Transport of Small Molecules. Available from: https://www.ncbi.nlm.nih.gov/books/NBK9847/
- http://eagri.org/eagri50/PPHY261/lec04.pdf
- https://www.onlinebiologynotes.com/membrane-transport-system-passive-active-transport/
- https://magadhmahilacollege.org/wp-content/uploads/2020/08/Transport-across-plasma-membrane.pdf
- https://www.shcollege.ac.in/wp-content/uploads/NAAC_Documents_IV_Cycle/Criterion-II/2.3.2/ppt/Dr_VidhuVV_DiffusionandOsmosis.pdf
- https://www.geeksforgeeks.org/transport-across-cell-membrane/
- https://www.studocu.com/in/document/jinnah-university-for-women/physiology/transport-across-cell-membrane-physio-notes/4244460/download/transport-across-cell-membrane-physio-notes.pdf
- https://thebiologyprimer.com/diffusion-and-osmosis
- https://rwu.pressbooks.pub/bio103/chapter/membrane-transport/
- https://derangedphysiology.com/main/cicm-primary-exam/required-reading/cellular-physiology/Chapter%20112/transport-substances-across-cell-membranes
- https://www.slideshare.net/MANISHSAHU106/ion-transport-through-cell-membrane
- https://chem.libretexts.org/Courses/American_River_College/CHEM_309%3A_Applied_Chemistry_for_the_Health_Sciences/11%3A_Lipids_-_An_Introduction/11.08%3A_Transport_Across_Cell_Membrane
- https://rwu.pressbooks.pub/bio103/chapter/membrane-transport/